Colorado River Basin Actionable and Strategic Integrated Science and Technology Project—Science Strategy
Links
- Document: Report (10.9 MB pdf) , HTML , XML
- Download citation as: RIS | Dublin Core
Acknowledgments
Jessica Brown, Jessica Driscoll, and Mathew Miller reviewed early drafts of this document and provided valuable feedback to improve its clarity and completeness.
Abstract
The U.S. Geological Survey (USGS) conducts a wide variety of science that improves understanding of droughts and their effects on ecosystems and society. This work includes data collection and monitoring of aquatic and terrestrial systems; assessment and analysis of patterns, trends, drivers, and impacts of drought; development and application of predictive models; and delivery of information and decision-making tools to stakeholders. Stakeholders, which include Federal, Tribal, State, and local agencies, nongovernmental organizations, and others, use this information to anticipate, assess, react to, and mitigate drought conditions and impacts. There is no obvious near-term solution to reduce the frequency and severity of droughts or to mitigate drought impacts. Multidecadal drought is a “grand challenge” that benefits from integration of existing technologies, data, knowledge, and models across related and disparate disciplines, facilitated by new science and technology. In response, the USGS initiated a new integrated-science approach in the Colorado River Basin in 2020. The Colorado River Basin was specifically selected because of concerns about future drought and its consequences for the region. This document explains how the Colorado River Basin Actionable and Strategic Integrated Science and Technology (ASIST) project extends and enhances the science supported by USGS Mission Areas and Programs and articulates scientific gaps and stakeholder needs to identify and reduce drought risks. The approach seeks to answer complex Earth science questions developed in partnership with stakeholders about severe long-term drought. An integrated approach is required to tackle these complex questions, which any single science discipline cannot answer on its own.
In addition to increased understanding of drought and drought effects in complex systems, the Colorado River Basin ASIST project was designed to improve efficiencies through rapid location of a broad array of data sources, assembly of model-ready multidisciplinary data, and delivery of actionable science to stakeholders at the speeds and scales needed for decision making. The project team identified the following actions needed for USGS to implement and advance an integrated science approach in the Colorado River Basin: (1) engage with stakeholders to document their needs and iteratively co-produce science and science delivery tools to address these needs, (2) integrate monitoring and observation systems developed by USGS and other agencies that track droughts and their effects, (3) collect and provide analysis-ready data to support integrated applications, (4) integrate data and model connections to predict multiple drought impacts, (5) conduct multidisciplinary coordination to improve interpretations, (6) leverage the knowledge base across USGS to enhance decision making, and (7) support the development of new integrated science approaches and technologies that provide analysis and management tools that can be used to adapt to the effects of drought in the Colorado River Basin. Proposals for initial short-term use-case projects were solicited, a subset of which was selected for funding to test implementation of these actions. Additionally, the project organized and convened a series of science and technology collaboration workshops in the USGS focused on challenges that were identified and prioritized by the short-term use-case projects and during the initial stakeholder analysis. These workshops were designed to bring together diverse perspectives to discuss science and technology challenges, stakeholder needs, capabilities, and knowledge gaps, with the goal of determining how the USGS can address challenges, identify future opportunities for continued engagement between participants, and inform the next steps for the Colorado River Basin ASIST project. Continuing to collaboratively engage with a wide range of stakeholders using an integrated approach will provide a suitable foundation of data and tools to formulate actionable intelligence for predicting droughts and informing adaptation to the effects of long-term drought in a holistic, timely, and effective manner.
Introduction
Leading science organizations worldwide continually improve their science and deliver high-quality, actionable information to society. As organizations seek opportunities to add value and enhance the benefits of their science, interactions between humans and the environment continue to increase the complexity of societal grand challenges. In 2017, U.S. Geological Survey (USGS) scientists and leadership met at the USGS John Wesley Powell Center for Analysis and Synthesis in Fort Collins, Colorado, for a workshop focused on “grand challenges” for integrated USGS science (Jenni and others, 2017). This workshop sought to continue development of the USGS’ long history of addressing complex societal problems by advancing traditional Earth science disciplines and identifying opportunities to integrate USGS science across disciplines. The workshop defined “grand challenges” for integrated science as
“* * * a fundamental problem with broad societal consequences and solutions in Earth system science” (Jenni and others, 2017, p. 2).
The workshop participants further defined “grand challenges” as large and important issues and proposed addressing these challenges through
“* * * transformative integration of existing technologies, data, knowledge, and models across related and disparate disciplines and facilitated by new science and technology that will become available in the near future (1 to 10 years)” (Jenni and others, 2017, p. 2).
During the “grand challenges” workshop, participants also identified a comprehensive science challenge that highlighted the need for integrative science, data, models, and tools—all interacting in a modular Earth Monitoring, Analysis, and Predictions (EarthMAP) framework to address these and other future grand challenges. To implement this vision, the USGS is exploring an integrated science program through several pilot projects.
To demonstrate the vision from the “grand challenges” workshop, the USGS elected to focus on characteristics and risks of long-term drought (multiyear or longer) to natural resource security and society in the Colorado River Basin (fig. 1) through a project initiated in 2020. Drought affects more people than any other natural hazard and is one of the most complex but least understood (Wilhite, 2000). Drought impacts are complicated and multifaceted and are typically classified as economic, social, and environmental. Monitoring, analyzing, and predicting drought impacts is particularly challenging due to compounding factors, including climate change, wildfire, landscape change, and infrastructure risks. Furthermore, climate change projections indicate increased drought frequency, making drought a crucial issue not only in the Colorado River Basin but also across the Nation (Ostroff and others, 2017; Lukas and Payton, 2020). The purpose of this document is to define the scientific focus of the Colorado River Basin ASIST project by explaining how the project extends and enhances the science supported by USGS Mission Areas and Programs and by articulating scientific gaps and stakeholder needs to identify and reduce drought risks. An overview of the definitions and patterns of drought, drivers of drought, and the various impacts that droughts have on different Earth systems is provided in appendix 1.
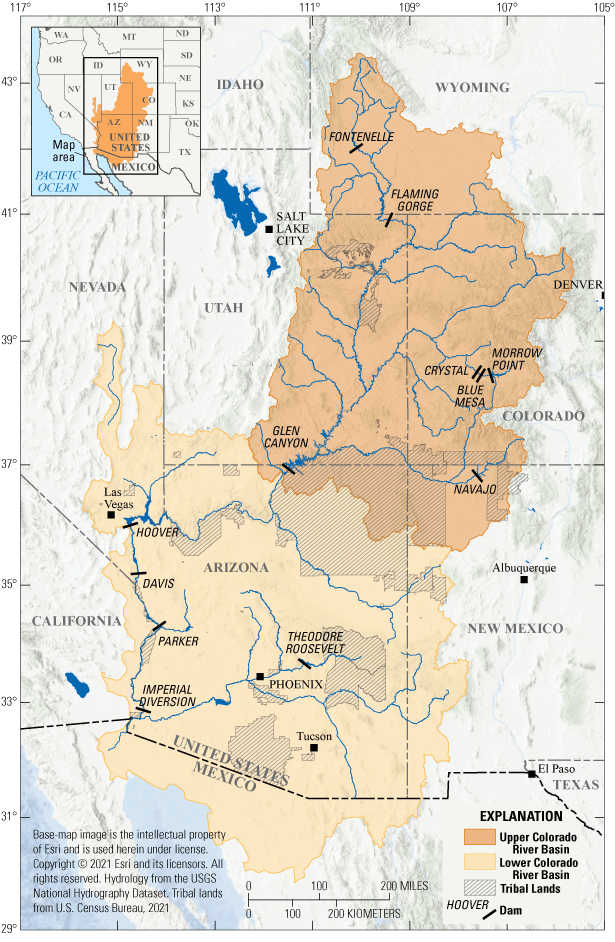
Location of Upper and Lower Colorado River Basin.
The Colorado River Basin ASIST Project Extends and Enhances USGS Science
The USGS is working with partners and stakeholders to understand the complexity of drought impacts and compounding factors, such as climate change and wildfire, on human and natural systems. A project team was established in 2020 to implement the EarthMAP approach described in the USGS 21st Century Science Strategy (U.S. Geological Survey 2021) through applications in the Colorado River Basin. This proof-of-concept project brings together the USGS’ interdisciplinary knowledge of the Colorado River Basin to better predict and understand the impacts of climate change and multidecadal drought on the natural and human systems in the Basin.
Organizational and scientific challenges will need to be addressed to achieve USGS integrated science. USGS Mission Areas and Programs sponsor research and collaborations with stakeholders to characterize the wide range of systems impacted by and influencing drought. Each Mission Area and Program has unique requirements, some congressionally mandated, as well as different funding streams that can be challenging to navigate. Thus, there remains a need for coordinated efforts that address organizational challenges while fostering collaboration and supporting integrated science across USGS Mission Areas (see the sidebar “USGS Mission Areas”), Programs, and Regions while also directly engaging stakeholders. The purpose of the Colorado River Basin ASIST project was to conduct a coordinated effort to address the critical issue of long-term regional drought due to climate change and reduce the associated risks to natural resources and society. Overarching strategies needed to accomplish this goal include assessing stakeholder science needs, integrating USGS science activities, and optimizing data management and information technology resources to efficiently combine data and models providing actionable information to stakeholders. The desired result of the pilot project is a better understanding of the cascading effects of long-term drought on the natural resources and ecosystem services provided by the Colorado River Basin, how these cascading effects impact communities that depend on those resources, and management strategies to reduce these impacts.
Box 112345. USGS Mission Areas
The USGS is organized into five Mission Areas, each with individual science strategy roadmaps and programs that support and organize drought-related research in coordination with other Federal agencies (Ostroff and others, 2017).
Core Science Systems Mission Area
The Core Science Systems Mission Area conducts national-scale science that develops and operates systems to acquire, produce, preserve, and deliver products and services to meet civil Earth observation requirements and research. This Mission Area also provides fundamental remote sensing science as well as data management services and produces foundational data layers including the national hydrography dataset and the National Land Cover Database. The Core Science Systems Mission Area builds and facilitates partnerships through the John Wesley Powell Center for Analysis and Synthesis and innovation through the Community for Data Integration and ScienceBase trusted digital repository. Specific to drought, the Core Science Systems Mission Area supports research examining how changes in climate and land use influence patterns of drought, how drought impacts ecologic, economic, hydrologic, and social systems, and how communities can adapt to reduce the risks of long-term drought.
Ecosystems Mission Area
The Ecosystems Mission Area conducts research to inform sustainable management and conservation of natural resources. The Ecosystems Mission Area conducts research to inform sustainable management and conservation of natural resources, with several programs that support the following topics: science related to the conservation, restoration, and management of priority species and ecosystems; effects of energy infrastructure on ecosystems; invasive species and fish and wildlife disease that threaten public safety, property, and ecosystems; and contaminants and pathogens impacting biological and public health. Additionally, the Ecosystems Mission Area supports basic research to understand and predict changes in areas critical to resource management and conservation—including climate, hydrology, the carbon cycle, land use, and land cover—and to distinguish between natural and anthropogenic sources of these changes.
Energy and Minerals Mission Area
The Energy and Minerals Mission Area conducts research and assessments on mineral and energy resources, including the economic and environmental effects of resource extraction and use. Science produced by this Mission Area contributes to understanding where energy and mineral resources are located and how changes in climate and drought conditions influence economic and natural systems and shifts in demand for energy and mineral resources.
Natural Hazards Mission Area
The Natural Hazards Mission Area coordinates with partners to monitor, assess, and research natural hazards, enabling policymakers and the public to prepare, respond, and recover from hazards. Relevant to drought, the Natural Hazards Mission Area supports assessments of a wide range of natural hazards so that policymakers and the public have the understanding they need to enhance preparedness, response, and resilience.
Water Resources Mission Area
The Water Resources Mission Area works with partners to monitor, assess, and conduct targeted research, and deliver information about a wide range of water resources and conditions including streamflow, groundwater, water quality, and water use and availability. Water information is fundamental to national and local economic well-being, protection of life and property, functioning of natural ecosystems, and effective management of the Nation’s water resources.
Colorado River Basin Stakeholders
Consistent and sustained engagement with stakeholders is central to USGS science. Achieving the project’s vision requires stakeholders to participate in identifying and coproducing science that supports policy development and decision making. The USGS has a long-standing tradition of providing stakeholders with credible, unbiased, and defensible science. Stakeholders rely on and trust the large body of knowledge collected by USGS because it undergoes rigorous quality assurance processes based on deep scientific experience and expertise. Throughout the Colorado River Basin, the USGS recognizes and interacts with many stakeholders who represent, protect, use, and manage natural resources. These stakeholders include: land and resource management agencies of Federal, State, Tribal, and local governments; nongovernmental organizations (NGOs) representing groups with specific concerns about the environment, recreation, or economic interests; industry partners in the Colorado River Basin who supply materials and services to the public; associations that help connect and inform agencies about natural resources; research institutions studying different landscape elements; and area residents who may be affected by management and project decisions. Each group of stakeholders in the Colorado River Basin has different interests and management priorities that underscore the need to understand and reduce risks arising from drought conditions.
The project team initiated a stakeholder needs assessment to determine what stakeholders had already written about their priorities and science needs (Frus and others, 2021). More than 200 recent publications and web pages were reviewed to determine stakeholder science needs. These stakeholder communications were used to characterize the stakeholders’ science needs by reviewing their priorities, strategies, issues, missions, and concerns about drought in the Colorado River Basin. Members of the project team identified each stakeholder’s science needs and categorized these needs based on science themes, science topics, and the actions necessary to address the need, including potential for additional monitoring, analysis, and prediction methods and identified alternative information delivery strategies that could be used to better characterize and communicate conditions in the Basin. Data analyses were completed to evaluate integrated science needs using visual and analytical methods such as word clouds and network analyses. These analyses helped to begin prioritizing stakeholder science needs by identifying common science themes and topics shared by different stakeholders.
The stakeholder needs assessment identified nearly 500 science needs. Each priority and science need listed an average of 6 unique science topics, and the stakeholder needs assessment identified 73 unique science topics in total. The average number of entries per science topic was 39; topics with 20 or more entries are shown in figure 2. Atmospheric and climatic processes, land-use change, streamflow, habitats, hazards, water use, water quality, and wildlife each appeared in more than 100 stakeholder priorities and needs. This high degree of overlap points to shared science needs by different stakeholders, making an integrated science research program that can bring to light interactions among processes and potential unknown linkages beneficial. Recognizing overlap among science needs helps to identify shared needs among stakeholders, enables USGS to focus outreach on groups of stakeholders with shared needs, and minimizes the potential that management decisions for one resource will have unintended consequences on another resource. The initial work on the stakeholder needs assessment will inform future engagements to produce integrated science in the Colorado River Basin.
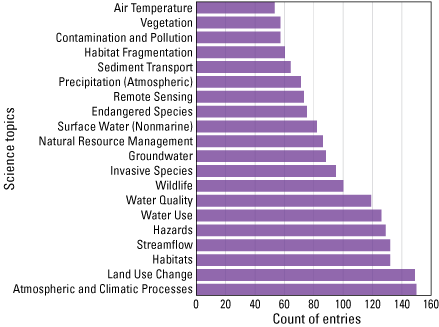
Histogram of the 20 most frequently listed science topics of drought-related needs from over 100 different stakeholder agencies and organizations associated with the Colorado River Basin.
USGS Drought-Science Capacity—Opportunities for Enhancement
Opportunities and approaches to enhance or improve management decisions can be identified by synthesizing stakeholder information needs and USGS science capacities. The stakeholder needs assessment indicated a strong need for integrated science to enhance and extend ongoing drought-related USGS science activities in the Colorado River Basin. Integrated science requires collaboration across traditional disciplines and capitalizes on the unique perspectives provided by each discipline to holistically assess and understand the complexity, connections, and dynamics of human and natural systems. Integrated science is vital for providing information to managers who are often faced with competing objectives and uncertain outcomes in their decision-making processes. The USGS has long recognized the need for integrated science (Myers and others, 2007; U.S. Geological Survey, 2007), and the relevance of integrated science will only increase as the complexity and scale of management challenges continue to increase.
Robust, defensible management decisions in the 21st century require high-quality, timely information about current conditions and circumstances under which decisions are being made, a thorough understanding of current conditions and predictions of potential outcomes, and documentation of assumptions with an explanation of the associated uncertainties. Shared science needs among the diverse group of Colorado River Basin stakeholders were evaluated to identify opportunities for USGS to provide relevant science considering USGS capacities across four broad themes: (1) data collection and monitoring, (2) analysis, (3) predictions, and (4) delivery of information and decision-making tools. For each theme, we broadly characterize relevant USGS capacity, describe how research related to the theme fits within an integrated science framework, and present a list of high-level science priorities (table 1).
Data Collection and Monitoring
There are many opportunities for science integration and collaboration within the USGS related to data collection and monitoring. Data collection and monitoring provide foundational information about drought conditions and impacts over a range of spatial and temporal extents using instrumentation, observational networks, or reconstructions. Data collection and monitoring activities include inventories or snapshots of information and repeated monitoring efforts to establish baseline conditions from which to detect and assess change and long-term trends. USGS activities designed to assess drought conditions and drought impacts are diverse and include but are not limited to: (1) collecting data from streamgages for tracking hydrologic impacts of drought, (2) collecting data from weather stations for tracking meteorological drought, and (3) remote sensing or in situ ecological studies that characterize drought-related ecosystem impacts. These efforts are organized at national to local scales.
Critical stakeholder science needs related to data collection and monitoring (table 1) include increasing the spatial and temporal resolution of monitoring efforts, directing monitoring to strategic locations and time periods, developing new monitoring tools, and integrating new and older information sources to ensure data continuity. Because of the strong linkages between drought occurrence and water availability, many monitoring needs are focused on hydrologic systems. Beyond hydrologic systems, monitoring needs also were identified for other components of the environment, including drought impacts on fire occurrence, and responses of habitats and individual species to drought. Many drought-monitoring and data-collection efforts operate under USGS Mission Areas and Programs, and this monitoring activity provides consistent, long-term baseline data that are foundational for other integrated science efforts. With that synergy in mind, there remains a need for coordinating monitoring efforts collaboratively to ensure optimal deployment of limited resources, seeking opportunities to collate smaller data collection projects into basin-wide resources, limiting redundancy or duplication of efforts, and sharing data with collaborators and stakeholders to facilitate science coproduction.
Analysis
Analysis is another area rich with opportunities for USGS integrated science. Analysis of drought-related data relies on information gathered by data collection and monitoring activities. USGS analysis activities include evaluating the accuracy and uncertainty of measurements, assessing spatial and temporal patterns or trends of droughts and their effects, and identifying drivers behind drought patterns and trends. Statistical and machine-learning models perform critical functions in analyses, including documenting or assessing patterns and trends, identifying drivers when controlling processes are not well understood, and informing detailed mechanistic models. Current USGS capabilities include analyses of several types of datasets, including historical tree rings, sediment cores, streamgage measurements, groundwater levels, atmospheric and soil moisture measurements, habitat assessments, forest inventories, and remote sensing data. Geospatial analyses can further improve our understanding of drought duration, extent, and severity. Analysis of model outputs can also evaluate drivers of droughts and their effects (for example, how drought severity, extent, and duration relate to water availability, fire occurrence, tree mortality, post-fire regeneration, habitat loss, invasive species distributions, and wildlife populations). Appendix 1 provides insight into the different USGS analyses focused on specific science topics.
Stakeholder needs for analyses that generate actionable drought science (table 1) include identifying important drivers of drought, vulnerabilities to drought, and the consequences of drought over space and time to resources in the Colorado River Basin. Such analyses require developing and refining our scientific understanding with new and updated combinations of data and models. Understanding the natural variations and human impacts of drought—including past frequencies, durations, severities, relations to climate change, and impacts on natural and man-made hydrologic systems—requires interpretation and analysis. Furthermore, analyses are needed to better understand the risks, impacts, and responses of aquatic and terrestrial ecosystems to drought.
Predictions
There are opportunities across science disciplines for science integration and collaboration within the USGS related to predicting drought impacts and effects across the landscape. Predictions are vital for anticipating and developing strategies to mitigate the impacts of potential shifts in drought patterns and trends, and integrative models can strengthen predictions. Such predictions provide estimates where observations are not available and include forecasts of short-term dynamics in well-understood, deterministic systems, such as expectations for tomorrow’s weather (Luo and others, 2011). In high-complexity systems that are poorly understood and not easily verified, prediction and forecasting are often not appropriate for anticipating how systems will evolve over long time periods (Bray and von Storch, 2009). Projections relying on scenarios of potential future conditions, such as the occurrence and distribution of wildfire under climate change and fuel-treatment scenarios, are useful in predicting high-complexity systems by showing a range of future possibilities for a range of assumptions (Peterson and others, 2003; Raskin, 2005; Gao and others, 2021 ). Projections do not include probabilities or likelihood of occurrence but often estimate uncertainties using a suite of scenarios with different assumptions. Representing uncertainties in both predictions and projections is essential for helping managers make decisions that are robust to scenario assumptions and range of potential outcomes (Mearns, 2010).
Predictions of future drought dynamics and resource impacts are vital for effective resource management because drought reduces water availability for aquatic and terrestrial ecosystems and affects land use and development patterns in the Colorado River Basin (table 1). Accurate predictions and long-term projections of drought effects in the Colorado River Basin require understanding both how drought conditions are likely to change in the future and how resources respond to drought conditions. The long-term balance between precipitation and potential evaporation (climatic aridity) does not translate directly into ecologically relevant measures of drought severity for many resources. Thus, predictions of drought impacts need to focus on measures of drought specific to different resources (for example, hydrological, agricultural, and ecological). In addition, because climate change is expected to alter drought regimes, projections need to represent a broad suite of future climate and development scenarios. As the occurrence and effects of drought vary over space and time and across systems, projections should consider different spatiotemporal scales and cascading effects for humans, flora, fauna, disturbances, and their interactions (such as invasive species and wildfire).
The USGS has many capabilities for prediction and long-term projections related to drought, including (1) long-term projections of hydrologic, agricultural, and ecological drought (see appendix 1) and land-use or land-cover change under scenarios of population growth, greenhouse gas emissions, and climate change scenarios; (2) population growth and development-driven demands for water resources; (3) scenarios of energy development and impacts on invasive species and wildlife populations; and (4) ecosystem and landscape simulation models to project how climate change and management measures interact to influence disturbances, species distribution, and ecosystem processes. Many of these efforts have already embraced an integrated-science approach. However, greater coordination of existing USGS modeling capabilities combined with identifying new opportunities for model integration and advanced technologies would create new opportunities for addressing stakeholder-identified integrated science needs related to risks of long-term drought in the Colorado River Basin.
Delivery of Information and Decision-Making Tools
Delivery of information and decision-making tools are needed to raise awareness and bring these integrated resources to stakeholders in formats that are accessible and useful for their objectives. These tools synthesize results from USGS efforts in data collection and monitoring, analyses, and predictions. Current USGS capabilities are served on numerous websites delivering information and data at a national scope, such as the ScienceBase Catalog, Earth Explorer, The National Map, and the National Water Information System. Additionally, the USGS also develops and manages interactive websites, dashboards, and online decision-making tools, to meet stakeholders' needs. For example, the StreamStats web application (https://streamstats.usgs.gov/ss/) produces reports on select scenarios for user-defined study areas, and USGS’ Cloud Hosting Solutions provides a suite of interactive dashboards that pull streamgage data from the National Water Information System. Finally, webinars, conference presentations, published reports, and journal articles are used extensively by USGS to deliver scientific findings to diverse audiences.
Stakeholder needs are diverse and rapidly evolving (table 1), and information and decision-support tools will need to be adaptive to accommodate those needs. The stakeholder needs analysis identified information and decision support tools, including: (1) data discovery and sharing tools such as data clearinghouses for tracking projects and spatially filtering information, (2) visualization tools to assess current and possible future drought conditions and impacts with uncertainties, and (3) decision support tools for drought risk and management planning. Specific examples of such tools include climate hubs and drought early-warning systems. To the extent possible, decision support tools should be spatially and temporally explicit and updated to reflect new capabilities and changing conditions in the Colorado River Basin to help stakeholders anticipate the occurrence, extent, severity, and consequences of drought for various ecological and socioeconomic systems. In addition, these tools should be responsive to stakeholder needs and readily updated to incorporate additional data and scientific or methodological advances.
Table 1.
Stakeholder needs related to data collection, monitoring, analysis, prediction, and delivery.Generate high-density in situ observations of critical variables—such as temperature, precipitation, snowpack, soil moisture, evaporation and evapotranspiration, groundwater discharge, stream temperature, and water quality potentially enabled by crowd-sourced data—over large spatial extents. |
Collect data to better represent spatial and temporal variability in temperature, precipitation, snowpack, evaporation and evapotranspiration, soil moisture, streamflow, groundwater discharge, stream temperature, water quality, ecosystem conditions, invasive species distribution, and land use change, making use of cutting-edge data interpolation and remote sensing data and techniques where possible. |
Collect remotely sensed information to assess how aquatic and terrestrial systems respond to and recover from drought, and track trends in vegetation condition and plant species composition. |
Develop consistent, spatially comprehensive, drought-related variables (precipitation, evapotranspiration, soil moisture, and fuel moisture) from in situ measurements that can be extrapolated across landscapes and downscaled from global circulation model projections. |
Monitor and characterize how individual species, communities, and ecosystems respond to drought, disturbances, and changing climate conditions and refugia, including processes of establishment, survival, dispersal, fecundity, productivity, disease, and mortality for individuals in aquatic and terrestrial wildlife populations, as well as vegetation communities. |
Compare and synthesize contemporary and paleo datasets and models for the Colorado River Basin, including subbasins and headwaters, to enable better understanding of past patterns, causes, and consequences of drought, stationarity of and interactions among drivers of drought, and place future droughts in a historical context. |
Improve understanding of snowpack dynamics and monsoon events on streamflow, soil moisture, and groundwater recharge, and better characterize microclimate effects on hydrology. |
Determine when and why discrepancies exist among different drought indicators. |
Strengthen dryland restoration efforts by identifying ecological resilience and thresholds of responses to drought duration and severity and determining both how to effectively control invasive species and how restoration, reintroduction, and assisted migration can mitigate drought impacts for wildlife and plant species. |
Quantify changes in disturbance regimes and ecosystem impacts driven by increasing drought frequency and severity. Assess implications of changes for vegetation composition and biomass (including invasive species), fuel moisture, fire risk, and post-disturbance recovery. |
Identify human and natural systems that are vulnerable to drought risks and determine past socioeconomic responses and management actions that effectively mitigated drought impacts while maintaining public safety and economic productivity. |
Identify how drought management contributes to contaminant exposures for humans, plants, and wildlife. Identifying populations with health risks will minimize mistakes and inefficiencies in drought management and protect the health of humans, wildlife, and livestock. |
Determine drought thresholds and conditions that impact natural resources, particularly mortality of widespread, dominant plant species. |
Generate near-term forecasts (weekly, monthly, and seasonal) and long-term drought projections (annual, decadal, multidecadal) and improve short-term and long-term drought forecasting by assessing the performance of weather and climate models, and the influence of downscaling and bias correction for estimating the specific drought conditions that impact natural resources. |
Estimate fuel moisture, fire behavior, and fire risk from downscaled weather and climate data, matching the resolution of drought and drought-index predictions. Predict areas susceptible to wildfires in response to future patterns of drought and potential for additional post-fire impacts such as debris flows, water quality changes, exotic species invasions, and vegetation transitions. |
Develop projections of future drought impacts on plant species distributions, invasive species, sensitive habitats, disease outbreaks, and wildlife populations. |
Identify candidate species and genotypes appropriate for seed germination and seedling increase under future climate. Identify and project seed transfer zones to facilitate restoration and assisted migration. Understand how seeding and restoration success will vary in the future across existing vegetation, soils, land use, disturbance history, and projected climate conditions. |
Refine long-term water-demand projections and assess water-supply vulnerability under future climate and management scenarios. Model the impacts of drought on water availability and quality, ecosystem services, and potential conflicts in relation to competing water demands. Use results to develop mitigation plans for future drought impacts on agricultural, hydrologic, and ecological systems. |
Establish robust data repositories with established semantics and quality-assurance protocols for broad categories such as ecological and biological data. The data repositories enable data retrieval by category and further refinement by predefined outputs. |
Develop tools that deliver synthesized information about past droughts, impacts, and future droughts for decision makers to enable informed long-term planning. |
Produce interactive visualizations that increase awareness of drought patterns and impacts for observed past and present conditions and future projected conditions. Incorporate multiple datasets and indices representing drought and impacts with data comparison functionality to identify differences and commonalities. |
Create effective multimedia to communicate drought risk and impacts through partnerships with stakeholders, decision makers, and the public (through stakeholder engagement and outreach), possibly by updating the Integrated Drought Science website (https://www.usgs.gov/special-topics/drought, accessed July 15, 2022). |
Create easy-to-use decision-support tools to inform short-term drought management, mitigation, and long-term planning. Represent uncertainties and impacts of uncertainty on outcomes. |
Build decision-support tools for seedling establishment, identifying when and where ecological drought conditions will be favorable to strengthen the effectiveness of limited restoration resources. |
Improve existing and develop new methods for early drought event detection and delivery of warning systems that alert managers and communities in the Colorado River Basin to impending drought impacts that directly influence the resources on which they depend. |
Integrated Science Approaches to Understand and Reduce Long-Term Drought Risks
As a recognized leader in drought-related research, the USGS conducts a wide variety of science that includes data collection and monitoring of aquatic and terrestrial systems; analysis of patterns, trends, drivers, and impacts of drought; development and application of predictive models related to drought; and delivery of information and decision-making tools to stakeholders to anticipate, assess, react to, and mitigate drought conditions and impacts. The USGS is pursuing a new integrated approach in the Colorado River Basin to answer complex Earth science questions identified in partnership with stakeholders that cannot be answered through a single discipline. The overall objective of an integrated drought-science approach in the Colorado River Basin is to increase understanding of the complexities of long-term drought in the Colorado River Basin and improve USGS efficiencies by reducing time to locate diverse data sources, translating multidisciplinary data to be ready for analysis and use in multiple types of models, and delivering science that stakeholders need for their decision making.
A well-developed knowledge base of multiple and varied stakeholder needs, shared across USGS Mission Areas and Regions, increases opportunities for planning integrated studies designed to answer multiple stakeholder questions simultaneously. Understanding stakeholder needs is an iterative process that requires continuous feedback among stakeholders and researchers. Thus, repeated analysis of stakeholder needs is important for understanding cross-disciplinary stakeholder science needs and how science needs may converge or diverge over time. Mapping links between stakeholders and science needs related to drought extends this process by informing potential interest from new and existing stakeholders. Continued stakeholder analysis can help to codevelop and prioritize work, identify and recruit additional partners, and coproduce data and analyses.
Continuous capacity assessment of the current USGS workforce expertise also is necessary to successfully produce monitoring, analysis, predictions, and delivery of information and decision-making tools to address stakeholder science needs. Evaluating USGS scientific and technical capacities and efficiently deploying them in response to specific information needs will enable the agency to identify and coordinate expertise to leverage the delivery of actionable information and also identify gaps in expertise and technology that must be addressed to accomplish science goals and objectives.
Developing a common taxonomy, syntax, and semantics to categorize expertise and science, including their spatial and temporal dimensions, is also necessary for successfully integrating USGS science. Having a common vocabulary improves our capacity for cross-disciplinary coordination and collaborations. Categorizing USGS research projects, data, analysis, and models into an accepted glossary will help support greater coordination and integration and create new opportunities for addressing stakeholder-identified integrated science needs related to risks of long-term drought in the Colorado River Basin.
To meet the current and future science needs in the Basin, the USGS will have to develop long-term decision support for integrated science. In addition, long-term support is required to foster collaboration and integration of USGS science related to science use cases. The USGS has a long history of delivering science and tools to help decision makers manage the effects of drought, but long-term support for integrated science is lacking. The following actions offer promise for an integrated science approach in the Colorado River Basin:
-
• Integration of USGS Drought Monitoring and Observation Systems: Evaluate opportunities to support colocation of sensors and monitoring systems across all USGS disciplines in the Basin, including correlating remote sensing products with on-the-ground monitoring.
-
• Investment in USGS Analysis-Ready Data to Support Integrated Applications: Collate independent data from focused studies in the Basin to treat small data as big data and increase understanding of each system’s complexity. Develop inventories and data standards by applying consistent vocabularies, syntax, and semantics to understand connections between studies and enable rapid data discovery.
-
• Establish Integrated Model Connections to Predict Drought Impacts: Work with interdisciplinary research groups in the Basin to establish connections, characterize integrated modeling frameworks, and map model networks to inputs and outputs across multiple disciplines.
-
• Multidisciplinary Coordination to Improve Interpretations: Coordinate across disciplines using communities of practice to better understand the complexity of scientific information and cross-disciplinary impacts and inform interpretations. This approach includes organizational support for USGS scientists to lead science forums for expanding knowledge of the state of the science.
-
• Utilize Whole Organization Knowledge Base for Decision Making: Communicate science to stakeholders for decision making by providing context for the entire USGS knowledge base across our diverse science portfolios. Actions to implement this approach include support from both internal and external communities of practice to make connections and prioritize science collaborations with organizations.
-
• Support Aspirational Efforts to Discover New Approaches: Take risks to explore new, innovative, integrated-science approaches for tackling big picture challenges and developing unique approaches to integrate drought science in the Colorado River Basin with sustained support for multiyear efforts.
Initiating, expanding, and continuing priority drought science is vital for implementing these actions. Proposals for initial short-term use-case projects were solicited, and a subset was selected for funding to test implementation of the integrated science priorities and test advanced data delivery applications (appendix 2). This portfolio of use-case projects identified integrated science connections among existing USGS projects and helped to identify science and technology gaps. Additionally, the project team organized and convened a series of science and technology collaboration workshops focusing on seven cross-cutting challenges and identified and developed examples of integrated science projects during the initial stakeholder analysis (appendix 3). The science and technology collaboration workshops gathered a diverse group of USGS science experts from multiple Science Centers to discuss the science and technology challenges, stakeholder needs, capabilities, knowledge gaps, and how the USGS can address challenges. The meetings were opportunities to foster internal working relationships that may develop use cases for applying integrated-science solutions to address the complex impacts of drought on the Colorado River Basin and identify future opportunities for continued engagement between participants and inform the next steps for this ASIST project.
Summary
The USGS has a diverse suite of drought-related expertise and ongoing research that is strengthened by partnerships with other agencies and stakeholders. This capacity is built across multiple disciplines to provide monitoring, analyses, and predictions to fulfill stakeholder science needs. The Colorado River Basin supports a variety of human communities and industries as well as natural resources, and as drought becomes more frequent and prolonged in the Basin, there is a pressing need to identify and integrate research and monitoring across disciplines and at relevant spatiotemporal scales. Stakeholder analyses identified several integrated drought science priorities, and the Colorado River Basin ASIST project proposed a series of actions designed to address these priorities. Pursuing these actions with the vision from the grand challenges workshop for integrated science will help the USGS and stakeholders to better address complex questions and data needs that arise from severe droughts. Using this collaborative approach with a wide range of stakeholders will create a suitable foundation of data and tools to provide actionable intelligence needed to predict droughts and adapt to the effects of long-term drought in a holistic, timely, and effective manner.
References Cited
Bray, D., and von Storch, H., 2009, “Prediction” or “Projection”?—The nomenclature of climate science: Science Communication, v. 30, no. 4, p. 534–543, accessed July 15, 2022, https://doi.org/10.1177/1075547009333698.
Frus, R., Hawbaker, T.J., Anderson, E., Anderson, P., Andrews, W., Bradford, J., Dean, D., Duniway, M., Jones, D., Monroe, A., Qi, S., Skinner, C., Thomas, K., Tillery, A., Torregrosa, A., and Dahm, K.H., 2021, A snapshot of stakeholder science needs related to drought in the Colorado River Basin: U.S. Geological Survey data release, accessed July 15, 2022, at https://doi.org/10.5066/P9CTXP26.
Gao, P., Terando, A.J., Kupfer, J.A., Morgan Varner, J., Stambaugh, M.C., Lei, T.L., and Kevin Hiers, J., 2021, Robust projections of future fire probability for the conterminous United States: Science of The Total Environment, v. 789, p. 147872, accessed July 15, 2022, at https://doi.org/10.1016/j.scitotenv.2021.147872.
Jenni, K.E., Goldhaber, M.B., Betancourt, J.L., Baron, J.S., Bristol, R.S., Cantrill, Mary, Exter, P.E., Focazio, M.J., Haines, J.W., Hay, L.E., Hsu, Leslie, Labson, V.F., Lafferty, K.D., Ludwig, K.A., Milly, P.C., Morelli, T.L., Morman, S.A., Nassar, N.T., Newman, T.R., Ostroff, A.C., Read, J.S., Reed, S.C., Shapiro, C.D., Smith, R.A., Sanford, W.E., Sohl, T.L., Stets, E.G., Terando, A.J., Tillitt, D.E., Tischler, M.A., Toccalino, P.L., Wald, D.J., Waldrop, M.P., Wein, A., Weltzin, J.F., and Zimmerman, C.E., 2017, Grand challenges for integrated USGS science—A workshop report: U.S. Geological Survey Open-File Report 2017–1076, 94 p., accessed March 5, 2021, at https://doi.org/10.3133/ofr20171076.
Lukas, J., and Payton, E., 2020, Colorado River Basin Climate and Hydrology, State of the Science: University of Colorado Boulder Western Water Assessment, 520 p., accessed March 5, 2021, at https://wwa.colorado.edu/publications/reports/CRBreport/.
Luo, Y., Ogle, K., Tucker, C., Fei, S., Gao, C., LaDeau, S., Clark, J.S., and Schimel, D.S., 2011, Ecological forecasting and data assimilation in a data-rich era: Ecological Applications, v. 21, no. 5, p. 1429–1442, accessed July 15, 2022, at https://doi.org/10.1890/09-1275.1.
Mearns, L.O., 2010, Quantification of Uncertainties of Future Climate Change—Challenges and Applications: Philosophy of Science, v. 77, no. 5, p. 998–1011, accessed July 15, 2022, at https://doi.org/10.1086/656817.
Myers, M.D., Ayers, M.A., Baron, J.S., Beauchemin, P.R., Gallagher, K.T., Goldhaber, M.B., Hutchinson, D.R., LaBaugh, J.W., Sayre, R.G., Schwarzbach, S.E., Schweig, E.S., Thormodsgard, J., van Riper, C., III, and Wilde, W., 2007, USGS Goals for the Coming Decade: Science, v. 318, no. 5848, p. 200–201, accessed July 15, 2022, at https://doi.org/10.1126/science.1147228.
Ostroff, A.C., Muhlfeld, C.C., Lambert, P.M., Booth, N.L., Carter, S.L., Stoker, J.M., and Focazio, M.J., 2017, USGS integrated drought science: U.S. Geological Survey Circular 1430, 32 p., accessed March 5, 2021, at https://doi.org/10.3133/cir1430.
Peterson, G.D., Cumming, G.S., and Carpenter, S.R., 2003, Scenario Planning—A Tool for Conservation in an Uncertain World: Conservation Biology, v. 17, no. 2, p. 358–366, accessed July 15, 2022, at https://doi.org/10.1046/j.1523-1739.2003.01491.x.
Raskin, P.D., 2005, Global Scenarios—Background Review for the Millennium Ecosystem Assessment: Ecosystems, v. 8, p. 133–142, accessed July 15, 2022, at https://doi.org/10.1007/s10021-004-0074-2.
U.S. Geological Survey, 2007, Facing tomorrow’s challenges—U.S. Geological Survey science in the decade 2007–2017: U.S. Geological Survey Circular 1309, 81 p., accessed June 7, 2021, at https://doi.org/10.3133/cir1309.
U.S. Geological Survey, 2021, U.S. Geological Survey 21st-Century Science Strategy 2020–2030: U.S. Geological Survey Circular 1476, 20 p., accessed September 30, 2022, at https://doi.org/10.3133/cir1476.
Appendix 1. Drought-Related Topics Relevant to Stakeholder Needs and Integrated Science Priorities
Drought Definitions
Drought is a general term that refers to an impactful lack of water, and there are specific definitions of drought that relate to distinctive forms of water deficit in different systems (Crausbay and others, 2017; Ostroff and others, 2017). Meteorological drought refers to periods of unusually low precipitation. These events can be exacerbated by unusually high temperatures, a phenomenon known as a global change drought (Breshears and others, 2005) that is expected to become increasingly common as temperatures rise in coming decades (Trenberth and others, 2014). Hydrological droughts are periods of unusually low flows in streams or rivers, whereas agricultural droughts are periods of low soil moisture that decrease the productivity of agricultural crops. Hydrologic drought science focuses specifically on short-term (days) to long-term (multiple years) negative anomalies in the quantity of surface (streamflow, lake, snowpack, and reservoir) and subsurface (soil and groundwater) water stores. What constitutes below-normal conditions will vary by geography, time period, and potential impact and must therefore be identified in an applications context. Ecological droughts impact ecological processes and include soil-moisture deficits (similar to agricultural droughts) that impact terrestrial ecosystem processes or hydrologic deficits that impact aquatic processes (Crausbay and others, 2017). The timing and causes of drought also are used to distinguish different types of droughts (Crausbay and others, 2020). For instance, a flash drought occurs when there is a rapid onset of drought conditions that persist for a short duration. Dry- and warm-snow drought differentiates drought conditions that are results of below-normal snow precipitation in contrast to warmer than normal temperatures that result in a lack of snow accumulation. Similar to warm-snow drought, hot drought occurs when higher temperatures increase evapotranspiration and shift drought conditions from moderate to severe (Udall and Overpeck, 2017). Differentiating the various types of droughts is critical for understanding the drivers behind drought and assessing the impacts of drought.
Drought Characteristics
Persistent and severe drought impacts human, ecological, and hydrological systems and creates challenges for water managers (Cook and others, 2015). Because of potential wide-ranging drought impacts, coordinated and integrated science designed to reduce risks of drought and increase resilience to drought has been a priority of USGS (Ostroff and others, 2017) and other Federal and State agencies (Lukas and Payton, 2020). Drought conditions are part of the natural variability in the Colorado River Basin, but there is evidence that droughts have increased in frequency, duration, and severity, and the drivers behind drought are not stationary. The southwestern United States, of which the Colorado River Basin is a major part, experienced severe droughts multiple times since 1900, including from 1900 to 1910, the 1920s, the late 1980s, the early 2000s (McCabe and others, 2020), and ongoing in 2021. Historically, droughts were primarily the result of below-normal precipitation, but in the late 1980s and early 2000s, droughts were caused by precipitation deficits combined with human-caused temperature increases that resulted in reduced snowpack and greater evapotranspiration (Mote and others, 2018; McCabe and others, 2020; Williams and others, 2020; McCabe and Wolock, 2021a). Additionally, the Southwest is particularly prone to multidecadal droughts, during which sustained drought conditions persist for at least 20 years (McCabe and Wolock, 2021b). There is some evidence that the unusually dry and warm conditions experienced in the Southwest during the 21st century may represent another emerging megadrought (Williams and others, 2020).
Drought duration, extent, and severity are expected to increase with projected temperature increases in the absence of climate change mitigation (Lukas and Payton, 2020). Multiple studies have found that the combination of increased temperature and enhanced weather variability will further exacerbate ongoing weather variability and drought severity (Seager and others, 2007; Trenberth and others, 2014; Prein and others, 2017; Lin and others, 2018; Ukkola and others, 2018; Bradford and others, 2020; Cook and others, 2020). Consequently, there is a need for coordinated and integrated science to anticipate and reduce risks of drought and increase resilience to drought while also considering future potential changes with and without historical analogs (Bradford and others, 2018). This anticipation can be long term (anticipating multidecadal climate change) or short term (using multimonth weather forecasts to plan management actions for maximum effectiveness). In addition to anticipating long-term changes, there are many opportunities for using forecasts of shorter-term ecological dynamics to improve resource management (Bradford and others, 2021).
Ecosystems, Ownership, and Land Use in the Colorado River Basin
The Colorado River Basin spans 640,000 square kilometers (km2), including parts of Arizona, California, Colorado, New Mexico, Nevada, Utah, and Wyoming (Bureau of Reclamation, 2012; fig. 1.1A). The Colorado River Basin is also partitioned into two regional hydrologic units: the Lower Basin and Upper Basin. Before European settlement, vegetation in the Colorado River Basin was primarily shrublands (59 percent), forest and woodlands (24 percent), grasslands (5 percent), riparian (5 percent) or sparsely vegetated areas (3 percent) (LANDFIRE Biophysical Settings v.2.0.0; Keane and others, 2006). The current distribution of vegetation, shown in figure 1.1B, is similar to pre-settlement levels but with less shrubland (7 percent less), grassland (1 percent less), and riparian area (4 percent less), and more forest and woodland (3 percent more) and sparsely vegetated areas (5 percent more) (LANDFIRE Existing Vegetation Type v 2.0.0; Picotte and others, 2019). Additional contemporary land cover types present in the Colorado River Basin include agriculture (3 percent of Colorado River Basin area), developed areas (2 percent), exotic trees and shrubs (2 percent), exotic herbaceous (1 percent), and quarries, mines, gravel pits, well and wind pads (0.1 percent). The majority of land (55 percent) in the Colorado River Basin is under Federal jurisdiction managed by the following agencies: Bureau of Land Management (31 percent), U.S. Department of Agriculture Forest Service (18 percent), National Park Service (3 percent), and other agencies (3 percent) (fig. 1.1C; Bureau of Land Management, 2021). Seventeen percent of the Colorado River Basin area encompasses lands of 29 federally recognized Native American tribes (U.S. Geological Survey Gap Analysis Project, 2020). The remaining land in the Colorado River Basin is under State and local jurisdiction (9 percent) or is privately owned (19 percent; Bureau of Land Management, 2021).
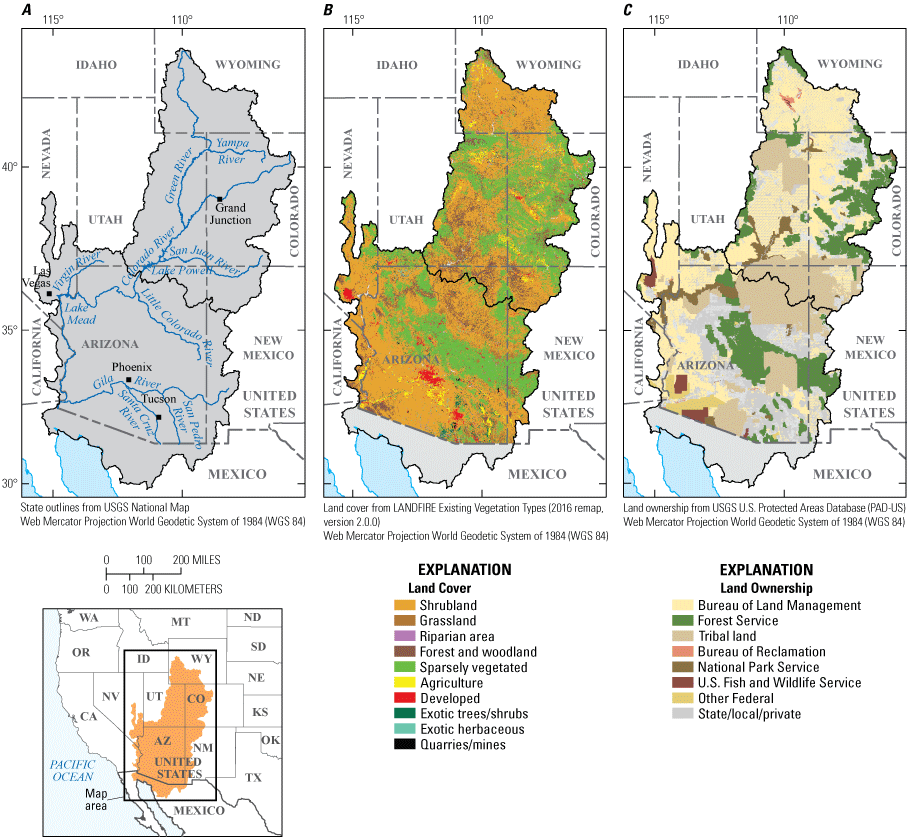
Three-panel map showing: A, the extent of the Upper and Lower Colorado River Basin, main tributary rivers; B, existing vegetation; and C, land ownership.
Present-day land use in the Colorado River Basin is diverse and varies across ownership and land-cover types. The total population in the Colorado River Basin in 2010 was 9.4 million people residing in 4.2 million housing units (Radeloff and others, 2018). That population is spatially concentrated in urban and developed areas (2 percent of Colorado River Basin area), especially in cities in the Lower Basin, including Las Vegas, Nev., Phoenix, Ariz., and Tucson, Ariz. The wildland-urban interface (WUI) is extensive, and large proportions of the population (45 percent) and homes (48 percent) are in the WUI even though the areal extent of the WUI covers only 2 percent of the Colorado River Basin (Radeloff and others, 2018). Land used for agricultural production in the Colorado River Basin (16,600 km2) is primarily irrigated forage crops and pasture (Cohen and others, 2013). Sales from commodities for States overlapping the Colorado River Basin total $39.5 billion per year from crops (20 percent of United States total) and $23.6 billion per year from livestock (12 percent of United States total; U.S. Department of Agriculture, 2019). Timber harvest is limited, and harvest and thinning operations were completed on 854 km2 of Forest Service lands from 2010 through 2019 (U.S. Department of Agriculture, 2021). Less timber harvest occurs on Bureau of Land Management districts overlapping the Colorado River Basin, with 62 km2 cut from 2010 through 2019 (T. Bottomley, Bureau of Land Management, written commun., 2021). Public lands in the Colorado River Basin support a wide range of economic activities. Grazing and timber activities on Department of the Interior (DOI) lands in the Colorado River Basin provided 25,200 jobs and $1.3 billion in economic output in 2019 (U.S. Department of the Interior, 2021). Energy development and mining are also important economic contributors on DOI lands in States overlapping the Colorado River Basin, supporting 220,000 jobs and $63 billion in economic output in 2019 (U.S. Department of the Interior, 2021). Additionally, recreational activities on DOI lands supported 153,000 jobs and $17 billion in economic output in 2019 in the States overlapping the Colorado River Basin (U.S. Department of the Interior, 2021), providing more jobs on DOI lands than any other activity considered in Arizona, California, and Utah.
Climate and Hydrology of the Colorado River Basin
The Colorado River begins at La Poudre Pass in Rocky Mountain National Park in Colorado and flows 2,334 kilometers (km) to its terminus in the Gulf of California. Tributaries to the Colorado River include the Green (Colo. and Utah), Yampa (Colo.), San Juan (Colo., Utah, and N. Mex.), Virgin (Nev. and Utah), and Little Colorado, Gila, Salt, San Pedro, and Santa Cruz Rivers (Ariz.). The Colorado River streamflow is heavily regulated through reservoir operations, and the Basin has large reservoir capacity (four times the annual flow, or 74 billion cubic meters). Much of the water in the Basin is stored in Lakes Powell and Mead, the largest reservoirs in the country, which comprise 85 percent of the Basin’s storage capacity (Christensen and others, 2004).
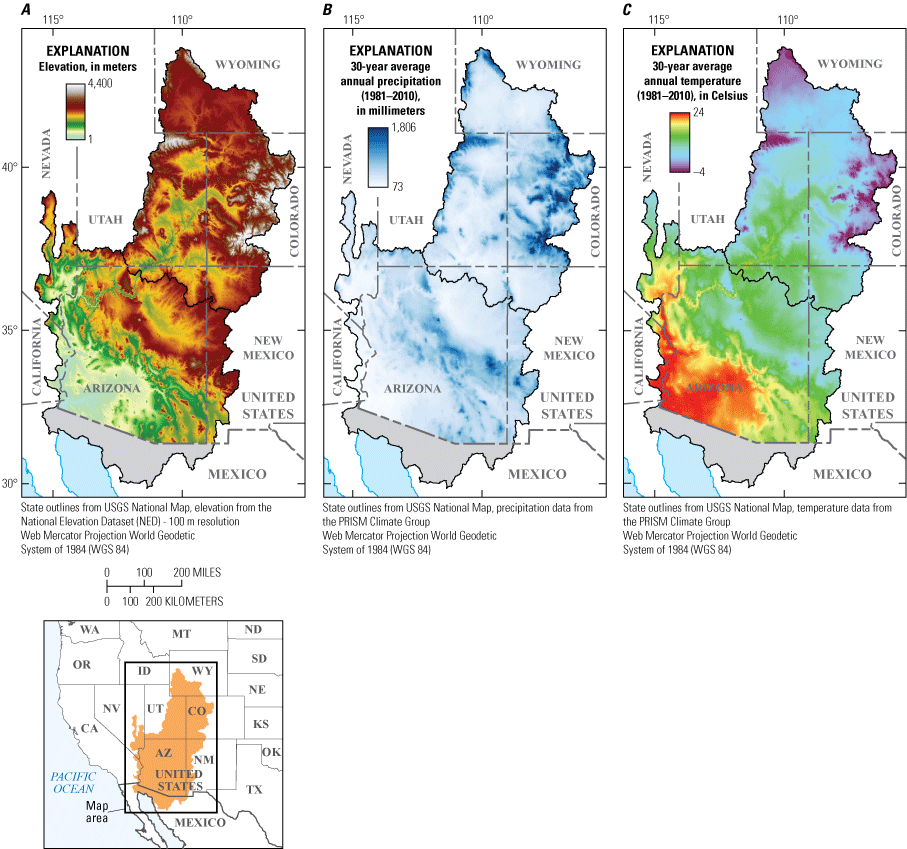
Three-panel map showing: A, elevation; B, mean annual precipitation; and C, mean annual temperature in the Colorado River Basin.
Topographic gradients and spatial and temporal variability in climate interact to influence the movement of water in the Colorado River Basin (fig. 1.2). Mean elevation across the Basin is 1,700 meters (m), ranging from sea level to 4,365 m, which creates abrupt climate gradients (fig. 1.2A). Across the Basin, precipitation is lowest in June. Spatial variability in precipitation is large and affected by topographic gradients, ranging from 187.2 centimeters per year (cm/yr) in mountainous areas and the headwaters to 7.6 cm/year in southwestern Arizona and southeastern California (800-m native grid resolution, from 1981–2010 averages) (fig. 1.2B; PRISM Climate Group, 2021). Mean annual precipitation across the Colorado River Basin is 36.0 cm, equating to 230 billion cubic meters per year of total precipitation. The majority of the Basin has an arid or semiarid climate, and 87 percent of the precipitation returns to the atmosphere through evaporation, sublimation, or evapotranspiration (Christensen and Lettenmaier, 2007). Most precipitation at higher elevations is in the form of snow. Less precipitation is returned to the atmosphere at high elevations than at lower elevations because of reduced evaporation, sublimation, and evapotranspiration (Lukas and Payton, 2020). In the Upper Colorado River Basin, winter snow accumulation and spring snowmelt from 15 percent of the Basin area contributes 85 percent of the Colorado River’s annual flow (Christensen and Lettenmaier, 2007; Li and others, 2017). In the Lower Colorado River Basin, intense and infrequent thunderstorms during the monsoon season (July and August) provide as much as 50 percent of annual rainfall for southern parts of Arizona and New Mexico (Jana and others, 2018). However, precipitation in the Lower Basin contributes just 8 percent to annual streamflow (Christensen and Lettenmaier, 2007), whereas about 90 percent of the flow in the Lower Basin is from Upper Basin flow released from the Glen Canyon Dam into Lake Mead (Ostroff and others, 2017; Lukas and Payton, 2020; McCabe and others, 2020; Tillman and others, 2020). Stable isotope analysis of surface water entering Lake Powell indicated that little evaporation occurs in the upper Colorado River but increases moving downstream, particularly in Lake Powell and Lake Mead (Guay and others, 2006). Spatially, mean annual temperature is lower at higher elevations and in the northern part of the Colorado River Basin (–5.5 degrees Celsius [°C]) and higher at lower elevations (24.6 °C); overall, the Lower Basin tends to be 7–10 °C warmer than the Upper Basin (800-m native grid resolution, from the 1981–2010 averages) (fig. 1.2C; PRISM Climate Group, 2021). Temporal variability in temperature follows a sinusoidal pattern, being lowest in January and highest in July.
Groundwater discharge to the Colorado River (baseflow) is a mix of recently recharged waters and older, deeply sourced regional water (Crossey and others, 2015). Groundwater discharge to streams is estimated to comprise 21 to 58 percent of the flow in the Upper Basin, whereas baseflow yield is lower in areas with higher average temperatures and potential evapotranspiration, such as the Lower Basin (Miller and others, 2014; Rumsey and others, 2015). Forty percent of the annual streamflow discharging to the Lower Basin at Glen Canyon Dam is estimated to be groundwater, and nearly all perennial tributary streams in the lower elevations of the Colorado River Basin are dependent on discharge from groundwater (Miller and others, 2016). What is less understood is the loss of surface waters to groundwater recharge. This “losing stream” scenario may contribute to the quantified loss of surface water availability and is a knowledge gap that could be important to understanding water resources of the Colorado River Basin.
In addition to groundwater discharging as baseflow to the Colorado River, more than 20,000 springs have been identified in the Colorado River Basin. Springs and associated wetlands in the Colorado River Basin are considered hotspots of biodiversity and act as refuges to aquatic and terrestrial flora and fauna (Stevens and Meretsky, 2008). Groundwater discharge measured at different spring sites is generally small and variable, ranging from ephemeral to perennial (Stevens and others, 2020). Groundwater flow paths are controlled by topography and subsurface geology, which can influence spring water quantity, quality, and discharge locations (Hubbs, 1995). However, substantial uncertainty remains about estimates of groundwater discharge to surface water because of variable connectivity with surface waters and the potentially long residence time of regional groundwater resources (Rumsey and others, 2015). Reduced groundwater recharge because of climate change affects spring discharge rates, including reduced discharge and dry spring sites (Dettinger and others, 2015).
Water Quality
The quality of groundwater is influenced by water-rock interactions, groundwater residence time, and groundwater mixing (Drever, 1982; Hem, 1985). Many of the geologic units in the Upper Basin are sedimentary rocks deposited in marine and lacustrine environments, which contribute to dissolved solids (salinity) concentrations and loads through erosion, dissolution, and transport through surface and groundwaters (Rumsey and others, 2017). Snowmelt and other winter precipitation are relatively dilute and are the major sources for groundwater recharge in the Colorado River Basin (Eastoe and others, 2004; Plummer and others, 2004; Earman and others, 2006; Eastoe and Towne, 2018). Groundwater discharging to streams can increase the salinity of surface water, and the effects of groundwater on dissolved solids concentration are related to the volume of water and the dissolved solids concentrations in the groundwater fraction of streamflow (Earman and Dettinger, 2011). In upper elevations of the Colorado River Basin, such as the Uinta Mountains, Wind River Range, and Colorado Rocky Mountains, groundwater has lower dissolved solids concentrations due to limited water-rock interactions, which contributes to lower dissolved solids concentrations in overall streamflow. In the mid and lower elevations, such as the mountain front and semiarid intermontane basins, groundwater has more water-rock interactions and can be a larger proportion of the overall streamflow, thereby increasing the dissolved solids concentrations in the streambed (Rumsey and others, 2017). The salinity of the Colorado River has increased, mainly due to agricultural runoff (Robison and others, 2014), and between 32 percent and 45 percent of dissolved solids loading in the Upper Basin originate from irrigated agricultural lands (Anning and others, 2007). Natural brine discharge, such as that sourced from the extensive groundwater brine system at Paradox Valley (Ball and others, 2020), also contributes to salt loads in the Colorado River (Watts, 2000; Mast, 2017).
Changes in precipitation, temperature, and conjunctive use that accompany drought can affect water quality through several mechanisms (Driscoll and Sherson, 2016). Reduced groundwater recharge due to drought and a warming climate can lead to increases in salinity, warmer water temperatures, and impaired water quality (Phillips and others, 2004; Earman and Dettinger, 2011). With diminished recharge of dilute waters, the salinity of discharging groundwaters at mid and low elevations will likely increase due to the loss of the more dilute groundwater (Earman and Dettinger, 2011). Less recharge also increases residence times due to loss of head pressure, which increases water-rock interaction and can lead to higher dissolved solids in groundwater (Hem, 1985). Water temperatures are likely to increase as there will be less groundwater discharging into the streambed (Earman and Dettinger, 2011; Green and others, 2011). Finally, drought can influence the concentration of contaminants in the subsurface with longer residence times and possible changes in flow paths (Green and others, 2011).
Although groundwater recharge can strongly affect water quality in the smaller rivers in the Colorado River Basin, streamflow and temperature in most of the larger rivers are controlled by releases from upstream dams and weather (for example, air temperature or solar radiation). Because water temperature controls primary production, fish growth rates, and reproduction, the management of dams in the Colorado River Basin has a substantial effect on aquatic communities. The temperature of water released from Colorado River Basin reservoirs is largely dependent upon reservoir level. When full, the water in most large reservoirs becomes stratified, and cold water is released from the lower portion of the reservoir, or hypolimnion. However, when reservoirs are relatively empty, water releases are warmer because water is sourced from the upper portion of the reservoir, or epilimnion (Dibble and others, 2021). Thus, river thermal regimes in the Colorado River Basin are substantially related to the condition and management of the large reservoirs in the Basin.
Water Use
A diverse range of stakeholders both within and outside the Colorado River Basin depend on its rivers, reservoirs, and aquifers for water supplies (Maupin and others, 2018). For example, water from the Colorado River Basin supports 40 million people across seven States and Mexico, generates more than 4,200 megawatts from hydropower, and irrigates 16,000 km2 of agriculture (Bureau of Reclamation, 2012; Evenson and others, 2018). Consequently, understanding the processes controlling water availability is of great interest, as water is a limiting factor for ecosystems, land use, and many socioeconomic activities in the Colorado River Basin (Evenson and others, 2018; Maupin and others, 2018). Maupin and others (2018) presented an accounting of water use and trends in the Colorado River Basin and documented the greatest water needs from agricultural, municipal, and industrial sectors. Agricultural withdrawals for irrigation accounted for the largest portion of water use (84 percent of total withdrawals excluding hydropower and interbasin transfers), followed by public and domestic supply (13 percent), then industrial and mining, and thermoelectric (3 percent). Agricultural systems are heavily dependent on irrigation, and water availability affects hay and, ultimately, livestock production (Cohen and others, 2013; Akbar and others, 2020). Grazing is widespread, and although direct water use by livestock constitutes a small proportion of total water demand in the Colorado River Basin (less than 1 percent; Maupin and others, 2018), this sector can rely heavily on irrigated croplands and pastures in addition to public lands (Cohen and others, 2013). Irrigation withdrawals were primarily from surface water (98 percent in Upper Basin and 61 percent in Lower Basin) and larger in the Upper Basin (57 percent of all withdrawals in the Colorado River Basin). Public supply withdrawals were greatest in the Lower Basin (91 percent) and were also primarily from surface water (74 percent in Upper Basin and 60 percent in Lower Basin).
The water supplied by the Colorado River Basin is currently over-allocated, and shortfalls are expected to increase in the future (Christensen and others, 2004; Barnett and Pierce, 2009). Furthermore, the quantity of groundwater stored in aquifers is decreasing as withdrawals exceed recharge (Konikow, 2013; Castle and others, 2014). Risks for stakeholders dependent on the hydrologic resources of the Colorado River Basin may be exacerbated by hydrologic drought as water availability will likely decline with increasing aridity (Copeland and others, 2017) and a warming climate (Treidel and others, 2012). With increased drought frequency and intensity, groundwater resources are relied on more heavily for public and agricultural use (Castle and others, 2014). Agricultural drought may increase costs for irrigation, but water use can also be decoupled from availability due to water rights access (Huckleberry and Potts, 2019). In addition to reducing the amount of available water for domestic, commercial, industrial, and agricultural water users and aquatic ecosystems, reduced reservoir levels and flow could decrease hydroelectric energy production and increase power costs, affecting the regional and national economies (Bain and Acker, 2018; Huckleberry and Potts, 2019).
Aquatic and Riparian Ecosystems
Hydrologic drought can have a profound impact on aquatic ecosystems through its effects on water availability, the amount and timing of streamflow, and water temperature. In headwater areas, spring-fed ecosystems serve as hotspots for biodiversity, hosting endemic and endangered species, including pupfish and spring snails (Stevens and others, 2020). Springs, seeps, and resulting wetlands exert substantial ecological influence over disproportionately large geographic areas (Perla and Stevens, 2008). Groundwater extraction and reductions in groundwater recharge associated with drought can threaten these spring habitats (Riggs and Deacon, 2004).
In spring-fed streams the effects of drought may be mitigated if the springs flow from deeply sourced waters, possibly providing a refugia in a changing climate (Cartwright and others, 2020). However, shallow-sourced spring-fed streams are more susceptible to effects of drought, such as drying and warming. Drying will have a direct impact on the quantity and quality of available spring habitat, and warming will adversely affect aquatic organisms that rely on cool water temperatures, thus potentially favoring warm-water organisms that may be in low abundance during nondrought times. The drying of springs may also negatively affect the riparian buffer around these features. Thus, drought is a major threat to groundwater-dependent ecosystems. The effects of drought on groundwater levels and spring discharge, however, can be diffuse, and quantifying trends in such ecosystems may take years (Kløve and others, 2014). This may impede resource managers from recognizing the full effects of droughts until the problems become untenable (Green and others, 2011).
In the larger regional rivers of the Colorado River Basin, the effects of drought may be severe, greatly affecting both the riparian and aquatic ecosystems. The regional rivers in the Colorado River Basin have streamflow regimes determined by both spring snowmelt and releases from upstream dams. Drought affects the amount of snow that falls at high elevations and the timing of melt. Additionally, water releases from upstream dams are primarily determined by reservoir volume, such that during dry years water releases may be substantially smaller and shorter than during years when reservoirs are full. Over recent decades, drought has resulted in heavily reduced streamflow volumes and peak flow magnitudes for many of the rivers within the Colorado River Basin (Manners and others, 2014; Topping and others, 2018; Dean and others, 2020; Walker and others, 2020).
Streamflow magnitude and intermittency control the quantity and quality of riparian and aquatic habitats, reductions in which can result in channel narrowing. Channel narrowing typically occurs during successive years of below-average peak flow, allowing riparian vegetation to establish on bare substrates within the channel, resulting in the conversion of active channel substrates to floodplains (Dean and others, 2020; Grams and others, 2020; Walker and others, 2020). Channel narrowing typically results in the loss of aquatic habitats such as side channels and backwaters, which are essential for the rearing and growth of juvenile fish. In gravel-bedded reaches of these rivers, peak streamflow also determines bed mobility. Clean gravel substrate is needed for the successful spawning of some native fish and for a healthy macroinvertebrate community. In extreme cases, reduction in streamflow can also result in declines in groundwater levels, which in turn causes dieoffs of important riparian forests.
Drought also has a substantial effect on river temperature, which affects the growth and reproduction of native and endemic fish, many of which are endangered. Higher water temperatures also can affect the growth and reproduction of nonnative fish that predate upon the native species. Thus, during drought conditions, endangered fish in the Colorado River Basin may be negatively affected by the loss of aquatic habitat and increased predation by nonnative fish, potentially limiting their survival and recovery.
Terrestrial Ecosystems
Among terrestrial resources in the Colorado River Basin, drought impacts on vegetation are primarily driven by soil moisture deficiencies and groundwater levels, potentially in combination with high temperatures. These terrestrial ecosystems are primarily water-limited and include arid and semiarid grasslands, shrublands, and dry forests, in which access to moisture in the soil determines the structure and function of terrestrial plant communities and the ecosystem services they provide (Reynolds and others, 2007; Bestelmeyer and others, 2015). Ecological droughts in uplands of the Colorado River Basin can be complex and may take various forms (Feng and others, 2018), including long-term chronic moisture deficits (Hoover and others, 2017), short-term extreme moisture deficiencies (especially impactful in combination with high-temperature conditions; Williams and others, 2013; Adams and others, 2017; Breshears and others, 2018; Zhou and others, 2019), and moisture deficiencies in particular seasons or soil layers (Sala and others, 1997). Patterns of drought and moisture availability in these drylands shape plant communities by affecting photosynthesis, biomass production, growth, plant mortality, and regeneration (Gremer and others, 2015; Schlaepfer and others, 2015; Witwicki and others, 2016; Shriver and others, 2018; Renne and others, 2019; Thoma and others, 2019). Prolonged drought stress exposes these ecosystems to potentially rapid species turnover and altered community structure by reducing plant growth and regeneration. Likewise, severe droughts or heat waves may catalyze rapid ecological change in drylands by killing adult plants. Ultimately, droughts and other disturbances may combine in coming decades to promote large-scale transformations in dryland vegetation in the Colorado River Basin (Breshears and others, 2005; Martínez-Vilalta and Lloret, 2016). Widespread plant mortality and regeneration failures have been observed in many dryland regions and are expected to continue (Allen and others, 2010; Williams and others, 2013; Stevens-Rumann and others, 2018). Drought has been found to be a secondary driver in increased dustiness (second to natural or human-induced geomorphic alterations; Arcusa and others, 2020) as the loss of vegetation increases the pool of material available for aeolian transport. More dust may lead to more dust-on-snow events, leading to reductions in runoff and further exacerbating terrestrial impacts and vegetation die off (Painter and others, 2010).
Drought also can alter disturbance regimes and increase the frequency, extent, and severity of wildfires and insect outbreaks (Westerling and others, 2006; Littell and others, 2016), exacerbating and compounding widespread impacts of drought on vegetation mortality, regeneration, and growth (Coop and others, 2020). In areas burned by wildfires and affected by insect mortality, the cascading impacts include loss of human life and property (Caggiano and others, 2016; Kramer and others, 2018), reduced ecosystem carbon storage and increased smoke emissions (Caldwell and others, 2013; Urbanski and others, 2018), altered flow paths, diminished water supply and quality (Clow and others, 2011; Bearup and others, 2014; Murphy and others, 2018), potential for increased sedimentation and debris flows (Gartner and others, 2014; Staley and others, 2018), and substantial changes in ecosystem services (Rocca and others, 2014). However, impacts often extend beyond the area where disturbances occur. Wildfire smoke can spread over extensive distances, impacting air quality and visibility (Goodrick and others, 2012). Smoke also increases risk of respiratory and cardiovascular diseases, especially for children, the elderly, and those with chronic respiratory conditions (Liu and others, 2015; Urbanski and others, 2018; Holm and others, 2021). Furthermore, similar to dust deposition, ash deposition on snowfields reduces albedo and leads to increased snowmelt rates (Warren, 1984).
Drought impacts on vegetation are not limited to uplands but can extend into riparian areas as well. Reductions in streamflow can lead to the spread of the invasive shrub Tamarix spp. (Glenn and others, 2017), which in turn alters the composition of avian communities (Hinojosa-Huerta and others, 2013). Alterations of streamflows also can affect cottonwood forests and other native floodplain vegetation (Andersen and others, 2007), inhibit establishment of Acer negundo (box elder) (DeWine and Cooper, 2007), and hamper restoration of native vegetation (Glenn and others, 2017; Shafroth and others, 2017).
Threatened and Endangered Species
Although drought affects most species in the Colorado River Basin to varying degrees, responses of threatened and endangered species to drought are of interest for management implications and recovery efforts (McNeeley and others, 2016; Dibble and others, 2021). Altering the amount and timing of reservoir releases in response to drought can affect thermal regimes and benefit nonnative fish species at the expense of endangered native species, including Gila elegans (bonytail), Ptychocheilus lucius (Colorado pikeminnow), Gila cypha (humpback chub), and Xyrauchen texanus (razorback sucker) (Dibble and others, 2021). Conversely, increasing reservoir levels can reduce Empidonax traillii extimus (southwestern willow flycatcher) reproductive success (Ellis and others, 2009). Drought also can affect species in terrestrial systems. Mustela nigripes (black-footed ferret) depends on Cynomys spp. (prairie dogs) for food and habitat, and drought may negatively impact prairie dog demography (Facka and others, 2010; Davidson and others, 2018; Stephens and others, 2018) and increase prairie dog flea loads (Eads and others, 2016). Sage-grouse may be affected directly by drought (Blomberg and others, 2014) and loss of sagebrush, which sage-grouse rely on for food and nesting cover, is expected in some areas with a changing climate (Homer and others, 2015). Drought also may affect Strix occidentalis lucida (Mexican spotted owl) demography by limiting food resources (Seamans and others, 2002; Peery and others, 2012). Climate change could alter whooping crane wintering habitat (Chavez-Ramirez and Wehtje, 2012), and whereas precipitation can contribute to whooping crane recruitment, it is likely less important than environmental factors occurring on breeding grounds (Butler and others, 2014, 2017). Drought is also detrimental to amphibians, including Ambystoma mavortium stebbinsi (Sonoran tiger salamander) (Hossack and others, 2017) and Lithobates chiricahuensis (Chiricahua leopard frog) (Howell and others, 2020). Finally, drought may impact threatened or endangered plant species, such as Echinocactus horizonthalonius (Turk’s head cactus) (McIntosh and others, 2020) and the Purshia X subintegra (Kearney) (Arizona cliffrose) (Maschinski and others, 2006).
Mineral, Energy, and Geothermal Resources
Mining has a long history in the Colorado River Basin, and water is one of the most vital resources to the industry for its role in damping dust, crushing ore, and transporting tailings. The demand for minerals, especially those required to manufacture batteries for electric vehicles, chips for computing and network technologies, and specialized medical devices will continue to increase. International sources are often the leading suppliers of these critical minerals; however, efforts to shift to domestic sources are increasing. In conjunction with the expansion of critical mineral sourcing is attention to understanding and mapping the entire supply chain with its associated environmental, social, and political impacts. The Earth Mapping Resources Initiative (Earth MRI), developed by the USGS, is helping address the potential vulnerabilities in the Nation’s supply of critical mineral resources (Day, 2020). The effort is using a mineral systems approach based on the current understanding of how ore deposits form and how they relate to the broader geologic frameworks and tectonic history of the Earth (Hofstra and Kreiner, 2020). Several focal areas for assessment have been identified in the Colorado River Basin (Hammarstrom and others, 2020). The potential critical minerals formed throughout Earth’s history in diverse geologic settings, including magmatic, magmatic-hydrothermal, epicontinental marine basin, and surficial systems. Magmatic-hydrothermal processes have formed important porphyry copper systems along with critical minerals in the Colorado River Basin (Leveille and Stegen, 2012). Millions of years of groundwater flow through the sedimentary rocks of the Paradox basin led to the formation of important copper and uranium-vanadium deposits (Thorson and MacIntyre, 2005; Hitzman and others, 2010; Barton and others, 2018). Favorable geologic and climatic conditions have produced lithium brines in the Salton Sea strike-slip basin in California (Bradley and others, 2013). Ore-deposit formation can lead to changes in rock properties and understanding these changes from a watershed approach can be used to assess and rank historical mining-affected sites for possible cleanup (Church and others, 2007, and references therein). However, drought can have significant impacts on mining activities, including disruption of supply lines which can halt mine operations (Jorgensen, 2016). Such closures can have profound economic impacts, and risk-assessment techniques that consider drought duration, severity, and frequency are developed to help characterize the drought-induced financial risk in the mining industry (Bonnafous and others, 2017).
Energy resources also are reliant on water and substantial energy resource production has occurred primarily on the Upper Basin. Such production has come from conventional hydrocarbon resources, coal bed methane, and tight oil and gas plays in several basins, including the Greater Green River, Uinta, Park, Piceance, Paradox, and San Juan basins (U.S. Energy Information Administration, 2016). Water from geologic formations producing oil and gas is produced as a byproduct. Understanding the compositions of these produced waters is important in understanding the regional hydrogeology, the source of the water, the efficacy of water treatment and disposal plans, potential economic benefits of mineral commodities in the fluids, and safety of potential sources of drinking or agricultural water. Therefore, attributes of these produced waters are recorded in a periodically updated produced-waters geochemical database (Blondes and others, 2019). Geothermal energy production also is reliant on water in at least two ways: (1) water from an underground reservoir is used to power the facility and (2) water is used to cool the plant. A recent assessment of moderate- and high-temperature geothermal resources indicates that geothermal resources are favorable in the Colorado River Basin, particularly in the Salton Sea, southwestern Utah, and northwestern Arizona (Williams and others, 2008). Finally, reduced reservoir levels and flow could decrease hydroelectric energy production and increase power costs, affecting regional and national economies (Bain and Acker, 2018; Huckleberry and Potts, 2019).
References Cited
Adams, H.D., Barron-Gafford, G.A., Minor, R.L., Gardea, A.A., Bentley, L.P., Law, D.J., Breshears, D.D., McDowell, N.G., and Huxman, T.E., 2017, Temperature response surfaces for mortality risk of tree species with future drought: Environmental Research Letters, v. 12, no. 11, p. 115014, accessed July 15, 2022, at https://doi.org/10.1088/1748-9326/aa93be.
Akbar, H., Allen, L.N., Rosenberg, D.E., and Chikamoto, Y., 2020, Ranchers Adapting to Climate Variability in the Upper Colorado River Basin, Utah: Climate, v. 8, no. 9, article 96, 23 p., accessed July 15, 2022, at https://doi.org/10.3390/cli8090096.
Allen, C.D., Macalady, A.K., Chenchouni, H., Bachelet, D., McDowell, N., Vennetier, M., Kitzberger, T., Rigling, A., Breshears, D.D., Hogg, E.H., Gonzalez, P., Fensham, R., Zhang, Z., Castro, J., Demidova, N., Lim, J.-H., Allard, G., Running, S.W., Semerci, A., and Cobb, N., 2010, A global overview of drought and heat-induced tree mortality reveals emerging climate change risks for forests: Forest Ecology and Management, v. 259, no. 4, p. 660–684, accessed July 15, 2022, at https://doi.org/10.1016/j.foreco.2009.09.001.
Andersen, D.C., Cooper, D.J., and Northcott, K., 2007, Dams, Floodplain Land Use, and Riparian Forest Conservation in the Semiarid Upper Colorado River Basin, USA: Environmental Management, v. 40, no. 3, p. 453–475, accessed July 15, 2022, at https://doi.org/10.1007/s00267-006-0294-7.
Anning, D.W., Bauch, N.J., Gerner, S.J., Flynn, M.E., Hamlin, S.N., Moore, S.J., Schaefer, D.H., Anderholm, S.K., and Spangler, L.E., 2007, Dissolved solids in basin-fill aquifers and streams in the southwestern United States: U.S. Geological Survey Scientific Investigations Report 2006–5315, 187 p., accessed June 7, 2021, at https://doi.org/10.3133/sir20065315.
Arcusa, S.H., McKay, N.P., Routson, C.C., and Munoz, S.E., 2020, Dust-drought interactions over the last 15,000 years—A network of lake sediment records from the San Juan Mountains, Colorado: The Holocene, v. 30, no. 4, p. 559–574, accessed July 15, 2022, at https://doi.org/10.1177/0959683619875192.
Bain, D.M., and Acker, T.L., 2018, Hydropower Impacts on Electrical System Production Costs in the Southwest United States: Energies, v. 11, no. 2, p. 368, accessed July 15, 2022, at https://doi.org/10.3390/en11020368.
Ball, L.B., Bedrosian, P.A., and Minsley, B.J., 2020, High-resolution mapping of the freshwater–brine interface using deterministic and Bayesian inversion of airborne electromagnetic data at Paradox Valley, USA: Hydrogeology Journal, v. 28, no. 3, p. 941–954, accessed July 15, 2022, at https://doi.org/10.1007/s10040-019-02102-z.
Barnett, T.P., and Pierce, D.W., 2009, Sustainable water deliveries from the Colorado River in a changing climate: Proceedings of the National Academy of Sciences of the United States of America, v. 106, no. 18, p. 7334–7338, accessed July 15, 2022, at https://doi.org/10.1073/pnas.0812762106.
Barton, M.D., Barton, I., and Thorson, J., 2018, Paleofluid Flow in the Paradox Basin—Introduction: Guidebook Series, v. 59, 12 p., accessed July 15, 2022, at https://par.nsf.gov/biblio/10113160-paleofluid-flow-paradox-basin-introduction.
Bearup, L.A., Maxwell, R.M., Clow, D.W., and McCray, J.E., 2014, Hydrological effects of forest transpiration loss in bark beetle-impacted watersheds: Nature Climate Change, v. 4, no. 6, p. 481–486, accessed July 15, 2022, at https://doi.org/10.1038/nclimate2198.
Bestelmeyer, B.T., Okin, G.S., Duniway, M.C., Archer, S.R., Sayre, N.F., Williamson, J.C., and Herrick, J.E., 2015, Desertification, land use, and the transformation of global drylands: Frontiers in Ecology and the Environment, v. 13, no. 1, p. 28–36, accessed July 15, 2022, at https://doi.org/10.1890/140162.
Blomberg, E.J., Sedinger, J.S., Gibson, D., Coates, P.S., and Casazza, M.L., 2014, Carryover effects and climatic conditions influence the postfledging survival of greater sage-grouse: Ecology and Evolution, v. 4, no. 23, p. 4488–4499, accessed July 15, 2022, at https://doi.org/10.1002/ece3.1139.
Blondes, M.S., Gans, K.D., Engle, M.A., Kharaka, Y.K., Reidy, M.E., Saraswathula, V., Thordsen, J.J., Rowan, E.L., and Morrissey, E.A., 2019, U.S. Geological Survey National Produced Waters Geochemical Database (ver. 2.3, January 2018): U.S. Geological Survey data release, accessed July 15, 2022, at https://doi.org/10.5066/F7J964W8.
Bonnafous, L., Lall, U., and Siegel, J., 2017, An index for drought induced financial risk in the mining industry: Water Resources Research, v. 53, no. 2, p. 1509–1524, accessed July 15, 2022, at https://doi.org/10.1002/2016WR019866.
Bradford, J.B., Andrews, C.M., Robles, M.D., McCauley, L.A., Woolley, T.J., and Marshall, R.M., 2021, Landscape-scale restoration minimizes tree growth vulnerability to 21st century drought in a dry forest: Ecological Applications, v. 31, no. 2, article e2238, accessed July 15, 2022, at https://doi.org/10.1002/eap.2238.
Bradford, J.B., Betancourt, J.L., Butterfield, B.J., Munson, S.M., and Wood, T.E., 2018, Anticipatory natural resource science and management for a changing future: Frontiers in Ecology and the Environment, v. 16, no. 5, p. 295–303, accessed July 15, 2022, at https://doi.org/10.1002/fee.1806.
Bradford, J.B., Schlaepfer, D.R., Lauenroth, W.K., and Palmquist, K.A., 2020, Robust ecological drought projections for drylands in the 21st century: Global Change Biology, v. 26, no. 7, p. 3906–3919, accessed July 15, 2022, at https://doi.org/10.1111/gcb.15075.
Bradley, D., Munk, L., Jochens, H., Hynek, S., and Labay, K.A., 2013, A preliminary deposit model for lithium brines: U.S. Geological Survey Open-File Report 2013–1006, 9 p., accessed June 7, 2021, at https://doi.org/10.3133/ofr20131006.
Breshears, D.D., Carroll, C.J.W., Redmond, M.D., Wion, A.P., Allen, C.D., Cobb, N.S., Meneses, N., Field, J.P., Wilson, L.A., Law, D.J., McCabe, L.M., and Newell-Bauer, O., 2018, A Dirty Dozen Ways to Die—Metrics and Modifiers of Mortality Driven by Drought and Warming for a Tree Species: Frontiers in Forests and Global Change, v. 1, no. 4, 10 p., accessed July 15, 2022, at https://doi.org/10.3389/ffgc.2018.00004.
Breshears, D.D., Cobb, N.S., Rich, P.M., Price, K.P., Allen, C.D., Balice, R.G., Romme, W.H., Kastens, J.H., Floyd, M.L., Belnap, J., Anderson, J.J., Myers, O.B., and Meyer, C.W., 2005, Regional vegetation die-off in response to global-change-type drought: Proceedings of the National Academy of Sciences of the United States of America, v. 102, no. 42, p. 15144–15148, accessed July 15, 2022, at https://doi.org/10.1073/pnas.0505734102.
Bureau of Land Management, 2021, BLM National Surface Management Agency Area Polygons - National Geospatial Data Asset (NGDA): Bureau of Land Management Landscape Approach Data Portal website, accessed April 16, 2021, at https://landscape.blm.gov/geoportal/catalog/search/resource/details.page?uuid=%7B2A8B8906-7711-4AF7-9510-C6C7FD991177%7D.
Bureau of Reclamation, 2012, Colorado River Basin Water Supply and Demand Study: Bureau of Reclamation website, accessed June 7, 2021, at https://www.usbr.gov/lc/region/programs/crbstudy.html.
Butler, M.J., Metzger, K.L., and Harris, G., 2014, Whooping crane demographic responses to winter drought focus conservation strategies: Biological Conservation, v. 179, p. 72–85, accessed July 15, 2022, at https://doi.org/10.1016/j.biocon.2014.08.021.
Butler, M.J., Metzger, K.L., and Harris, G.M., 2017, Are whooping cranes destined for extinction? Climate change imperils recruitment and population growth: Ecology and Evolution, v. 7, no. 8, p. 2821–2834, accessed July 15, 2022, at https://doi.org/10.1002/ece3.2892.
Caggiano, M.D., Tinkham, W.T., Hoffman, C., Cheng, A.S., and Hawbaker, T.J., 2016, High resolution mapping of development in the wildland-urban interface using object based image extraction: Heliyon, v. 2, no. 10, article e00174, accessed July 15, 2022, at https://doi.org/10.1016/j.heliyon.2016.e00174.
Caldwell, M.K., Hawbaker, T.J., Briggs, J.S., Cigan, P.W., and Stitt, S., 2013, Simulated impacts of mountain pine beetle and wildfire disturbances on forest vegetation composition and carbon stocks in the Southern Rocky Mountains: Biogeosciences, v. 10, no. 12, p. 8203–8222, accessed July 15, 2022, at https://doi.org/10.5194/bg-10-8203-2013.
Cartwright, J.M., Dwire, K.A., Freed, Z., Hammer, S.J., McLaughlin, B., Misztal, L.W., Schenk, E.R., Spence, J.R., Springer, A.E., and Stevens, L.E., 2020, Oases of the future? Springs as potential hydrologic refugia in drying climates: Frontiers in Ecology and the Environment, v. 18, no. 5, p. 245–253, accessed July 15, 2022, at https://doi.org/10.1002/fee.2191.
Castle, S.L., Thomas, B.F., Reager, J.T., Rodell, M., Swenson, S.C., and Famiglietti, J.S., 2014, Groundwater depletion during drought threatens future water security of the Colorado River Basin: Geophysical Research Letters, v. 41, no. 16, p. 5904–5911, accessed July 15, 2022, at https://doi.org/10.1002/2014GL061055.
Chavez-Ramirez, F., and Wehtje, W., 2012, Potential Impact of Climate Change Scenarios on Whooping Crane Life History: Wetlands, v. 32, no. 1, p. 11–20, accessed July 15, 2022, at https://doi.org/10.1007/s13157-011-0250-z.
Christensen, N.S., and Lettenmaier, D.P., 2007, A multimodel ensemble approach to assessment of climate change impacts on the hydrology and water resources of the Colorado River Basin: Hydrology and Earth System Sciences, v. 11, no. 4, p. 1417–1434, accessed July 15, 2022, at https://doi.org/10.5194/hess-11-1417-2007.
Christensen, N.S., Wood, A.W., Voisin, N., Lettenmaier, D.P., and Palmer, R.N., 2004, The Effects of Climate Change on the Hydrology and Water Resources of the Colorado River Basin: Climatic Change, v. 62, no. 1, p. 337–363, accessed July 15, 2022, at https://doi.org/10.1023/B:CLIM.0000013684.13621.1f.
Church, S.E., von Guerard, P., and Finger, S.E., 2007, Integrated Investigations of Environmental Effects of Historical Mining in the Animas River Watershed, San Juan County, Colorado: U.S. Geological Survey Professional Paper 1651, 1096 p., accessed June 7, 2021, at https://doi.org/10.3133/pp1651.
Clow, D.W., Rhoades, C., Briggs, J., Caldwell, M., and Lewis, W.M., Jr., 2011, Responses of soil and water chemistry to mountain pine beetle induced tree mortality in Grand County, Colorado, USA: Applied Geochemistry, v. 26, p. S174–S178, accessed July 15, 2022, at https://doi.org/10.1016/j.apgeochem.2011.03.096.
Cohen, M., Christian-Smith, J., and Berggren, J., 2013, Water to Supply the Land: Irrigated Agriculture in the Colorado River Basin: Pacific Institute Blog, May 2013, accessed June 7, 2021, at https://pacinst.org/publication/water-to-supply-the-land-irrigated-agriculture-in-the-colorado-river-basin/.
Cook, B.I., Ault, T.R., and Smerdon, J.E., 2015, Unprecedented 21st century drought risk in the American Southwest and Central Plains: Science Advances, v. 1, no. 1, article e1400082, accessed July 15, 2022, at https://doi.org/10.1126/sciadv.1400082.
Cook, B.I., Mankin, J.S., Marvel, K., Williams, A.P., Smerdon, J.E., and Anchukaitis, K.J., 2020, Twenty-First Century Drought Projections in the CMIP6 Forcing Scenarios: Earth’s Future, v. 8, no. 6, article e2019EF001461, accessed July 15, 2022, at https://doi.org/10.1029/2019EF001461.
Coop, J.D., Parks, S.A., Stevens-Rumann, C.S., Crausbay, S.D., Higuera, P.E., Hurteau, M.D., Tepley, A., Whitman, E., Assal, T., Collins, B.M., Davis, K.T., Dobrowski, S., Falk, D.A., Fornwalt, P.J., Fulé, P.Z., Harvey, B.J., Kane, V.R., Littlefield, C.E., Margolis, E.Q., North, M., Parisien, M.-A., Prichard, S., and Rodman, K.C., 2020, Wildfire-Driven Forest Conversion in Western North American Landscapes: Bioscience, v. 70, no. 8, p. 659–673, accessed July 15, 2022, at https://doi.org/10.1093/biosci/biaa061.
Copeland, S.M., Bradford, J.B., Duniway, M.C., and Schuster, R.M., 2017, Potential impacts of overlapping land-use and climate in a sensitive dryland—A case study of the Colorado Plateau, USA: Ecosphere, v. 8, no. 5, article e01823, accessed July 15, 2022, at https://doi.org/10.1002/ecs2.1823.
Crausbay, S.D., Betancourt, J., Bradford, J., Cartwright, J., Dennison, W.C., Dunham, J., Enquist, C.A.F., Frazier, A.G., Hall, K.R., Littell, J.S., Luce, C.H., Palmer, R., Ramirez, A.R., Rangwala, I., Thompson, L., Walsh, B.M., and Carter, S., 2020, Unfamiliar Territory—Emerging Themes for Ecological Drought Research and Management: One Earth, v. 3, no. 3, p. 337–353, accessed July 15, 2022, at https://doi.org/10.1016/j.oneear.2020.08.019.
Crausbay, S.D., Ramirez, A.R., Carter, S.L., Cross, M.S., Hall, K.R., Bathke, D.J., Betancourt, J.L., Colt, S., Cravens, A.E., Dalton, M.S., Dunham, J.B., Hay, L.E., Hayes, M.J., McEvoy, J., McNutt, C.A., Moritz, M.A., Nislow, K.H., Raheem, N., and Sanford, T., 2017, Defining Ecological Drought for the Twenty-First Century: Bulletin of the American Meteorological Society, v. 98, no. 12, p. 2543–2550, accessed July 15, 2022, at https://doi.org/10.1175/BAMS-D-16-0292.1.
Crossey, L.C., Karlstrom, K.E., Dorsey, R., Pearce, J., Wan, E., Beard, L.S., Asmerom, Y., Polyak, V., Crow, R.S., Cohen, A., Bright, J., and Pecha, M.E., 2015, Importance of groundwater in propagating downward integration of the 6–5 Ma Colorado River system—Geochemistry of springs, travertines, and lacustrine carbonates of the Grand Canyon region over the past 12 Ma: Geosphere, v. 11, no. 3, p. 660–682, accessed July 15, 2022, at https://doi.org/10.1130/GES01073.1.
Davidson, A.D., Hunter, E.A., Erz, J., Lightfoot, D.C., McCarthy, A.M., Mueller, J.K., and Shoemaker, K.T., 2018, Reintroducing a keystone burrowing rodent to restore an arid North American grassland—Challenges and successes: Restoration Ecology, v. 26, no. 5, p. 909–920, accessed July 15, 2022, at https://doi.org/10.1111/rec.12671.
Day, W.C., 2020, Technical overview of the U.S. Geological Survey Earth Mapping Resources Initiative (Earth MRI): U.S. Geological Survey Fact Sheet 2020–3055, 4 p., accessed June 7, 2021, at https://doi.org/10.3133/fs20203055.
Dean, D.J., Topping, D.J., Grams, P.E., Walker, A.E., and Schmidt, J.C., 2020, Does Channel Narrowing by Floodplain Growth Necessarily Indicate Sediment Surplus? Lessons from Sediment Transport Analyses in the Green and Colorado Rivers, Canyonlands, Utah: Journal of Geophysical Research—Earth Surface, v. 125, no. 11, article e2019JF005414, accessed July 15, 2022, at https://doi.org/10.1029/2019JF005414.
Dettinger, M., Udall, B., and Georgakakos, A., 2015, Western water and climate change: Ecological Applications, v. 25, no. 8, p. 2069–2093, accessed July 15, 2022, at https://doi.org/10.1890/15-0938.1.
DeWine, J.M., and Cooper, D.J., 2007, Effects of river regulation on riparian box elder (Acer Negundo) forests in Canyons of the upper Colorado River Basin, USA: Wetlands, v. 27, no. 2, p. 278–289, accessed July 15, 2022, at https://doi.org/10.1672/0277-5212(2007)27[278:EORROR]2.0.CO;2.
Dibble, K.L., Yackulic, C.B., Kennedy, T.A., Bestgen, K.R., and Schmidt, J.C., 2021, Water storage decisions will determine the distribution and persistence of imperiled river fishes: Ecological Applications, v. 31, no. 2, article e02279, accessed July 15, 2022, at https://doi.org/10.1002/eap.2279.
Driscoll, J.M., and Sherson, L.R., 2016, Variability of surface-water quantity and quality and shallow groundwater levels and quality within the Rio Grande Project Area, New Mexico and Texas, 2009–13: U.S. Geological Survey Scientific Investigations Report 2016-5006, 33 p., accessed July 15, 2022, at https://doi.org/10.3133/sir20165006.
Eads, D.A., Biggins, D.E., Long, D.H., Gage, K.L., and Antolin, M.F., 2016, Droughts may increase susceptibility of prairie dogs to fleas—Incongruity with hypothesized mechanisms of plague cycles in rodents: Journal of Mammalogy, v. 97, no. 4, p. 1044–1053, accessed July 15, 2022, at https://doi.org/10.1093/jmammal/gyw035.
Earman, S., Campbell, A.R., Phillips, F.M., and Newman, B.D., 2006, Isotopic exchange between snow and atmospheric water vapor—Estimation of the snowmelt component of groundwater recharge in the southwestern United States: Journal of Geophysical Research, v. 111, no. D9, article D09302, accessed July 15, 2022, at https://doi.org/10.1029/2005JD006470.
Earman, S., and Dettinger, M., 2011, Potential impacts of climate change on groundwater resources – a global review: Journal of Water and Climate Change, v. 2, no. 4, p. 213–229, accessed July 15, 2022, at https://doi.org/10.2166/wcc.2011.034.
Eastoe, C.J., Gu, A., and Long, A., 2004, The Origins, Ages and Flow Paths of Groundwater in Tucson Basin—Results of a Study of Multiple Isotope Systems, in Hogan, J.F., Phillips, F.M., Scanlon, B.R., eds., Groundwater Recharge in a Desert Environment—The Southwestern United States, Volume 9: Washington, D.C., American Geophysical Union, p. 217–234, accessed July 15, 2022, at https://doi.org/10.1029/009WSA12.
Eastoe, C., and Towne, D., 2018, Regional zonation of groundwater recharge mechanisms in alluvial basins of Arizona—Interpretation of isotope mapping: Journal of Geochemical Exploration, v. 194, p. 134–145, accessed July 15, 2022, at https://doi.org/10.1016/j.gexplo.2018.07.013.
Ellis, L.A., Stump, S.D., and Weddle, D.M., 2009, Southwestern Willow Flycatcher Population and Habitat Response to Reservoir Inundation: The Journal of Wildlife Management, v. 73, no. 6, p. 946–954, accessed July 15, 2022, at https://doi.org/10.2193/2008-297.
Evenson, E.J., Jones, S.A., Barber, N.L., Barlow, P.M., Blodgett, D.L., Bruce, B.W., Douglas-Mankin, K., Farmer, W.H., Fischer, J.M., Hughes, W.B., Kennen, J., Kiang, J.E., Maupin, M.A., Reeves, H.W., Senay, G.B., Stanton, J.S., Wagner, C.R., and Wilson, J.T., 2018, Continuing progress toward a national assessment of water availability and use: U.S. Geological Survey Circular 1440, 64 p., accessed June 7, 2021, at https://doi.org/10.3133/cir1440.
Facka, A.N., Roemer, G.W., Mathis, V.L., Kam, M., and Geffen, E., 2010, Drought Leads to Collapse of Black-Tailed Prairie Dog Populations Reintroduced to the Chihuahuan Desert: The Journal of Wildlife Management, v. 74, no. 8, p. 1752–1762, accessed July 15, 2022, at https://doi.org/10.2193/2009-208.
Feng, X., Ackerly, D.D., Dawson, T.E., Manzoni, S., Skelton, R.P., Vico, G., and Thompson, S.E., 2018, The ecohydrological context of drought and classification of plant responses: Ecology Letters, v. 21, no. 11, p. 1723–1736, accessed July 15, 2022, at https://doi.org/10.1111/ele.13139.
Gartner, J.E., Cannon, S.H., and Santi, P.M., 2014, Empirical models for predicting volumes of sediment deposited by debris flows and sediment-laden floods in the transverse ranges of southern California: Engineering Geology, v. 176, p. 45–56, accessed July 15, 2022, at https://doi.org/10.1016/j.enggeo.2014.04.008.
Glenn, E.P., Nagler, P.L., Shafroth, P.B., and Jarchow, C.J., 2017, Effectiveness of environmental flows for riparian restoration in arid regions—A tale of four rivers: Ecological Engineering, v. 106, p. 695–703, accessed July 15, 2022, at https://doi.org/10.1016/j.ecoleng.2017.01.009.
Goodrick, S.L., Achtemeier, G.L., Larkin, N.K., Liu, Y., and Strand, T.M., 2012, Modelling smoke transport from wildland fires—A review: International Journal of Wildland Fire, v. 22, no. 1, p. 83–94, accessed July 15, 2022, at https://doi.org/10.1071/WF11116.
Grams, P.E., Dean, D.J., Walker, A.E., Kasprak, A., and Schmidt, J.C., 2020, The roles of flood magnitude and duration in controlling channel width and complexity on the Green River in Canyonlands, Utah, USA: Geomorphology, v. 371, p. 107438, accessed July 15, 2022, at https://doi.org/10.1016/j.geomorph.2020.107438.
Green, T.R., Taniguchi, M., Kooi, H., Gurdak, J.J., Allen, D.M., Hiscock, K.M., Treidel, H., and Aureli, A., 2011, Beneath the surface of global change—Impacts of climate change on groundwater: Journal of Hydrology, v. 405, no. 3, p. 532–560, accessed July 15, 2022, at https://doi.org/10.1016/j.jhydrol.2011.05.002.
Gremer, J.R., Bradford, J.B., Munson, S.M., and Duniway, M.C., 2015, Desert grassland responses to climate and soil moisture suggest divergent vulnerabilities across the southwestern United States: Global Change Biology, v. 21, no. 11, p. 4049–4062. https://doi.org/10.1111/gcb.13043.
Guay, B.E., Eastoe, C.J., Bassett, R., and Long, A., 2006, Identifying sources of groundwater in the lower Colorado River valley, USA, with δ18O, δD, and 3H—Implications for river water accounting: Hydrogeology Journal, v. 14, p. 146–158, accessed July 15, 2022, at https://doi.org/10.1007/s10040-004-0334-4.
Hammarstrom, J.M., Dicken, C.L., Day, W.C., Hofstra, A.H., Drenth, B.J., Shah, A.K., McCafferty, A.E., Woodruff, L.G., Foley, N.K., Ponce, D.A., Frost, T.P., and Stillings, L.L., 2020, Focus areas for data acquisition for potential domestic resources of 11 critical minerals in the conterminous United States, Hawaii, and Puerto Rico—Aluminum, cobalt, graphite, lithium, niobium, platinum-group elements, rare earth elements, tantalum, tin, titanium, and tungsten, chap. B of Focus Areas for Data Acquisition for Potential Domestic Sources of Critical Minerals: U.S. Geological Survey Open-File Report 2019-1023-B, 67 p., accessed June 7, 2021, at https://doi.org/10.3133/ofr20191023B.
Hem, J.D., 1985, Study and interpretation of the chemical characteristics of natural water: U.S. Geological Survey Water Supply Paper 2254, 263 p., accessed June 7, 2021, at https://doi.org/10.3133/wsp2254.
Hinojosa-Huerta, O., Nagler, P.L., Carrillo-Guererro, Y.K., and Glenn, E.P., 2013, Effects of drought on birds and riparian vegetation in the Colorado River Delta, Mexico: Ecological Engineering, v. 51, p. 275–281, accessed July 15, 2022, at https://doi.org/10.1016/j.ecoleng.2012.12.082.
Hitzman, M., Selley, D., and Bull, S.W., 2010, Formation of Sedimentary Rock-Hosted Stratiform Copper Deposits through Earth History: Economic Geology and the Bulletin of the Society of Economic Geologists, v. 105, no. 3, p. 627–639, accessed July 15, 2022, at https://doi.org/10.2113/gsecongeo.105.3.627.
Hofstra, A.H., and Kreiner, D., 2020, Systems-deposits-commodities-critical minerals table for the earth mapping resources initiative: U.S. Geological Survey Open-File Report 2020–1042, 26 p., accessed June 7, 2021, at https://doi.org/10.3133/ofr20201042.
Holm, S.M., Miller, M.D., and Balmes, J.R., 2021, Health effects of wildfire smoke in children and public health tools—A narrative review: Journal of Exposure Science & Environmental Epidemiology, v. 31, p. 1–20, accessed July 15, 2022, at https://doi.org/10.1038/s41370-020-00267-4.
Homer, C.G., Xian, G., Aldridge, C.L., Meyer, D.K., Loveland, T.R., and O’Donnell, M.S., 2015, Forecasting sagebrush ecosystem components and greater sage-grouse habitat for 2050—Learning from past climate patterns and Landsat imagery to predict the future: Ecological Indicators, v. 55, p. 131–145, accessed July 15, 2022, at https://doi.org/10.1016/j.ecolind.2015.03.002.
Hoover, D.L., Duniway, M.C., and Belnap, J., 2017, Testing the apparent resistance of three dominant plants to chronic drought on the Colorado Plateau: Journal of Ecology, v. 105, no. 1, p. 152–162, accessed July 15, 2022, at https://doi.org/10.1111/1365-2745.12647.
Hossack, B.R., Honeycutt, R.K., Sigafus, B.H., Muths, E., Crawford, C.L., Jones, T.R., Sorensen, J.A., Rorabaugh, J.C., and Chambert, T., 2017, Informing recovery in a human-transformed landscape—Drought-mediated coexistence alters population trends of an imperiled salamander and invasive predators: Biological Conservation, v. 209, p. 377–394, accessed July 15, 2022, at https://doi.org/10.1016/j.biocon.2017.03.004.
Howell, P.E., Hossack, B.R., Muths, E., Sigafus, B.H., Chenevert-Steffler, A., and Chandler, R.B., 2020, A statistical forecasting approach to metapopulation viability analysis: Ecological Applications, v. 30, no. 2, article e02038, accessed July 15, 2022, at https://doi.org/10.1002/eap.2038.
Hubbs, C., 1995, Perspectives—Springs and Spring Runs as Unique Aquatic Systems: Copeia, v. 1995, no. 4, p. 989–991, accessed July 15, 2022, at https://doi.org/10.2307/1447053.
Huckleberry, J.K., and Potts, M.D., 2019, Constraints to implementing the food-energy-water nexus concept—Governance in the Lower Colorado River Basin: Environmental Science & Policy, v. 92, p. 289–298, accessed July 15, 2022, at https://doi.org/10.1016/j.envsci.2018.11.027.
Jana, S., Rajagopalan, B., Alexander, M.A., and Ray, A.J., 2018, Understanding the Dominant Sources and Tracks of Moisture for Summer Rainfall in the Southwest United States: Journal of Geophysical Research—Atmospheres, v. 123, no. 10, p. 4850–4870, accessed July 15, 2022, at https://doi.org/10.1029/2017JD027652.
Jorgensen, D., 2016, The Garden and Beyond—The Dry Season, the Ok Tedi Shutdown, and the Footprint of the 2015 El Niño Drought: Oceania, v. 86, no. 1, p. 25–39, accessed July 15, 2022, at https://doi.org/10.1002/ocea.5121.
Keane, R.E., Holsinger, L.M., and Pratt, S.D., 2006, Simulating historical landscape dynamics using the landscape fire succession model LANDSUM version 4.0: Gen. Tech. Rep. RMRS-GTR-171. Fort Collins, CO: U.S. Department of Agriculture, Forest Service, Rocky Mountain Research Station, 73 p., v. 171, accessed July 15, 2022, at https://doi.org/10.2737/RMRS-GTR-171.
Kløve, B., Ala-Aho, P., Bertrand, G., Gurdak, J.J., Kupfersberger, H., Kværner, J., Muotka, T., Mykrä, H., Preda, E., Rossi, P., Uvo, C.B., Velasco, E., and Pulido-Velazquez, M., 2014, Climate change impacts on groundwater and dependent ecosystems: Journal of Hydrology, v. 518, p. 250–266, accessed July 15, 2022, at https://doi.org/10.1016/j.jhydrol.2013.06.037.
Konikow, L.F., 2013, Groundwater Depletion in the United States (1900–2008): U.S. Geological Survey Scientific Investigations Report 2013–5079, 63 p., accessed June 7, 2021, at https://doi.org/10.3133/sir20135079.
Kramer, H.A., Mockrin, M.H., Alexandre, P.M., Stewart, S.I., and Radeloff, V.C., 2018, Where wildfires destroy buildings in the US relative to the wildland-urban interface and national fire outreach programs: International Journal of Wildland Fire, v. 27, no. 5, p. 329–341, accessed July 15, 2022, at https://doi.org/10.1071/WF17135.
Leveille, R.A., and Stegen, R.J., 2012, The Southwestern North America Porphyry Copper Province, in Hedenquist, J.W., Harris, M., Camus, F., eds., Geology and Genesis of Major Copper Deposits and Districts of the World—A Tribute to Richard H. Sillitoe: Littleton, Colo., Society of Economic Geologists, p. 361–401, accessed July 15, 2022, at https://doi.org/10.5382/SP.16.15.
Li, D., Wrzesien, M.L., Durand, M., Adam, J., and Lettenmaier, D.P., 2017, How much runoff originates as snow in the western United States, and how will that change in the future?: Geophysical Research Letters, v. 44, no. 12, p. 6163–6172, accessed July 15, 2022, at https://doi.org/10.1002/2017GL073551.
Lin, L., Gettelman, A., Fu, Q., and Xu, Y., 2018, Simulated differences in 21st century aridity due to different scenarios of greenhouse gases and aerosols: Climatic Change, v. 146, no. 3, p. 407–422, accessed July 15, 2022, at https://doi.org/10.1007/s10584-016-1615-3.
Littell, J.S., Peterson, D.L., Riley, K.L., Liu, Y., and Luce, C.H., 2016, A review of the relationships between drought and forest fire in the United States: Global Change Biology, v. 22, no. 7, p. 2353–2369, accessed July 15, 2022, at https://doi.org/10.1111/gcb.13275.
Liu, J.C., Pereira, G., Uhl, S.A., Bravo, M.A., and Bell, M.L., 2015, A systematic review of the physical health impacts from non-occupational exposure to wildfire smoke: Environmental Research, v. 136, p. 120–132, accessed July 15, 2022, at https://doi.org/10.1016/j.envres.2014.10.015.
Lukas, J., and Payton, E., 2020, Colorado River Basin Climate and Hydrology—State of the Science: University of Colorado Boulder Western Water Assessment, 520 p., accessed March 5, 2021, at https://wwa.colorado.edu/resources/colorado-river-resources/CRBreport.
Manners, R.B., Schmidt, J.C., and Scott, M.L., 2014, Mechanisms of vegetation-induced channel narrowing of an unregulated canyon river—Results from a natural field-scale experiment: Geomorphology, v. 211, p. 100–115, accessed July 15, 2022, at https://doi.org/10.1016/j.geomorph.2013.12.033.
Martínez-Vilalta, J., and Lloret, F., 2016, Drought-induced vegetation shifts in terrestrial ecosystems—The key role of regeneration dynamics: Global and Planetary Change, v. 144, p. 94–108, accessed July 15, 2022, at https://doi.org/10.1016/j.gloplacha.2016.07.009.
Maschinski, J., Baggs, J.E., Quintana-Ascencio, P.F., and Menges, E.S., 2006, Using population viability analysis to predict the effects of climate change on the extinction risk of an endangered limestone endemic shrub, Arizona cliffrose—Conservation Biology: Conservation Biology, v. 20, no. 1, p. 218–228, accessed July 15, 2022, at https://doi.org/10.1111/j.1523-1739.2006.00272.x.
Mast, A., 2017, Estimation of salt loads for the Dolores River in the Paradox Valley, Colorado, 1980–2015: U.S. Geological Survey Scientific Investigations Report 2017–5059, 20 p., accessed June 7, 2021, at https://doi.org/10.3133/sir20175059.
Maupin, M.A., Ivahnenko, T.I., and Bruce, B., 2018, Estimates of water use and trends in the Colorado River Basin, Southwestern United States, 1985–2010: U.S. Geological Survey Scientific Investigations Report 2018–5049, 61 p., accessed February 17, 2021, at https://doi.org/10.3133/sir20185049.
McCabe, G.J., and Wolock, D.M., 2021a, Multi-year hydroclimatic droughts and pluvials across the conterminous United States: International Journal of Climatology, v. 41, no. 3, p. 1731–1746, accessed July 15, 2022, at https://doi.org/10.1002/joc.6925.
McCabe, G.J., and Wolock, D.M., 2021b, Water balance of the turn-of-the-century drought in the Southwestern United States: Environmental Research Letters, v. 16, no. 4, article 044015, accessed July 15, 2022, at https://doi.org/10.1088/1748-9326/abbfc1.
McCabe, G.J., Wolock, D.M., Woodhouse, C.A., Pederson, G.T., McAfee, S.A., Gray, S., and Csank, A., 2020, Basinwide Hydroclimatic Drought in the Colorado River Basin: Earth Interactions, v. 24, no. 2, p. 1–20, accessed July 15, 2022, at https://doi.org/10.1175/EI-D-20-0001.1.
McIntosh, M.E., Boyd, A.E., Arnold, A.E., Steidl, R.J., and McDade, L.A., 2020, Growth and demography of a declining, endangered cactus in the Sonoran Desert: Plant Species Biology, v. 35, no. 1, p. 6–15, accessed July 15, 2022, at https://doi.org/10.1111/1442-1984.12251.
McNeeley, S.M., Beeton, T.A., and Ojima, D.S., 2016, Drought Risk and Adaptation in the Interior United States—Understanding the Importance of Local Context for Resource Management in Times of Drought: Weather, Climate, and Society, v. 8, no. 2, p. 147–161, accessed July 15, 2022, at https://doi.org/10.1175/WCAS-D-15-0042.1.
Miller, M.P., Buto, S.G., Susong, D.D., and Rumsey, C.A., 2016, The importance of base flow in sustaining surface water flow in the Upper Colorado River Basin: Water Resources Research, v. 52, no. 5, p. 3547–3562, accessed July 15, 2022, at https://doi.org/10.1002/2015WR017963.
Miller, M.P., Susong, D.D., Shope, C.L., Heilweil, V.M., and Stolp, B.J., 2014, Continuous estimation of baseflow in snowmelt-dominated streams and rivers in the Upper Colorado River Basin—A chemical hydrograph separation approach: Water Resources Research, v. 50, no. 8, p. 6986–6999, accessed July 15, 2022, at https://doi.org/10.1002/2013WR014939.
Mote, P.W., Li, S., Lettenmaier, D.P., Xiao, M., and Engel, R., 2018, Dramatic declines in snowpack in the western US: npj Climate and Atmospheric Science, v. 1, no. 2, 6 p., accessed July 15, 2022, at https://doi.org/10.1038/s41612-018-0012-1.
Murphy, S.F., McCleskey, R.B., Martin, D.A., Writer, J.H., and Ebel, B.A., 2018, Fire, Flood, and Drought—Extreme Climate Events Alter Flow Paths and Stream Chemistry: Journal of Geophysical Research. Biogeosciences, v. 123, no. 8, p. 2513–2526, accessed July 15, 2022, at https://doi.org/10.1029/2017JG004349.
Ostroff, A.C., Muhlfeld, C.C., Lambert, P.M., Booth, N.L., Carter, S.L., Stoker, J.M., and Focazio, M.J., 2017, USGS integrated drought science: U.S. Geological Survey Circular 1430, 24 p., accessed March 5, 2021, at https://doi.org/10.3133/cir1430.
Painter, T.H., Deems, J.S., Belnap, J., Hamlet, A.F., Landry, C.C., and Udall, B., 2010, Response of Colorado River runoff to dust radiative forcing in snow: Proceedings of the National Academy of Sciences of the United States of America, v. 107, no. 40, p. 17125–17130, accessed July 15, 2022, at https://doi.org/10.1073/pnas.0913139107.
Peery, M.Z., Gutiérrez, R.J., Kirby, R., LeDee, O.E., and LaHaye, W., 2012, Climate change and spotted owls—Potentially contrasting responses in the Southwestern United States: Global Change Biology, v. 18, no. 3, p. 865–880, accessed July 15, 2022, at https://doi.org/10.1111/j.1365-2486.2011.02564.x.
Perla, B.S., and Stevens, L.E., 2008, Biodiversity and productivity at an undisturbed spring, in comparison with adjacent grazed riparian and upland habitats, chap. 11 of Stevens, L.E., and Meretsky, V.J., eds., Aridland springs in North America—Ecology and conservation: Tuscon, Ariz., University of Arizona Press, p. 230–243.
Phillips, F.M., Walvoord, M.A., and Small, E.E., 2004, Effects of Environmental Change on Groundwater Recharge in the Desert Southwest, in Hogan, J.F., Phillips, F.M., and Small, E.E., eds., Groundwater Recharge in a Desert Environment—The Southwestern United States, Volume 9: Washington, D.C., American Geophysical Union, p. 273–294, accessed July 15, 2022, at https://doi.org/10.1029/009WSA15
Picotte, J.J., Dockter, D., Long, J., Tolk, B., Davidson, A., and Peterson, B., 2019, LANDFIRE Remap Prototype Mapping Effort—Developing a New Framework for Mapping Vegetation Classification, Change, and Structure: Fire, v. 2, no. 2, p. 35, accessed July 15, 2022, at https://doi.org/10.3390/fire2020035.
Plummer, L.N., Sanford, W.E., Bexfield, L.M., Anderholm, S.K., and Busenberg, E., 2004, Using Geochemical Data and Aquifer Simulation to Characterize Recharge and Groundwater Flow in the Middle Rio Grande Basin, New Mexico, in Hogan, J.F., Phillips, F.M., and Small, E.E., eds., Groundwater Recharge in a Desert Environment—The Southwestern United States, Volume 9: Washington, D.C., American Geophysical Union, p. 185–216, accessed July 15, 2022, at https://agupubs.onlinelibrary.wiley.com/doi/10.1002/9781118665664.ch11.
Prein, A.F., Rasmussen, R.M., Ikeda, K., Liu, C., Clark, M.P., and Holland, G.J., 2017, The future intensification of hourly precipitation extremes: Nature Climate Change, v. 7, p. 48–52, accessed July 15, 2022, at https://doi.org/10.1038/nclimate3168.
PRISM Climate Group, 2021, Northwest Alliance for Computational Science and Engineering: Oregon State University, PRISM Climate Group website, accessed July 15, 2022, at https://prism.oregonstate.edu.
Radeloff, V.C., Helmers, D.P., Kramer, H.A., Mockrin, M.H., Alexandre, P.M., Bar-Massada, A., Butsic, V., Hawbaker, T.J., Martinuzzi, S., Syphard, A.D., and Stewart, S.I., 2018, Rapid growth of the US wildland-urban interface raises wildfire risk: Proceedings of the National Academy of Sciences of the United States of America, v. 115, no. 13, p. 3314–3319, accessed July 15, 2022, at https://doi.org/10.1073/pnas.1718850115.
Renne, R.R., Schlaepfer, D.R., Palmquist, K.A., Bradford, J.B., Burke, I.C., and Lauenroth, W.K., 2019, Soil and stand structure explain shrub mortality patterns following global change–type drought and extreme precipitation: Ecology, v. 100, no. 12, article e02889, accessed July 15, 2022, at https://doi.org/10.1002/ecy.2889.
Reynolds, J.F., Smith, D.M.S., Lambin, E.F., Turner, B.L., II, Mortimore, M., Batterbury, S.P.J., Downing, T.E., Dowlatabadi, H., Fernández, R.J., Herrick, J.E., Huber-Sannwald, E., Jiang, H., Leemans, R., Lynam, T., Maestre, F.T., Ayarza, M., and Walker, B., 2007, Global Desertification—Building a Science for Dryland Development: Science, v. 316, no. 5826, p. 847–851, accessed July 15, 2022, at https://doi.org/10.1126/science.1131634.
Riggs, A.C., and Deacon, J.E., 2004, Connectivity in desert aquatic ecosystems—The Devils Hole story., in Conference Proceedings, Spring-fed Wetlands—Important Scientific and Cultural Resources of the Intermountain Region, 2002: Reno, Nev., Desert Research Institute Report No. 41175, 38 p., accessed July 15, 2022, at https://citeseerx.ist.psu.edu/pdf/f14bcdcfaac07e66fea90474b43d5f73fd8043f0.
Robison, J., Bratrschovsky, K., Latcham, J., Morris, E., Palmer, V., and Villanueva, A., 2014, Challenge and response in the Colorado River Basin: Water Policy, v. 16, no. S1, p. 12–57, accessed July 15, 2022, at https://doi.org/10.2166/wp.2014.003.
Rocca, M.E., Brown, P.M., MacDonald, L.H., and Carrico, C.M., 2014, Climate change impacts on fire regimes and key ecosystem services in Rocky Mountain forests: Forest Ecology and Management, v. 327, p. 290–305, accessed July 15, 2022, at https://doi.org/10.1016/j.foreco.2014.04.005.
Rumsey, C.A., Miller, M.P., Schwarz, G.E., Hirsch, R.M., and Susong, D.D., 2017, The role of baseflow in dissolved solids delivery to streams in the Upper Colorado River Basin: Hydrological Processes, v. 31, no. 26, p. 4705–4718, accessed July 15, 2022, at https://doi.org/10.1002/hyp.11390.
Rumsey, C.A., Miller, M.P., Susong, D.D., Tillman, F.D., and Anning, D.W., 2015, Regional scale estimates of baseflow and factors influencing baseflow in the Upper Colorado River Basin: Journal of Hydrology—Regional Studies, v. 4, p. 91–107, accessed July 15, 2022, at https://doi.org/10.1016/j.ejrh.2015.04.008.
Schlaepfer, D.R., Taylor, K.A., Pennington, V.E., Nelson, K.N., Martyn, T.E., Rottler, C.M., Lauenroth, W.K., and Bradford, J.B., 2015, Simulated big sagebrush regeneration supports predicted changes at the trailing and leading edges of distribution shifts: Ecosphere, v. 6, no. 1, p. 1–31, accessed July 15, 2022, at https://doi.org/10.1890/ES14-00208.1.
Seager, R., Ting, M., Held, I., Kushnir, Y., Lu, J., Vecchi, G., Huang, H.-P., Harnik, N., Leetmaa, A., Lau, N.-C., Li, C., Velez, J., and Naik, N., 2007, Model Projections of an Imminent Transition to a More Arid Climate in Southwestern North America: Science, v. 316, no. 5828, p. 1181–1184, accessed July 15, 2022, at https://doi.org/10.1126/science.1139601.
Seamans, M.E., Gutiérrez, R.J., and May, C.A., 2002, Mexican Spotted Owl (Strix Occidentalis) Population Dynamics—Influence of Climatic Variation on Survival and Reproduction: The Auk, v. 119, no. 2, p. 321–334, accessed July 15, 2022, at https://doi.org/10.1093/auk/119.2.321.
Shafroth, P.B., Schlatter, K.J., Gomez-Sapiens, M., Lundgren, E., Grabau, M.R., Ramírez-Hernández, J., Rodríguez-Burgueño, J.E., and Flessa, K.W., 2017, A large-scale environmental flow experiment for riparian restoration in the Colorado River Delta: Ecological Engineering, v. 106, p. 645–660, accessed July 15, 2022, at https://doi.org/10.1016/j.ecoleng.2017.02.016.
Shriver, R.K., Andrews, C.M., Pilliod, D.S., Arkle, R.S., Welty, J.L., Germino, M.J., Duniway, M.C., Pyke, D.A., and Bradford, J.B., 2018, Adapting management to a changing world—Warm temperatures, dry soil, and interannual variability limit restoration success of a dominant woody shrub in temperate drylands: Global Change Biology, v. 24, no. 10, p. 4972–4982, accessed July 15, 2022, at https://doi.org/10.1111/gcb.14374.
Staley, D.M., Tillery, A.C., Kean, J.W., McGuire, L.A., Pauling, H.E., Rengers, F.K., and Smith, J.B., 2018, Estimating post-fire debris-flow hazards prior to wildfire using a statistical analysis of historical distributions of fire severity from remote sensing data: International Journal of Wildland Fire, v. 27, no. 9, p. 595, accessed July 15, 2022, at https://doi.org/10.1071/WF17122.
Stephens, T., Wilson, S.C., Cassidy, F., Bender, D., Gummer, D., Smith, D.H.V., Lloyd, N., McPherson, J.M., and Moehrenschlager, A., 2018, Climate change impacts on the conservation outlook of populations on the poleward periphery of species ranges—A case study of Canadian black-tailed prairie dogs (Cynomys ludovicianus): Global Change Biology, v. 24, no. 2, p. 836–847, accessed July 15, 2022, at https://doi.org/10.1111/gcb.13922.
Stevens, L.E., Jenness, J., and Ledbetter, J.D., 2020, Springs and Springs-Dependent Taxa of the Colorado River Basin, Southwestern North America—Geography, Ecology and Human Impacts: Water, v. 12, no. 5, p. 1501, accessed July 15, 2022, at https://doi.org/10.3390/w12051501.
Stevens-Rumann, C.S., Kemp, K.B., Higuera, P.E., Harvey, B.J., Rother, M.T., Donato, D.C., Morgan, P., and Veblen, T.T., 2018, Evidence for declining forest resilience to wildfires under climate change: Ecology Letters, v. 21, no. 2, p. 243–252, accessed July 15, 2022, at https://doi.org/10.1111/ele.12889.
Thoma, D.P., Munson, S.M., and Witwicki, D.L., 2019, Landscape pivot points and responses to water balance in national parks of the southwest US: Journal of Applied Ecology, v. 56, no. 1, p. 157–167, accessed July 15, 2022, at https://doi.org/10.1111/1365-2664.13250.
Thorson, J.P., and MacIntyre, T.J., 2005, Geology of the Cashin Mine Sandstone-Hosted Disseminated Copper Deposit, Montrose County, Colorado, in Thorson, J.P., ed., Lisbon Valley Sediment-Hosted Copper Deposits and Paradox Basin Fluids Trip: Littleton, Colo., Society of Economic Geologists, p. 43–50, accessed July 15, 2022, at https://doi.org/10.5382/GB.37.ch4.
Tillman, F.D., Gangopadhyay, S., and Pruitt, T., 2020, Trends in recent historical and projected climate data for the Colorado River Basin and potential effects on groundwater availability: U.S. Geological Survey Scientific Investigations Report 5107, 24 p., accessed June 7, 2021, at https://doi.org/10.3133/sir20205107.
Topping, D.J., Mueller, E.R., Schmidt, J.C., Griffiths, R.E., Dean, D.J., and Grams, P.E., 2018, Long-Term Evolution of Sand Transport Through a River Network—Relative Influences of a Dam Versus Natural Changes in Grain Size From Sand Waves: Journal of Geophysical Research. Earth Surface, v. 123, no. 8, p. 1879–1909, accessed July 15, 2022, at https://doi.org/10.1029/2017JF004534.
Trenberth, K.E., Dai, A., van der Schrier, G., Jones, P.D., Barichivich, J., Briffa, K.R., and Sheffield, J., 2014, Global warming and changes in drought: Nature Climate Change, v. 4, p. 17–22, accessed July 15, 2022, at https://doi.org/10.1038/nclimate2067.
Udall, B., and Overpeck, J., 2017, The twenty-first century Colorado River hot drought and implications for the future: Water Resources Research, v. 53, no. 3, p. 2404–2418, accessed July 15, 2022, at https://doi.org/10.1002/2016WR019638.
Ukkola, A.M., Pitman, A.J., Kauwe, M.G.D., Abramowitz, G., Herger, N., Evans, J.P., and Decker, M., 2018, Evaluating CMIP5 Model Agreement for Multiple Drought Metrics: Journal of Hydrometeorology, v. 19, no. 6, p. 969–988, accessed July 15, 2022, at https://doi.org/10.1175/JHM-D-17-0099.1.
Urbanski, S.P., Reeves, M.C., Corley, R.E., Silverstein, R.P., and Hao, W.M., 2018, Contiguous United States wildland fire emission estimates during 2003–2015: Earth System Science Data, v. 10, no. 4, p. 2241–2274, accessed July 15, 2022, at https://doi.org/10.5194/essd-10-2241-2018.
U.S. Department of Agriculture, 2019, 2017 Census of Agriculture: U.S. Department of Agriculture, National Agricultural Statistics Service website, accessed June 14, 2021, at https://www.nass.usda.gov/Publications/AgCensus/2017/index.php.
U.S. Department of Agriculture, 2021, Forest Service FSGeodata Clearinghouse - Timber Harvests, accessed February 16, 2021, at https://data.fs.usda.gov/geodata/edw/datasets.php.
U.S. Department of the Interior, 2021, Economic Contributions Report FY2019: U.S. Department of the Interior ScienceBase website, accessed February 16, 2021, at https://doi.sciencebase.gov/doidv/index.html.
U.S. Energy Information Administration, 2016, Shale gas and oil plays, Lower 48 States (updated 6/30/2016): U.S. Energy Information Administration website, accessed May 18, 2021, at https://www.eia.gov/maps/maps.htm.
U.S. Geological Survey Gap Analysis Project , 2020, Protected Areas Database of the United States (PAD-US) 2.1: U.S. Geological Survey data release, accessed July 15, 2022, at https://doi.org/10.5066/P92QM3NT.
Walker, A.E., Moore, J.N., Grams, P.E., Dean, D.J., and Schmidt, J.C., 2020, Channel narrowing by inset floodplain formation of the lower Green River in the Canyonlands region, Utah: Geological Society of America Bulletin, v. 132, no. 11–12, p. 2333–2352, accessed July 15, 2022, at https://doi.org/10.1130/B35233.1.
Warren, S., 1984, Impurities in Snow—Effects on Albedo and Snowmelt: Annals of Glaciology, v. 5, p. 177–179, accessed July 15, 2022, at https://doi.org/10.3189/1984AoG5-1-177-179.
Watts, K.R., 2000, Effects of the Paradox Valley Unit on dissolved solids, sodium, and chloride in the Dolores River near Bedrock, Colorado, water years 1988−98: U.S. Geological Survey Water-Resources Investigations Report 00-4011, 8 p., accessed June 7, 2021, at https://doi.org/10.3133/wri004011.
Westerling, A.L., Hidalgo, H.G., Cayan, D.R., and Swetnam, T.W., 2006, Warming and earlier spring increase western US forest wildfire activity: Science, v. 313, no. 5789, p. 940–943, accessed July 15, 2022, at https://doi.org/10.1126/science.1128834.
Williams, A.P., Allen, C.D., Macalady, A.K., Griffin, D., Woodhouse, C.A., Meko, D.M., Swetnam, T.W., Rauscher, S.A., Seager, R., Grissino-Mayer, H.D., Dean, J.S., Cook, E.R., Gangodagamage, C., Cai, M., and others, 2013, Temperature as a potent driver of regional forest drought stress and tree mortality: Nature Climate Change, v. 3, no. 3, p. 292–297, accessed July 15, 2022, at https://doi.org/10.1038/nclimate1693.
Williams, A.P., Cook, E.R., Smerdon, J.E., Cook, B.I., Abatzoglou, J.T., Bolles, K., Baek, S.H., Badger, A.M., and Livneh, B., 2020, Large contribution from anthropogenic warming to an emerging North American megadrought: Science, v. 368, no. 6488, p. 314–318, accessed July 15, 2022, at https://doi.org/10.1126/science.aaz9600.
Williams, C.F., Reed, M.J., Mariner, R.H., DeAngelo, J., and Galanis, S.P., Jr., 2008, Assessment of Moderate- and High-Temperature Geothermal Resources of the United States: U.S. Geological Survey Fact Sheet 2008-3082, 4 p., accessed June 7, 2021, at https://doi.org/10.3133/fs20083082.
Witwicki, D.L., Munson, S.M., and Thoma, D.P., 2016, Effects of climate and water balance across grasslands of varying C3 and C4 grass cover: Ecosphere, v. 7, no. 11, article e01577, accessed July 15, 2022, at https://doi.org/10.1002/ecs2.1577.
Zhou, S., Zhang, Y., Williams, A.P., and Gentine, P., 2019, Projected increases in intensity, frequency, and terrestrial carbon costs of compound drought and aridity events: Science Advances, v. 5, no. 1, article eaau5740, accessed July 15, 2022, at https://doi.org/10.1126/sciadv.aau5740.
Appendix 2. Colorado River Basin ASIST Project Use Cases
Table 2.1.
List of Colorado River Basin Short-Term Use Case Projects funded in fiscal year 2021 (October 2020 – September 2021).[SBSC, Southwest Biological Science Center; GECSC, Geosciences and Environmental Chance Science Center; UTWSC, Utah Water Science Center; CAWSC, California Water Science Center; COWSC, Colorado Water Science Center; CERSC, Central Energy Resources Science Center; EMMA, Energy and Minerals Mission Area; SPARROW, SPAtially Referenced Regressions On Watershed Attributes; NVWSC, Nevada Water Science Center]
Colorado River Basin Short-Term Use Case Project Descriptions
Descriptions of short-term use cases funded by the Colorado River Basin ASIST project team are included in this section. These use cases along with other example USGS projects are highlighted on the Colorado River Basin Projects web application: https://apps.usgs.gov/colorado-river-basin/integrated-drought-science.html.
A Drought Data Explorer for the Colorado River Basin—Integrated and Dynamic Web-Based Delivery of Actionable Information
Principal Investigator: Kathryn Thomas (kathryn_a_thomas@usgs.gov), U.S. Geological Survey, Southwest Biological Science Center, Tucson, Arizona
Project Description: The Terrestrial Drylands Ecology (TDE) Branch of Southwest Biological Science Center (SBSC) recognizes that dynamic delivery of integrated data, models and tools resulting from their collective dryland and drought research is needed to maximize the actionable utility of their science and to support the diverse stakeholders who rely upon this work. An essential step to increase the utility of this science is an interactive web-based drought data explorer (hereafter shortened to Explorer) to help managers with their critical decisions to mitigate, adapt, and prepare for drought-induced changes to Colorado River Basin landscapes. In 2021, a pilot of the Explorer was initiated. This includes initiating an implementation plan, determining how to make data intended for the Explorer analysis-ready with attention toward cloud portability, and developing a conceptual scientific framework for presentation of scientist’s drought products with attention to stakeholder needs within the Colorado River Basin. The informational needs of land managers and other science users within the Colorado River Basin are being identified by a collaborative effort between SBSC, TDE and Northern Arizona University to address ecological drought and guide the refinement of the Explorer’s dashboard design. Development of the public Explorer is expected to be iterative with each step by (1) expanding upon the capabilities of the SBSC information technology and data management, and the TDE scientists understanding, (2) expanding upon the use of a 21st century technology for visualization and analysis, and (3) integrating these center-oriented activities with USGS advanced computing resources.
Associated Data:
Schlaepfer, D.R., Andrews, C.M., and Bradford, J.B., 2022, Historical and future ecological drought conditions for rangelands of the western U.S.: U.S. Geological Survey data release, accessed July 15, 2022, at https://doi.org/10.5066/P97S8RAC.
Stakeholders: U.S. Geological Survey scientists, Bureau of Land Management, Bureau of Indian Affairs, National Park Service, U.S. Fish and Wildlife Service, Department of Energy, Department of Defense, U.S. Department of Agriculture, Arizona Game and Fish Department, Arizona Forestry and Fire Management, Utah Division of Natural Resources, Navajo Nation Division of Natural Resources, Hopi Department of Natural Resources, Ute Mountain Ute Tribe, Northern Arizona University, University of Arizona, Arizona State University, Utah State University, University of Utah, Brigham Young University, New Mexico State University.
Keywords: droughts, ecology, terrestrial ecosystems, biogeography, habitats, geographic information systems, plants (organisms), insects, desert ecosystems
Advanced Integrated Fire Science (AIFS) to Enhance Prediction of Post-Fire Hazards, Risk Assessments, and Decision Making
Principal Investigators: Paul Steblein (psteblein@usgs.gov), U.S. Geological Survey, Ecosystems, Reston, Va.; Todd Hawbaker (tjhawbaker@usgs.gov), U.S. Geological Survey, Geosciences and Environmental Changes Science Center, Denver, Colo.; Rachel Loehman (rloehman@usgs.gov), U.S. Geological Survey, Alaska Science Center, Anchorage, Alaska; Adam Wells (agwells@usgs.gov), U.S. Geological Survey, Southwest Biological Science Center, Flagstaff, Ariz.
Project Description: The danger posed by wildland fires has grown because of past land management decisions and the results of long-term drought and a warming climate. The effects of wildland fires on the landscape are varied depending on the severity and spatial extent of a fire. The AIFS team is developing an integrated approach to assess where severe wildfires may occur, to predict the possible impacts of severe wildfires on these landscapes, and to prioritize mitigation and remediation activities to reduce the likelihood, severity, and impacts of wildland fires.
The Wildland Fire Leadership Council (hereafter the Council) was created to inform researchers on the needed science to provide actionable information to stakeholders. The Council represents a diverse group of stakeholders including Federal, State, regional, Tribal, and local communities. A priority of the Council is to assess and predict the occurrence, severity, and impact of wildland fires on communities and resources in the Colorado River Basin. The work for the AFIS project is focused on this priority by sourcing data and models that will be used to assess burn severity and determine the potential for post-fire debris flows from burned areas, and the impacts that debris flows may have on water quality. These results will help to identify and prioritize subbasins in the Colorado River Basin that may benefit from mitigation actions to help reduce the potential for severe wildland fires.
Associated Websites:
https://www.usgs.gov/special-topics/wildland-fire-science, accessed September 30, 2022
Associated Publications:
Steblein, P.F., Loehman, R.A., Miller, M.P., Holomuzki, J.R., Soileau, S.C., Brooks, M.L., Drane-Maury, M., Hamilton, H.M., Kean, J.W., Keeley, J.E., Mason, R.R., Jr., McKerrow, A., Meldrum, J.R., Molder, E.B., Murphy, S.F., Peterson, B., Plumlee, G.S., Shinneman, D.J., van Mantgem, P.J., and York, A., 2021, U.S. Geological Survey wildland fire science strategic plan, 2021–26: U.S. Geological Survey Circular 1471, 30 p., https://doi.org/10.3133/cir1471.
Stakeholders: Department of the Interior, Department of Agriculture, Department of Health and Safety, Department of Defense, Department of Energy, States, Tribes, Communities.
Key Words: fires, droughts, remote sensing, water quality, invasive species, land surface
Assimilating Complex Biogeochemical Dust Measurements Supports Community Standardization, Collaboration, and Environmental Health Research
Principal Investigators: Annie Putman (aputnam@usgs.gov), Molly Blakowski (mblakowski@usgs.gov), and Dan Jones (dkjones@usgs.gov), U.S. Geological Survey, Utah Water Science Center, West Valley City, Utah
Project Description: A rich array of biogeochemical data exist in supplemental material, as private collections, and are available from network datasets, for example the Critical Zone Network (https://criticalzone.org/). However, due to the current decentralized model for data preservation and access, it is difficult for researchers to find and obtain appropriate datasets for contextualizing their work, or to identify gaps in the science. These issues pervade many disciplines, including dust biogeochemistry. To address this issue and to provide a workflow and best practices prototype for organizing biogeochemical point-source data, we plan to deploy an open-access biogeochemical dust database. The database will conform to findable, accessible, interoperable, and reusable (FAIR) data standards (see https://www.go-fair.org/fair-principles/), and support interdisciplinary collaboration, standards development, and cross-scale data analysis. This project will be a case study in developing workflows and best practices for organizing and relating data from individual and network data collection efforts.
Associated Websites:
https://wateriso.utah.edu/waterisotopes/index.html, accessed September 30, 2022
https://www.usgs.gov/programs/toxic-substances-hydrology/science/geospatial-analyses-and-applications-salt-lake-city?qt-science_center_objects=0#qt-sc ience_center_objects, accessed September 30, 2022
Stakeholders: Dust research communities, land managers, health agencies
Keywords: nutrient cycling, environmental health, biogeochemistry, drought science, atmospheric, human, land surface, soils, atmospheric and climatic processes, contamination and pollution, ecosystems, health and disease, land surface characteristics, land use change, soil moisture, soil sciences, topography, transportation, vegetation
Basin Characterization Model: Development and Preliminary Application for the Colorado River Basin
Principal Investigators: Joe Hevesi (jhevesi@usgs.gov), Michelle Stern (mstern@usgs.gov), Lorraine Flint, Retired (leflint@sbcgobal.net), and Alan Flint, Retired (alflint@sbcglobal.net), U.S. Geological Survey, California Water Science Center, Sacramento, California
Project Description: Studies of the spatial and temporal variability in the natural hydrologic water balance for the Colorado River Basin for future climate and land cover scenarios are needed to evaluate the long-term sustainability of water resources of the Colorado River Basin. Predictive and adaptive modeling tools are useful to develop water management scenarios based on changing climate and land cover in the Colorado River Basin. The Basin Characterization Model (BCM) is a flexible and integrative set of modeling tools that can be applied to better understand the spatial and temporal variability of water budget components in the Colorado River Basin and to help develop predictive and integrated modeling products. The Basin Characterization Model for the Colorado River Basin (BCM-CRB) provides a flexible, integrative, and readily adaptable modeling approach for developing high-resolution maps of monthly water balance components (climate, snow cover, snow melt, sublimation, evapotranspiration, soil moisture, recharge, runoff, and climatic water deficit). The BCM-CRB is calibrated using historical climate data (1980–2020) and other historical datasets including snow cover, snow water equivalent (SWE), evapotranspiration, soil moisture, and streamflow. The calibrated BCM-CRB is updated monthly and is applied to develop monthly water balance results for future (2021–2100) climate and land cover scenarios.
An update of the BCM, from v6.5 to v8, is planned for the for the BCM-CRB. The updated model would include (1) downscaled monthly historical climate maps, from 1980 to 2020, at a 270-meter grid spacing, covering the full extent of the Colorado River Basin, (2) example model results, at 270-meter grid spacing, using an uncalibrated BCM v8 simulation for the Colorado River Basin, and (3) development of the SWE and actual evapotranspiration (AET) datasets and inputs needed for initial calibration of the BCM. The downscaled monthly climate maps will include precipitation (PPT), maximum air temperature (TMAX), minimum air temperature (TMIN), and potential evapotranspiration (PET). The simulated monthly water budget maps will include AET, SWE, sublimation, snow melt, soil moisture, runoff, recharge, and climatic water deficit (CWD), for water years 1980–2020. Initial calibration results will include SWE, AET, sublimation, soil moisture, and CWD.
Associated Websites:
https://www.usgs.gov/centers/california-water-science-center/science/basin-characterization-model-bcm, accessed September 30, 2022
Associated Publications:
Flint, L.E., Flint, A.L., and Stern, M.A., 2021, The basin characterization model—A regional water balance software package: U.S. Geological Survey Techniques and Methods 6–H1, 85 p., accessed 15 July, 2022, at https://doi.org/10.3133/tm6H1.
Keywords: hydrology, runoff, precipitation (atmospheric), groundwater and surface-water interaction, percolation, evaporation, transpiration, air temperature, soil moisture
Effects of the East Troublesome Fire on Water Quality in Rocky Mountain National Park
Principal Investigator: David Clow (dwclow@usgs.gov), U.S. Geological Survey, Colorado Water Science Center, Lakewood, Colo.
Project Description: Like much of the western United States, Colorado experienced a devastating wildfire season in 2020, with multiple large fires burning concurrently through summer and fall. One of the largest fires in state history, the 2020 East Troublesome fire, burned approximately 809 km2, including over 121 km2 in Rocky Mountain National Park (RMNP). The fires were fueled by persistent drought, large numbers of beetle-killed trees, and high winds.
Although wildfire plays an important role in maintaining healthy ecosystems, the 2020 fires were unprecedented in size for Colorado. Large “megafires” (>405 km2 or 100,000 acres) have become more common in the western United States since the mid-1980s because of warming temperatures and earlier spring snowmelt, suggesting western parks are under increasing threat from wildfire. The fires may have severe effects on the water quality of RMNP streams and lakes, which are Cold Water Aquatic Life–Class 1 Outstanding Natural Resource Waters as determined by the Colorado Water Quality Control Commission under the Clean Water Act, indicating they are of exceptional ecological and recreational significance, designated for cold water aquatic life, and receive the highest level of protection at the Federal level.
Streams in RMNP are not only of critical ecological and recreational importance; they are also a crucial source of high-quality drinking water for the public. Streams in the burned areas of RMNP flow into Grand Lake, part of a water-supply system that provides drinking water to over 1 million residents along the Colorado Front Range. This critical water-supply system is at risk from potential runoff of sediment, ash, nutrients, and carbon from burned hillslopes upstream from the reservoirs.
Previous studies researching the effects of the fire on water quality have been hampered by a lack of pre-fire water-quality data, precluding a quantitative assessment of changes in water quality associated with fire. Instead, changes could only be inferred by comparing post-fire chemistry in streams draining burned and unburned watersheds. This study benefits from a wealth of pre-fire stream-chemistry data from a previous study, providing a unique opportunity to quantify changes in water quality associated with the fire. By comparing pre- and post-fire stream-chemistry data using a statistical approach the changes in water quality in response to wildfire were evaluated. This study built statistical time-series models of carbon and turbidity concentrations using the pre-fire data. Pre-fire stream chemistry data were used as a baseline, with post-fire stream chemistry plotted as an overlay to illustrate how post-fire chemistry compares with that expected based on the pre-fire model. Consistent deviations outside the expected range of the pre-fire model estimates would indicate significant differences between pre- and post-fire chemistry.
Associated Publications:
Mast, M.A., Murphy, S.F., Clow, D.W., Penn, C., and Sexstone, G., 2015, Water-quality response to a high-elevation wildfire in the Colorado Front Range: Hydrological Processes, v. 30, no. 12, p. 1811–1823, accessed September 30, 2022, at https://doi.org/10.1002/hyp.10755.
Murphy, S.F., McCleskey, R.B., Martin, D.A., Writer, J.H., and Ebel, B.A., 2018, Fire, flood, and drought—extreme climate events alter flow paths and stream chemistry: Journal of Geophysical Research—Biogeosciences, v. 123, no. 8, p. 2513–2526, accessed September 30, 2022, at https://doi.org/10.1029/2017JG004349.
Associated Data:
Clow, D.W., Qi, S.L., and Akie, G.A., 2021, Continuous water-quality data for selected streams in Rocky Mountain National Park, Colorado, water years 2011-19: U.S. Geological Survey data release, accessed September 30, 2022, at https://doi.org/10.5066/P9RNS5FP.
Stakeholders: local communities, National Park Service, water management agencies in the Upper Colorado River Basin
Keywords: wildfire, water quality, carbon, nutrients, drinking water, debris flow
Energy Resource Development Potential and Tradeoffs, to Support Integrated Analysis for “All of the Above” Energy Strategies
Principal Investigators: Seth Haines (shaines@usgs.gov), U.S. Geological Survey, Central Energy Resources Science Center, Lakewood, Colo.; Darius Semmens (dsemmens@usgs.gov), U.S. Geological Survey, Geosciences and Environmental Change Science Center, Denver, Colo.; Jay Diffendorfer (jediffendorfer@usgs.gov), U.S. Geological Survey, Geosciences and Environmental Change Science Center, Denver, Colo.; Karen Jenni (kjenni@usgs.gov), U.S. Geological Survey, Science and Decisions Center, Denver, Colo.
Project Description: Decision makers considering possible impacts associated with development of fossil fuel and renewable energy resources often desire information on areas of potential conflict between energy resource development and land, water and socioecological resources. The U.S. Geological Survey (USGS) can address these stakeholder needs by developing approaches for linking surface and subsurface resources. As a starting point, in fiscal year 2021 (FY 2021), this project plans to assemble resource-related geospatial data that are anticipated to be useful and necessary for a suite of purposes. Compiled data will include: (1) oil and gas assessment information for the Colorado River Basin area (USGS data); (2) wind and solar energy resource assessment information from the National Renewable Energy Laboratory and other sources; (3) tabulated oil, gas, and water production per square mile grid area, water use for hydraulic fracturing, and the number of oil and gas wells; (4) energy infrastructure; (5) land-use and land-cover data; and (6) USGS water use and withdrawal data. USGS scientists plan to conduct geospatial analyses aimed at developing approaches for addressing societally relevant energy concerns.
Associated Websites:
https://www.usgs.gov/software/energysim-r-package-spatial, accessed September 30, 2022
Associated Publications:
Haines, S.S., Diffendorfer, J., Balistrieri, L.S., Berger, B.R., Cook, T.A., Gautier, D.L., Gallegos, T.J., Gerritsen, M., Graffy, E., Hawkins, S., Johnson, K., Macknick, J., McMahon, P., Modde, T., Pierce, B., Schuenemeyer, J.H., Semmens, D.J., Simon, B., Taylor, J., Walton-Day, K., 2013, A framework for quantitative assessment of impacts related to energy and mineral resource development: Natural Resources Research, v. 23, no. 1, p. 3–17, accessed September 30, 2022, at https://doi.org/10.1007/s11053-013-9208-6.
Haines, S.S., Varela, B.A., Hawkins, S.J., Gianoutsos, N.J., Thamke, J.N., Engle, M.A., Tennyson, M.E., Schenk, C.J., Gaswirth, S.B., Marra, K.R., Kinney, S.A., Mercier, T.J., Martinez, C.D., 2017, Assessment of water and proppant quantities associated with petroleum production from the Bakken and Three Forks Formations, Williston Basin Province, Montana and North Dakota, 2016: U.S. Geological Survey Fact Sheet 2017–3044, 4 p., accessed September 30, 2022, at https://doi.org/10.3133/fs20173044.
Martinez, C.D., Preston, T.M., 2018, Oil and gas development footprint in the Piceance Basin, western Colorado: Science of the Total Environment, v. 616–617, p. 355–362, accessed September 30, 2022, at https://doi.org/10.1016/j.scitotenv.2017.10.280.
Keywords: surface and groundwater, land surface characteristics, geology, adaptive management, natural resource extraction
Evaluating the Impacts of Climate Change, Drought, and Irrigated Agriculture in the Colorado River Basin Using SPARROW
Principal Investigator: Olivia Miller (omiller@usgs.gov), U.S. Geological Survey, Utah Water Science Center, Salt Lake City, Utah
Project Description: The southwestern United States relies on the Colorado River to sustain its ecosystems, communities, and economies. Climate change and drought threaten water supplies while salinity in the Colorado River Basin causes an estimated $300 to $400 million per year in economic damages. In light of these challenges, we present two projects focused on (1) bracketing potential future streamflow under climate change and drought, and (2) understanding temporal variation in salinity loading from irrigated agricultural lands in the Colorado River Basin. For the first project, we estimate future streamflow in seven water resource regions in the Southwest using a new SPAtially Referenced Regressions on Watershed Attributes (SPARROW) streamflow model. We present streamflow projections corresponding to input data from seven climate models and two greenhouse gas concentration pathways (Representative Concentration Pathway [RCP] 4.5 and RCP8.5) for three 30-year intervals centered on the 2030s, 2050s, and 2080s, and for a historical 30-year interval centered on the 1990s. Results indicate widespread changes in streamflow, with 51 percent of RCP4.5 models and 67 percent of RCP8.5 models indicating decreases in streamflow by the 2080s. Among these models, our results indicate the risk of 35–80 percent decreases in all water resource regions for the 2080s relative to a historical 30-year interval centered on the 1990s, and streamflow decreases of up to 20–45 percent at sites along the Colorado River used for measuring compliance with interstate and international water agreements. For the second project, we plan to estimate loads of dissolved solids over time using a temporally dynamic SPARROW model we are developing. We also highlight relevance and applicability of SPARROW modeling in integrated science.
Associated Publications:
Miller, O.L., Putman, A.L., Alder, J., Miller, M., Jones, D.K., and Wise, D.R., 2021, Changing climate drives future streamflow declines and challenges in meeting water demand across the southwestern United States: Journal of Hydrology X, v. 11, p. 100074, accessed September 30, 2022, at https://doi.org/10.1016/j.hydroa.2021.100074.
Associated Data:
Putman, A.L., and Miller, O.L., 2021, Historical and projected streamflow for the southwestern United States: U.S. Geological Survey data release, accessed July 15, 2022, at https://doi.org/10.5066/P9J0J1I5.
Stakeholders: States, Federal agencies, local water-resources managers, the general public, academics
Keywords: climate change, salinity, streamflow, water quality, water resources
Evaluating the Effect of Streamflow on Temperature in the Lower Virgin River, near Mesquite, Nevada
Principal Investigator: Katherine Earp (kjearp@usgs.gov), U.S. Geological Survey, Nevada Water Science Center, Henderson, Nevada
Project Description: The Virgin River hosts a unique assemblage of native fish species, several of which have seen significant reductions in population sizes in the last few decades. Two of the six native fish species, Gila seminuda (Virgin River chub) and Plagopterus argentissimus (woundfin), are listed as endangered under the Endangered Species Act. Reductions in native fish populations across the arid southwestern United States have been attributed to agricultural, municipal, and industrial water-use projects, which often result in reduced streamflow, among other issues. Stakeholders consider changing the timing and number of diversions from the lower Virgin River as a promising strategy for improving habitat for native fishes. Aside from physical loss of habitat, reduced streamflow (especially during summer low-flow conditions) often results in higher stream temperatures, which can increase mortality, reduce breeding, limit population connectivity, and favor nonnative species. Research has indicated in water temperatures above 28 °C, native Virgin River fish change their behavior and seek cooler thermal refuges; above 31 °C, the fish start to die. Continuous water temperature data from 2019 and 2020 indicate that both thermal-threshold temperatures were exceeded on many days throughout the summer and remain elevated above these thresholds for more than six hours each day. The preliminary dataset indicates that temperatures in the study reach are primarily correlated to air temperature and solar radiation, with little relationship to flow except in outlier cases (floods and extremely low flow). The quantitative relation developed during this study between streamflow and temperature will help wildlife and land management agencies assess the feasibility of proposed fish restoration activities to improve river temperatures for native and endangered fish species during critical summer months.
Stakeholders: Bureau of Land Management, Nevada Department of Wildlife, U.S. Fish and Wildlife Service, the Virgin River Coalition, other Virgin River stakeholders
Keywords: water temperature, endangered fish, streamflow, water resources
Smart Energy Development
Principal Investigator: Mike Duniway (mduniway@usgs.gov), U.S. Geological Survey, Southwest Biological Science Center, Moab, Utah
Project Description: “By providing a public-facing portal, Smart Energy allows all stakeholders to access the same, definitive, and current spatial data, thereby facilitating informed decision making. The Smart Energy web tools aggregate a variety of biophysical data layers relevant to energy development, including information on the following topics:
-
• Energy resources
-
• Environmental resources
-
• Surface land management and ownership
-
• Land treatment and fire history
-
• Soils and topography
-
• Climate and weather
-
• Transportation
-
• Landcover and vegetation
-
• Recreation and visual resources
Smart Energy analysis tools allow user to select and explore data layers, define a specific area of interest, and generate a summary report of data layers in their area of interest” (U.S. Geological Survey, 2017).
Through collaboration with the Colorado River Basin Actionable and Strategic Integrated Science and Technology (ASIST) project team, the project is facilitating the addition of a Colorado River Basin-specific set of high-resolution geospatial data to facilitate decisions on energy management in the region.
Associated Websites:
https://sciencebase.usgs.gov/smartenergy/, accessed September 30, 2022
Stakeholders: Bureau of Land Management, National Park Service, U.S. Department of Agriculture Forest Service
Keywords: Oil resources, natural gas resources, wind energy, energy resources, soil resources, natural resource management, adaptive management, decision support methods, wildlife, habitat fragmentation
Forecasting Responses of Federally Listed Fishes in the Colorado River Basin to Water Storage Decisions
Principal Investigator: Charles Yackulic (cyackulic@usgs.gov), U.S. Geological Survey, Southwest Biological Science Center, Flagstaff, Ariz.
Project description: There are currently four endangered fish species within the Colorado River Basin. The survival and recovery of these species is dependent upon the amount and timing of streamflow that occurs, water temperature, the availability of suitable habitat, and predation by nonnative fish. All of these factors are affected by decisions that determine how water is stored within, and withdrawn from, Colorado River Basin reservoirs. Changes in the streamflow and temperature of dam releases directly affect the growth and reproduction of the endangered fish as well as nonnative fish that predate upon the native species. Thus, complex feedbacks exist among water supply and storage decisions, the duration and magnitude of flows, water temperature, and interactions between native and nonnative fish.
By 2026, State and Federal agencies are required to negotiate new water-supply agreements that will govern water supply and storage decisions in the Colorado River Basin through 2046. The outcome of these negotiations will have important implications for river ecosystems and the recovery of endangered fish. The focus of this work is on incorporating models of native and nonnative fish dynamics, water temperature, and streamflow, in order to forecast responses of two endangered fish species (Ptychocheilus lucius [Colorado pikeminnow] and Xyrauchen texanus [Razorback sucker]), and three nonnative predatory fish species (Micropterus dolomieu [smallmouth bass], Cyprinella lutrensis [red shiner], and Esox lucius [northern pike]) to changes in reservoir management. These predictive models will be used to build user-friendly web applications to explore different water-storage scenarios, and to share information and results with stakeholders within the Colorado River Basin.
Associated Publications:
Dibble, K.L., Yackulic, C.B., Kennedy, T.A., Bestgen, K.R., and Schmidt, J.C., 2021, Water storage decisions will determine the distribution and persistence of imperiled river fishes: Ecological Applications, v. 31, no. 2, e02279, accessed September 30, 2022, at https://doi.org/10.1002/eap.2279.
Wheeler, K., Kuhn, E., Bruckerhoff, L., Udall, B., Wang, J., Gilbert, L., Goeking, S., Kasprak, A., Mihalevich, B., Neilson, B., Salehabadi, H., and Schmidt, J.C., 2021, Alternative management paradigms for the future of the Colorado and Green Rivers: Center for Colorado River Studies White Paper no. 6, 132 p., accessed July 15, 2022, at https://www.fs.usda.gov/treesearch/pubs/63176.
Associated Data:
Dibble, K.L., C.B. Yackulic, and Bestgen, K.R., 2020, Water temperature models, data and code for the Colorado, Green, San Juan, Yampa, and White rivers in the Colorado River basin: U.S. Geological Survey data release, accessed September 30, 2022, at https://doi.org/10.5066/P9HFKV7Q.
Stakeholders: National Park Service, Bureau of Reclamation, U.S. Fish and Wildlife Service
Keywords: fauna, surface water, groundwater, population dynamics, aquatic ecosystems, droughts, biogeography, endangered species, wildlife, fish, streamflow, water quality, natural resource management
Postfire Tree Regeneration in Dry Forests—The Impact of Climate Change and Restoration
Principal Investigators: John Bradford (jbradford@usgs.gov), U.S. Geological Survey, Southwest Biological Science Center, Flagstaff, Ariz.; Sasha Reed (screed@usgs.gov), U.S. Geological Survey, Southwest Biological Science Center, Moab, Utah
Project Description: This Colorado River Basin project is a modest expansion of two existing research projects examining the impact of wildfire, climate change, and forest restoration on the sustainability of pinyon-juniper forests in the southwestern United States. In particular, the work enhances field projects designed to understand what controls post-wildfire tree regeneration, a notoriously episodic process that is being affected by climate change. Pinyon-juniper woodlands are a dominant community type across the western United States and these systems are experiencing significant effects from increasing fire and from treatments designed to reduce the frequency and intensity of fire (for example, stand thinning). At the same time, wildfire occurrence and severity are on the rise and are expected to continue to rise in the face of climate change. Shifts in fire regime have numerous consequences for pinyon-juniper woodlands that comprise over a million acres of public lands and that provide critical cultural, economic, and environmental benefits. An associated decline in pinyon-juniper woodlands represents large challenges for land managers tasked with protecting, restoring, and facilitating the use of these vast landscapes. With this project, we plan to use water-balance modeling (using the SOILWAT2 model, https://github.com/DrylandEcology/SOILWAT2) to quantify detailed soil moisture conditions in the past and future, and we plan to leverage the data we are collecting in our burned and unburned field sites. We also plan to further support the data collection by our Ute Mountain Ute Tribal partners. Taken together, results will provide a predictive and scalable understanding of how wildfire, climate change, and forest restoration treatments are interacting to affect the soil moisture conditions that enable tree regeneration in dry forest ecosystems across the western United States and will offer innovative management options for facilitating pinyon-juniper recovery.
Associated Publications:
Hurteau, M.D., Bradford, J.B., Fulé, P.Z., Taylor, A.H. and Martin, K.L., 2014, Climate change, fire management, and ecological services in the southwestern US: Forest Ecology and Management, v. 327, p. 280–289, accessed September 30, 2022, at https://doi.org/10.1016/j.foreco.2013.08.007.
Bradford, J.B., Schlaepfer, D.R., and Lauenroth, W.K., 2014, Ecohydrology of adjacent sagebrush and lodgepole pine ecosystems—The consequences of climate change and disturbance: Ecosystems, v. 17, no. 4, p. 590–605, accessed September 30, 2022, at https://doi.org/10.1007/s10021-013-9745-1.
McCann, E., Reed, S.C., Saud, P., Reibold, R.H., Howell, A. and Faist, A.M., 2021, Plant growth and biocrust-fire interactions across five North American deserts: Geoderma, v. 401, p. 115325, accessed September 30, 2022, at https://doi.org/10.1016/j.geoderma.2021.115325.
Stakeholders: National Park Service, Ute Mountain Ute Tribe, Bureau of Indian Affairs, Bureau of Land Management, U.S. Fish and Wildlife Service.
Keywords: fires, climate change, natural resource management co-produced science, mathematical modeling, remediation
Predicting Water Quality and Environmental Health in Rivers of the Colorado River Basin Affected by Wildfire
Principal Investigator: Rebecca Frus (rfrus@usgs.gov), U.S. Geological Survey, Nevada Water Science Center, Henderson, Nev.
Project Description: Water quality in streams and rivers can be affected by runoff from burned areas. Pulses of fire residue, such as ash to surface waters, can create large sags in dissolved oxygen levels and pH, as well as extreme highs in turbidity and specific conductance, which can lead to significant dieoffs in aquatic life. Understanding how different chemical reactions from burned materials can produce dissolved oxygen sags is a needed first step in developing a predictive tool of stream water-quality change that will help Federal, State, and local resource managers preserve protected or endangered species, maintain valuable technological resources, and provide early warnings to water treatment facilities.
Stakeholders: U.S. Army Corps of Engineers, U.S. Geological Survey, University of New Mexico, U.S. Geological Survey California Water Science Center, Arizona Water Science Center, Western Ecological Research Center
Keywords: fires, ecosystems, habitats, hazards, water quality, water resources
Rapid Assessment of Post-Fire Emergency Stabilization and Rehabilitation Effectiveness
Principal Investigators: Mike Duniway (mduniway@usgs.gov), Travis Nauman (tnauman@usgs.gov), and Brandon McNellis (bmcnellis@usgs.gov), U.S. Geological Survey, Southwest Biological Science Center, Moab, Utah
Project Description: Scientists at the U.S. Geological Survey Southwest Biological Science Center have been developing new approaches to evaluate the effectiveness of management activities designed to improve soil stability and reduce weeds after wildfire. This approach utilizes new satellite data, new land-cover products, and new soil maps to conduct land-cover change and trend estimates due to the fires or other disturbances and subsequent restoration actions. Our approach explicitly controls for the condition of land before disturbance as well as confounding climate effects (for example, long periods of drought). We have implemented this approach to evaluate effectiveness of oil or gas pad reclamation, recovery from historical livestock grazing, woody shrub removal, and fuel reduction treatments. In this study, we are applying these tools to assess post-burn conditions and recovery effectiveness on the Colorado Plateau. The Bureau of Land Management Emergency Stabilization and Rehabilitation (ES&R) and similar programs at the U.S. Department of Agriculture Forest Service and other Federal agencies seek to limit accelerated erosion and restore a stable and functioning ecosystem. However, robust information on the success of various ES&R treatments is often lacking, limiting the ability of land management agencies and scientists to learn and adapt management approaches. If our approach is successful in assessing post-wildfire trajectories on the Colorado Plateau (where the necessary soil and terrain data already exist), we will seek to expand the tool functionality to work in the rest of the southwestern United States, including California and other states that have been severely affected by increasing wildfires in the past decade.
Associated Websites:
https://www.blm.gov/sites/blm.gov/files/docs/2021-02/WDD%20Fire%20Fuels%20and%20Rehab%20Fact%20Sheet_Final.pdf, accessed September 30, 2022
https://www.doi.gov/sites/doi.gov/files/uploads/chapter_3_preparedness.pdf, accessed September 30, 2022
Associated Publications:
Di Stéfano, S., Karl, J.W., Bailey, D.W., and S. Hale, 2020, Evaluation of the automated reference toolset as a method to select reference plots for oil and gas reclamation on Colorado Plateau rangelands: Journal of Environmental Management, v. 265, p. 110578, accessed September 30, 2022, at https://doi.org/10.1016/j.jenvman.2020.110578.
Fick, S.E., Nauman, T.W., Brungard, C.C., and M. C. Duniway, 2021, Evaluating natural experiments in ecology—Using synthetic controls in assessments of remotely sensed land‐treatments: Ecological Applications, v. 31, no. 3, p. e02264, accessed September 30, 2022, at https://doi.org/10.1002/eap.2264.
Nauman, T.W., and M.C. Duniway, 2016, The Automated Reference Toolset—A soil-geomorphic ecological potential matching algorithm: Soil Science Society of America Journal, v. 80, no. 5, p. 1317–1328, accessed September 30, 2022, at https://doi.org/10.2136/sssaj2016.05.0151.
Nauman, T.W., Duniway, M.C., Villarreal, M.L., and T.B. Poitras, 2017, Disturbance automated reference toolset (DART)—Assessing patterns in ecological recovery from energy development on the Colorado Plateau: Science of The Total Environment, v. 584, p. 476–488, accessed September 30, 2022, at https://doi.org/10.1016/j.scitotenv.2017.01.034.
Stakeholders: Bureau of Land Management, U.S. Department of Agriculture Forest Service, other State and Federal land management agencies, tribes, grazing and livestock managers, conservation organizations, landowners
Keywords: fires, hazards, erosion, water quality, landslides
Rocky Mountain Snow Cover—Developing a Landsat-Derived Winter Forest Canopy Layer to Improve Remote Sensing and Modeling of Snow Cover in the Rocky Mountains of Colorado
Principal Investigators: Dave Selkowitz (dselkowitz@usgs.gov), U.S. Geological Survey, Wyoming-Montana Water Science Center, Helena, Mont.; Graham Sexstone (sextone@usgs.gov), U.S. Geological Survey, Colorado Water Science Center, Denver, Colo.
Project Description: Effective reservoir management in basins where runoff is dominated by spring and summer snowmelt is often hindered by inaccurate estimates of patchy high-elevation snow-water equivalent (SWE). A forest canopy layer optimized for the montane and subalpine forests of the western United States that reflects winter canopy conditions has the potential to significantly increase the accuracy of modeled SWE. Improved accuracy for estimates of high-elevation stored SWE and runoff will allow managers to optimize reservoir operations (such as balancing supply and demand), provide information to flood forecasters to predict potentially hazardous events, and facilitate the management of aquatic species that require minimum flow volumes or specific stream temperatures.
This project is focused on modeling spatial and temporal variability for a specific land-surface property (snowpack) and is designed to be used for surface water (runoff) predictions. The gridded SWE outputs may be useful for modeling and predictions involving certain flora and fauna as well (for example, animal behavior and movement patterns are often closely related to snowpack conditions, and vegetation phenology is often tied to snowpack as well). Key inputs for the model come from atmospheric science (gridded meteorological data) and flora (vegetation type and forest canopy coverage).
A combination of higher spatial resolution physics-based modeling and remote sensing can more accurately estimate high-elevation SWE, improve snowmelt runoff forecasts for the Basin, and enable better reservoir management decisions. Both remote sensing and modeling of snowpack conditions depend on forest canopy maps that accurately reflect forest canopy conditions for each date in the modeled time series. For remote sensing of snow-covered areas, approaches developed to adjust the viewable snow cover fraction (such as the fraction of a pixel appearing to be snow covered when viewed from above) to reflect the fraction of snow-covered ground (a much more useful metric for hydrological modeling applications) require forest canopy fraction as an input. For physics-based snow modeling, forest canopy fraction is used as a key input for models that account for the amount of snow captured by forest canopies and then lost to sublimation. Forest canopy fraction is also a required input for modules that simulate wind conditions and the redistribution of snow cover by wind. Consequently, forest canopy fraction input layers that do not accurately describe forest canopy conditions as they existed at each model time step can lead to substantial errors in modeled SWE. This work provides a promising strategy to improve estimates of stored SWE in high-elevation basins.
Associated Websites:
https://www.usgs.gov/centers/eros/science/usgs-eros-archive-landsat-landsat-level-3-fractional-snow-covered-area-fsca?qt-science_center_objects=0#qt-s cience_center_objects, accessed September 30, 2022.
Associated Publications:
Sexstone, G.A., Clow, D.W., Fassnacht, S.R., Liston, G.E., Hiemstra, C.A., Knowles, J.F., and Penn, C.A., 2018, Snow Sublimation in Mountain Environments and Its Sensitivity to Forest Disturbance and Climate Warming: Water Resources Research, v. 54, no. 2, p. 1191–1211, accessed September 30, 2022, at https://doi.org/10.1002/2017WR021172.
Sexstone, G.A., Penn, C.A., Liston, G.E., Gleason, K.E., Moeser, C.D., and D.W. Clow, 2020, Spatial variability in seasonal snowpack trends across the Rio Grande headwaters (1984–2017): Journal of Hydrometeorology, v. 21, no. 11, p. 2713–2733, accessed September 30, 2022, at https://doi.org/10.1175/JHM-D-20-0077.1.
Stakeholders: Bureau of Reclamation, Northern Colorado Water Conservancy District
Keywords: snow and ice cover, remote sensing, prediction, hydrology, water resources
StreamStats for Nevada
Principal Investigators: Rose Medina (rlmedina@usgs.gov) and Justin Mayers (cjmayers@usgs.gov), U.S. Geological Survey, Nevada Water Science Center, Carson City, Nev.; Geoff Moret (gmoret@usgs.gov), Chris Morris (cmmorris@usgs.gov), Nancy Damar (nadamer@usgs.gov), and Meg Hederman (mhederman@usgs.gov), U.S. Geological Survey Nevada Water Science Center, Henderson, Nev.
Project Description: Streamflow statistics and basin characteristics are used by many stakeholders for water-use planning, management, and regulatory activities. Streamflow statistics in Nevada are either out of date (peak-flow frequencies were previously computed for data through 1986) or have never been estimated for Nevada as a whole. Additionally, the computation of basin characteristics requires specialized skills, is time consuming, and can lead to different results when different methodologies are used. The StreamStats web application allows users to quickly obtain streamflow statistics, basin characteristics, and descriptive information at U.S. Geological Survey (USGS) streamgage stations and at any user-selected ungaged site along a stream. The purpose of this project is to update selected streamflow statistics and basin characteristics for Nevada using a consistent and automated methodology provided by the USGS StreamStats web application.
Associated Websites:
StreamStats Tools: https://www.usgs.gov/mission-areas/water-resources/science/streamstats-streamflow-statistics-and-spatial-analysis-tools, accessed September 30, 2022
StreamStats application: https://streamstats.usgs.gov/ss/, accessed September 30, 2022
Stakeholders: Bureau of Land Management, as well as other Federal, State, and local agencies
Keywords: web map services, geospatial datasets, surface water (nonmarine), scientific software, water resources
Reference Cited
U.S. Geological Survey, 2017, Smart Energy Development: Smart Energy Development website, accessed July 15, 2022, at https://sciencebase.usgs.gov/smartenergy/.
Appendix 3. Science and Collaboration Workshops
Conduct Integrated Science to Address Climate Change Impacts on Drought Conditions in the Colorado River Basin with a Focus on Past and Future Patterns
Crosscutting Stakeholder Science Needs: Resource management agencies, regulators, nongovernmental organizations, States, Tribes, local governments, and international partners in the Colorado River Basin have identified the need to evaluate historical and future-projected drought conditions across sectors to assess converging trends in models informed by global climate-model projections. Stakeholders are seeking science to improve understanding of uncertainty related to climate change impacts on drought conditions in the Basin. Stakeholders are focused on communication and categorization of uncertainty concerning drought adaptation planning to understand opportunities for reducing uncertainty.
USGS Science Question: How can the USGS leverage its diverse portfolio of predictive models to make drought predictions across time, space, and Earth science disciplines and better characterize uncertainty related to future drought conditions and impacts in the Colorado River Basin?
Evaluate Impacts of Drought at High Elevations in the Colorado River Basin, Including for Forest Health and Snowpack and as a Function of Landcover
Crosscutting Stakeholder Science Needs: Stakeholders across both the Upper and Lower Colorado River Basin recognize the critical hydrologic contribution from the more productive upper elevation headwaters. Improved high-elevation hydrologic monitoring is needed for local, regional, and basin-scale models used for water resource decision making. A pressing challenge is to reduce uncertainty and improve the quantification of hydrologic processes associated with snow accumulation, melt, and variability over time and as a function of land surface cover.
USGS Science Question: How can the USGS integrate the array of datasets and models within or relevant to the Colorado River Basin to better support analyses of high-elevation ecohydrological conditions?
Study the Nexus Between Groundwater-Dependent Streams, Ecosystems, and Research in the Colorado River Basin
Crosscutting Stakeholder Science Needs: Stakeholders across the Colorado River Basin are seeking science to monitor, assess, and predict the impacts of drought on groundwater-dependent streams and ecosystems, including the effects of drought on wildlife habitats, agriculture, and drinking-water availability. Integrated science on spring systems can use hydrology, ecology, geology, and land-use data to understand how changes to spring discharge coupled with a warming climate will impact spring ecosystems. This information will be used by stakeholders to prioritize remediation and reclamation efforts and reduce the impacts drought will have on refugia.
USGS Science Question: How can the USGS leverage current and historical work on groundwater-dependent streams and ecosystems to develop basin-wide models that predict the impacts of drought, increased groundwater development, climate change, and other stressors on groundwater-dependent streams and ecosystems?
Predict Wildfire Risk and Post-Fire Impacts Due to Long-Term Drought in the Colorado River Basin
Crosscutting Stakeholder Science Needs: Year after year, communities in the western United States are made painfully aware of expensive and long-term economic and social impacts caused by wildfires, including the loss of life, livelihoods, and property. However, fire in wildlands is essential to reduce future wildfire risk, improve wildlife habitat, maintain or restore ecological resilience, and manage fire-adapted species in many ecosystems across the Basin. Finding a balance between wildfire benefits and risks requires information to support decision-making and evaluate management alternatives. A critical need is integrated predictive models for fire occurrence and severity and the potential for post-fire debris flows and changes in water quality and quantity.
USGS Science Questions: How will drought influence patterns of fire occurrence, fire severity, and post-fire vegetation response? What actions can decision makers and managers take before, during, and after fires to mitigate drought impacts on ecological, hydrological, and social systems? Which fire-science activities, datasets, and predictive models across all USGS disciplines are most ready for integration to support decision making?
Drought Prediction, Integrated Predictive Modeling, and Early Warning Indicators in the Colorado River Basin
Crosscutting Stakeholder Science Needs: The influence of drought dynamics on resources is recognized by stakeholders engaged in a broad array of systems, including hydrological, agricultural, and ecological (aquatic and terrestrial). Because many of these systems are inherently linked, predictive models that adopt an integrated approach and represent connections between meteorological, hydrological, and ecological processes will best characterize complex drought responses across this diverse region. In addition, integrated models will forecast drought impacts on diverse resources and thus generate relevant information for a broad suite of potential stakeholders and resource management needs.
USGS Science Question: How can short-term forecasts of drought dynamics be constructed, validated, and integrated into resource management to anticipate drought impacts on ecological and social systems?
Quantifying the Impacts of Drought on Human Systems and Development in the Colorado River Basin, Including for Grazing, Agriculture, Human and Environmental Health, and Energy and Mineral Development
Crosscutting Stakeholder Science Needs: Drought has far-reaching impacts on the human system within the Colorado River Basin (summarized in appendix 1), including: the availability of water for agriculture, energy, and cattle production on rangelands; production of dust that may affect public health; and the environmental health of public lands used for recreation and tourism. These phenomena may be further stressed by the rapid population growth of urban centers within the intermountain west and the desert southwest of the United States. Widespread challenges exist related to the effective management of resources, and an integrated scientific approach will be needed to address these complex drought-related issues.
USGS Science Question: How can land managers make informed decisions on where to support land use and predict the impacts of that development on the land, water, and socio-ecological resources?
Integrating Ecosystem Responses to Drought, Climate Change, and Other Drivers of Change in the Colorado River Basin to Evaluate Impacts to Species or Habitats
Crosscutting Stakeholder Science Needs: Changes in ecosystem processes typically result from multiple, interacting drivers that work over space and time. These changes, when coupled with drought and other aspects of climate change—including changes in precipitation frequency, intensity, duration, and seasonal characteristics—can lead to feedbacks that alter additional drivers of ecosystem change. Resource managers across the Colorado River Basin must proactively plan for ecosystem-wide responses to drought in riverine, riparian, and terrestrial environments. Stakeholders need predictive and scenario-based models that include direct drought effects, secondary feedback effects across aquatic and terrestrial environments, and a better understanding of how species and population-level responses vary across geographic and topographic features. Because species and land responses are intimately associated, resource managers need interdisciplinary science outcomes and products to guide proactive planning and mitigation that reduce current and foreseeable impacts.
USGS Science Questions: How do ecosystem responses vary between the direct and secondary effects of drought and cascading impacts on priority species and habitats? How can this knowledge be used to guide and support resource managers in proactive planning and mitigation to reduce vulnerabilities? Are there areas that we can identify as potential refugia for species under increasing drought? Which plants may be most vulnerable to long-term drought? How will drought impact different wildlife groups?
Conversion Factors
U.S. customary units to International System of Units
Multiply | By | To obtain |
---|---|---|
inch (in.) | 2.54 | centimeter (cm) |
foot (ft) | 0.3048 | meter (m) |
mile (mi) | 1.609 | kilometer (km) |
mile, nautical (nmi) | 1.852 | kilometer (km) |
yard (yd) | 0.9144 | meter (m) |
acre | 4,047 | square meter (m2) |
acre | 0.4047 | hectare (ha) |
acre | 0.004047 | square kilometer (km2) |
square mile (mi2) | 2.590 | square kilometer (km2) |
gallon (gal) | 0.003785 | cubic meter (m3) |
million gallons (Mgal) | 3,785 | cubic meter (m3) |
cubic foot (ft3) | 0.02832 | cubic meter (m3) |
cubic yard (yd3) | 0.7646 | cubic meter (m3) |
Temperature in degrees Fahrenheit (°F) may be converted to degrees Celsius (°C) as follows:
°C = (°F – 32) / 1.8.
Datum
Vertical coordinate information is referenced to the North American Vertical Datum of 1988 (NAVD 88).
Horizontal coordinate information is referenced to the North American Datum of 1983 (NAD 83).
Abbreviations
ASIST
Colorado River Basin Actionable and Strategic Integrated Science and Technology
BCM
Basin Characterization Model
DOI
Department of the Interior
Earth MRI
Earth Mapping Resources Initiative
NGO
nongovernmental organization
RMNP
Rocky Mountain National Park
SBSC
Southwest Biological Science Center
SWE
snow water equivalent
TDE
Terrestrial Drylands Ecology
USGS
U.S. Geological Survey
WUI
wildland urban interface
Disclaimers
Any use of trade, firm, or product names is for descriptive purposes only and does not imply endorsement by the U.S. Government.
Although this information product, for the most part, is in the public domain, it also may contain copyrighted materials as noted in the text. Permission to reproduce copyrighted items must be secured from the copyright owner.
Suggested Citation
Dahm, K., Hawbaker, T., Frus, R., Monroe, A., Bradford, J., Andrews, W., Torregrosa, A., Anderson, E., Dean, D., and Qi, S., 2023, Colorado River Basin Actionable and Strategic Integrated Science and Technology Project—Science strategy: U.S. Geological Survey Circular 1502, 57 p., https://doi.org/10.3133/cir1502.
ISSN: 2330-5703 (online)
Study Area
Publication type | Report |
---|---|
Publication Subtype | USGS Numbered Series |
Title | Colorado River Basin Actionable and Strategic Integrated Science and Technology Project—Science strategy |
Series title | Circular |
Series number | 1502 |
DOI | 10.3133/cir1502 |
Year Published | 2023 |
Language | English |
Publisher | U.S. Geological Survey |
Publisher location | Reston VA |
Contributing office(s) | Geosciences and Environmental Change Science Center |
Description | vi, 53 p. |
Country | Mexico, United States |
State | Arizona, California, Colorado, Nevada, New Mexico, Sonora, Utah, Wyoming |
Other Geospatial | Colorado River Basin |
Online Only (Y/N) | Y |