
Sustainability of Ground-Water Resources--Circular 1186
GENERAL FACTS AND CONCEPTS ABOUT GROUND WATER
The following review of some basic facts and concepts
about ground water serves as background for the discussion of ground-water
sustainability.
- Ground water occurs almost everywhere beneath the land surface.
The widespread occurrence of potable ground water is the reason
that it is used as a source of water supply by about one-half the population
of the United States, including almost all of the population that is served
by domestic water-supply systems.
- Natural sources of freshwater that become ground water are (1)
areal recharge from precipitation that percolates through
the unsaturated zone to the water table (Figure 4) and (2) losses
of water from streams and other bodies of surface water such as lakes and
wetlands. Areal recharge ranges from a tiny fraction to about one-half
of average annual precipitation. Because areal recharge occurs over broad
areas,even small average rates of recharge (for example, a few inches per year)
represent significant volumes of inflow to ground water. Streams and other
surface-water bodies may either gain water from ground water or lose
(recharge) water to ground water. Streams commonly are a significant source
of recharge to ground water downstream from mountain fronts and steep
hillslopes in arid and semiarid areas and
in karst terrains (areas underlain by limestone and other soluble rocks).
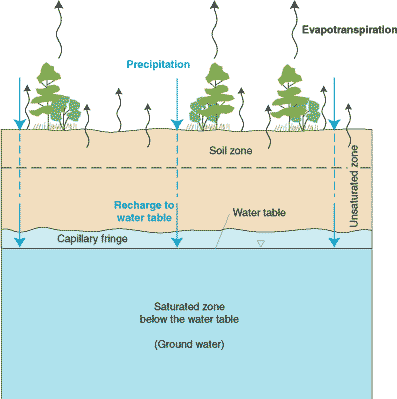
Figure 4. The unsaturated zone,
capillary fringe, water table, and saturated zone.
Water beneath the land surface occurs in two principal zones, the
unsaturated zone and the saturated zone. In the unsaturated zone, the spaces between particle grains and the cracks
in rocks contain both air and water. Although a considerable amount of water
can be present in the unsaturated zone, this water cannot be pumped by wells
because capillary forces hold it too tightly.
In contrast to the unsaturated zone, the voids in the saturated zone
are completely filled with water. The approximate upper surface of the
saturated zone is referred to as the water table. Water in the saturated
zone below the water table is referred to as ground water. Below the water
table, the water pressure is high enough to allow water to enter a well as
the water level in the well is lowered by pumping, thus permitting ground
water to be withdrawn for use.
Between the unsaturated zone and the water table is a transition zone,
the capillary fringe. In this zone, the voids are saturated or almost
saturated with water that is held in place by capillary
forces.
- The top of the subsurface ground-water body, the water table, is a
surface, generally below the land surface, that fluctuates seasonally and
from year to year in response to changes in recharge from precipitation and
surface-water bodies. On a regional scale, the configuration of the
water table commonly is a subdued replica of the land-surface topography.
The depth to the water table varies.
In some settings, it can be at or near the land surface; for example, near
bodies of surface water in humid climates. In other settings, the depth to
the water table can be hundreds of feet below land surface.
- Ground water commonly is an important source of surface water. The
contribution
of ground water to total streamflow varies widely among streams, but
hydrologists estimate the average contribution is somewhere between 40 and
50 percent in small and medium-sized streams. Extrapolation of these numbers
to large rivers is not straightforward; however, the ground-water
contribution to all streamflow in the United States may be as large as 40
percent. Ground water also is a major source of water to lakes and wetlands.
- Ground water serves as a large subsurface water reservoir. Of all the
freshwater that exists, about 75 percent is estimated to be stored in polar
ice and glaciers and about 25 percent is estimated to be stored as
ground water. Freshwater stored in rivers, lakes, and as soil moisture
amounts to less than 1 percent of the world's freshwater. The reservoir
aspect of some large ground-water systems can be a key factor in the
development of these systems. A large ratio of total ground-water storage
either to ground-water withdrawals by pumping or to natural discharge is one
of the potentially useful characteristics of a ground-water system
and enables water supplies to be maintained through long periods of drought.
On the other hand, high ground-water use in areas of little recharge
sometimes causes widespread declines in ground-water levels
and a significant decrease in storage in
the ground-water reservoir.
- Velocities of ground-water flow generally are low and are orders of
magnitude less than velocities of streamflow. The movement
of ground water normally occurs as slow seepage through the pore spaces
between particles of unconsolidated earth materials or through networks of
fractures and solution openings in consolidated rocks. A velocity of 1 foot
per day or greater is a high rate of movement for ground water, and
ground-water velocities can be as low as 1 foot per year or 1 foot per
decade. In contrast, velocities of streamflow generally are measured in feet
per second. A velocity of 1 foot per second equals about 16 miles per day.
The low velocities of ground-water flow can have important implications,
particularly in relation to the movement
of contaminants.
- Under natural conditions, ground water moves along flow paths from areas
of recharge to areas of discharge at springs
or along streams, lakes, and wetlands. Discharge also occurs as seepage
to bays
or the ocean in coastal areas, and as transpiration by plants whose roots
extend to near the water table. The three-dimensional body of earth material
saturated with moving ground water that extends from areas of recharge to
areas of discharge is referred to as a ground-water-flow system (Figure 5).
Figure 5. A local scale
ground-water-flow system.
In this local scale ground-water-flow system,inflow of water from areal recharge occurs at the water table. Outflow of
water occurs as (1) discharge to the atmosphere as ground-water
evapotranspiration (transpiration by vegetation rooted at or near the water
table or direct evaporation from the water table when it is at or close to
the land surface) and (2) discharge of ground water directly through the
streambed. Short, shallow flow paths originate at the water table near the
stream. As distance from the stream increases, flow paths to the stream are
longer and deeper. For long-term average conditions, inflow to this natural
ground-water system must equal outflow.
- The areal extent of ground-water-flow systems varies from a few square
miles or less to tens of thousands of square miles. The length of
ground-water-flow paths ranges from a few feet to tens, and sometimes
hundreds, of miles. A deep ground-water-flow system with long flow paths
between areas of recharge and discharge may be overlain by, and in hydraulic
connection with, several shallow, more local, flow systems (Figure 6). Thus,
the definition of a ground-water-flow system is to some extent subjective
and depends in part on the scale of a study.
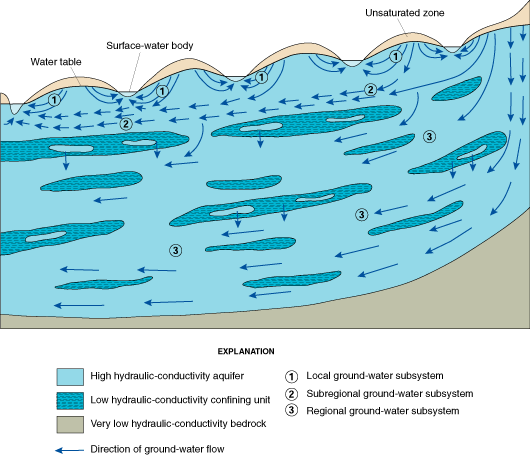
Figure 6. A regional
ground-water-flow system that comprises subsystems at different scales and
a complex hydrogeologic framework. (Modified from Sun, 1986.)
Significant features of this depiction of part of a regional
ground-water-flow system include (1) local ground-water subsystems in the
upper water-table aquifer that discharge to the nearest surface-water bodies
(lakes or streams) and are separated by ground-water divides beneath
topographically high areas; (2) a subregional ground-water subsystem in the
water-table aquifer in which flow paths originating at the water table do
not discharge into the nearest surface-water body but into a more distant
one; and (3) a deep, regional ground-water-flow subsystem that lies
beneath the water-table subsystems and is hydraulically connected to them.
The hydrogeologic framework of the flow system exhibits a complicated
spatial arrangement of high hydraulic-conductivity aquifer units and low
hydraulic-conductivity confining units. The horizontal scale of the figure
could range from tens to hundreds of miles.
- The age (time since recharge) of ground water varies in different parts
of ground-water-flow systems. The age of ground water increases steadily
along a particular flow path through the ground-water-flow system from an
area of recharge to an area of discharge. In shallow, local-scale flow
systems, ages of ground water at areas of discharge can vary from less than
a day to a few hundred years. In deep, regional flow systems with long flow
paths (tens of miles), ages of ground water may reach thousands or tens of
thousands of years.
- Surface and subsurface earth materials are highly variable in their
degree of particle consolidation, the size of particles, the size and shape
of pore or open spaces between particles and between cracks in consolidated
rocks, and in the mineral and chemical composition of the particles. Ground
water occurs both in loosely aggregated and unconsolidated materials, such
as sand and gravel, and in consolidated rocks, such as sandstone, limestone,
granite, and basalt.
- Earth materials vary widely in their ability to transmit and store ground
water. The ability of earth materials to transmit ground water
(quantified as hydraulic conductivity) varies by orders of magnitude and is
determined by the size, shape, interconnectedness, and volume of spaces
between solids in the different types of materials. For example,
the interconnected pore spaces in sand
and gravel are larger than those in finer grained sediments, and the
hydraulic conductivity of sand and gravel is larger than the hydraulic
conductivity of the
finer grained materials. The ability of earth materials to store ground
water also varies among different types of materials. For example, the
volume of water stored in cracks and fractures per unit volume of granite is
much smaller than the volume stored per unit volume in the intergranular
spaces between particles of sand and gravel.
- Wells are the principal direct window to study the subsurface
environment. Not only are wells used to pump ground water for many
purposes, they also provide essential information about conditions in the
subsurface. For example, wells (1) allow direct measurement of water levels
in the well, (2) allow sampling of ground water
for chemical analysis, (3) provide access for
a large array of physical measurements in the borehole (borehole geophysical
logging) that give indirect information on the properties of the fluids and
earth materials in the neighborhood of the well, and (4) allow
hydraulic testing (aquifer tests) of the earth materials in the neighborhood
of the well to determine local values of their transmitting and storage
properties. In addition, earth materials can be sampled directly at any
depth during the drilling of the well.
- Pumping ground water from a well always causes (1) a decline in
ground-water levels (heads; see Figure 7) at and near the well, and (2) a
diversion to the pumping well of ground water that was moving slowly to its
natural, possibly distant, area of discharge. Pumping of a single well
typically has a local effect on the ground-water-flow system. Pumping of
many wells (sometimes hundreds or thousands of wells) in large areas can
have regionally significant effects on ground-water systems.
Figure 7. The concept of
"hydraulic head" or "head"
at a point in an aquifer.
Consider the elevations above sea level at points A and B in an
unconfined aquifer and C in a confined aquifer. Now consider the addition of
wells with short screened intervals at these three points. The vertical
distance from the water level in each well to sea level is a measure of hydraulic head or head, referenced to a common datum at each
point A, B, and C, respectively. Thus, head at a point in an aquifer is the
sum of (a) the elevation of the point above a common datum, usually sea
level, and (b) the height above the point of a column of static water in a
well that is screened at the point. When we discuss declines or rises in
ground-water levels in a particular aquifer in this report, we are referring
to changes in head or water levels in wells that are screened or have an
open interval in that aquifer.
- Ground-water heads respond to pumping to markedly different degrees in
unconfined and confined aquifers. Pumping the same quantity of water
from wells in confined and in unconfined aquifers initially results in much
larger declines in heads over much larger areas for the confined aquifers
(see Box A). This is because less water is available from storage in
confined aquifers compared to unconfined aquifers. At a
later time, as the amount of water derived from storage decreases and the
system approaches equilibrium, the response of
the system no longer depends upon being confined or unconfined. The amount
of head decline at equilibrium is a function of the transmitting properties
of the aquifers and confining units, discharge rate of the well, and
distance to ground-water-system boundaries. Many aquifers, such as the upper
part of the deep flow subsystem shown in Figure 6, exhibit a response
to pumping that is intermediate between
a completely confined and a completely unconfined aquifer system.
Back to Contents
Back to Introduction
Next--Box A