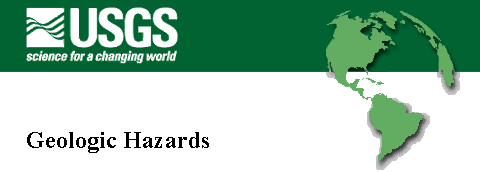
PRE-1980 TEPHRA-FALL DEPOSITS ERUPTED FROM MOUNT ST. HELENS, WASHINGTON
By Donal R. Mullineaux
U. S. Geological Survey Professional Paper 1563
A study of the stratigraphy, age, distribution, and characteristics of more than 100 tephra-fall
deposits erupted during the past 40,000 years.
INTRODUCTION
Mount St. Helens in Washington State (figs. 1,
2) has been one of the most frequently active and most explosive volcanoes in the Cascade
Range. Within the past 40,000 years it has erupted more than 100 tephra deposits now recognizable as distinct strata.
Its repeated eruptions have also produced abundant fragmental flowage deposits, lava flows, and domes. The fragmental
flowage deposits are described in a comprehensive report by Crandell (1987). The present report describes the other
major category of explosively erupted deposits, variously called tephra-fall, air-fall, or pyroclastic-fall deposits,
or simply tephra. As used here, the word tephra describes only fall deposits, thus excluding deposits that resulted
from pyroclastic flows, pyroclastic surges, and their accompanying ash clouds.

Figure 1. Mount St. Helens from the northeast in 1964 showing relatively smooth pre-1980 profile and
fan of debris that dammed preexisting valleys to form Spirit Lake (in the foreground). Letters D, S, and G designate
the Dogs Head, Sugar Bowl, and Goat Rocks domes. (88K)
|
Strata described in this report record only some of the eruptions that have
produced tephra in the past. They record only those eruptions that were large enough to produce widespread strata
that have been preserved well enough to be recognized and traced. Among the tephra deposits recognized, those of
relatively small to moderate volume far outnumber those of larger volume. Many other eruptions, which left deposits
too small or too disturbed to be recognized in this study, also must have occurred. Thus, more tephra-producing
eruptions surely occurred than are now recorded by the deposits described herein.
Both the distribution and physical character of the tephra strata demonstrate
that they are fall deposits. The deposits originated from eruptions that projected volcanic detritus up into the
air, where it commonly was carried laterally before falling back to the ground. The deposits relatively uniformly
blanket diverse terrain and are present as remnants on widely separated ridge crests; such distributions show that
the deposits dropped down through the air. The strata also are either widespread, coarse, or thick enough to ensure
that they are not local deposits reworked by wind from preexisting strata. Each stratum described was identified
in multiple outcrops, each except the upper layers of set X contains fragments of lapilli size or larger, and each
is, at least locally, more than 1 cm thick.
Almost all tephra strata described herein are separated from each other by
ash of mostly undetermined origin. Many of those ash beds probably are also tephra, ejected during early or late
stages of eruptions that produced the coarser layers or during separate minor eruptive pulses. They probably also
include some ash of pyroclastic-surge, ash-cloud, and eolian origin. A few ash beds are distinctive and widespread
enough to be useful for correlation and are described as parts of the stratigraphic sequence (see table
4). Tephra strata are also commonly interbedded with coarser pyroclastic-flow, mudflow, and colluvial deposits.
Recognition of the tephra sequence is based mainly on observed stratigraphic
superposition. Unless otherwise noted, the relative stratigraphic position of each tephra unit was established
by overlapping field relations. If the stratigraphic relation of two strata was not observed, the best estimate
of that relation is stated and the absence of overlap noted.
These deposits provide important information about kinds, frequency, and magnitude
of eruptions and about probable effects. Their thicknesses and distributions indicate that similar eruptions in
the future would endanger life and property over large areas. An assessment of those potential hazards and hazards
from other eruptive products was a principal goal of this study and that of Crandell (1987). These two studies
describe data that were the basis of a pre-1980 assessment of volcanic hazards at Mount St. Helens (Crandell and
Mullineaux, 1978).

Figure 2. Location of Mount St. Helens relative to some nearby volcanoes in the Cascade Range of southern
Washington and northern Oregon. Modified from Doukas (1990). (21K)
|
The tephras also provide information about compositional variations in magmas
under Mount St. Helens. The tephra sequence records both gradual and abrupt changes of mineralogical and chemical
composition within and between successive eruptive periods. It records, for example, the appearance of mafic andesite
that marks the beginning of the modern Mount St. Helens. Other changes that followed, including numerous variations
in composition among andesite, dacite, and basalt, are also recorded.
Many tephra deposits are so widespread that they are especially useful as time-stratigraphic
marker beds that allow correlation and dating of associated deposits from one location to another. Near Mount St.
Helens, many distinctive tephra units can be identified in wide arcs around the volcano, allowing volcanic and
other kinds of flowage deposits to be correlated from one valley to another. In addition, many individual layers
are distinctive enough to be used as markers hundreds of kilometers from the volcano. The voluminous layer Yn in
set Y, for example, forms a single, thick stratum recognizable to the north-northeast past Mount Rainier National
Park (Crandell and others, 1962; Mullineaux, 1974) and into Canada (Fulton and Armstrong, 1965; Westgate and others,
1970). Similarly, layers Wn and We of set W extend northeast and east of the volcano across Washington State into
Canada and Idaho, respectively (Smith and others, 1977). Because they are dated, the tephras provide ages as well
as traceable time horizons for a variety of geologic and other scientific studies.
The value of several tephras as markers is enhanced by the fact that they contain
distinctive mineral suites. In the Pacific Northwest, cummingtonite has been found in ferromagnesian mineral suites
of volcanic ejecta from only Mount St. Helens, and thus is virtually diagnostic of a Mount St. Helens origin. Some
Mount St. Helens tephras contain other uncommon components, such as iron-rich hypersthene phenocrysts whose refractive
index is high enough to discriminate them from hypersthene in other tephras.
Terminology
Tephra
The term "tephra" refers to particles that were erupted into the
air and then fell back to the ground or to deposits of those particles. The term was introduced by Thorarinsson
(1944, 1954) to describe volcanic ash and coarser detritus that were projected through the air. Deposits of pyroclastic
flows were not included. Later, Thorarinsson (1974) expanded the term to include pyroclastic-flow materials. As
such, tephra would describe virtually all pyroclastic material and be nearly synonomous with pyroclastic and pyroclastic
deposit. In this report, the term "tephra" is used in the earlier, more restricted sense in order
to differentiate the deposits thus described from deposits of pyroclastic flowage events. As used here, tephra
includes the materials and deposits resulting from events described as tephra fall, air fall, and pyroclastic fall
but not deposits resulting from flowage events.
Most particles in Mount St. Helens tephra deposits were transported by wind.
Some large ballistic fragments, however, were little affected by wind and fell very near the source vent. In contrast,
many wind-carried deposits form elongate, lobate blankets far downwind from the volcano that become progressively
thinner and their particles finer with increasing distance. They also become thinner and finer from their long
axes toward the sides of the lobes.
Tephra is classified chiefly by clast size, shape, vesicularity, and composition.
Particles whose intermediate axes measure 2 mm or less are described as ash (table 1). Fine
and coarse ash particles are smaller and larger than l/l6 mm across, respectively. Lapilli have intermediate axes
from 2 to 64 mm in length, and blocks and bombs are more than 64 mm wide. Bombs have shapes or textures such as
vesicularity that indicate they were liquid or plastic when erupted. Blocks generally are more angular and were
solid when erupted.

Tephra clasts from Mount St. Helens are composed mostly of vesicular glass;
pale clasts are called pumice and darker ones scoria. Pumice and scoria clasts record eruption of new magma; that
is, they represent rock material that was molten when erupted and that expanded into a froth before solidifying.
The terms "pumice" and "scoria" are used both for highly vesicular particles and for some particles
that are only moderately vesicular and have specific gravities greater than 1.
All tephra deposits from Mount St. Helens contain some lithic fragments that
are dense or only slightly vesicular rock composed of glass and minerals. Tephras commonly also contain discrete
mineral crystals and fragments of such crystals.
Compositions of Mount St. Helens tephras range from dacitic to basaltic. Compositional
terms used are chiefly field names, based on color and ferromagnesian mineral suites. If chemical compositions
are known, names are as recommended by Le Bas and others (1986). Most pumice is dacitic or andesitic, and all the
scoria is andesitic or basaltic.
Sets, layers, and beds
The tephra sequence is subdivided into sets, layers, and beds, based chiefly
on evidence of elapsed time, differences in composition, and differences in grain size. Evidence of elapsed time
between deposition of strata generally consists of oxidation or other soil development or deposition of intervening
strata. Compositional differences may be recognizable in the field by differences in color and ferromagnesian phenocryst
suites. Mineral content is especially useful: criteria such as grain size, thickness, vesicularity, color, and
evidence of elapsed time can vary significantly from one outcrop to another, but ferromagnesian mineral suites
are almost constant for specific strata.
Tephra sets are groups of strata that are similar in age and can be separated
from older and younger deposits by composition or evidence of elapsed time. Each set records an episode of more
than one eruption. A tephra layer consists of one or more beds that can be traced as a stratigraphic unit, even
though each bed might not be individually traceable. Units described as beds are strata that were observed locally
but not traced laterally. Layers and beds record different eruptions within episodes represented by sets.
Color
Colors are described in word terms from the Rock-Color Chart distributed
by the National Research Council (Goddard and others, 1948) or the Munsell Soil Color Charts (Munsell Color Company,
Inc., 1954). Names alone are used, rather than names and(or) the more specific color notations. Use of names alone
describes a general rather than specific color, which is appropriate for these tephra deposits. The color of the
tephras varies greatly according to local conditions, especially in the important hues of yellow and red, even
with the depth one excavates into a cutbank. In contrast, the relative hues and strength of colors tend to be more
consistent. Thus, pumice layers of set S are consistently lighter in color and less reddish than similar layers
in set J, both where the tephras are strongly colored and where they are pale.
Letter designations
Letters used to name tephra strata are derived mostly from words used for
field descriptions and do not follow an alphabetical or other regular pattern. Layer We, for example, is white
and extends east of the volcano. Many of these designations have been defined in various earlier reports of D.R.
Crandell, J.H. Hyde, and D.R. Mullineaux.
Thickness
The tephra strata show strong variation in thickness, over distances of
less than a meter as well as over longer distances (see fig 3). Some of that variation developed
as the tephra fell and represents primary differences from the average thickness of tephra that fell. Such differences
can result from a variety of factors such as downslope sloughing during fall, being carried down by rain, shielding
of ground next to tree trunks, excess accumulation between trees by shedding of detritus from tree limbs (Waitt
and Dzurisin, 1981, p. 607), and flocculation of particles (Sorem, 1982) into larger (and thus faster falling)
clasts (Carey and Sigurdsson, 1982).
Secondary variations in thickness developed after tephra falls have ended result
chiefly from thinning or removal on the one hand and excess accumulation on the other. Erosion by water and ice,
creep, and more rapid landsliding cause major variations. A long list of other factors are known to cause differences
that are lesser but distinct. Animal burrowing and tree-root throw are especially notable near Mount St. Helens.
As expected, the greatest variations in thickness are associated with slopes.
Slopes commonly are stripped or thinned of a deposit. Coarse particles, especially pumice, tend to collect and
form thick deposits near their bases. Fine particles, in contrast, commonly are blown up slopes that are steep
and bare and form anomolously thick deposits near their tops.
Except in measured sections, thicknesses presented in this report represent
my estimate of average thickness for the deposit, determined where possible from multiple outcrops. Their main
purpose is to facilitate correlation and identification.
Deposits of ash clouds and other pyroclastic flowage phenomena
Deposits of ash clouds derived from pyroclastic flows (Crandell and Mullineaux,
1973) are interbedded with tephra in almost every set recognized at Mount St. Helens. In addition, tephra strata
are interbedded with deposits that originated from pyroclastic flows and other events called pyroclastic surges,
base surges, and pyroclastic density flows. In general, those deposits are more variable in thickness and grain
size than the tephra strata and less traceable. In this report, ash deposits that appear to have been formed by
any kind of pyroclastic fragment-and-gas flow, whether directly from the flow itself or from elutriate clouds rising
above it, are described as either ash-cloud deposits or ash of flowage origin.
Oxidized ash-rich deposits of undetermined origin
Poorly sorted, massive to faintly bedded, ash-rich deposits that commonly
are 50-200 cm thick are present between all the tephra sets of Pleistocene age. These deposits contain abundant
volcanic glass particles and ferromagnesian minerals and scattered pumice lapilli. They are finer grained, more
coherent, and more oxidized than most of the recognized tephras; many form protruding ledges in outcrops. These
deposits commonly are widespread enough to serve as stratigraphic markers between the Pleistocene tephra sets.
In most places, the oxidized ash-rich deposits are mostly massive and have
been strongly disturbed by near-surface turnover within the soil zone. Locally, faint bedding shows a sequence
of thin depositional units. In some deposits between tephras having different ferromagnesian mineral composition,
scattered lapilli were sampled to compare with lapilli in the tephras. Some of these lapilli have ferromagnesian
mineral compositions similar to those of the tephra below the ash-rich deposit, whereas others have compositions
similar to those of the tephra above. It is likely that lapilli from the tephras have mixed both upward and downward
into the intervening ash-rich deposit.
These ash-rich deposits probably resulted from a variety of small-magnitude
events including tephra eruptions, flowage phenomena, and eolian reworking of older deposits. Eruptions that contributed
to the deposits probably were of low volume and low frequency. Consequently, individual deposits were thin, and
considerable time ensued between their emplacement. During that time, near-surface turnover disrupted most evidence
of stratification before overlying deposits were laid down, resulting in the generally massive character of the
deposits.
Direction and distance from Mount St. Helens
Direction and distance reported herein are measured from a presumed central
vent of Mount St. Helens, unless otherwise stated. Because of the volcano's size, most tephra that is within about
4 km of that center is on its flanks. Most deposits, however, were carried much farther downwind. Wind-carried
tephra is distributed in all directions from Mount St. Helens, although most was blown into the easterly quadrant
from northeast to southeast of the volcano center.
Ferromagnesian minerals
Ferromagnesian phenocrysts are prominent constituents in Mount St. Helens
rocks. They are especially useful for identification and correlation of tephra units, chiefly because the kinds
and abundances of ferromagnesian phenocrysts can differ markedly from one tephra unit to another. Commonly, the
ferromagnesian mineral assemblage alone allows reliable identification of a set or even a layer. Within some sets,
however, identification of specific layers or beds by mineral content is not feasible because the mineral suites
are too similar among their strata. Nevertheless, mineral suites used in conjunction with other evidence such as
stratigraphic and textural characteristics are adequate to identify and correlate most tephra strata. Ferromagnesian
minerals were used rather than other phenocrysts because they are more readily separated and identified and because
ferromagnesian mineral assemblages vary enough to be effective as criteria for identification.
Ferromagnesian mineral suites in Mount St. Helens tephras consist almost entirely
of two or more of the following: cummingtonite, hornblende, hypersthene, augite, olivine, and biotite. In most
dacitic tephras the suites consist mostly of a pair of minerals, either cummingtonite and hornblende or hypersthene
and hornblende. The distinction of cummingtonite from hypersthene is critical and reliably discriminates between
many otherwise similar tephras. Augite, biotite, and even olivine are present but less abundant in dacitic tephras.
Augite and olivine, however, are predominant in the more mafic tephras.
In this investigation, ferromagnesian mineral phenocrysts were studied as crushed
fragments rather than in thin section. The fragment-study method allows rapid preparation of samples and determination
of mineral identities. Moreover, the great number of fragments made available by crushing facilitates estimation
of relative mineral abundances as well as discovery of minerals that are present in small amounts. Crushing of
about 1 cm3 of Mount St. Helens pumice, for example, typically produces several thousand fragments of
ferromagnesian minerals suitable for study.
Most ferromagnesian mineral samples were prepared by crushing lumps of pumice,
scoria, or denser rock, and separating heavy- from light-mineral fractions. In the field, this was done by crushing
the lumps with a small mortar and pestle, then washing out the light fraction with water in the mortar. Although
the resulting heavy fraction contains much glass and plagioclase, the ferromagnesian minerals can be concentrated
enough to identify most of them. In the laboratory, samples were crushed, screened to obtain a desired size fraction
(usually 1/16-1/8 mm), and separated in a heavy liquid. A brief rinsing of ferromagnesian mineral samples in hydrofluoric
acid removed adhering glass and facilitated identifications.
Mineral suites were examined first with a binocular microscope, with which
thousands of grains can be viewed in a single field. Next, several representatives of each mineral species that
could be segregated by color, shape, and cleavage were picked up individually on spindle-stage needles and examined
in index oils using a spindle stage (Wilcox, 1959a; Bloss, 1981) and a petrographic microscope. Identification
of each ferromagnesian mineral species noted for each layer or bed was confirmed using the petrographic microscope.
With practice, most mineral suites from Mount St. Helens tephras can be recognized using a binocular microscope.
In addition, the suites typical of many important tephra units can be recognized in the field using a hand lens.
Ferromagnesian minerals present consistently in carefully cleaned samples of
a pumiceous tephra are regarded as primary phenocrysts representative of the parent magma. Minerals that were found
only sporadically are regarded as possible accidental minerals. Such minerals are common but generally make up
only a small proportion of any given sample.
Although the different proportions of minerals from one tephra unit to another
are useful for identification, those proportions can vary widely for different samples of the same unit. Estimates
of hypersthene:hornblende ratios for various samples of layer Wn in this study, for example, range from about 10:1
to about 2:1. Similarly, modal abundances for layer Wn determined from counts of more than 300 grains show a range
of hypersthene:hornblende ratios of about 10:1 to 3:1(Smith and Leeman, 1982).
Chemical analyses
Chemical analyses were used sparingly during this study. At least one sample
from most of the described tephra units was obtained (appendix), but results are cited only infrequently for describing
tephras, magmas, or chemical differences. Data shown in the appendix are as reported by laboratories. Silica percentages
reported in the body of the text are derived from recalculation of laboratory analyses to 100 percent, free of
H2O.
Age
Tephra ages are based chiefly on numerical dates obtained from radiocarbon
samples (table 2). All reported ages older than about 600 years are based on radiocarbon
and are stated in radiocarbon years rather than calendar years. Some ages younger than 600 years are based on radiocarbon,
but most are derived from growth-ring counts of trees. Carbonaceous material suitable for dating is common in deposits
from Mount St. Helens; preservation of much of it has been enhanced by charring from hot tephra or pyroclastic
flows.
Radiocarbon ages provide the chronologic framework for this tephra study. They
are approximate, however, and should not be used for precise dating or detailed correlation. Radiocarbon ages have
wide ranges of uncertainty, even though they may be reported precisely, in some reports to even a specific year.
Multiple samples and analyses obtained during this study suggest that the ages older than about 30,000 years from
Mount St. Helens deposits have ranges of uncertainty of as much as thousands of years. Similar multiple analyses
of samples younger than about 4,000 years indicate much smaller uncertainties, but still measured in hundreds of
years. Ages derived from growth-ring counts of trees, in contrast, are relatively precise and can provide even
specific-year dates if trees were killed or severely damaged by tephra falls.
Uncertainties relative to radiocarbon ages result from many factors, mainly
those associated with difficulties in measuring the amount of radiocarbon remaining in a sample, variations in
amount of radiocarbon in the atmosphere in the past, the fact that usually only limiting ages can be determined,
and contamination by older or younger organic matter.
Realistic measurement uncertainties commonly are greater than reported, in
part because uncertainties due to counting variation typically are reported to one standard deviation, to allow
results to be compared from one laboratory to another. Radiocarbon ages obtained during this study were calculated
to one standard deviation using the Libby half-life (5,568 ± 30 yr) and are referenced to A.D. 1950 (Meyer
Rubin, U.S. Geological Survey, written commun., 1986). The ages are presented here as reported by the U.S. Geological
Survey Radiocarbon Laboratory, Reston, Virginia. They were not recalculated to account for past changes in atmospheric
radiocarbon contents or for recalculations of the radiocarbon half-life. They can be adjusted to estimates of calendar-year
dates by using data from the 1993 calibration volume of Radiocarbon (v. 35, no. 1).
In some earlier reports on Mount St. Helens tephras, we reported uncertainties
larger than one standard deviation in order to allow for sample characteristics that suggested probable errors
greater than those introduced by counting variation alone. Although those samples have not been since more accurately
dated, some uncertainties reported herein are lower. My experience with many samples taken from stratigraphically
controlled deposits at Mount St. Helens suggests that an uncertainty larger than that reported at one standard
deviation, perhaps by as much as a factor of two, should be considered likely when attempting to correlate ages
or deposits.
Table 2. Radiocarbon dates pertaining to age of tephra layers at Mount St. Helens.
W-2993
|
Wood, from Sugar Bowl deposit
|
1,150
|
±60
|
Crandell and others (1981)
|
W-5343
|
Wood, from Sugar Bowl deposit
|
1,200
|
±200
|
Crandell and Hoblitt (1986)
|
W-3138
|
Wood, from below Sugar Bowl deposit
|
1,410
|
±70
|
Crandell and others (1981)
|
|
|
|
|
|
W-2990
|
Wood, above layer Bu
|
1,620
|
±50
|
Crandell and others (1981)
|
W-2527
|
Charcoal, between layers Bu and Bo
|
1,740
|
±70
|
Crandell and others (1981)
|
W-2924
|
Peat, above layer Bi
|
1,780
|
±60
|
Crandell and others (1981)
|
W-2925
|
Peat, below layer Bi
|
1,850
|
±60
|
Crandell and others (1981)
|
W-2923
|
Charcoal, above layer Bh
|
2,200
|
±60
|
Crandell and others (1981)
|
|
|
|
|
|
W-2541
|
Charcoal, within set P
|
2,670
|
±70
|
Crandell and others (1981)
|
W-2829
|
Charred wood, between sets Y and P
|
2,930
|
±60
|
Crandell and others (1981)
|
W-2675
|
Charred wood, between sets Y and P
|
2,960
|
±50
|
Crandell and others (1981)
|
W-3262
|
Wood, from Lahar
|
3,280
|
±90
|
Crandell and others (1981)
|
|
|
|
|
|
W-2549
|
Charred wood, within set Y
|
3,350
|
±50
|
Crandell and others (1981)
|
W-3144
|
Charred wood, within set Y
|
3,380
|
±60
|
Crandell and others (1981)
|
W-1752
|
Charred wood, beneath layer Yn
|
3,510
|
±80
|
Crandell and others (1981)
|
W-3911
|
Charcoal, below set Y
|
3,850
|
±70
|
Crandell and others (1981)
|
W-2677
|
Charcoal, below set Y
|
3,900
|
±50
|
Crandell and others (1981)
|
W-1751
|
Charcoal, beneath set Y
|
4,680
|
±80
|
Crandell and others (1981)
|
|
|
|
|
|
W-2587
|
Charcoal, within set J
|
8,300
|
±90
|
Crandell and others (1981)
|
W-2702
|
Charcoal, within set J
|
8,430
|
±100
|
Crandell and others (1981)
|
W-2991
|
Charcoal, within set J
|
8,900
|
±70
|
Crandell and others (1981)
|
W-3257
|
Wood, above set J
|
9,170
|
±100
|
Crandell and others (1981)
|
W-5722
|
Peat, above Jb, Conboy bog
|
9,260
|
±300
|
This study
|
W-5731
|
Peat, above Jg, Fargher Lake
|
10,580
|
±250
|
This study
|
W-3548
|
Wood, below Jg, South Fork Toutle
|
10,710
|
±150
|
Crandell and others (1981)
|
W-5718
|
Peat, above Jb, Conboy
|
10,740
|
±250
|
This study
|
W-5724
|
Peat, below Jg, Fargher Lake
|
10,980
|
±250
|
This study
|
W-5719
|
Peat, below Jy(?), Fargher Lake
|
11,580
|
±250
|
This study
|
|
|
|
|
|
W-2868
|
Charcoal, between S and J, Rd 140
|
12,110
|
±110
|
Crandell and others (1981)
|
W-2870
|
Charcoal, between S and J, Rd 140
|
11,550
|
±230
|
Crandell and others (1981)
|
W-2866
|
Charcoal, between S and J, Rd 140
|
11,900
|
±190
|
Crandell and others (1981)
|
W-2832
|
Charcoal, below set J
|
11,700
|
±90
|
Crandell and others (1981)
|
W-2441
|
Charcoal, below set J
|
11,880
|
±110
|
Crandell and others (1981)
|
|
|
|
|
|
W-3133
|
Peat, above upper set S
|
12,120
|
±100
|
Crandell and others (1981)
|
W-3141
|
Charcoal, beneath part of set S
|
12,910
|
±160
|
Crandell and others (1981)
|
W-3136
|
Peat, beneath upper set S
|
13,650
|
±120
|
Crandell and others (1981)
|
|
|
|
|
|
W-2413
|
Charcoal, above set K
|
18,560
|
±180
|
Crandell and others (1981)
|
W-4531
|
Charcoal, above set K
|
19,160
|
±250
|
Crandell and others (1981)
|
W-2540
|
Charcoal, below sets M and K
|
20,350
|
±350
|
Crandell and others (1981)
|
W-2976
|
Charcoal, within set C
|
36,000
|
±2,500
|
Crandell and others (1981)
|
W-2661
|
Charcoal, within set C
|
37,600
|
±1,300
|
Crandell and others (1981)
|
W3259
|
Charcoal, within(?) set C
|
more than 42,000
|
|
Crandell and others (1981)
|
The amount of radiocarbon in the atmosphere has varied enough in the past that
radiocarbon content can be equal in samples of different calender ages (Suess, 1970). Thus, even if the amount
of radiocarbon left in some samples could be measured exactly, it would not necessarily identify the specific age
of the samples.
Radiocarbon samples also generally provide only limiting dates for tephra deposits
because the organic material formed either before or after the short time during which the tephra fell. Although
some tephras enclosed in peat have been dated approximately by combining samples of peat from just below and just
above the tephra to obtain a single sample for dating, the date relies on the assumption that ages of the peat
sampled below and above differ equally from the age of the tephra.
Contamination can result in anomalously old or young ages, from incorporation
of old, nonradioactive carbon or from incorporation of younger carbon into organic material long after formation
of the latter. Anomolously young ages were obtained for several samples during this investigation. The problem
was especially noticeable for charcoal samples taken from old, coarse pumice layers that had been exposed at the
surface for long periods of time. The charcoal may have absorbed younger organic material after deposition, perhaps
from humic compounds in water that infiltrated the pumice.
Thus, although radiocarbon dates provide the ages for most of the deposits
described, those ages are considered to be approximate. For comparisons and correlations, they commonly are rounded
to the nearest 100, 500, or even 1,000 years.
Previous work
Studies whose results have been used extensively during this study start
with that of Verhoogen (l937). Verhoogen's report on Mount St. Helens noted the youth of the modern volcano and
the presence of an "old Mount St. Helens series" older than the modern cone. He also described both a
young widespread pumice and an older yellowish pumice on the flanks of Mount St. Helens.
Lawrence (l938, l939, l941, 1954) described young pumice deposits at Mount
St. Helens and used tree-ring counts to assign ages to some of them. He noted that a thick pumice deposit buried
the roots of many trees near Spirit Lake. Examination of their growth rings gave an age of about A.D. l800 for
the pumice (Lawrence, 1939, 1954). That pumice is designated layer T (Mullineaux, 1964) in the sequence described
here.
Carithers (l946) described the Mount St. Helens pumice deposits and their economic
importance. He described distributions, thicknesses, and volumes for a thick, coarse "older" yellowish
pumice and for a thick, white "younger" pumice. In addition, he reported a scattering of even younger
gray pumice lumps at the ground surface. The yellowish and white layers of Carithers consist chiefly of layers
described here as Yn and Wn, respectively, and the younger, gray lumps are part of layer T of this report.
D.R. Crandell and others recognized several widespread tephra marker beds in
Mount Rainier National Park during studies of origin of the Osceola Mudflow (Crandell and Waldron, l956), of surficial
geology in the park (Crandell, 1969), and of glaciation in the park (Crandell and Miller, l974). They suspected
that three of those marker beds, then termed layers O, Y and W, originated at one or more volcanoes other than
Mount Rainier, and they determined that two of them were relatively thick in the southern part of the park. R.D.
Miller and I traced those two southward and found that they originated at Mount St. Helens. Additional studies
at Mount St. Helens showed that a younger thick and coarse tephra (layer T) could also be distinguished (Mullineaux,
l964). Hyde (1975) discovered several older tephra beds on the south side of the volcano, and further work led
to the classification of a tephra sequence that spans the known history of the volcano (Mullineaux and others,
l972, l975, 1978; Mullineaux, 1986).
C.A. Hopson (1971), during geologic mapping at Mount St. Helens in the late
1960's, noted that the distribution of layer T on the north flank of the volcano suggested that the layer originated
at a vent on that flank rather than at the summit of the volcano.
Acknowledgments. This study was carried out as part of investigations
with D.R. Crandell of eruptive products, histories, and potential volcanic hazards of Cascade Range volcanoes.
It was conducted jointly with a study of flowage deposits of Mount St. Helens by Crandell, who has made countless
important contributions to this work. It has also benefitted significantly from concurrent studies at Mount St.
Helens by J.H. Hyde and R.P. Hoblitt of the U.S. Geological Survey and C.A. Hopson of the University of California
at Santa Barbara and from other contributions by U.S. Geological Survey colleagues R.E. Wilcox, R.D. Miller, and
C.D. Miller. Laboratory identification of most of the ferromagnesian minerals used to classify tephra units depended
on application of Wilcox's development of a spindle stage and focal masking methods (Wilcox, l959a, 1962, 1983).
In addition, Wilcox and H.A. Powers in 1963 first identified cummingtonite as phenocrysts in the Mount St. Helens
tephras (Wilcox, 1965). That discovery has proved extremely important for studies of the Mount St. Helens products
and for recognition of downwind marker beds. Valuable assistance provided by many members of the Gifford Pinchot
National Forest, U.S. Forest Service, greatly facilitated the study. J. Archuleta ably assisted me during the field
season of 1972.
Radiocarbon dates obtained for this study and those reported in Crandell and
others (1981), were analyzed in the U.S. Geological Survey Radiocarbon Laboratory in Reston, Virginia, under the
supervision of Meyer Rubin.
OVERVIEW OF TEPHRA SEQUENCE
The abundant tephra deposits produced by Mount St. Helens during the past 40,000
years are present in thick sequences around the volcano (fig. 3) and record many different
eruptive episodes. Those episodes are grouped into stages and periods, each of which includes multiple events and
is separated from others by hundreds or thousands of years of either dormancy or relative quiescence (tables
3, 4). The tephra strata are interbedded with abundant coarse flowage deposits and
ash deposits probably derived from flowage events as well as lava flows.
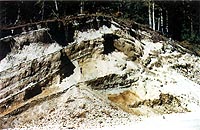
Figure 3. Thick tephra sequence about 6 km northeast of the center of Mount St. Helens along USFS Road
99. Outcrop is about 6 m high. All tephra deposits exposed here were erupted during the past 13,000 years. Layers
Yn, Wn, and T are the most conspicuous tephras northeast of the volcano. Layers Yn and Wn are also two of the largest
volume eruptions known of the past 13,000 years. Layer Yn thins noticeably from the middle of the outcrop to the
left; only a small part of the difference evident is from scraping of the outcrop. Layer Wn, in contrast, thickens
from the middle of the outcrop to the left. Photograph taken in 1965. (84K)
|
Compositionally, the volcano erupted only dacite and silicic andesite throughout
most of its history. The appearance of mafic andesite and basalt about 2,500 years ago marks increasing compositional
complexity and the beginning of the modern volcano.
The prominent tephra strata are subdivided into ten sets and two single layers
(table 3); the sets include more than 100 depositional units (table
4). One or more tephra sets are recognized in each stage and each period of the volcano's eruptive history.
In addition, a single thin ash bed probably erupted during the mid-19th century is described separately.
Distribution
Most tephra deposits from Mount St. Helens lie in the 180° sector east
of a north-south line through the volcano because the prevailing winds blow toward that sector. Wind records suggest
that almost 90 percent of the tephra should be expected in that 180º sector and more than 50 percent in the
90º sector from northeast to southeast of the volcano (fig. 4).
Preservation
Preservation of these tephras varies greatly, chiefly with age and altitude.
Older tephras are more weathered than young ones regardless of altitude because of the longer time exposed to alteration.
In addition, they have been more disrupted by physical processes such as creep, rapid landsliding, frost heave,
tree-root throw, and animal burrowing that mix, turn over, or simply remove parts of the sequence.
The three tephra sets erupted before 18,000 years ago exhibit the strongest
disturbance, from long exposure to weathering and the intensity of cold-climate processes active during the maximum
of the last major (Fraser) glaciation between about 25,000 and 15,000 years ago. The next two younger sets, erupted
from about 13,000 to 10,500 years ago, are less disturbed yet show some effects of late-glacial rigorous climate.
Tephras formed during the past 4,000 years are remarkably well preserved; part of that good preservation results
from the frequency of eruptions during that time. Most of these tephra strata were buried soon after deposition,
and thus the effects of surface weathering and other, mostly physical, disturbances were minimized. Although innumerable
rills and streams have sliced down into the deposits of the past 4,000 years, nearly undisturbed stacks of those
strata are preserved between the eroded gullies.

The pre-1980 tephra strata less than 4,000 years old are almost as well
preserved as those erupted in 1980. Comparison of the 1980 tephras with earlier ones of the past 4,000 years suggests
that virtually any stratum prominent enough to be identified in the 1980 sequence would, if duplicated in the older
deposits, be identifiable in the older sequence.
Figure 4. Approximate percentage of time that wind blows in various directions over Mount St. Helens.
Percentages are rounded averages of frequencies determined at various altitudes between 3,000 and 16,000 m at Salem,
Oreg., and Quillayute, Wash. (Winds Aloft Summary of the Air Weather Service, U.S. Air Force, available from the
National Climatic Center, Asheville, N.C.). Modified from Crandell and Mullineaux (1978).
|

Disturbance of tephra of all ages has been greater at higher altitudes,
chiefly because of the colder climate. Some deposits at relatively high altitudes were simply removed by glaciers
during the Fraser glaciation. Moreover, processes such as creep, landsliding, and frost heave were increasingly
severe with altitude. Tephra sets C, M, and K, all of which were deposited before or during the maximum of the
Fraser glaciation, generally are well enough preserved for interpretation of eruptive events only at altitudes
lower than about 600 m around Mount St. Helens (fig. 5).
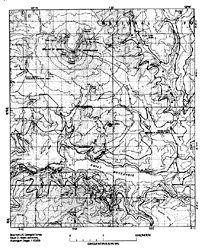
Figure 5. Approximate area below 600 m altitude (between heavy lines) in valleys immediately east and
south of Mount St. Helens, and several sites (circles) at which relatively well preserved tephra sequences older
than the Fraser Glaciation are exposed. Base from U.S. Geological Survey Mount St. Helens and vicinity, Washington-Oregon,
scale 1:100,000. (138K)
|
Tephras of sets S and J, which were laid down during the waning stages of the Fraser glaciation, commonly are well
preserved at altitudes of about 1,000 m or less and are sparsely preserved up to an altitude of about 1,250 m.
Location of relatively complete tephra sequences
Because of the westerly winds and increased disturbance with altitude, areas
that are both in the quadrant from northeast to southeast of the volcano and at low altitudes are most likely to
display relatively complete tephra sequences (fig. 5). The most complete sequence at a single
site that I found is east-southeast of the volcano at the confluence of Smith Creek and Muddy River; all major
tephra units except layer T are well represented there. The sequence crops out above a rock quarry. It is referred
to as the Muddy River quarry site and has been used frequently as a reference section. Unfortunately, U.S. Forest
Service (USFS) Road N92 leading to the site was destroyed in 1980. In 1989, access was possible from USFS Road
83 by crossing Smith Creek on foot upstream from its junction with Muddy River and traversing southward along the
east valley wall. Several other nearby outcrops in the Smith Creek-Muddy River valley display relatively complete
tephra sequences but were inaccessible or almost so by road in 1989.