The results of analyses completed so far show a consistent picture: the samples
are largely composed of gypsum, cellulose, and miscellaneous materials common
in a building, with minor asbestiform minerals. However, one sample analyzed,
the coating on a steel beam, indicates the presence of a significant abundance
of chrysotile asbestos (as much as 20% by volume). The confirmed abundant chrysotile
sample and the potential pockets of chrysotile indicated in the AVIRIS mineral
maps indicates that asbestos can be found in localized concentrations. Thus,
appropriate precautions should be taken when handling debris, especially coatings
on metal beams.
Sample results are summarized in Table 1, below. To see the full resolution
SEM image and description, click on the image in the table. In the spectroscopy
column in the table below, CH indicates organic compounds, including paints
and plastics. Fe2+ indicates minerals or materials containing ferrous iron. Amounts are qualitative
and indicate (from low to high) trace as (tr), weak as (wk), and strong as
(str). No indication indicates between weak and strong.
The composition of samples collected in the WTC area, as indicated by spectroscopy,
XRD, SEM, and from the visual examination during splitting of the samples,
show similarities, yet each sample shows differences. Thus, while the samples
appear to be a "grey dust", the data indicate the dust was not well mixed. The sample analyses and the
AVIRIS mapping results agree in this regard.
Sample Number |
Spectroscopy |
XRD |
SEM |
Leach
pH |
Location |
WTC01-01 (calibration) |
|
|
|
|
asphalt from NJ |
WTC01-02 |
gypsum,
muscovite (tr),
CH,
Fe2+ |
Quartz - Minor
Calcite - Minor
Gypsum - Minor
Dolomite - Trace
Anhydrite - Trace
Orthoclase - Trace
*Major amorphous material |
|
10.1 |
Water &
Vietnam Vet. Plaza
4506052N
583559E |
WTC01-03 |
gypsum,
muscovite (tr),
CH,
Fe2+ (wk) |
Quartz - Major
Gypsum - Minor
Calcite - Minor
Anhydrite - Trace
*Major amorphous material |
 |
9.51 |
Battery Park,
east central
4506123N
583217E
drainage disc |
WTC01-04 |
gypsum,
muscovite and/or portlandite (tr),
CH,
Fe2+ (wk) |
Calcite - Major
Gypsum - Minor
Anhydrite - Minor
Quartz - Trace
Muscovite - Trace
Microcline - Trace
*Major amorphous material |
|
|
Battery Park,
NE end
4506284N
583233E |
WTC01-05 |
gypsum,
muscovite and/or portlandite (tr),
CH,
Fe2+(wk) |
Calcite - Major
Quartz - Minor
Gypsum - Minor
Anhydrite - Minor
Calcium Sulfate Hydrate - Trace
*Major amorphous material |
|
9.9 |
Broadway &
Wall St.
from Bank of NY |
WTC01-06 |
gypsum,
muscovite and/or portlandite (tr),
CH,
Fe2+ |
Calcite - Minor
Quartz - Minor
Gypsum - Minor
Anhydrite - Minor
Muscovite - Trace
Calcium Sulfate Hydrate - Trace
*Major amorphous material |
|
9.65 |
Greenwich &
Morris |
WTC01-07 |
gypsum,
muscovite and/or portlandite (tr),
CH,
Fe2+ |
Gypsum - Minor
Calcite - Minor
Quartz - Minor
Anhydrite - Minor
Muscovite - Trace
Microcline - Trace
*Major amorphous material |
|
|
Rector &
Greenwich
from sidewalk |
WTC01-08 |
chrysotile
gypsum
CH,
Fe2+ (str) |
Calcite - Minor
Chrysotile - Minor
Gypsum - Minor
Talc - Trace
Anhydrite - Trace
Microcline - Trace
Rozenite - Trace
*Possible trace rozenite
*Major amorphous material |
 |
|
West End &
Vesey, steel girder |
WTC01-09 |
gypsum,
muscovite,
CH,
Fe2+ (str) |
Calcite - Minor
Gypsum - Minor
Thaumasite - Minor
Quartz - Trace
Calcium Silicate - Trace
Larnite - Trace
*Major amorphous material |
|
10.8 |
West End &
Vesey, steel girder |
WTC01-10 |
gypsum,
muscovite (tr),
CH,
chrysotile (tr)
Fe2+ |
Gypsum - Minor
Calcite - Minor
Quartz - Minor
Anhydrite - Trace
Muscovite - Trace
*Major amorphous material |
|
|
West End &
Vesey |
WTC01-11 |
gypsum,
muscovite (tr),
CH,
Fe2+ |
Quartz - Minor
Gypsum - Minor
Calcite - Minor
Anhydrite - Minor
Microcline Trace
Muscovite - Trace
Chrysotile - Trace
Calcium Sulfate - Trace
*Major amorphous material |
|
|
West End &
Barclay |
WTC01-12 |
gypsum,
muscovite (tr),
CH,
Fe2+ |
Quartz - Minor
Calcite - Minor
Gypsum - Minor
Anhydrite - Minor
Muscovite - Trace
Chrysotile - Trace
*Major amorphous material |
|
|
West End &
Warren |
WTC01-13 |
gypsum,
muscovite (tr),
CH,
Fe2+ (wk)
possible trace chrysotile |
Quartz - Minor
Calcite - Minor
Gypsum - Minor
Anhydrite - Minor
Muscovite - Trace
*Major amorphous material |
|
|
Broadway &
Barclay |
WTC01-14 |
gypsum,
muscovite,
CH,
Fe2+ (wk),
chrysotile (wk) |
Gypsum - Minor
Calcite - Minor
Quartz - Minor
Anhydrite - Trace
Muscovite - Trace
*Major amorphous material |
|
9.68 |
Church &
Barclay
from a windowsill |
WTC01-15 |
gypsum,
muscovite,
CH,
Fe2+ (wk)
possible trace chrysotile |
Gypsum - Major
Anhydrite - Minor
Quartz - Minor
Calcite - Minor
Muscovite - Trace
*Major amorphous material |
|
10 |
Maiden Ln. &
Church |
WTC01-16 |
gypsum,
muscovite and/or portlandite (tr),
CH,
Fe2+ (wk) |
Quartz - major
Gypsum - Major
Calcite - Minor
Anhydrite - Trace
Albite - Trace
Orthoclase - Trace
*Major amorphous material |
|
8.22 |
Broadway &
Dey |
WTC01-17 |
gypsum,
muscovite and/or portlandite (tr),
CH,
Fe2+ |
Quartz - Minor
Gypsum - Minor
Calcite - Minor
Anhydrite - Minor
Calcium Sulfate Hydrate - Trace
Muscovite - Trace
*Major amorphous material |
|
9.47 |
Greenwich &
Albany |
WTC01-18 |
gypsum,
portlandite (tr),
CH,
Fe2+ (wk) |
Gypsum - Minor
Calcite - Minor
Quartz - Minor
Muscovite - Minor
Anhydrite - Minor
Portlandite - Trace
Bassanite - Trace
Chrysotile - Trace
*Possible trace chrysotile
*Major amorphous material |
|
|
Washington &
Albany |
WTC01-19 |
gypsum,
muscovite (tr),
CH,
Fe2+ (wk) |
Gypsum - Minor
Calcite - Minor
Quartz - Minor
Muscovite - Trace
Anhydrite - Trace
Bassanite - Trace
*Major amorphous material |
|
|
South End &
Ceder |
WTC01-20 |
gypsum,
muscovite and/or portlandite (tr),
CH,
chrysotile (tr),
Fe2+ (wk) |
Quartz - Minor
Calcite - Minor
Gypsum - Minor
Anhydrite - Minor
Portlandite - Trace
Bassanite - Trace
Muscovite - Trace
Clinochrysotile - Trace
*Very slight possibility of a trace of chrysotile
*Portlandite and bassanite are trace to minor
*Major amorphous material |
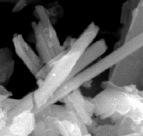 |
11.8 |
Liberty &
South End
2 World Financial Center: indoor sample |
WTC01-21 |
gypsum,
muscovite,
CH,
chrysotile (tr)
Fe2+ (wk) |
Gypsum - Minor
Quartz - Minor
Calcite - Minor
Anhydrite - Minor
Microcline - Trace
Clinochrysotile - Trace
Muscovite
*possible trace Chrysotile
*Major amorphous material |
|
9.98 |
Directly West of WTC at
ferry dock and ped. mall |
WTC01-22 |
gypsum,
muscovite,
CH,
Fe2+ (wk) |
Gypsum - Major
Anhydrite - Minor
Quartz - Minor
Calcite - Minor
Muscovite - Trace
Paragonite - Trace
Lizardite - Trace
Magnesionhornblende - Trace
Clinochrysotile - Trace
*possible trace (<.5%) chrysotile and/or Lizardite
*trace amphibole (<.5%)
*Major amorphous material |
 |
10.4 |
1/2 way between WTC and
Ferry Dock along ped. mall
from a windowsill |
WTC01-23 |
gypsum,
muscovite (tr),
CH,
chrysotile (tr)
Fe2+ (wk) |
Gypsum - Minor
Muscovite - Minor
Calcite - Minor
Quartz - Minor
Anhydrite - Trace
Bassanite - Trace
*Major amorphous material |
|
|
Broadway &
Murray |
WTC01-24 |
gypsum,
muscovite (tr),
CH,
chrysotile (tr)
Fe2+ (wk) |
Gypsum - Minor
Calcite - Minor
Anhydrite - Minor
Dolomite - Minor
Muscovite - Minor
Quartz - Trace
Microcline - Trace
Calcium Sulfate - Trace
*Major amorphous material |
|
|
Broadway &
Chambers |
WTC01-25 |
gypsum,
muscovite/illite,
CH,
chrysotile (tr)
Fe2+ (wk) |
Calcite - Minor
Quartz - Minor
Gypsum - Minor
Anhydrite - Minor
Dolomite - Trace
Illite - Trace
*Major amorphous material |
|
9.37 |
Church &
Warren |
WTC01-26 |
gypsum,
muscovite (tr),
CH,
Fe2+ (wk) |
Calcite - Minor
Gypsum - Minor
Anhydrite - Minor
Muscovite - Minor
Quartz - Trace
Dolomite - Trace
Microcline - Trace
Calcium Sulfate - Trace
Chrysotile - Trace
*Major amorphous material |
|
|
1/2 way between Broadway
and Church on Park |
WTC01-27 |
gypsum,
muscovite,
CH
|
Anhydrite - Minor
Calcite - Minor
Quartz - Minor
Gypsum - Minor
Orthoclase - Trace
Albite - Trace
Muscovite - Trace
Clinochrysotile - Trace
*Major amorphous material |
 |
10 |
Broadway &
Vesey |
WTC01-28 |
gypsum,
muscovite,
CH,
chrysotile (wk),
Fe2+ (wk) |
Calcite - Minor
Gypsum - Minor
Quartz - Minor
Anhydrite - Minor
Chrysotile - Trace
*Major amorphous material |
|
9.93 |
Ann &
Nassau |
WTC01-29 |
gypsum,
muscovite (tr),
CH,
chrysotile (tr),
Fe2+ (wk) |
Calcite - Minor
Gypsum - Minor
Quartz - Minor
Anhydrite - Minor
Muscovite - Trace
Chrysotile - Trace
*Major amorphous material |
|
|
William &
Fulton |
WTC01-30 |
gypsum,
muscovite,
CH,
Fe2+ |
Calcite - Minor
Quartz - Minor
Dolomite - Minor
Anhydrite - Minor
Gypsum - Minor
Chrysotile - Trace
*Possible trace chrysotile
*Major amorphous material |
|
|
Fulton &
Cliff St.
from on top of a car |
WTC01-31 |
gypsum,
muscovite,
CH,
Fe2+ (wk) |
Calcite - Minor
Gypsum - Minor
Quartz - Minor
Anhydrite - Minor
Muscovite - Trace
Chrysotile - Trace
*Major amorphous material |
|
|
William &
Platt |
WTC01-32 |
gypsum,
muscovite,
CH,
Fe2+ |
Calcite - Minor
Gypsum - Minor
Quartz - Minor
Anhydrite - Minor
Muscovite - Trace
Chrysotile - Trace
*Possible trace chrysotile
*Major amorphous material |
|
|
William &
Pine |
WTC01-33 |
gypsum,
muscovite (tr),
CH,
Fe2+ (wk) |
Gypsum - Minor
Calcite - Minor
Quartz - Minor
Muscovite - Minor
Anhydrite - Trace
Magnesiohornblende - Trace
Chrysotile - Trace
*Major amorphous material |
|
|
Battery Park,
middle |
WTC01-34 |
gypsum,
muscovite,
CH,
chrysotile (tr) Fe2+ (wk) |
Quartz - Major
Calcite - Minor
Gypsum - Minor
Anhydrite - Trace
*Major amorphous material |
|
9.8 |
South End &
Thames |
WTC01-35 |
gypsum,
CH,
muscovite,
chrysotile (tr)
Fe2+ |
Gypsum - Minor
Calcite - Minor
Quartz - Minor
Anhydrite - Trace
Muscovite - Trace
Chrysotile - Trace
*Major amorphous material |
|
;
|
Albany &
Hudson River |
WTC01-36 |
gypsum,
muscovite and/or portlandite (tr),
CH,
Fe2+,
possible trace chrysotile |
Gypsum - Minor
Calcite - Minor
Quartz - Minor
Anhydrite - Minor
Bassanite - Minor
Portlandite - Minor
Dolomite - Trace
Illite - Trace
Chrysotile - Trace
*possible trace chrysotile
*Major amorphous material |
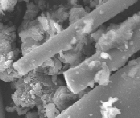 |
11.8 |
South End &
Albany
(30th floor):
Indoor sample |
WTC01-37A |
gypsum,
muscovite,
portlandite,
Fe2+ |
Quartz - Major
Albite - Major
Portlandite - Minor
Magnesiohornblende - Minor
Orthoclase - Trace
Muscovite - Trace |
|
|
concrete from WTC area |
WTC01-37B |
portlandite,
Fe2+ |
Quartz - Major
Orthoclase - Minor
Portlandite - Minor
Albite - Trace
Calcite - Trace
Magnesiohornblende - Trace
Muscovite |
|
|
concrete from WTC area |
* Amorphous material is not identifiable by XRD, but its presence is detectable.
* "Possible trace chrysotile" means at or near the detection limit with XRD.
The question of asbestos distribution was investigated and the results show
an asymmetric distribution pattern (Results Figure 1). More chrysotile was
detected in an east-west direction than south. This pattern occurs in both
the AVIRIS maps and from field samples (Results Figure 2). While there is a
general trend, it is not exclusive, meaning that chrysotile was detected in
all directions. It also should be noted that samples obtained next to each
other (on the map this means a city block apart) can show different results:
one has asbestos, another has no chrysotile above the detection limit.
Composition of samples on a centimeter scale was examined with a spectrometer.
Small variations in chrysotile content throughout a sample were observed. Thus
from scales of cm to tens of meters, chrysotile content varies. Such variability
makes sampling and overall assessment of a site difficult.
The fact that some materials in the WTC debris were observed to contain higher
levels of chrysotile (sample WTC01-08) on a steel beam, and that the coatings
on the beams have largely been stripped, leads to the question of where did
the coatings go and how well distributed/dispersed is the chrysotile? Because
a patch of coating showed up to 20% chrysotile, and the field samples and the
AVIRIS maps show varying levels of serpentine (chrysotile) leads to the possibility
that other patches of chrysotile may exist in the debris.
The asymmetry in the AVIRIS iron-bearing materials map may be related to the
asymmetry in the asbestiform minerals map. The AVIRIS data and the laboratory
analyses of the field samples indicate a lower abundance of chrysotile in the
the southern direction from the WTC, the same direction of the increase in
iron-bearing materials. The one field sample, WTC01-08, from an iron beam,
which had up to 20% chrysotile also contains a strong Fe2+ absorption. Thus one might expect a higher chrysotile content in iron-bearing
materials. However, this is clearly not the case, at least in general. This
may indicate other sources of the chrysotile besides the beam coatings.
AVIRIS imaging spectroscopy mapping provides a synoptic view that samples more
area than possible with other methods. The AVIRIS maps shown here represent
only a portion of the data collected, and effectively provide data for about
4.7 million sample locations, all obtained within a couple of hours. The sampling
includes land, air and water.
The fact that the field sampling missed the highest concentrations of serpentines
in the AVIRIS maps shows the limitations of limited sampling methodologies.
Ideally, the field sampling team would have the AVIRIS materials maps to guide
the field sampling. Unfortunately, this was not possible in this rapid response
case (but we routinely employ such methods in geologic studies where the region
does not change rapidly). Even so, the materials maps for this study were produced
faster than any other imaging spectroscopy effort to our knowledge. The AVIRIS
data were received within 24 hours of acquisition, and the data were initially
calibrated to help the field team obtain the final calibration data with real
time feedback via cell phone. In this case, scientists in Denver communicated
composition of field calibration sites using initially calibrated AVIRIS data
(of the parking lot structure) while the field team was investigating where
the best portion of the parking lot was located. The real-time feedback resulted in avoidance of portions of the parking lot with strong absorption
features, not visible to the human eye, that could have compromised the quality
of the final calibration.
With further development of on-board solar calibration targets on the aircraft
with the AVIRIS sensor, the refinement of analysis software, the development
of more reference spectral libraries, and the use of faster computers, an even
faster response is possible in the future. The challenge is formidable. To
analyze the data for this study, we used approximately 300 gigabytes of disk
space and performed over 50 trillion calculations. The results of the AVIRIS
mapping are limited by knowledge of the spectral properties of materials and
the detection levels are limited by the sensor signal-to-noise. The detection
limits could be substantially improved with existing technology in a new sensor
design.
The combination of field sampling with laboratory analysis and imaging spectroscopy
remote sensing provide a powerful assessment combination. We estimate the analysis
effort of this highly experienced team to be 1.8 person years to complete this
study plus another 0.6 person-year for the AVIRIS data collection effort. This
study includes analysis of 20% of the AVIRIS data from Sept 16, and 7% of the
data from Sept 23 (thermal hot spot analysis only).
The scientific data from this study is presented with no assessment of health
effects. It is beyond the scope of this study to assess health effects of a
fraction of a percent chrysotile asbestos, for example.
Other Conclusions have already been presented in the Executive Summary.