Core OL-92 from Owens Lake, southeast California
Geochemistry of sediments in Owens Lake Drill Hole OL-92
- James L. Bischoff
- Jeffrey P. Fitts
- John A. Fitzpatrick
- U.S. Geological Survey, Menlo Park, California
- Kirsten M. Menking
- Earth Sciences Board, University of California, Santa Cruz
- Bi-Shia W. King
- U.S. Geological Survey, Menlo Park, California
Contents:
Introduction
In historic time, throughout the Holocene, and during other dry periods of
the past (interglacials), Owens Lake was saline and alkaline. During
extreme wet periods (glacials), in contrast, the lake must have been
flushed and overflowing with fresh water as shown by down-stream evidence
at Searles Lake. The postulate of the present study was that the sediment
composition should reflect the cycling between glacials and interglacials
During the spring of 1992, a 323 m core (7.6 -cm diameter) was recovered
from Owens Lake in order to obtain a record of climate history in this
part of the Great Basin. Drilling was carried out in three sub-sections:
from the surface to 7.16 m (OL-923), from 5.49 to 61.37 m (OL-92-1) and
from 61.26 to 322.86 m (OL-92-2), together representing about 800 kyr of
sedimentation (Smith, 1993). Sediments were analyzed for chemistry, grain-
size, clay-mineralogy, radiocarbon, and pore water volume and
composition. We report here on the sediment chemistry, which includes
analyses for grain-density organic carbon, carbonate, cation-exchange
capacity (CEC), major oxides and minor elements. Results for the other
parameters are reported in companion articles (Bischoff et al 1993a,
Bischoff et al, 1993b, Menking et al, 1993a and 1993b).
Sampling
Both channel and point samples were taken for from the drill core
geochemical studies. Channel samples, which are composite strip samples,
were taken in a continuous series to represent the entire sedimentary
column without gaps (total of 91 samples,) and they constitute the major
focus of the geochemical analyses. This methodology avoids the bias of
single point samples which may not represent the entire sedimentary unit
from which they were taken (for point-sample studies of OL-92 see Bischoff
et al, 1993a, b, and Menking et al, 1993). The advantage of the channel
samples is that each represents a smoothed or running-mean of conditions
represented by the time span of the sample, that no important events are
missed, and that geochemical budget calculations can be carried out. The
time-resolution of such samples is inverse to the thickness of the section
sampled. In the present, study we took strip samples of approximately 3 m
length, each deemed to represent about 7000 years of deposition based on
the thickness of section above the Bishop Tuff at 309 m (Smith, 1993).
Samples were taken longitudinally from the working half of the core with a
U-shaped spatula. The resulting sample is a continuous semi-cylindrical
strip about 1.5 cm wide and 1 cm deep and about 3 m long. The core tube
used in drilling was about 4.5 m long. After each drilling "run" of 4.5 m
or less, the drill-string was pulled from the hole and the sediment
retrieved from the core tube. In the field, the sediment from each run
was divided into 1.5 m "slugs" for convenience of handling. These slugs
were labeled in sequence A through D for wrapping, preservation, and
transportation to the laboratory. In the majority of runs only two slugs
(A and B) were retrieved. The labeling of the channel samples, therefore,
first gives the drilling run (1 to 110) and secondly the alphabetical
"slugs" (A through D) within the run. For example, a typical channel
sample represents two slugs and will have a number like 20 A+B, which
translates to run 20, slugs A and B.
The point samples were taken during the drilling operations at every 2 to
3 m (120 samples). They were specially preserved and used for
determination of water content and pore water chemistry as reported in the
accompanying report (Bischoff et al, 1993a). These samples were also
analyzed for organic carbon and for carbonate content (but not the other
components). These results are reported here to supplement similar
analyses of the channel samples.
Laboratory Procedures
A. Initial processing and splits
The wet channel-samples (150-200 cc each) were placed in 250 ml centrifuge
bottles and suspended in distilled water to flush interstitial salts. The
flushing and centrifugation was repeated (typically to three times) until
the salinity of the flush was less than 0.1 % as determined by
refractometer The samples were then dried at 60°C and mechanically
homogenized by light grinding in a ceramic mortar. A 5-gram aliquot was
split for Cs treatment and major element analysis. The remaining sample
was used for determination of grain-density, organic carbon and carbonate
(org C and CO2), XRD determination of carbonate minerals, and acid-
leachable Mg, Ca, and Sr. For the point samples, 1-2 cc aliquots of the
wet sediment were dried and ground for determination of organic carbon and
carbonate, taken from the same aliquot used for determination of water
content reported in Bischoff et al (1993a).
B. Cs treatment and major elements
An aqueous CsCl solution was used to displace all exchangeable cations in
the sample with Cs ion. Thus, the amount of Cs taken up by the sample is
a measure of its cation-exchange capacity (CEC). CEC, in turn, should be
a measure of the relative abundance of weathering zone clay minerals such
as smectite, and a measure of warm weathering conditions. The Cs split
was suspended in 200 mls of 0.09 molal CsCl solution and periodically
agitated for 24 hours, after which it was collected on filter paper and
rinsed of excess solution with distilled water. The sample was then dried
at 60°C after which Cs was analyzed by the U.S. Geological Survey
Analytical Laboratory (Bi-Shia King and P. Lamothe, analysts) using energy-
dispersive X-ray fluorescence spectrometry (XRF). Standards were mixtures
of an analyzed granite (1 ppm Cs) and a Cs-saturated standard smectite
(13% Cs), previously analyzed for absolute Cs content by ICP. The energy-
dispersive XRF analysis was non-destructive so the sample was retrieved
and analyzed in turn for the major rock-forming oxides SiO2, Al2O3, Fe2O3,
MgO, CaO, Na2O, K2O, TiO2, P2O5, and MnO, by dispersive XRF. LOI (loss on
ignition at 900°C) was determined gravimetrically as part of the sample-
fusion step in the XRF analysis. Dispersive XRF and LOI analyses were
performed by the U.S. Geological Survey Analytical Laboratory (D. Hopkins,
analyst). Because the samples contain significant amounts of X-ray
absorbing Cs, which is lacking in the usual XRF analyzed-rock standards,
oxide sums (including Cs2O) plus LOI were systematically somewhat low
(95±2%). Because LOI is unaffected by the Cs, we corrected the sum
discrepancy by normalizing the major oxides plus Cs2O to sum to 100 %
minus LOI. A split of each channel sample was also analyzed for minor
elements by semi-quantitative optical emission spectroscopy (D. Siems,
analyst).
D. Other properties
Organic carbon and carbonate were analyzed from the bulk sample by
standard coulometry (UIC, Inc. Coulometrics Model 5010 CO2 Coulometer)
which successively measures the carbonate as CO2 released by strong acid
attack and then the total carbon in the sample. Org C is calculated as
the difference between total carbon and carbonate carbon (see Engleman,
Jackson and Norton, 1985; and Huffman, 1977). Splits of bulk samples were
leached in 3 molar HCl overnight for analysis of acid-leachable cations.
After centrifugation, the supernate was analyzed for Ca, Mg and Sr by
standard atomic absorption spectroscopy. Standard XRD scans of powder-
mounts were performed on a selection of 36 carbonate-rich samples to
identify the major carbonate minerals. Grain densities were determined on
six composites of the channel samples using a gas comparison pyncnometer
(Beckman model 930). After weighing, the sample's volume was measured by
the pyncnometer which maintains equal and precisely measured pressures of
helium gas in matched sample and reference cylinders. The amount of
helium gas displaced by the sample in the sample cylinder is accurately
measured by pressure deviations. Sample density is simply the weight
divided by the volume.
Results and Discussion
Analytical results for carbonate, organic carbon, carbonate mineralogy,
and leachable Ca, Mg, and Sr are given in tables 1 and 2; for major
oxides, LOI, and Cs2O in table 3; minor elements in table 4; and densities
in Table 5. Table 6 summarizes bulk sediment contents of CaCO3, organic
carbon, CEC, and density, while table 7 summarizes the bulk sediment
composition. Carbonate (CO2,) and organic carbon (org C) show cyclic
covariation down the core (Fig. 1). It is remarkable that both point
samples (representing ca 40 yrs each) and channel samples (representing ca
8000 yrs each) show almost coincident patterns, indicating that even on
the small scale of the point samples the sediments are representative of
larger thicknesses of sediment. This observation points to rather slowly
changing homogeneous conditions rather than widely fluctuating conditions
on a decade scale. Based on radiocarbon results (Bischoff et al, 1993b),
maximum glacial conditions at 17 to 25 kyrs occur at 10 to 17 m depth in
the core, where CO2 and org C display conspicuous and sharp minima, very
close to zero values. These results suggest that at glacial maxima the
lake was overflowing with cold fresh water and was relatively non-
productive. Five similar minima in these parameters continuing down the
core to about 230 meters are interpreted as successively older glacial
maxima. Conversely, the recurring and broader maxima in CO2 and org C are
interpreted to represent full interglacial conditions during which the
lake was the terminus and was saline and biologically highly-productive.
Below 230 m there is a striking change in depositional conditions from
silty clays to the prevalence of thick sandy units as described in the
accompanying reports (Smith, 1993; Menking et al, 1993), which may signal
irregular fluctuations from lacustrine to non-lacustrine conditions. For
the section above 230 m, however, lacustrine conditions apparently
prevailed. The narrowness of the carbonate minima and the relative
thickness of the maxima down the core suggest that closed lake conditions
were only periodically interrupted by brief periods of overflow (Fig. 1).
XRD results on selected samples (Table 1) indicate that calcite is the
dominant carbonate mineral, with detectable dolomite occurring in about a
third of the samples, and aragonite in only two. The relative amounts of
Ca and Mg carbonates, calculated by balancing the analyzed acid-leachable
Ca and Mg against analyzed CO2, indicates that the MgCO3 component
accounts for only about 5 mole % of the total carbonate with the rest
being Ca. This suggests that about 86 % of the total acid-leachable Mg is
actually non-carbonate (Fig. 2) which we postulate to be authigenic
Mg-hydroxysilicates, varyingly crystalline and amorphous, and including such
phases as sepiolite, kerolite, and stevensite (Jones, 1986). Such acid-
soluble authigenic phases form in saline lakes by reaction of dissolved Mg
and silica in alkaline solution, both reacting directly with each other
and/or reacting with preexisting clastic phyllosilicates that were either
suspended in the water column or at the sediment-water interface. Thus,
calculating averages from Tables 1 and 3 indicates that of the total bulk
Mg, 9% is as carbonate, 57% as acid-soluble authigenic silicate, and 34%
is in the non-leachable clastic component. Fig. 2 shows that abundance of
both carbonate-Mg and authigenic Mg-silicate follows that for total-
carbonate and that both Mg phases are likely indicators of saline and
alkaline conditions.
The Cs2O content of each channel sample (Table 3) is a function of both
the relative percent clay in the sample, and of the CEC of the clays
therein. The parameter of interest is the CEC of the clay-fraction, which
is obtained from the analytical results by normalizing the Cs content
(carbonate-free basis) to the weight fraction of the <2 µ component of the
sample (reported in Menking et al,1993b). The variation of this clay-
normalized CEC with depth (Fig. 3) shows a remarkable correlation with
carbonate down to 230 m. As with CaCO3, CEC shows a conspicuous and sharp
minimum coinciding with the glacial maximum at 10-20 m, and others
successively deeper in the core at the same points of carbonate minima.
This correlation suggests that during glacial maxima the clay-size
material has a low exchange capacity, perhaps representing a glacial rock-
flour component. Below 230 m, normalized CEC shows dampened cyclical
variation that are de-coupled from the carbonate pattern. If CEC is a
reflection of drainage-basin conditions rather than deposition-basin
conditions, then CEC cycles might represent climatic cycles even though
the depositional basin is alternating between lacustrine and non-
lacustrine conditions. The average CEC of clay material in the core is 32.
7 meq/100 g (table 6) which compares to a range of 80-150 meq/100g for
pure smectites and to about 10-40 meq/100g for pure illite (Grimm, 1968,
p. 189). Menking et al (1993b) report that illite and smectite are the
dominant clay minerals in the Owens sediment. During the interglacials,
CEC reaches values within the pure smectite range, while during the
glacials CEC is within the range of pure illite.
Major oxides and minor elements (Tables 3 and 4) show little systematic
variation with depth in the core. Most variation is explainable by
variations of sand:silt:clay proportions. Table 5 shows that grain
density is remarkably constant at 2.63±0.05 g/cc. Table 6 shows that
average Owens Lake sediment has about 12.5 % CaCO3 and about 0.92 %
organic carbon. The average bulk composition, normalized after removing
carbonate, organic carbon, and acid-soluble Ca and Mg (Table 7), shows
remarkable similarity in all major oxide components to granodiorite, the
predominant rock of the Sierran batholith. The minor components of Owens
sediment (Table 7) show the same strong granodiorite affinity and a
contrast to average shale with the single exception of Zr (56 ppm versus
500 in granodiorite and 120 ppm in shale). Granodioritic Zr is primarily
zircon which fractionates with the sand fraction, and therefore, is
relatively depleted in the dominantly silt-clay sediment of the lake
basin. Triangular diagrams of all the data points show the same general
affinity to granodiorite, but with small scale variability attributable
to grain-size variations. The Al2O3-Na2O-K2O plot (Fig. 4a) shows a
grouping of points towards the Al2O3-rich side but close to the Lamark
granodiorite, a rock typical of the drainage to Owens Lake from the east-
central Sierra Nevada (Bateman et al, 1963). The Fe2O3-Na2O-K2O plot
(Fig. 4b) shows a pattern elongate with respect to the Fe2O3 apex with the
Lamark granodiorite at the center of the trend. Samples relatively
enriched in Fe2O3, tending toward average shale, are clay-rich samples,
while those in the opposite direction are rich in arkosic sand. The
Fe2O3-SiO2-Al2O3 plot (Fig 4c) shows an elongate trend with respect to
the SiO2 apex with the Lamark granodiorite at the mid-point. The
SiO2-depleted side trends toward average shale and represent the more
clay-rich samples, while the SiO2-enriched represent sandy units. The
bifurcation of the trend on the SiO2-enriched side distinguishes between
quartz sands and arkosic sands.
Tables
- Analyses of sediment channel-samples
Analyses of sediment channel-samples from OL-92 (1, 2, and 3) for wt %
carbonate (CO2), wt % organic carbon (org C) wt % acid-leachable Mg, Ca,
ppm Sr, and XRD carbonate mineralogy (min; c=calcite, d=dolomite,
a=aragonite). Sample designation refers to drilling run (numerical) and
slug (alphabetical). Depth refers to midpoint of sample from surface, and
range refers to half-length of channel sample, both in meters (i.e.,
depth ± range indicates depth span of sample)
- Carbonate and organic carbon
Carbonate (as CO2), and organic carbon (org C) content of point-samples of
Owens Lake OL-92 drill hole.
- Major oxides, Cs2O, and ignition loss (LOI)
Major oxides, Cs2O, and ignition loss (LOI) as wt. % of dry sediment
channel-samples from OL-92 drill hole. Sample designations are the same
as listed in Table 1 as indicated by depth to mid-ponts. Analyses by the
USGS Analytical Laboratory; major oxides by x-ray fluorescence
spectroscopy, LOI (900°C) by gravimetry (D. Hopkins, analyst), Cs2O by
non-dispersive X-ray fluorescence spectrsocopy (P. Lamothe and Bi-Shia
King, analysts).
- Minor-element composition
Minor-element composition (ppm) of sediment channel-samples from OL-92
drill hole. Analyses by semi-quantitative optical emission spectroscopy.
USGS Analytical Laboratory (D.F. Siems, analyst)
- Densities of composite samples
Densities of composite samples from the OL-92 core. The composition of
these samples is as follows:
- OL-92-1, 6A+7A to 13A+B+C
- OL-92-1, 14A+B to 27A+B plus OL-92-2, 1A+B to 9A+B
- OL-92-2, 10A+B to 33A+B
- OL-92-2, 34A+B to 60B+C
- OL-92-2, 61A+B to 93A+94A
- OL-92-2, 95A+B to 110
- Averaged characterstics of sediments
Averaged characteristics of sediments from Owens Lake OL-92 drill hole. Values are reported as mean ± one standard deviation.
mean standard deviation units
CaCO3 12.5 10.9 weight percent
org C 0.92 0.87 weight percent
CEC (cfb) 32.7 6.8 meq/100g
grain density 2.63 0.05 g/cc
Cation exchange capacity is given for the clay size-fraction on a carbonate-free basis.
-
Comparison of OL-92 sediment with granodiorite and shale
Averaged composition of sediment from Owens Lake OL-92 drill hole, on an
acid-insoluble basis (carbonate-free), compared to granodiorites and
average shale.
Notes:
- GSP-1 is a granodiorite collected near Silver Plume, Colorado (Flanagan, 1976).
- Lamarck granodiorite was collected from east central Sierra Nevada, California (Bateman et al, 1963).
- Shale oxides are from Clark (1924), minor elements from Rankama and Sahama (1950).
Figures
- Carbonate (as CO2) and organic carbon content of sediments from Owens Lake
drill hole OL-92. Solid lines are from channel samples, dotted lines from
point samples.
(PostScript version)
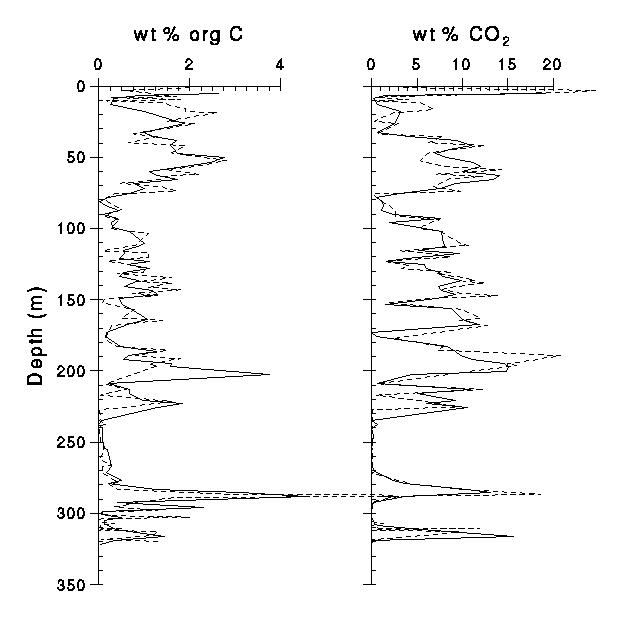
-
Relative amounts of CaCO3, MgCO3, and acid-soluble Mg-silicate.
Relative amounts of CaCO3, MgCO3, and acid-soluble Mg-silicate components
on a moles/100g basis of sediments from Owens Lake drill hole OL-92.
-
CEC and CO2 vs depth
Covariation of cation exchange capacity (CEC) of clay fraction and bulk
carbonate content (CO2) with depth in Owens Lake drill hole OL-92.
-
Major oxide composition
Triangular diagrams showing major oxide composition of sediments from
Owens Lake drill hole OL-92 compared to average shale and diorite (GSP-1
and Lamarck, see table 7). A. Al-Na-K. B. Fe-Na-K. C. truncated
triangle (lower left corner) of Fe-Si-Al.
References
- Bateman, P.C., Clark, L.D., Huber, N.K., and Moore, J.G. (1963) The Sierra Nevada Batholith, U.S. Geological Survey Prof. Paper 414-D.
- Bischoff, J.L., Fitts, J.P., and Menking, K., (1993a) Sediment pore-waters of Owens Lake drill hole OL-92. U.S. Geological Survey Open-File report 93-683
- Bischoff, J.L., Stafford, T. W., and Rubin, M. (1993b) AMS radiocarbon dates on sediments from Owens Lake drill hole OL-92. U.S. Geological Survey Open-File report 93-683
- Clark, F.W. (1924) The Data of Geochemistry, U.S. Geological Survey Bulletin 616.
- Engleman, E.E., Jackson, L.L. and Norton, D.R. (1985) Determination of carbonate carbon in geological materials by coulometric titration. Chemical Geology 53 125-128.
- Flanagan, F.J. (1976) Descriptions and analyses of eight new USGS rock standards, U.S. Geological Survey Prof. Paper 840.
- Grim, R.E., (1968) Clay Mineralogy, McGraw-Hill, New York, 596 p.
- Huffman, Jr., E.W.D. (1977) Performance of a new automatic carbon dioxide coulometer. Microchemical Journal v. 22, p. 567-573.
- Jones, B. (1986) Clay mineral diagenesis in lacustrine sediments, in F.A. Mumpton, eds., Studies in Diagenesis, U.S. Geological Survey Bulletin 1578, p. 291-300.
- Menking, K., Musler, H.M., Fitts, J.P., Bischoff, J.L., and Anderson, R.S. (1993a) Sediment size analyses of the Owens Lake core. U.S. Geological Survey Open-File report 93-683
- Menking, K., Musler, H.M., Fitts, J.P., Bischoff, J.L., and Anderson, R.S. (1993b) Clay mineralogical analyses of the Owens Lake core. U.S. Geological Survey Open-File report 93-683
- Rankama, K., and Sahama, T. (1950) Geochemistry, Univ. Chicago Press, 911 p.
- Smith, G.I., (1993) Core OL-92. U.S. Geological Survey Open-File report 93-683
U.S. Department of Interior, U.S. Geological Survey
URL of this page: https://pubs.usgs.gov/openfile/of93-683/4-geochem/1-sediment/sed-geochem.html
Maintained by: Eastern Publications Group Web Team
Last modified: 03.01.01 (krw)