Core OL-92 from Owens Lake, southeast California
Sediment Size Analyses of the Owens Lake Core
- Kirsten M. Menking
- Hannah M. Musler
- Earth Sciences Board, University of California, Santa Cruz
- Jeffrey P. Fitts
- James L. Bischoff
- U.S. Geological Survey, Menlo Park, California
- Robert S. Anderson
- Earth Sciences Board, University of California, Santa Cruz
Contents:
Abstract
Sedimentary analyses of a 323-m core from Owens Lake, southeastern
California, show variations on many different time scales. Grain size
analysis was determined on point samples taken at 2 to 3 m intervals down
the core. In addition, sand plus gravel, silt, and clay percentages were
measured on 3.5-m-long channel samples to supplement point sample grain
size data. The upper 195 m of sediment consist of interbedded fine silts
and clays. The lower 128 m, however, comprise interbedded silts and fine
sands. Fluctuations in mean grain size of sediments in the top 200 m of
the core correlate well with variations in carbonate content of channel
samples.
Introduction
Owens Lake is the first in a chain of lakes lying to the east of the
Sierra Nevada. The lake, which lies at the southern end of the
topographically closed Owens Valley, receives runoff primarily from the
Sierra Nevada via the Owens River and its tributaries. During "pluvial"
(high ratio of precipitation to evaporation) periods, Owens Lake exceeded
the confines of the southern Owens Valley and spilled over a sill into the
China and Searles Lake basins (Smith, 1984). During drier periods, the
lake shrank and remained nearer to or at levels lower than the spillover
sill. In this paper, we report results of grain size analyses conducted
on sediments from a 323-m-long core of Owens Lake which reflect the
variations in pluvial-interpluvial climate in the Sierra Nevada.
Sample Types
Two types of samples were taken from the Owens Lake core for sedimentary
analyses. "Point samples," which represent about 2 to 3 cm of core
length, were collected at the drill site at 1 to 2 m spacings down the
core. These samples comprise about 60 g of bulk sediment and have been
used to determine water content, pore water chemistry, organic carbon and
carbonate content, and grain size; grain size is reported here. "Channel
samples" were produced in the laboratory and represent integrated ribbons
of sediment, each spanning 3.5 m of core and comprising about 50 g of
sediment. Organic carbon/carbonate, grain size, clay mineralogy and bulk
chemical analyses have been performed on these samples; grain size is
reported here.
Methods
Grain Size Analysis--Point Samples
About 10 g of each point sample were placed in a beaker with 100 ml of
deionized water. The samples were lightly disaggregated with a glass
stirring rod. To remove carbonate and organic material, 150 ml of Morgan's
solution (1 L solution of deionized water, acetic acid, and sodium
acetate, containing 27 ml of glacial acetic acid buffered to pH 5 with 82
g of sodium acetate) and 20 ml of 30 wt.% H2O2 were added (Note: we have
assumed that fine grained carbonates were chemically precipitated in the
lake rather than detrital in nature. In order to assure complete
disaggregation of detrital clastics, then, it was necessary to remove any
binding cements). Morgan's solution was used in place of dilute HCl
because it is considered less damaging to clay minerals (Mark Johnsson,
personal communication), and the clay-sized fractions of some grain size
samples were collected for X-ray diffraction analysis. Samples sat in
this solution for two days and were stirred once every 8 to 12 hrs.
Heating to 150°C for 2 to 4 hours removed excess H2O2. Samples were
centrifuged for 30 minutes at 5000 rpm and the supernate discarded. The
remaining sediment was wet sieved to separate gravel, sand, and silt plus
clay fractions. U.S. standard sieves of 20 and 230 mesh (equivalent to -1
and 4 ø grain sizes) were used in the sieving process. The clay and silt
fraction of each sample was collected in a 1000 ml graduated cylinder.
Sands and gravels were poured into evaporating dishes and dried in an oven
at 60°C.
Dried gravels (-2 to -1 ø) were weighed and then sieved at 0.5ø intervals
to determine their grain size distributions. Sands (-1 to 4 ø) were
weighed and then split to arrive at 0.1 to 0.5 g representative
subsamples. These subsamples were introduced into specially-constructed
settling tubes owned by the U.S. Geological Survey Pacific Marine Geology
Branch. Each settling tube consists of a 2 m long section of PVC pipe
closed at one end and mounted vertically into a metal scaffold. The pipe
is filled with water to a level resulting in a grain fall height of 205
cm. Near the base of each tube, a small plastic disk is suspended from a
metal rod connected to a strain gauge. Sand grains are spread onto a
wetted plate which is lowered into the PVC pipe, initiating settling. As
settling proceeds, grains are deposited onto the plastic disk displacing
the metal rod downward. The resulting strain gauge voltage signal is
recorded on a chart recorder which plots voltage versus time. A suite of
calibration standards allows the conversion of these voltages to grain
sizes. Further descriptions of the settling tube can be found in
Galehouse (1971), Cook (1969), and in Felix (1969). Sand grain sizes were
determined at 0.5 ø intervals by this method.
To prevent flocculation, 5 ml of 5% sodium hexa-metaphosphate ("calgon")
dispersant solution were added to each clay and silt solution (<4 ø) and
the graduated cylinders filled to 1000 ml with deionized water. Each
solution was agitated for two minutes, then allowed to settle for 20
seconds, after which 20 ml were removed with a pipette submerged to 20 cm
depth (as described by Folk, 1968). To determine the weight of silt and
clay in each sample, the 20 ml aliquot was placed in a previously weighed
aluminum dish and allowed to dry for two days in an oven heated to 40°C.
After drying, the resulting mass value was multiplied by 50 to derive the
total mass of silt and clay held in the graduated cylinder. Each solution
was re-agitated for two minutes and another 20 ml sample drawn off after
20 seconds at 20 cm depth. The resulting aliquots were introduced into a
Cimax Inc. Model TSS8005-H Hydrophotometer at the U.S. Geological Survey
Pacific Marine Geology Branch.
The hydrophotometer uses both Stokes' Law of settling and the Beer-Lambert
Law to calculate grain sizes of sediments in suspension. The instrument
consists of a series of 12-cm-long tubes bored into a transparent slab of
plexiglass. The tubes have windows near their bases on each side of the
slab. Suspended sediment is introduced into each cell until all are
filled to a known height (we used a grain-fall height of 8 cm). A light
beam is shined through the windows of each tube into a detector on the
other side of the plexiglass slab, and the light transmittance through the
suspended sediment is calculated by a microprocessor. Light transmission
is measured at specified time intervals determined by Stokes' Law which
states that:

where d is the particle diameter, g is the acceleration due to gravity,
Delta-p is the difference in density between a sedimentary grain and the
liquid in which it is suspended, n is the viscosity of the liquid, and V
is the velocity at which the grain is falling (Jordan, Fryer and Hemmen,
1971). Given that the fall height of the grains is known, the settling
time can be calculated by dividing the fall height by the velocity at
which the grain is settling. In this way, the hydrophotometer can measure
light transmittance at those times when each successive grain size class
has fully settled out of suspension. Conversion of light transmittance
values to weight percents of individual grain size classes results from
the Beer-Lambert Law:

where I with subscript zero is the intensity of the beam emanating from
the light source, I with subscript n is the intensity of the beam
transmitted through the sample cell and hitting the detector, alpha is a
constant related to grain shape, c is the concentration of particles of
size dx in grams per cubic centimeter, L is the length that the light beam
traverses through the sample cell, Kx is an empirically derived constant
dependent on grain size, and Nx is the number of particles, per gram of
sample, of size dx (Jordan, Fryer and Hemmen, 1971; and Simmons, 1959).
Using this technique, grain sizes were determined at 0.5 ø intervals. For
a further explanation of hydrophotometers and the theory behind them, see
Jordan, Fryer and Hemmen (1971), and Simmons (1959). Because of the
rather lengthy nature of grain size analyses, only a few replicate
measurements have been produced as of yet. These analyses indicate an
average precision of about ±0.25 ø. Torresan (1987) has determined a
precision of about ± 0.5 ø for the hydrophotometer used in these analyses.
Grain Size Analysis--SDSZ program
The U.S. Geological Survey computer program SDSZ, written by Graig
McHendrie, welds the sieve, hydrophotometer and settling tube data sets
into one cumulative grain size curve on which the program calculates
statistical parameters such as mean and median grain size, sorting,
skewness, and kurtosis. The information necessary to construct the
cumulative curve consists of the weight fraction of each grain size class,
i.e. the weights of gravel, sand, and silt plus clay in each sample, and
the size distribution of each grain size class (as determined by sieving,
settling tube or hydrophotometer). The program employs the graphical
statistic equations developed by Folk and Ward (1957), Inman (1962), and
Trask (1930) and was used to analyze the Owens Lake core point-sample
grain size data. The statistical equations used by the program follow:
Folk and Ward (1957) statistics:

where ø with subscript 16 refers to the ø grain size at the sixteenth
percentile on the cumulative grain size curve, other subscripted numbers
referring to corresponding percentiles.
Inman (1962) statistics

Trask (1930) statistics

In plotting our data (see results section), we have chosen to use the Folk
and Ward (1957) statistics because they take the "tails" of the grain size
distribution into account (however, we have converted the ø units they use
into mm). According to these workers, sorting values from 1.00 to 2.00 ø
units are classified as poorly sorted. Skewness values between -0.10 and
+0.10 are defined as symmetrical while skewness values between +0.10 and
+0.30 are positively skewed. A positive skewness implies that samples are
weighted toward fine grains while negative skewness implies a weighting
toward coarse grains. Kurtosis is a ratio of the degree of sorting of the
central part of a grain size distribution to the sorting of the extreme
ends of the distribution and can also be thought of as the "peakedness" of
a grain size distribution. Values of 1.11-1.50 are classified as
"leptokurtic" meaning the central part of the distribution is better
sorted than the ends. Values between 1.50 and 3.00 are considered "very
leptokurtic" implying that the central part of the distribution is
extremely well sorted compared to the ends.
Sand, Silt and Clay Contents--Channel Samples
Sand plus gravel, silt, and clay contents were determined on ninety-one
3.5 m long channel samples. Ten-gram splits were subjected to the same
chemical treatments used on the point samples. Sand and gravel were
separated from silt and clay by wet sieving with a sieve of U.S. standard
mesh size 20 (-1 ø). The sands and gravels were collected together in
evaporating dishes and weighed after drying. Silts and clays were
collected in 1000 ml graduated cylinders. A scaled-down pipette analysis
was used to determine concentrations of coarse silt, fine silt and clay in
each sample (Galehouse, 1971): twenty milliliter aliquots were removed at
various time intervals based on the grain size of interest and the
temperature of the solution. These aliquots were dried and weighed and
their weights multiplied by 50 to estimate the weight of sample in each
size fraction.
Results
Grain-size analysis of point samples defines two distinct depositional
regimes (figure 1). With the exception of a coarse
grained oolite layer at the top of the core, mean grain size fluctuates
between 5 and 15 mm (clay- to silt-sized material) between 7 and 195 m in
depth. In contrast, between 195 m and the base of the core at 323 m, mean
grain size fluctuates between 10 mm (medium fine silt) and 50-100 mm
(coarse silt to fine sand). Sand plus gravel, silt, and clay contents
(measured as weight percents) of 3.5 m long channel samples broadly mimic
the point sample trends, with fine silts and clays predominating from the
top of the core to 190 m depth and then changing to coarser grained
material between 190 and 323 m depth (figure 2).
Clay content (figure 3) of the channel samples
varies widely from less than 10 weight percent to nearly 80 weight
percent. A closer look at the top 200 m of the core reveals periodicity
in mean grain size with depth in the point sample record
(figure 4). Comparison of the mean grain size
versus depth curve to the channel sample carbonate content versus depth
curve reveals great similarities in trends (figure
5). For example, lows in carbonate content are matched with very fine
mean grain sizes, while highs in carbonate content correspond to coarser
grain sizes.
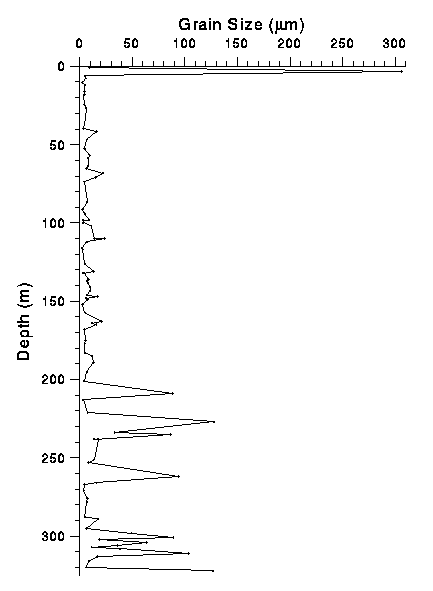
Figure 1: Mean grain size of point samples (in micrometers) versus
depth (in meters). Note the change in depositional style at 195m from
interbedded silts and clays at the top of the core to interbedded sands
and silts at the base. Mean grain size was calculated using Folk and
Ward's (1957) statistic and then converted to micrometers.

Figure 2: Sand plus gravel content of channel samples (in weight
percent) versus depth (in meters). Note the change in depositional style
at 190m depth from samples with little sand and gravel at the top of the
core to samples with high quantities of sand and gravel at the base of the
core.

Figure 3: Clay content (in weight percent) versus depth (in
meters). Note the great variability.
Table 1 lists mean grain size, sorting, skewness, and kurtosis for the top
195 m of the core (excluding the very coarse oolite layer at the top) and
the bottom 128 m of the core. The difference in depositional style
between these two core sections is evident in the mean grain size and
skewness parameters, while the sorting and kurtosis are much the same in
the two sections.
Table 1: Grain size parameters for the top 195 m of the core and the
bottom 128 m (Folk and Ward, 1957, statistical parameters).
Statistical Parameter top 195m bottom 128m
mean grain size (ø) 7.40 ± 0.79 5.73 ± 1.56
mean sorting (ø) 1.80 ± 0.43 1.90 ± 0.52
mean skewness 0.16 ± 0.14 0.04 ± 0.25
mean kurtosis 1.53 ± 0.39 1.41 ± 0.60
In the Owens Lake core, all sediments fall into the poorly sorted category
whether they are the fine grained sediments in the top of the core or the
coarser grained sediments of the lower core. Those sediments in the top
195 m of the core are positively skewed implying a weighting toward fine
grains. Sediments in the bottom 128 m show a basically normal grain size
distribution. Both sections of the core are leptokurtic.
Discussion
A particularly striking feature in the Owens Lake core is the change from
sediments composed largely of interbedded sands and silts in the lower
third of the core to sediments consisting primarily of silt and clay in
the upper two-thirds. It is possible that the lower, sandy section of the
core represents a shallow lake present in a drier climate. On the other
hand, it is also possible that the change in depositional style at 195 m
occurred as the result of tectonic factors, namely valley deepening or
uplift of the spillover sill with respect to the surface of the lake,
rather than from climate change. At this point, we do not have enough
information to determine the nature of the depositional change. Grain
size statistics collected to date show little difference between the
sediments from 0 to 195 m and those from 195 to 323 m.
Like the channel- and point-sample carbonate records, the mean grain size
record in the top 200 m of the core exhibits oscillation in deposition.
Mean grain size may reflect variations in lake level, with coarse-grained
materials deposited during times of lake lowstands, when the shore of
Owens Lake more closely approached our core site, and fine-grained
sediments deposited during highstands, when the shoreline was at a greater
distance from the core site.
References
- Cook, D.O. (1969) Calibration of the University of Southern California automatically recording settling tube. Journal of Sedimentary Petrology 39 781-786.
- Felix, D.W. (1969) An inexpensive recording settling tube for analysis of sands. Journal of Sedimentary Petrology 39 781-786.
- Folk, R.L., Petrology of Sedimentary Rocks. Austin, Texas: Hemphill's, 1968.
- Folk, R.L., and Ward, W.C. (1957) Brazos River bar, a study in the significance of grain-size parameters. Journal of Sedimentary Petrology 27 3-27.
- Galehouse, J.S. (1971) Sedimentation Analysis, in Carver, R.E., ed., Procedures in Sedimentary Petrology: New York, Wiley-Interscience, p.69-94.
- Inman, D.L. (1962) Measures for describing the size distribution of sediments. Journal of Sedimentary Petrology 22 125-145.
- Jordan, Jr., C.F., Fryer, G.E. and Hemmen, E.H. (1971) Size analysis of silt and clay by hydrophotometer. Journal of Sedimentary Petrology. 41 489-496.
- Simmons, G. (1959) The photo-extinction method for the measurement of silt-size particles. Journal of Sedimentary Petrology 29 233-245.
- Smith, G.I. (1984) Paleohydrologic regimes in the southwestern Great Basin, 0-3.2 my ago, compared with other long records of "global" climate. Quaternary Research 22 1-17.
- Torresan, M.E. (1987) A review and comparison of the hydrophotometer and pipette methods in the analysis of fine-grained sediment. U.S. Geological Survey Open-File Report 87-514
- Trask, P.D. (1930) Mechanical analysis of sediments by centrifuge. Economic Geology 25 581-599.
Appendix: Tables of observations and statistics
The tables are given as tab-delimited ASCII. Since the tab character
(ASCII 9) is used to delimit columns on each line, the values may not line
up as expected when displayed outside of a spreadsheet program. It is
expected that these tables will be imported into spreadsheet programs.
- Raw point sample grain size data
Raw point sample grain size data in the format accepted by the program
SDSZ. The first four columns, -2.0 phi through -0.5 phi, are gravel
weights in grams. The next twelve columns, -1.0 phi through 4.5 phi, are
cumulative percentages of sands measured with a settling tube. The
remaining 11 columns, 4.5 phi through 14 phi, are light transmission
values determined by hydrophotometer on silt and clay samples. Some
overlaps in grain size between different methods exists resulting in the
two values each for -1.0 phi, -0.5 phi, and 4.5 phi.
- Mass of gravel, sand, and silt plus clay used in each point sample grain size analysis
- Statistical measures of grain size
Statistical measures of grain size produced by the program SDSZ. FW
refers to statistics developed by Folk and Ward, 1957; I refers to
statistics developed by Inman, 1962; T refers to statistics developed by
Trask, 1930.
- Channel sample grain size data
HTML encoding by Peter Schweitzer