X-Ray Powder Diffraction
This handout provides background on the use and theory of X-ray powder diffraction. Examples of applications of this method to geologic studies are provided.
Introduction
Rocks, sediments, and precipitates are examples of geologic materials that are composed of minerals. Numerous analytical techniques are used to characterize these materials. One of these methods, X-ray powder diffraction (XRD), is an instrumental technique that is used to identify minerals, as well as other crystalline materials. In many geologic investigations, XRD complements other mineralogical methods, including optical light microscopy, electron microprobe microscopy, and scanning electron microscopy. XRD provides the researcher with a fast and reliable tool for routine mineral identification. XRD is particularly useful for identifying fine-grained minerals and mixtures or intergrowths of minerals, which may not lend themselves to analysis by other techniques. XRD can provide additional information beyond basic identification. If the sample is a mixture, XRD data can be analyzed to determine the proportion of the different minerals present. Other information obtained can include the degree of crystallinity of the mineral(s) present, possible deviations of the minerals from their ideal compositions (presence of element substitutions and solid solutions), the structural state of the minerals (which can be used to deduce temperatures and (or) pressures of formation), and the degree of hydration for minerals that contain water in their structure. Some mineralogical samples analyzed by XRD are too fine grained to be identified by optical light microscopy. XRD does not, however, provide the quantitative compositional data obtained by the electron microprobe or the textural and qualitative compositional data obtained by the scanning electron microscope.
Theory and Methodology
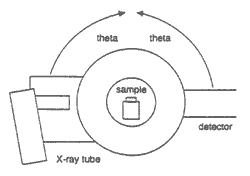
Figure 1. Simplified sketch of one possible configuration of the X-ray source (X-ray tube), the X-ray detector, and the sample during an X-ray scan. In this configuration, the X-ray tube and the detector both move through the angle theta ( ), and the sample remains stationary.
|
The three-dimensional structure of nonamorphous materials, such as minerals, is defined by regular, repeating planes of atoms that form a crystal lattice. When a focused X-ray beam interacts with these planes of atoms, part of the beam is transmitted, part is absorbed by the sample, part is refracted and scattered, and part is diffracted. Diffraction of an X-ray beam by a crystalline solid is analogous to diffraction of light by droplets of water, producing the familiar rainbow. X-rays are diffracted by each mineral differently, depending on what atoms make up the crystal lattice and how these atoms are arranged.
In X-ray powder diffractometry, X-rays are generated within a sealed tube that is under vacuum. A current is applied that heats a filament within the tube; the higher the current the greater the number of electrons emitted from the filament. This generation of electrons is analogous to the production of electrons in a television picture tube. A high voltage, typically 15-60 kilovolts, is applied within the tube. This high voltage accelerates the electrons, which then hit a target, commonly made of copper. When these electrons hit the target, X-rays are produced. The wavelength of these X-rays is characteristic of that target. These X-rays are collimated and directed onto the sample, which has been ground to a fine powder (typically to produce particle sizes of less than 10 microns). A detector detects the X-ray signal; the signal is then processed either by a microprocessor or electronically, converting the signal to a count rate. Changing the angle between the X-ray source, the sample, and the detector at a controlled rate between preset limits is an X-ray scan (figs. 1 and 2).
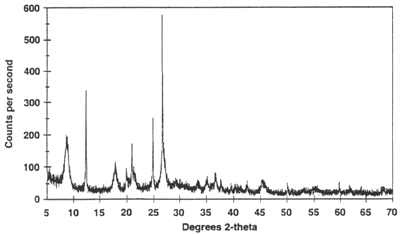
Figure 2. Example of an X-ray powder diffractogram produced during an X-ray scan. The peaks represent positions where the X-ray beam has been diffracted by the crystal lattice. The set of d-spacings (the distance between adjacent planes of atoms), which represent the unique "fingerprint" of the mineral, can easily be calculated from the 2-theta (2 ) values shown. The use of degrees 2-theta in depicting X-ray powder diffraction scans is a matter of convention, and can easily be related back to the geometry of the instrument, shown in figure 1. The angle and the d-spacings are related by Bragg's Law, as described in the text.
|
When an X-ray beam hits a sample and is diffracted, we can measure the distances between the planes of the atoms that constitute the sample by applying Bragg's Law. Bragg's Law is:
where the integer n is the order of the diffracted beam,
is the wavelength of the incident X-ray beam, d is the distance between adjacent planes of atoms (the d-spacings), and
is the angle of incidence of the X-ray beam. Since we know
and we can measure
, we can calculate the d-spacings. The geometry of an XRD unit is designed to accommodate this measurement (fig. 1). The characteristic set of d-spacings generated in a typical X-ray scan provides a unique "fingerprint" of the mineral or minerals present in the sample. When properly interpreted, by comparison with standard reference patterns and measurements, this "fingerprint" allows for identification of the material.
Applications
XRD has a wide range of applications in geology, material science, environmental science, chemistry, forensic science, and the pharmaceutical industry, among others. At the U.S. Geological Survey, researchers use XRD to characterize geologic materials from a wide variety of settings; below are just a few examples.
Mineral-Environmental Studies
In studies of areas affected by acid mine drainage, the identification of secondary minerals and fine-grained precipitates is a critical element. Acid is generated when iron sulfide minerals, such as pyrite, weather. Elements derived from the alteration of the sulfide minerals can form secondary minerals or go into solution. Elements that go into solution may form mineral precipitates as conditions (temperature, acidity, solution composition) change. Accurate mineralogical characterization of the precipitates and secondary minerals, together with hydrogeochemical data, help us to better understand the solubility, transport, and storage of metals.
Ore Genesis Studies
Minerals form under specific ranges of temperature and pressure. Mineralogical identification of ore minerals and associated minerals, including fine-grained hydrothermal alteration minerals, provides evidence used to deduce the conditions under which ore deposits formed and the conditions under which, in many cases, they were subsequently altered.
Predictive Stratigraphic Analysis
Mineralogical characteristics of paleosols (ancient buried soil horizons) and underclays (the fine-grained detrital material lying immediately beneath a coal bed) have been instrumental in correlating coal zones from the Appalachian basin into the Western Interior basin. They have been the key to quantifying the paleolatitudinal climate gradient in North America during the late Middle Pennsylvanian.
Remote-Sensing Studies
Mineralogical analysis by XRD is used in conjunction with remotely sensed data in several research investigations. XRD is used to identify the minerals composing clay-rich, hydrothermally altered rocks that occur on several Cascade volcanoes. Such rocks are believed to play an important role in the generation of large landslides and mudflows. XRD is used to analyze saline minerals, including borates. Many saline hydrate minerals produce diagnostic spectral bands, and spectral data provide a basis for mineral exploration using remote-sensing data. Analysis of airborne imaging spectrometer data can directly map mineral occurrences by detecting diagnostic spectral absorption bands, the shape and position of which are determined by individual mineral structures. A detailed knowledge of sample mineralogy, provided at least in part by XRD, is required to understand the observed spectral absorption features.
Genesis of Coal Beds
XRD is one of the primary tools used to evaluate the lateral and vertical variations in mineral matter and major, minor, and trace elements in coal beds. These data are used to help determine the impact of geologic and geochemical processes on coal bed formation in order to understand and predict both inorganic and organic variability within and among minable coal beds.
Mineral-Resource Assessments
Mineralogical characterization provides part of the data required to determine the particular kind of mineral deposits encountered in mineral-resource assessment studies. XRD allows us to identify fine-grained mixtures of minerals found in associated gangue and alteration assemblages, which cannot be resolved by other methods.
- Prepared by Marta J.K. Flohr
For more information, please contact:
Frank T. Dulong
Eastern Energy Resources Team
U.S. Geological Survey, MS 956
Reston, VA 20192
(703) 648-6416
fdulong@usgs.gov
John C. Jackson
Eastern Mineral Resource Surveys Team
U.S. Geological Survey, MS 954
Reston, VA 20192
(703) 648-6321
jjackson@usgs.gov
|
This page is https://pubs.usgs.gov/info-handout/diffraction/html/
Maintained by Eastern Publications Group Web Team
Last revised 1-28-00