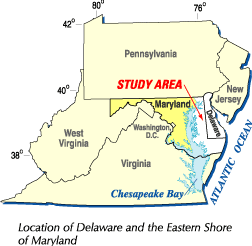
Adjustments to U.S. Geological Survey Peak-Flow Magnitude-Frequency Relations in Delaware and Maryland Following Hurricane Floyd, September 1999
by Edward J. Doheny and Jonathan J.A. Dillow
USGS Fact Sheet FS-152-02
This report is available in Portable Document Format (PDF). If you do not have the Adobe Acrobat PDF Reader, it is available for free download from Adobe Systems Incorporated.
View the full report in PDF (1.5MB)
Document Accessibility: Adobe Systems Incorporated has information about PDFs and the visually impaired. This information provides tools to help make PDF files accessible. These tools and the accessible reader may be obtained free from Adobe at Adobe Access.
Introduction
On September 16, 1999, Hurricane Floyd entered the lower half of Maryland's Eastern Shore and followed a northeasterly track over the lower part of the Delmarva Peninsula. Total rainfall amounts varied across the region. Some areas received more than 10 inches of rain, while other areas received less than 5 inches. The heaviest recorded rainfall occurred in the central part of Maryland's Eastern Shore, where a maximum of 12.59 inches was reported at Chestertown, Maryland (Tallman and Fisher, 2001).
Record-high peak discharges were recorded at several active streamflow-gaging stations (referred to as "gages" in this report) in northern Delaware and on the upper Eastern Shore of Maryland. U.S. Geological Survey (USGS) personnel also surveyed high-water marks to estimate peak-flow magnitudes for the storm at selected discontinued gages in the region (Tallman and Fisher, 2001).
When severe flooding occurs, information about peak-flow magnitude and frequency of recurrence must be disseminated quickly to Federal, State, and local agencies concerned with public safety and emergency response, as well as to the public. The initial estimates of peak-flow frequency associated with a particular flood are based on peak-flow magnitude-frequency relations established prior to the flood. These relations have not incorporated the effect of the peak flows associated with the flood because peak-flow data must be reviewed for accuracy before being published or used in any analyses. As a result, it may take several months to incorporate new peak-flow data and revise the peak-flow magnitude-frequency relation for a given stream location. If the new peak flows associated with a flood are significantly greater than previously recorded or historical peak flows at a specific gage, adjustments reflecting the inclusion of the peak flows can cause significant changes to the magnitude-frequency relations.
This report summarizes the basic principles that are used to derive peak-flow magnitude-frequency relations, and discusses the adjustment techniques that may be applied to refine these relations. A comparison of the initial peak-flow recurrence-interval estimates and subsequent, adjusted estimates resulting from peak flows associated with Hurricane Floyd in 1999 for 28 gages in Delaware and Maryland are also presented.
Peak-Flow Magnitude-Frequency Relations
The relation between the magnitude and frequency of peak flow in streams describes how often, in terms of a long-term average, peak flow of a given magnitude may be equaled or exceeded at a given gage. For example, the relation can be used to define the "100-year flood." This is the peak-flow magnitude that may be equaled or exceeded, on average, once every 100 years, or that has a 1-percent chance of being equaled or exceeded in any given year at a gage. The length of the average time period between peak flows of a specified magnitude, 100 years in the preceding example, is commonly known as the recurrence interval. This relation is based on knowledge of the temporal distribution of peak flows at a gage, and an understanding of the statistical characteristics of these data.
Peak-Flow Data
Most peak-flow data collected by the USGS are derived from a continuous record of stream stage and a stage-discharge relation for each gage. In 2002, stream stage and related data are being collected at 135 gages on streams throughout Delaware and Maryland. Data collected include: (1) stream stage, which is measured continuously by use of a recorder, or periodically by field observation of a staff gage or by use of crest-stage gages, (2) stream discharge, which is measured throughout a range of stages, either directly, by use of a current meter, or by indirect methods, and (3) other miscellaneous information that describes factors affecting the stage-discharge relation.
These data are used to develop a stage-discharge relation for each gage where data have been collected. The stage-discharge relation is a continuous curve determined from the discrete points at which both stage and discharge have been measured (fig. 1). The accuracy of the stage-discharge relation for all active gages is regularly verified. When there is a substantial change in the stream-channel geometry in the vicinity of the gage, the stage-discharge relation is revised to reflect the new conditions. Because the stage-discharge relation is a continuous curve, peak flow can be determined for any stage included on the curve. Peak-flow discharges associated with stages beyond the defined curve of the stage-discharge relation can be estimated by extending the curve using analytical techniques such as rating extension and indirect methods (Benson and Dalrymple, 1967; Kennedy, 1984).
Statistical Distribution
USGS uses the annual peak-flow series from a gage in analyzing recurrence intervals of peak flows (Interagency Advisory Committee on Water Data, 1982). The annual peak-flow series consists of the largest peak for each water year (October 1 through September 30) in which peak-flow data were collected at a gage. On the basis of results of statistical tests performed on annual peak-flow data from 300 gages in the United States, the log-Pearson Type III statistical distribution with a weighted skew coefficient was found to produce the least bias in magnitude-frequency estimates with the lowest level of uncertainty among eight distributions that were evaluated (Beard, 1975). An example of the log-Pearson Type III peak-flow statistical distribution (W.O. Thomas, Jr. and others, USGS, written communication, 1998) for station number 01478000, Christina River at Coochs Bridge, Delaware, is shown in figure 2.
The three parameters that define the log-Pearson Type III distribution are the mean logarithm of the annual peak flows, the standard deviation of the logarithms, and the skew coefficient of the logarithms (Water Resources Council, 1967), and are defined as follows:
In equation 3, G represents the skew as calculated from the data collected at a gage. However, the method recommended by Beard (1975) requires a weighted skew coefficient that is derived from both the station skew and skew from other nearby gages. The weighted skew coefficient generally provides a more reliable estimate of skew for a gaged watershed and the surrounding regional area. As a result, magnitude-frequency relations for a given gage will incorporate regional data into the estimates by use of a weighted skew coefficient. The method for calculating the weighted skew coefficient is described by the Interagency Advisory Committee on Water Data (1982).
Magnitude-Frequency Relation
The peak-flow magnitude-frequency relation for streamflow at a gage is defined by a curve, obtained by fitting the log-Pearson Type III distribution with a weighted skew coefficient to the annual peak-flow series from that location. The fitting technique computes the base-10 logarithm of discharge for selected exceedance probabilities using the following equation:
The method for calculating the peak-flow magnitude-frequency curve, including the equation used to compute K, is also described by the Interagency Advisory Committee on Water Data (1982).
Data Assumptions
The analysis methods discussed in this report may be used only if the recorded peak-flow data from a specific gage can be reasonably assumed to represent the peak-flow magnitude and frequency characteristics of a stream over time. This assumption can be reasonably made if certain factors that can affect change in the hydraulic and hydrologic properties of a watershed are considered. These factors include land-use changes in the watershed over time; the presence of flow-control structures in the stream channel; and channel alterations or improvements at or near the gage.
Land-Use Changes
Land-use changes in a watershed, such as development of forest or cropland, can alter both the amount of precipitation that reaches the stream channel as overland flow and the timing of its arrival. These changes, in turn, will alter the magnitude-frequency relation of peak flow for the stream. If substantial land-use changes occur in a watershed during the period when peak-flow data are being collected, the magnitude-frequency relation for the stream should be based upon the part of the peak-flow record that was collected after the changes occurred. Geographic Information System (GIS) data or aerial photographs can be used to identify and quantify land-use changes. The significance of land-use changes on peak-flow magnitude over time may also be evaluated by performing nonparametric tests for monotonic trends in the peak-flow data. For example, a Kendall's Tau test could be performed on a peak-flow dataset to determine if a trend of increasing or decreasing discharge exists at a gage where the watershed land use has significantly changed over the period of record (Helsel and Hirsch, 1992).
Flow-Control Structures
Flow-control structures, such as dams or levees, are designed to regulate streamflow by altering the magnitude and timing of flow in the stream channel. The presence of these structures in a stream channel can alter the magnitude-frequency relation of peak flows at all downstream points in the channel.
Dams tend to store water from the upstream reaches of a watershed and reduce the magnitude of the peak flow downstream of the dam. If the volume of storage in the reservoir behind a dam is small compared to the total volume of the average annual peak-flow event on the stream, the reduction of the peak-flow magnitude will be minimal and can be ignored for analytical purposes. When the reduction of the peak-flow magnitude is substantial, peak-flow data from locations downstream from a dam should not be analyzed using the methods discussed in this report because the statistical characteristics of regulated peak-flow data differ significantly from those of peak-flow data from unregulated watersheds.
Levees are structures that prevent water from flooding specific low-lying areas after the banks of the stream channel have been overtopped. Levees tend to disrupt the natural interaction of a stream channel and its flood plain by affecting overbank flow and storage. Levees can alter the magnitude-frequency relation of peak flows in a stream by forcing more water into the main channel, which in turn can increase the peak-flow magnitudes at locations downstream from the levee. For any analysis, the analyst must assess whether or not the effect of a levee is significant enough so that the peak-flow magnitude-frequency relations for downstream locations cannot be calculated using the methods described in this report.
Channel Alterations
Channel alterations or improvements, such as straightening or lining with impervious material, can alter the magnitude-frequency relation of peak flows in the stream. Channel straightening tends to decrease the channel length and sinuosity, thereby increasing the stream gradient and flow velocity. Lining the banks of the channel with impervious materials tends to reduce channel roughness and increase flow velocities. In both cases, flow velocities are increased, which may result in an increase in the peak-flow magnitude. A Kendall's Tau test could be performed on the peak-flow data to determine if a trend of increasing peak flow exists that coincides with the timing of the channel alterations. For any analysis, it must be determined if the effects of any channel alterations are significant enough that the peak-flow magnitude-frequency relations for downstream locations cannot be calculated using the methods discussed in this report.
Peak-Flow Record Characteristics Affecting Magnitude-Frequency Relations
Many factors can affect peak-flow magnitude-frequency relations. The factors mentioned previously are related to hydraulic and hydrologic properties of watersheds. This section describes certain characteristics of peak-flow records and the accepted methods used to account for their individual effects on the peak-flow magnitude-frequency relations of a given stream. All factors discussed in this section concern the relative importance of individual peak-flow values within the context of the entire peak-flow record, and are related to the length or continuity of the peak-flow record.
Length of Record
To be considered representative, a sufficient amount of peak-flow data must be collected at a location to capture the natural variability in peak-flow magnitude over time. Ten years is usually considered to be the minimum length of time that peak-flow data should be collected at a gage to fulfill this requirement. For stations with 10 years of peak-flow data or slightly more, watershed comparison and precipitation records may be used to increase accuracy of peak-flow magnitude-frequency relations. The longer the peak-flow record, the more likely the peak-flow magnitude-frequency relation derived from it is accurate. The methods discussed in this report are not applicable to gages with less than 10 years of peak-flow data.
Discontinuous Peak-Flow Record
Annual peaks for specific years may be missing or unavailable because of the destruction or removal, and subsequent reactivation, of a gage. This scenario results in a broken peak-flow record that spans a discontinuous time series. In this case, the individual continuous-record segments are typically analyzed as a single continuous record with a length equal to the sum of the lengths of both segments. This procedure is not applicable, however, if there is some physical change in the watershed during the ungaged interval that would make the overall record non-homogeneous (Interagency Advisory Committee on Water Data, 1982). Significant land-use changes in the watershed and the introduction of flood-control structures during the interval between record segments are examples of physical changes that could cause the overall peak-flow record to be non-homogeneous.
Incomplete Peak-Flow Record
An incomplete peak-flow record results when certain peaks are missing because they were either too low or too high to record, or because the gage was not operational for a short period of time due to flooding. An incomplete record can result in erroneous peak-flow magnitude-frequency relations if missing peaks are simply excluded from the dataset and subsequent analysis. When one or more annual peaks are missing from the peak-flow record, other resources should be used to make a reasonable estimate of the missing peaks (Interagency Advisory Committee on Water Data, 1982). These resources include data from nearby gages, regional rainfall data, and regional peak-flow magnitude-frequency estimation equations such as those presented in Dillow (1996a, 1996b).
Historical Peak Flows
Historical data describing the magnitude of peak flows is sometimes available from sources other than gages, and can be used to effectively increase the length of the peak-flow record. Adding this type of historical data to the peak-flow record increases confidence in the resulting magnitude-frequency relation. If any peak flows that occurred before, after, or between segments of the peak-flow record were maximums over an extended period of time, these peak flows must be considered when developing the peak-flow magnitude-frequency relation for the station.
The underlying assumption inherent in the use of historical data is that watershed and meteorological conditions during the period when the actual peak-flow record was collected are representative of the intervening period between the beginning or end of the peak-flow record and the time of the historical peak flow. The historical data should be used unless there is evidence to indicate that the historical data are not indicative of the intervening period. The recommended method for including historical peak-flow data in the peak-flow magnitude-frequency analysis is described and demonstrated by the Interagency Advisory Committee on Water Data (1982).
Outliers
Outliers are individual peak flows with magnitudes that are significantly smaller or larger than most other values in the peak-flow record. The retention, modification, or deletion of an outlier can substantially alter the statistical parameters computed from the dataset, especially if the peak-flow record is relatively short. When determining the peak-flow magnitude-frequency relation for a gage, the presence of low or high outliers must be determined, and appropriate adjustments to the relation must be made.
Low Outliers
Low outliers are peak flows that plot significantly below the rest of the data in the peak-flow record. Low outliers generally occur during years in which there is a hydrologic drought. The low-outlier threshold is determined as follows:
Generally, if the peak-flow record includes a peak that is below the low-outlier threshold, a statistical procedure is conducted to adjust the peak-flow magnitude-frequency relation for the gage. The procedure for low-outlier adjustment eliminates the influence of the low outlier on the peak-flow magnitude-frequency relation by excluding the low outlier from the analysis, and is described by the Interagency Advisory Committee on Water Data (1982).
High Outliers
High outliers are peak flows that plot significantly higher than the data in the peak-flow record. High outliers generally represent extreme floods, and are significantly higher than other values in the peak-flow record. The high-outlier threshold is determined as follows:
If the peak-flow record includes a value that is near or above the high-outlier threshold, and if historical data are available to provide a suitable context for adjustment, a statistical procedure can be conducted to adjust the peak-flow magnitude-frequency relation for the gage. The procedure assigns a statistical weight to the high outlier in relation to the rest of the dataset to reflect an appropriate amount of influence in the context of the historical data, providing an adjusted peak-flow magnitude-frequency relation. The procedure for high-outlier adjustment is described by the Interagency Advisory Committee on Water Data (1982).
Adjustments Reflecting the Influence of Hurricane Floyd
At many locations in northern Delaware and Maryland's Eastern Shore, Hurricane Floyd produced record-high peak flows at both active and discontinued gages (fig. 3). Evaluation of the Hurricane Floyd peaks using pre-Floyd magnitude-frequency relations indicates that Floyd caused peak flows with recurrence intervals greater than 500 years at several gages. This section presents a comparison of initial recurrence-interval estimates and both the unadjusted and adjusted recurrence-interval estimates resulting from updated magnitude-frequency analyses that include peak flows caused by Hurricane Floyd. Adjustments were made to magnitude-frequency relations at several gages on the basis of various factors. The resulting recurrence-interval estimates for peak flows caused by Hurricane Floyd are also discussed.
Comparison of Pre- and Post-Hurricane Floyd Recurrence Intervals
Peak-flow magnitude-frequency relations calculated prior to September 1999 provided the initial recurrence-interval estimates for Hurricane Floyd at 28 active and discontinued gages in Delaware and Maryland. These relations produced recurrence-interval estimates of 500 or more years at seven gages, and 100 or more years at three other gages. Subsequent analyses at these 28 gages, which included peak flows caused by Hurricane Floyd, resulted in peak-flow magnitude-frequency relations that indicated a maximum recurrence-interval estimate of 260 years for Hurricane Floyd. These relations were not adjusted to account for any of the factors discussed previously, and produced estimated recurrence intervals of 200 or more years at four gages, and between 100 and 200 years at three other gages. After making the adjustments described below, the largest estimated recurrence interval for any gage was reduced to 200 years, with estimates ranging between 100 and 170 years at seven other gages (table 1).
Inclusion of Hurricane Floyd peak flows at several gages also caused significant, but less substantial changes in the magnitude-frequency analyses. For example, the estimated recurrence intervals for the Floyd peak flows at Shellpot Creek at Wilmington, Delaware increased from 10 years to 18 years; Choptank River near Greensboro, Maryland decreased from 40 years to approximately 25 years; and Marshyhope Creek near Adamsville, Delaware decreased from 25 years to about 17 years.
Two gages, White Clay Creek at Newark, Delaware and Chesterville Branch near Crumpton, Maryland, currently have record lengths that are too short for peak-flow magnitude-frequency relations to be considered valid. As noted in table 1, a recurrence-interval estimate was made for White Clay Creek at Newark, Delaware using other methods, but comparisons of pre- and post-Floyd recurrence intervals are not applicable for either gage.
Historical, Outlier, and Discontinuous Record Perspectives
After the finalized peaks for Hurricane Floyd were included in the peak-flow records, revised flood-frequency analyses indicated that high-outlier thresholds were exceeded by the Floyd peak flows at several gages. Estimates of peak flow made by indirect measurements at three discontinued gages indicated that the Floyd peak flows were the largest ever recorded, thus requiring that the peak-flow magnitude-frequency relations be historically adjusted. Of the 28 active and discontinued gages, nine required adjustments to their peak-flow magnitude-frequency relations to account for high outliers or historical data. The adjustments that were applied to the peak-flow magnitude-frequency relations resulted in smaller recurrence-interval estimates for the Floyd peaks at six of the nine gages.
A high-outlier adjustment was required for the magnitude-frequency relation at the gage on Christina River at Coochs Bridge, Delaware because the addition of the peak flow from Hurricane Floyd was larger than any peak discharge recorded in the systematic record and caused the high-outlier threshold to be exceeded. The initial recurrence-interval estimate for the Floyd peak flow for this gage was determined to be between 100 and 500 years, but likely closer to 500 years. After including the Floyd peak flow and adjusting the relation to account for the high outlier, the recurrence interval was estimated to be 165 years, as shown in figure 4 (W.O. Thomas, Jr. and others, USGS, written communication, 1998).
Figure 4 illustrates some significant differences in magnitude-frequency relations between pre-Floyd and post-Floyd conditions at this gage. Between the 10-percent (10-year flood) and 0.2-percent (500-year flood) exceedance probabilities, the unadjusted curves differ from 8 to 30 percent. When adjustments are applied to the post-Floyd magnitude-frequency relation, the 10-percent and 0.2-percent exceedance probabilities differed by an additional 0.2 to 4.6 percent, respectively.
High-outlier adjustments were required for the magnitude-frequency relations at the discontinued gage on White Clay Creek above Newark, Delaware and at the active gage on White Clay Creek near Newark, Delaware. The relation for the gage on White Clay Creek above Newark, Delaware also met the requirement for historical-data adjustment to account for the combined effect of a large peak and a discontinuous systematic record. After adjustments were made, the estimated recurrence intervals for the Floyd peak flows at White Clay Creek above Newark, Delaware and White Clay Creek near Newark, Delaware were 170 years and 150 years, respectively. These recurrence-interval estimates are significantly less than the initial estimates of 500 years or greater.
The peak-flow record for the active gage on White Clay Creek at Newark, Delaware is currently 8 years long. Because 10 years of peak-flow data are required to establish a magnitude-frequency relation, recurrence-interval estimates based on the peak-flow data for this gage are not considered to be reliable. A recurrence-interval estimate can be made, however, on the basis of the estimated recurrence intervals for upstream and downstream gages on the same stream. Because the adjusted recurrence-interval estimates range from 150 to 170 years upstream and downstream of the gage, an estimate of 160 years can be made for the recurrence interval of the Floyd peak flow at this gage.
The active gage on Morgan Creek near Kennedyville, Maryland required an adjustment to the magnitude-frequency relation because the peak flow from Hurricane Floyd was almost twice the value of the high-outlier threshold. After the high-outlier adjustment was made, the recurrence interval for Hurricane Floyd was estimated to be 85 years. This represents a significant reduction from the initial recurrence-interval estimate of greater than 500 years.
The peak-flow magnitude-frequency relation for the active gage on Big Elk Creek at Elk Mills, Maryland was adjusted for historical data because data were available from a record peak in June 1884, nearly 50 years before the continuous-record gage was activated. The resulting recurrence-interval for the Floyd peak flow was estimated to be about 30 years, which is the same as the initial recurrence-interval estimate. This is probably because the 1884 peak is nearly twice as large as any peak flow recorded in the systematic record.
The peak-flow magnitude-frequency relation for the discontinued gage on Little Elk Creek at Childs, Maryland was adjusted because the Floyd peak flow exceeded the high-outlier threshold for the gage. The gage was discontinued in 1958, but peak-flow data are available for floods in 1989 and 1999. Because the systematic record is only 10 years long, the historical-data adjustment is critical to putting the 1989 and 1999 peaks in the proper statistical perspective. The initial Floyd peak-flow recurrence interval was estimated to be 500 years. After historical-data and high-outlier adjustments were made, the recurrence interval for the Floyd peak flow at this gage was estimated to be 155 years.
The peak-flow magnitude-frequency relation for the discontinued gage on Northeast Creek at Leslie, Maryland was adjusted to include the effect of historical data and to give the proper weight to the peak flow caused by Hurricane Floyd, which exceeded the high-outlier threshold and was nearly twice as large as any other peak in the record. The gage was discontinued in 1984 after 36 years of continuous monitoring. The peak flow caused by Hurricane Floyd in 1999 was estimated by use of stage-discharge rating extension techniques. The initial Hurricane Floyd recurrence-interval estimate was reported to be 500 years. After historical-data and high-outlier adjustments were made, the Floyd peak-flow recurrence interval was estimated to be 80 years.
The discontinued gage on Octoraro Creek near Rising Sun, Maryland required an analysis to determine the extent of flow regulation and a historical-data adjustment to the peak-flow magnitude-frequency relation. The peak flow caused by Hurricane Floyd in 1999 did not exceed the high-outlier threshold, but the gage has been discontinued since 1977. The historical peak-flow record also includes peaks in 1884 and 1918 that occurred prior to the beginning of the continuous peak-flow record in 1932. Since the length of the historical period associated with this gage is more than twice that of the systematic record, a historical-data adjustment was required to put the 1999 peak flow in the proper statistical perspective.
A water-supply reservoir was constructed and began storing water upstream from the gage in 1951; therefore, the effect of flow regulation on the peak-flow magnitude-frequency relation also must be determined. After considering the drainage area of the watershed, the size of the reservoir, and the increase in watershed storage from the reservoir, it was determined that peak flows with recurrence intervals of more than 2 years would be largely unaffected by flow regulation.
The Floyd peak-flow recurrence interval was initially estimated to be 15 years. After inclusion of the Floyd peak flow into the record and historical-data adjustment of the magnitude-frequency relation, the Hurricane Floyd peak-flow recurrence interval was estimated to be 11 years.
Selected References
- Beard, Leo R., 1975, Flood flow frequency techniques: University of Texas at Austin, Center for Research, Water Resources Report No. 119, 221 p.
- Benson, M.A., and Dalrymple, Tate, 1967, General field and office procedures for indirect discharge measurements: U.S. Geological Survey Techniques of Water-Resources Investigations, book 3, chap. A1, 30 p.
- Dillow, J.J.A., 1996a, Technique for estimating magnitude and frequency of peak flows in Maryland: U.S. Geological Survey Water-Resources Investigations Report 95-4154, 55 p.
- ______, 1996b, Technique for estimating magnitude and frequency of peak flows in Delaware: U.S. Geological Survey Water-Resources Investigations Report 95-4153, 26 p.
- Helsel, D.R., and Hirsch, R.M., 1992, Statistical methods in water resources: Amsterdam, Netherlands, Elsevier Science Publishers, 522 p.
- Interagency Advisory Committee on Water Data, 1982, Guidelines for determining flood flow frequency: Water Resources Council Bulletin 17B, 28 p.
- James, R.W. , Jr., Saffer, R.W., Pentz, R.H., and Tallman, A.J., 2002, Water resources data, Maryland and Delaware, water year 2001, Volume 1. Surface-water data: U.S. Geological Survey Water-Data Report MD-DE-01-1, 448 p.
- Kennedy, E.J., 1984, Discharge ratings at gaging stations: U.S. Geological Survey Techniques of Water-Resources Investigations, book 3, chap. A10, 59 p.
- Tallman, A.J., and Fisher, G.T. , 2001, Flooding in Delaware and the Eastern Shore of Maryland from Hurricane Floyd, September 1999: U.S. Geological Survey Fact Sheet FS-073-01, 4 p.
- Water Resources Council, Hydrology Committee, 1967, A uniform technique for determining flood flow frequencies: Water Resources Council Bulletin 15, 15 p.
Editor: Valerie M. Gaine
Graphics and design: Timothy W. Auer
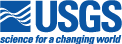
For further information contact:
District Chief
U.S. Geological Survey
8987 Yellow Brick Road
Baltimore, MD 21237
Visit the Maryland-Delaware-D.C. District Homepage on the World Wide Web at:
http://md.water.usgs.gov
USGS Fact Sheet FS-152-02
Go to top of page
Maintainer:webmaster@md.water.usgs.gov
|