Interdisciplinary Science Approach for Harmful Algal Blooms (HABs) and Algal Toxins—A Strategic Science Vision for the U.S. Geological Survey
Links
- Document: Report (8.23 MB pdf) , HTML , XML
- Appendix: Appendix 1 (332 KB pdf)
- Download citation as: RIS | Dublin Core
Acknowledgments
This document is the result of input from many scientists across the U.S. Geological Survey engaged in work on harmful algal blooms. Many personnel provided photographs and helped with figures. Kathy E. Lee helped organize and guide the process, and thoughtful reviews were provided by Keith A. Loftin, Jill S. Baron, Sandra M. Eberts, Robert L. Joseph, Keith Bouma-Gregson, and Geoffrey S. Plumlee.
Executive Summary
Overview
Algal blooms in water, soils, dusts, and the environment have captured national attention because of concerns associated with exposure to algal toxins for humans and animals. Algal blooms naturally occur in all surface-water types and are important primary producers for aquatic ecosystems. However, excessive algae growth can be associated with many harmful effects ranging from aesthetic to toxicity concerns, so this excessive growth is commonly called a harmful algal bloom (HAB).
Ecological imbalances that can lead to excessive algal growth, such as increased nutrient availability to waterbodies from natural and anthropogenic sources, are well documented in scientific literature. On the other hand, fundamental scientific understandings of environmental causes and controls leading to algal toxin production, environmental exposures, and adverse health outcomes for humans and animals could benefit from more attention by U.S. Geological Survey (USGS) scientists. Understanding when, why, and how the toxin is produced by individual algal cells or communities and why the toxin is released to the surrounding waterbody requires fundamental research to determine a toxin’s role, whether it provides competitive advantage or if other potential reasons exist for toxin production and release, such as secretions from otherwise benign biological processes. This research will require groundbreaking scientific discovery about underlying biologic and abiotic (non-living) processes commonly complicated by local variation in land use, microbial species composition, and ecosystem structure of the surrounding watershed.
Although underlying processes by which HABs form may be similar from one waterbody to another, individual waterbodies may be controlled by local factors for HAB development and toxin production that are unique to the watershed. Consequently, many fundamental science gaps exist that prevent informed mitigation and prevention of toxic HAB events. There are also gaps in understanding local conditions that control algal growth unique to specific watersheds. Addressing these science gaps is needed to inform evidence-based decisions that protect human and animal health and that reduce recreational and socioeconomic losses.
This vision document identifies opportunities where USGS science can address critical gaps and presents a Bureau-wide vision for integrated harmful algae science (sidebar E1). It is organized based on the following questions that the USGS is poised to answer:
-
• What are the beneficial and detrimental functions associated with harmful algae or HABs?
-
• What are the natural and anthropogenic drivers of algal blooms and the degree of harm across various environmental gradients in surface waters?
-
• What are the fundamental processes that drive algal toxin production and release?
-
• How can the USGS provide the knowledge to support local, regional, and national early warning, prevention, and mitigation efforts?
-
• What are the USGS capabilities and tools needed to characterize algal blooms, toxin production, and exposure?
This document is intentionally designed for flexible future implementation of science, scoping up or down in proportion to resources, stakeholder priorities, and other considerations. The interdisciplinary science opportunities envisioned in this document can be designed to address HABs issues at local, regional, and national scales and at varying timeframes as stakeholders are engaged and their needs evolve. This vision document is intended to serve as a resource for USGS scientists who are prioritizing and planning research on HABs or the processes that make them harmful and intended to be useful for developing partnerships with other scientists, agencies, and stakeholders.
Vision for an Interdisciplinary Science Approach for Research on Harmful Algal Blooms and Algal Toxins
The U.S. Geological Survey strategic vision is to provide knowledge that supports fundamental and applied understanding of harmful algal bloom proliferation and toxin production through interdisciplinary research and monitoring approaches. Achieving this vision would provide natural resource and health protection stakeholders with critical information needed to minimize the effects of harmful algal blooms and the toxins they produce.
Fiscal Support
This vision document does not provide detailed funding guidance but rather identifies HAB- and algal toxin-related science opportunities that the USGS could address. These opportunities are divided into short term (1–3 years, using existing resources) and longer term (that would likely require additional resources).
USGS Interdisciplinary Science Capabilities and Opportunities
The USGS has world-class analytical capabilities, national groundwater- and surface-water quality monitoring networks, and remote observations of surface water from satellite imaging. The USGS also has long-standing partnerships with the drinking-water and wastewater industries, other Federal agencies, Native American Tribes, academia, and a range of local, regional, and national decision makers. The USGS workforce is rare, if not unique across Federal science agencies in its interdisciplinary makeup, including biologists, chemists, data scientists, ecologists, geographers, geologists, geochemists, hydrologists, numerical and process-based modelers, phycologists, physical scientists, social scientists, programmers, statisticians, and wildlife toxicologists, among others. Integrating science input from these disciplines is essential to understand HABs complexities. Integrated science across disciplines that is done with a nationally consistent framework, oversight of field and laboratory protocols and approaches, and consistent and long-term datasets are unique strengths of the USGS not common in academia, industry, or other Government agencies. These capabilities underpin opportunities for USGS to address current HAB science needs.
USGS scientists have contributed a wide range of capabilities to many research activities related to harmful algae (refer to app. 1). The authors of this document conducted a series of meetings, assembled literature, and reviewed the state of the science for each subtopic to determine the science gaps and opportunities. The science opportunities identified herein are integral to the strategic vision for USGS harmful algae science and represent high-priority areas of research for USGS scientists who are planning harmful algae research. The opportunities are intentionally independent of scale and scope; thus, interdisciplinary science activities aligned with these priorities can be designed to address individual stakeholder needs. The subtopics for the major scientific opportunities are summarized in table E1 and are detailed in individual sections of chapter 2. The integration of these topics as a collaborative opportunity for USGS science, dependent on available funding, is summarized in chapter 3.
Table E1.
Summary of scalable science opportunities for studying harmful algal blooms and algal toxins determined through literature review and gap analysis.Science opportunities that could be addressed by U.S. Geological Survey capabilities | Table |
---|---|
Beneficial functions and harmful effects of algae or blooms | Table 1 |
Natural and anthropogenic drivers of algal blooms | Table 2 |
Fundamental processes that drive toxin production and release | Table 3 |
Provide knowledge and models for prevention, early warning, and mitigation | Table 4 |
Advance capabilities for characterizing algal blooms, toxin production, and toxin exposures to humans and other organisms | Table 5 |
Chapter 1. Introduction
Excessive growth of algae in water bodies can be associated with many harmful effects ranging from aesthetic to toxicity concerns; thus, when algae growth is excessive, it is often called a harmful algal bloom (HAB). Harmful algal blooms (refer to sidebar 1 for definitions) have been identified in all surface-water types. These include natural water bodies such as ponds, streams, rivers, wetlands, lakes, reservoirs, and coastal and marine waters, as well as constructed water bodies such as canals or aqueducts. Algal blooms can produce toxins that cause mortality or illness of humans, pets, livestock, fish, and wildlife (National Science and Technology Council, 2017). Other possible harmful effects include hypoxia (depletion of dissolved oxygen in the water column), ecosystem-wide food web impairment, secondary poisoning through the consumption of fish or shellfish, taste-and-odor problems in drinking water, lost recreational opportunities, and declining property values.
Sidebar 1. Harmful Algal Blooms and Algal Toxins
The term “harmful algal bloom” (HAB) commonly refers to all unwanted algal blooms. The underlying generalizations implicit in the HAB phrase can be confusing. Blooms can include rapid reproduction of filamentous algae that degrade the aesthetics of a waterbody and cause no health hazard; excessive algal biomass that depletes oxygen upon decay and harms the health of oxygen-dependent aquatic organisms; and cyanobacteria, dinoflagellates, or diatoms that release health-threatening toxins. Thus, algal blooms may, or may not, release toxins to the environment. Therefore, effective and accurate communication requires clarity by calling out specific types of blooms along with the associated harm they may cause.
Definitions of HAB terms, as used in this vision document, are as follows:
Harmful The term harmful refers to the myriad of adverse effects that may be associated with excessive algal growth. These harmful effects may include clogged filters and increased oxidant demand in drinking-water treatment plants, aesthetic issues, fish mortality because of oxygen depletion, as well as animal illness or mortality or human illness caused by exposure to algal toxins. However, it is not always clear if or how much harm will be caused by a bloom until after the effects are observed and measured.
Algae The term algae is commonly used as an umbrella term for microscopic aquatic plants. As scientific understanding has progressed, the organisms traditionally called algae are currently (2024) recognized as a diverse collection of organisms. Herein, the term “algae” is used to describe an ecological grouping of unicellular or simple multicellular organisms, including cyanobacteria, diatoms, and dinoflagellates, which conduct oxygenic photosynthesis using chlorophyll a and are primary producers that serve as the base of aquatic food webs. This term includes free-floating phytoplankton and benthic algae attached to sediment or rocks. In the scientific context, true algae include eukaryotic organisms such as diatoms, dinoflagellates, euglenoids, and haptophytes distinguished separately from cyanobacteria. We have retained the umbrella term of “algae” (inclusive of cyanobacteria) for this vision document and specifically mention cyanobacteria when necessary.
Bloom Herein, the term “bloom” is used to describe excessive algal growth and accumulation.
Harmful algal bloom Herein, the term “harmful algal bloom” describes an algal bloom that could or has caused adverse effects in any waterbody, including freshwater and marine or coastal areas.
[Refer to the Glossary at the end of this document for definitions of other technical terms.]
The environmental factors that cause some types of HABs are generally understood, such as nutrient inputs from either natural or anthropogenic sources that promote excessive growth (Heisler and others, 2008; Glibert, 2017). In those situations, site-specific monitoring data, assessments, and decision tools can inform evidence-based, targeted mitigation and prevention. However, options to reduce legacy nutrients can be challenging and HABs can exist along a gradient of trophic or nutrient conditions (Carey and others, 2012; Cottingham and others, 2015; Glibert, 2017). Understanding HAB occurrences along multiple environmental gradients is essential for mitigation and prevention, and traditional approaches are insufficient for oligotrophic systems (those having low nutrients or productivity) where drivers are not well understood. Causal factors also remain poorly understood for the toxins produced and released by some HABs, and these factors are the focus of current ecological and environmental health research; consequently, effective, targeted prevention and mitigation remains elusive.
Clearly, as technological capabilities improve, as scientific knowledge and education advance, and as human and animal populations expand, contact with and recognition of algal blooms and their associated harmful effects are detected and reported more frequently (Hallegraeff and others, 2021). Localized studies (Trevino-Garrison and others, 2015) document quantifiable increases in HABs over the last three decades and regional changes in weather patterns may benefit many species of harmful cyanobacteria (Paerl and Huisman, 2009).
The ecological purpose of toxin production by algae remains unknown; toxin production may result from a benign physiological process. Geologic evidence suggests this phenomenon predated humans (Castle and Rodgers, 2009) and some researchers hypothesize that toxin production is not accidental but rather a defense mechanism or other process of adaptation to particular environments (Baselga-Cervera and others, 2015). The role humans have had on exacerbating the spread and increase of blooms and the potential harmful effects associated with exposure are not clear. Likewise, clarity is needed on actions that are available to prevent and mitigate harmful effects when they do occur. Socioeconomic losses remain unclear from a national perspective, although they are estimated to exceed several million U.S. dollars annually (Anderson and others, 2000; Bingham and others, 2015).
1.1. Existing Literature on HABs and Algal Toxins
The vision for USGS HAB science defined by this document is based upon a thorough review of the literature and assessment of the state of the science, which are well summarized in previously published reviews such as by Ramsdell and others, (2005), Hudnell (2008, 2010), Carmichael and Boyer (2016), Anderson and others (2021), and Chorus and Welker (2021). USGS studies have already contributed substantially to this body of knowledge about HABs (refer to app. 1). These studies illustrate the range of USGS capabilities and expertise that can be applied to tackle many other opportunities for USGS HAB science. The USGS has developed expertise in emerging areas of HAB research, resulting in numerous publications on the harmful effects and study design of HABs, algae, and algal toxins. Opportunities become evident where USGS expertise overlaps with existing gaps (for example, new research on the possible beneficial functions of HABs). Gaps are described in more detail in chapter 2, along with related opportunities uniquely suited for USGS research teams, based on capabilities and expertise.
1.2. Overarching Research Questions
Complex challenges create opportunities that require an integrated and interdisciplinary science approach involving multiple disciplines working together and in partnership with stakeholders, policymakers, and the public. The USGS has been involved with many studies documenting blooms and toxins in waterbodies from inland freshwaters to coastal and marine waters (Mize and Demcheck, 2009; Drake and others, 2010; Graham and others, 2010, 2012, 2018, 2020455049; Miller and others, 2012; Stelzer and others, 2013; Terrio and others, 2013; Loftin and others, 2016a, 2016b; Moorman and others, 2017; Rosen and others, 2017; Larson and others, 2018; Tatters and others, 2021; Zuellig and others, 2021). Human illness and animal illness and mortality occur across the freshwater to marine continuum with sufficient frequency that Federal agencies, States, and Tribes are reporting events to systems such as the One Health voluntary reporting system (Roberts and others, 2020) at https://www.cdc.gov/habs/ohhabs.html or the USGS National Wildlife Health Center’s Wildlife Health Information Sharing Partnership (WHISPers) at https://www.usgs.gov/centers/nwhc/science/whispers. In conjunction with other agencies, the USGS is poised to answer the following overarching questions to understand the magnitude and scope of effects of HABs and algal toxins on ecosystems and human health.
1.2.1. What Are the Beneficial and Detrimental Functions Associated With Harmful Algae or HABs?
Although most emphasis is placed on the harmful consequences of blooms, algae have clear benefits, including beneficial uses for toxin-producing algae (for example, anticarcinogenic effects; Zanchett and Oliveira-Filho, 2013), cyanobacteria (Demay and others, 2019), or accumulations (for example, sequestration of microplastics; Peller and others, 2021), and reduction of mercury in fish and zooplankton (Pickhardt and others, 2002). Algae and cyanobacteria living in the Earth’s surface waters are primary producers that provide atmospheric oxygen and fix carbon, which reduces carbon dioxide in the atmosphere. Aquatic food webs are supported by algal primary production, including some taxa that form blooms; thus, algae support healthy fisheries and other ecosystem services. Although evidence suggests that cyanobacteria are less nutritious than green algae (Zhang and others, 2020), even toxin-producing algae provide food and produce oxygen. In terrestrial environments, cyanobacteria crusts add moisture and organic matter to rebuild soil horizons (Ling and others, 2022), reversing the process of land transitioning into desert by adding carbon and moisture. Some cyanobacteria can fix atmospheric nitrogen to augment fertilizer requirements for crops like soybeans, other legumes, and rice (Sanyal and others, 2022), and can help reduce the need for nitrogen amendment in future crop rotations. Rarely does mitigation only affect harmful algae; therefore, mitigation of harm should be balanced with the benefits provided by algae and cyanobacteria.
The USGS continues to use an interdisciplinary science approach to document when and if blooms lead to harm, particularly for blooms that threaten human and animal health. Understanding the balance between benefits and harmful effects of blooms is critical to defining better management practices to sustain natural resources. Food-web bloom dynamics and ecotoxicological studies of toxin exposure that examine potential human and ecological health effects should consider organism life stages, preferred nutrition, habits, habitats, sustainable population levels, weather patterns, resource availability for intended uses, and other factors.
1.2.2. What Are the Natural and Anthropogenic Drivers of Algal Blooms and the Degree of Harm Across Various Environmental Gradients in Surface Waters?
Variations among waterbodies present a challenge for a universal approach to address harmful algae. The scientific community generally agrees that nutrients are a primary factor affecting bloom occurrence and growth. However, theories citing the importance of nutrients are changing (Glibert, 2017) and challenging long-held paradigms of bloom occurrence. Blooms can occur in nutrient-poor surface waters (Cottingham and others, 2015), suggesting that understanding vertical mobility of algae and bloom occurrence along the trophic gradient is essential for mitigation and prevention. Benthic blooms may commonly occur in clear, nutrient-poor systems because of nutrient influx from groundwater, lake hydrodynamics, or food web structure (Vadeboncoeur and others, 2021). Additionally, water temperatures are a key factor, and interactions between multiple factors such as nutrients and increasing water temperatures have been reported as having a synergistic effect (Havens and Paerl, 2015).
The USGS can address this problem through interdisciplinary science to help understand multiple drivers. Although nutrients and temperature may account for substantial variation in biomass production, they may not account for the complexity of co-occurring biological processes, such as successional changes amongst the microbial community, which vary substantially among watersheds and coastal systems. Many other factors, such as the continuum between fresh and saline water, variations in ecosystems, geology, sediment type, weather patterns, and anthropogenic factors among watersheds, may result in unique controls on HAB development in individual waterbodies and shorelines. With interdisciplinary research required to understand the influence of all these processes, USGS is in a unique position to advance HAB science.
1.2.3. What Are the Fundamental Processes that Drive Algal Toxin Production and Release?
Drivers of toxin production remain poorly understood for the biosynthesis of toxins, and scientists do not have the same level of foundational knowledge to build upon as they have for algal biomass production. Moreover, multiple understudied toxin classes and hundreds of toxin variants within some classes exist (for detailed lists, refer to Meriluoto and others, 2017), and each variant may have different drivers. Some algal toxin producing species are found more frequently in certain types of waters, for example saline or brackish (Patiño and others, 2023; Buratti and others, 2017; Van Dolah, 2000). The presence of species known to produce various toxin classes does not automatically indicate toxin production will occur. More research is needed to understand how, why, and when some blooms are associated with toxin production and release, whereas others are not. More work is also needed to determine whether cyanobacteria in soil crusts can produce toxins and if so, which toxins they produce, how they are released, their potential exposure pathways (including transport through wind or dust; Massey and others, 2018), and toxicological impacts on humans and other terrestrial organisms.
The USGS can address the multifaceted issue of the drivers and controls leading to algal toxin production and release through interdisciplinary collaboration. Input from scientists from many disciplines will be key to solving this complex problem that sits at the nexus of constraints in knowledge, scientific observations, sufficient experimental control, complete system characterization, and technological limitations. An improved understanding of the drivers of toxin production and release will require consistent approaches and multiyear experiments. The USGS is particularly suited to undertake long-term studies. USGS scientists are working on long-term laboratory to field scale studies evaluating multiple drivers and interactions with biological, chemical, and physical factors. Short-term studies cannot capture the full range of environmental variability that can affect toxin production (changes in weather patterns, water management, water quality, land use, and land cover) or effects of treatment practices for bloom and toxin mitigation. An interdisciplinary science approach will be critical to understanding algal toxin drivers, leading toward more reliable predictive capabilities and clarifying management options.
1.2.4. How Can We Provide the Knowledge and Models to Support Local, Regional, and National Early Warning, Prevention, and Mitigation Efforts?
Understanding the drivers of HAB formation and toxin generation is likely the best way to determine successful, time-appropriate management solutions. Fully implementing strategies for nutrient reduction in water bodies is a challenge. Best management practices combined with natural attenuation may not reduce nutrients to an acceptable level for decades, even if no new nutrients are added. The urgency for a solution to the harmful effects is commonly demanded within days of a potentially harmful bloom event, but this timescale is incongruent with nutrient management strategies. Solutions are needed that fit the urgency of the problem in real time until long-term solutions start to have an effect. Defining what constitutes an unacceptable harm, determining where and when a bloom will occur, and identifying the environmental indicators and thresholds that can help define an unacceptable change (for example changes in bloom size, duration, location, or toxicity) are critical to identify and prioritize actions that best minimize risks to quality of life, property, and the economy.
USGS scientists can address this issue by integrating knowledge of HAB drivers with our understanding of toxin production. An understanding of the bloom ecology is needed to model individual systems, which will require scientists from multiple disciplines, and collaboration inside and outside the agency (for example, using the National Ecological Observing Network; National Science Foundation, 2019). This interdisciplinary approach puts the USGS in a position to help collaborators in prevention, early warning, and mitigation of harmful events. For example, the USGS has long-term datasets, as well as the technological capability of providing data on drivers in real time, and thus is well suited to provide improved short-term forecasts needed by drinking-water purveyors so that they can protect their intakes from a bloom.
1.2.5. What Capabilities and Tools Do We Need to Characterize Algal Blooms, Toxin Production, and Exposure?
To effectively assess blooms and their associated harmful effects, additional advancements in ground-to-satellite methods are necessary to provide measurements that can help evaluate trends through time or geographic space. To evaluate the intersection of occurrence and health outcomes, the USGS already has developed a variety of methods covering algal bloom assessment, toxin and taste-and-odor compound occurrence, and biological assessments over short periods (for example, Francy and others, 2015; Graham and others, 2016; Christensen and others, 2021). To have sufficient statistical rigor, trends analysis requires assessment across decadal timescales to integrate the multitude of changes that may affect trends. More long-term datasets are needed for trend analysis, and the scale of effort would have to match the scale of the question (local, regional, national, or global).
The scientists at the USGS are poised to scope the duration, magnitude, and frequency of algal blooms and associated harmful effects through an interdisciplinary science approach. The USGS has a rich history in trends analysis using validated, robust methods to understand changes to the Earth and its processes, and is uniquely positioned for long-term data collection. The USGS is positioned through continued advancement in these methods when coupled with refinement in experimental approaches that uncover the factors controlling algal blooms and their associated harmful effects.
Chapter 2. Data Gaps, Capabilities and Expertise, and Opportunities for Algal Bloom and Algal Toxin Monitoring and Research Activities
2.1. Weighing the Beneficial Functions of Algae or Blooms Against the Harmful Effects Caused by HABs and Their Toxins
Vision.—Scientists should be able to balance the complex benefits of algal blooms or individual algal species against known harmful effects so that resource managers can make informed water-resource decisions that consider HAB effects on ecosystems, humans, animals, agriculture, and socioeconomics.
2.1.1. Science Gaps
In many cases, algae are beneficial for ecosystems because they are primary producers that form the foundation of aquatic food webs. Even harmful algae can be considered primary producers (Demoulin and others, 2019) and contribute to ecosystems in many ways. Although much research details the benefit of algae, and new research on the benefits of algal toxins and other secondary metabolites is emerging (for example, cancer-fighting properties, Zanchett and Oliveira-Filho, 2013; Ferdous and Yusof, 2021), evidence and documentation of the often-overlooked beneficial aspects of algal blooms is needed. These aspects may include quantifying oxygen production (Rai and others, 2000), exploring the potential for bioenergy applications (Blank and Sánchez-Baracaldo, 2010), and documenting the role of spring algal blooms in driving aquatic food webs. For example, documenting the role of spring algal blooms in freshwater and marine ecosystems may be important in some ecosystems where the absence of a spring bloom could lead to insufficient energy resources for zooplankton and planktivorous young-of-the-year fish, potentially causing food webs to collapse.
Likewise, although the scientific community increasingly regards the relation between eutrophication and HABs as complicated (for example, Glibert, 2017; Heisler and others, 2008), communication about environmental and societal effects of excessive algal growth could be improved with a consistent and universal definition of a “harmful algal bloom” (HAB) with specific conditions that define when an algal bloom is harmful. An example is the recent framework suggested by USGS scientists (Gorney and others, 2023). Harmful effects from algal blooms can be viewed in terms of hazards and risks and incorporating an assessment of risk into a consistent definition is warranted (fig. 1). According to Holmes and others (2013, p. 57–58), the USGS defines hazard, risk, and exposure as follows:
-
• A hazard is “a dangerous process, phenomenon, substance, activity, or condition that may cause loss of life, injury, or other health impacts, property damage, loss of livelihoods and services, social and economic disruption, or environmental damage.”
-
• A risk is “a combination of the probability of a hazard occurring and the value of assets in harm’s way.”
-
• An exposure is “people, property, infrastructure, or other societal or valued natural elements that are present in hazard zones and, thus, subject to potential losses.” Simply put, a hazard poses no risk if assets are not exposed to the hazard.
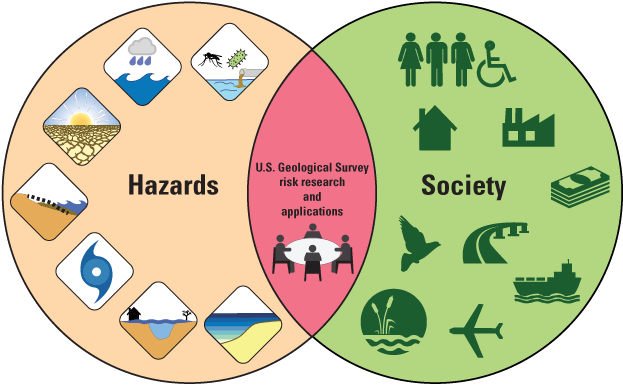
The relation between hazards, risk, and exposure.
Given those definitions, potential harmful effects could be better defined in terms of ecosystems, humans, animals, agriculture, or socioeconomics. Acute, chronic, lethal, and sublethal effects of algal toxins in fish and wildlife are poorly understood with few exceptions; moreover, for almost all algal toxins, gaps remain in understanding acute, subacute, and chronic toxicity. Far less is known about the long-term health effects from chronic exposures, exposures to toxin mixtures, or co-exposures with other chemicals.
Research on algal toxin exposures is ongoing, and several gaps in knowledge remain. For example, ingestion of animals, plants, or drinking water that contain toxins is a known route of exposure, but the frequency, magnitude, and health implications of this exposure route are not yet understood. Exposure to algal toxins through inhalation and through skin or mucous membranes is poorly understood. Although skin irritation has been observed in some studies, these observations do not address the potential for absorption of toxins through tissues (Nielsen and Jiang, 2020). Improving the scientific knowledge around toxin absorption, distribution, metabolism, and excretion would help to understand adverse outcomes.
2.1.2. USGS Capabilities and Expertise
The USGS continues to build capabilities to address the environmental and socioeconomic effects of excessive algal growth. The USGS has documented capabilities of aquatic primary productivity research (Godwin and others, 2014; Hall and others, 2015) and algae research in desert soils (Belnap, 2001). Studying benefits of algae related to biofuels would be an opportunity for interdisciplinary studies among USGS programs and mission areas with mineral resource and ecosystems capabilities.
In terms of harmful effects, the USGS has substantial expertise on algae-driven hypoxia (Battaglin and others, 2009; Sullivan and others, 2010) and there are opportunities for integration within the USGS on hypoxia effects on aquatic life, evaluating impaired food web dynamics, and acute (lethal and sublethal) toxicity to fish and wildlife, particularly with the laboratory capabilities at the USGS National Wildlife Health Center, USGS Columbia Environmental Research Center, and other USGS facilities.
The USGS also has developed tools to help managers select, implement, and evaluate natural resource management decisions (Alhassan and others, 2020) and is poised to use these tools to manage, mitigate, and reduce the effects of harmful algae (Pindilli and Loftin, 2022). One recent example of USGS capabilities (refer to sidebar 2) demonstrates how the USGS and collaborators provided a decision-making framework to the New York State Office of Parks, Recreation and Historic Preservation to help them make decisions about preventing and responding to blooms in lakes under their jurisdiction (Graham and others, 2022).
Sidebar 2. A Tool to Help Decisions for Managing Harmful Algal Blooms in New York State Parks
In 2020, the U.S. Geological Survey partnered with the New York State Office of Parks, Recreation and Historic Preservation and the New York State Department of Environmental Conservation to develop a structured decision-making template for cyanobacterial bloom management (fig. S1). Structured decision making is a systematic approach that breaks down complex decisions into elements and reconstructs the problem in a framework, allowing collaborative examination and development of suitable actions. The New York State Office of Parks, Recreation and Historic Preservation faces many unknowns and difficult decisions about preventing and responding to blooms in park lakes. Management goals and strategies for bloom mitigation were described, consequences were evaluated, tradeoffs were managed, and the potential challenges of implementing preferred alternatives were discussed. Two parks served as case studies to inform the decision-making process at other parks throughout New York. The process and findings are presented in Graham and others (2022).
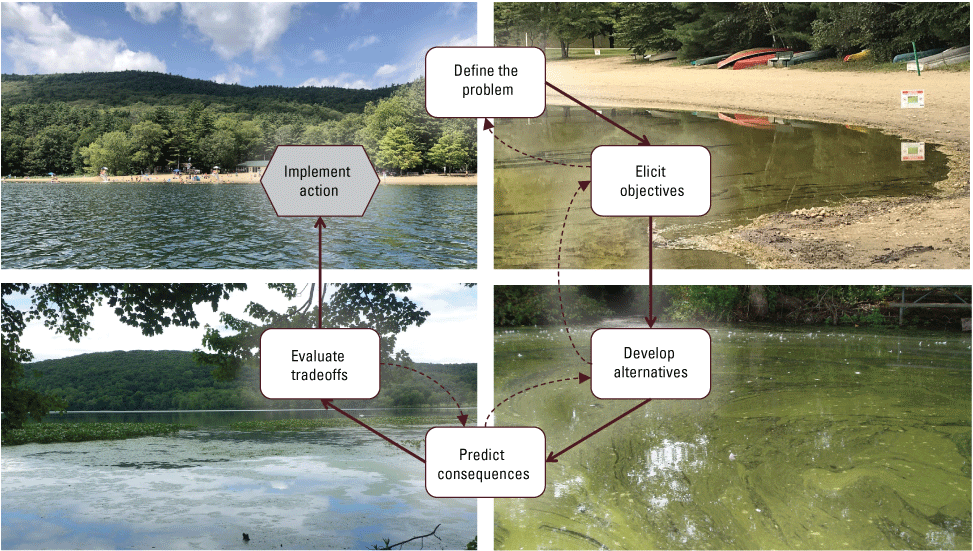
A structured decision-making template to provide knowledge for managing harmful algal blooms in New York State parks. Modified from Graham and others (2022).
2.1.3. USGS Short- and Long-Term Science Opportunities
Benefits.—The USGS could invest in projects that quantify the benefits of algae, particularly cyanobacteria and algae blooms (table 1). Evaluating HAB primary productivity requires consistent definitions and a consideration of tradeoffs between non-HAB primary productivity and HAB primary productivity. Long-term opportunities on the benefits of algae could include research in the areas of oxygen production, carbon dioxide consumption, promotion of biocrust formation through reverse desertification, agricultural soil amendments, and measurement of the potential benefits of algae used in select bioremediation applications. Additionally, exploring bioenergy applications provides an opportunity to integrate studies on algae and energy, such as exploring the use of algae as biofuel or the efficacy of using microbes to assist in oil extraction. Algae may be beneficial as food supplements or pharmaceuticals (Hassan and others, 2022), which could be studied in collaboration with other agencies, and developing a multitiered, interdisciplinary approach for bloom characterization would be helpful.
Table 1.
Scalable science opportunities to study the beneficial functions and harmful effects of algae or blooms.Harmful effects.—Certain harmful effects to ecosystems could be addressed by the USGS in the short term, such as documenting algae-driven hypoxia effects on specific aquatic species. The USGS could also improve toxin dosage methods in ecotoxicology studies (studies of substances that are harmful to the environment) and could develop and optimize bioaccessibility methods to prioritize potential health risks to toxin exposure. Longer-term opportunities related to ecological harmful effects may include integrating HAB studies with investigations on invasive species (including the interactions between multiple species) and how invasives affect food web dynamics and other aspects of the algal environment through direct consumption, food web alteration, altered nutrient cycling, and physical change (such as light or substrate). Food web studies could include determining whether HABs or their toxins cause population-level harm to consumers. Other long-term studies could measure acute, chronic, lethal, and sublethal toxicity to fish and wildlife or could develop and validate biometric and chemometric approaches to measure up and down regulation of toxin production, toxin exposure, and health outcome biomarkers in vertebrates. The development and optimization of bioaccessibility methods (simulated digestion, for example) could prioritize potential health risks to toxin exposure. Opportunities related to potential harm to humans and companion animals include developing studies of chronic, acute, lethal, and sublethal toxicity and examining secondary intoxication by consumption of drinking water (including the creation of disinfection byproducts; refer to sec. 2.4.3) and agricultural food products. The USGS could address some of the socioeconomic harmful effects on a smaller scale or case-study basis, similar to the recently completed evaluation of management costs for reservoirs in Kansas (Pindilli and Loftin, 2022). Long-term opportunities exist to expand socioeconomic research to other natural resources. Further long-term opportunities exist related to socioeconomic harmful effects including evaluating increased costs for drinking water treatment and agricultural production, estimating decreased tourism and tax revenues, identifying natural resource access limitations, studying the effects of impaired aesthetics, and evaluating decreased property values.
2.2. Drivers of Algal Blooms
Vision.—Scientists should understand the contribution of natural and anthropogenic bloom drivers for a range of surface-water types in response to stakeholder needs.
2.2.1. Science Gaps
Algal blooms are driven by a complex interplay of physical, chemical, and ecological factors (fig. 2). Algal bloom drivers can be any natural or anthropogenic factor that directly or indirectly triggers the initiation of a bloom, affects its duration, or changes its species composition. Although much work has been conducted to understand drivers of algal blooms, largely focusing on nutrients (for example, Day and Henneberg, 2023; Fraker and others, 2023), knowledge gaps remain for a multitude of environmental variables that can influence HABs. Testing multi-stressor effects at the population, community, or ecosystem level is exceedingly difficult. However, emerging research is showing that chemical mixtures and other environmental factors may be important drivers for aquatic ecosystem health (Maity and others, 2021). Future analysis focused on sustained, or longer-term, effects of water-quality conditions on aquatic community structure and function may provide a better understanding of HABs and toxin production.
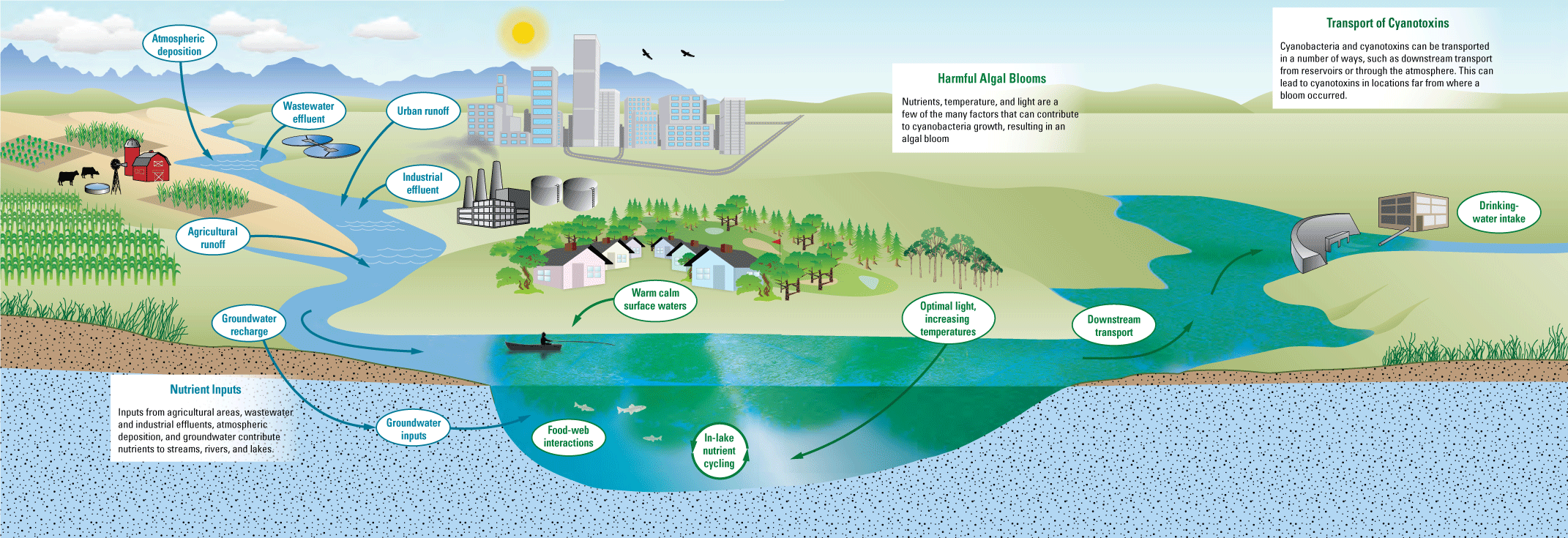
Harmful algal blooms and potential toxins may be driven by many factors, and the complex interplay of those factors is not easy to unravel. Modified from Graham and others (2016).
Additional research gaps include overwintering of key organisms, the significance of sediment as a source of nutrients and trace metals, ways in which aquatic system interconnectivity affects blooms, lag times between nutrient load changes and HAB response (whether within the same season or across years), and HABs in low-nutrient and remote systems. Furthermore, climate change may affect the timing and magnitude of nutrient delivery and alter the physical structure of the water column and thermal optima. Likewise, some nutrient sources (for example, atmospheric deposition) have been overlooked and relative contributions of current versus historical nutrient sources to HABs lack clarity. Mechanisms driving shifts in watershed nutrient retention for different land cover types also lack understanding. Finally, effects of natural resource management practices on land, water, and biota, and how they may or may not affect HABs, need clarifying. To date, few watershed monitoring studies have demonstrated improvements in water quality that could be attributed directly to the implementation of conservation practices, partially because of a lack of best management practice (BMP) information (García and others, 2016; Kreiling and others, 2020).
2.2.2. USGS Capabilities and Expertise
Major HAB drivers such as climate and nutrient inputs are being studied (Ryberg and Chanat, 2022; Burford and others, 2020; Zuellig and others, 2021) but alone cannot fully explain bloom conditions observed across the United States. The scale of study is critical, and USGS programs are well situated to integrate large-scale monitoring and assessment with focused smaller scale studies led by individual USGS science centers that can help address gaps in understanding of HAB environmental drivers. The USGS could develop and advance HAB science of small- to medium-size lakes and reservoirs that are interconnected through vast stream and river systems at a national scale.
The USGS measures, manages, and distributes a plethora of environmental data records (hydrological, aquatic, biological, and chemical) and a comprehensive suite of land change data products that could aid in quantifying the effects of land use pressure on hydrological and aquatic systems where HABs have been documented. The USGS Landsat program is now producing an operational remote sensing surface temperature product (https://www.usgs.gov/landsat-missions/landsat-collection-2-us-analysis-ready-data) at fine spatial scales that spans nearly four decades (Crawford and others, 2023). This product could serve as an unparalleled baseline for how more recent variability, change, and trends in warming surface temperatures is encoded in waterbodies that are either prone to or increasingly susceptible to HABs with toxin-production potential. Additionally, USGS has recently completed the Lake Trophic State–US (LTS–US) dataset for 55,662 lakes (Meyer and others, 2024), which couples satellite-based remote sensing with fundamental limnological principles to provide the means to identify macroscale patterns and trends at a national scale. The USGS real-time water quality network (https://waterwatch.usgs.gov/wqwatch/), with improved sensing capabilities particularly for toxins, could help determine trends in HAB-related data and support modeling and prediction efforts (refer to sec. 2.4). Developing systems or platforms for compiling and integrating these different data sources would better support scientific research on the complexities of HAB drivers.
Finally, USGS maintains sediment core repositories (Honey and Ivis, 2023) and has expertise determining water-quality trends from lake sediment cores (Juracek, 2015). The USGS has applied paleolimnological research to diatoms (Benson and others, 2021) and combining these areas of expertise could improve our understanding of toxin producing species or indicators of blooms in the sedimentary record before human influence.
2.2.3. USGS Short- and Long-Term Science Opportunities
The USGS could identify potential natural and anthropogenic factors related to HABs, including the roles of bottom sediment, internal loading, watershed inputs, atmospheric contributions, and predation on HAB initiation, proliferation, succession, and transport (table 2). These research efforts could include additional investigation of bottom sediment as a source of metals due to the requirement for metals in phytoplankton metabolic functions (Larson and others, 2023). USGS scientists also have an opportunity to investigate factors and determine the thresholds that control successional transition between different algae and cyanobacteria.
Further work is needed to understand the pathways and exposure routes of aerosolized algal toxins. Cyanobacteria play key roles in stabilizing arid soils by forming crusts composed of sands bound to polysaccharides that they secrete; these cyanobacteria also produce a variety of toxins that can be aerosolized if the crusts are physically disturbed (Richer and others, 2015). Algal toxins in dusts generated from disturbed cyanobacterial soil crusts have been postulated as the potential cause of sporadic amyotrophic lateral sclerosis among veterans of the Gulf War (Cox and others, 2009). Additionally, algal toxins from aerosolized red tides were suspected in the death of green tree frogs at Padre Island National Seashore (Buttke and others, 2018). Finally, the USGS is also beginning to study the effects of toxins and pathogens in waterfall mist (Krall and others, 2023).
Long-term monitoring also is required to characterize changes in HAB trends and understand the interplay of various drivers and best management practices to develop mitigation and control strategies. Further research on the drivers of HABs could be addressed with long-term monitoring to explain HAB trends and with experimental studies at various scales and across environmental gradients and water types (for example, from fresh to saline). Existing Earth-observing networks could be used to relate drivers of HABs to short-term HAB occurrence; however, new sensors resulting from a longer-term investment in these networks would be required to relate real-time parameters to bloom intensity, persistence, and toxin production.
Table 2.
Scalable science opportunities to study natural and anthropogenic drivers of algal blooms.[HAB, harmful algal bloom]
2.3. Drivers of Toxin Production and Release
Vision.—Scientists should have a fundamental understanding of the mechanisms of toxin production and release to provide resource managers with the information needed for the protection of wildlife, fish, domestic animals, and human health.
2.3.1. Science Gaps
The exact mechanisms and the natural and anthropogenic drivers of algal toxin production are poorly understood. Clearly differentiating processes that control HAB growth from processes that control toxin production is needed. Given the energetic cost of toxin production to algae, the toxin production likely serves a purpose in their survival, but this purpose remains a research gap. Moreover, determining the effects of infection by phages, fungi, and bacteria on toxin production may help scientists distinguish between infection that alters HAB succession from infection that may turn on, amplify, or decrease toxin production.
2.3.2. USGS Capabilities and Expertise
The environmental causes and controls leading to algal toxin production and release have been a subject of research of the USGS Ecosystems Mission Area and the Toxins and Harmful Algal Blooms Research Team. In collaboration with scientists inside and outside the USGS, this group has examined cyanotoxin and potential exposures across public lands (Laughrey and others, 2021), studied neurotoxins and rapid-detection methods (Christensen and Khan, 2020; LeDuc and others, 2020), and studied co-occurring algal toxins (Howard and others, 2021), which may help explain the mechanisms that cause toxin production and release.
USGS laboratories have a long history of large-scale studies and consistent analytical techniques that include documented experimental control, system characterization, and technical limitations (Romanok and others, 2018). In collaboration with external partners, this expertise has been applied to mesocosm studies (Harris and others, 2014), cyanobacteria in drinking water (Coffer and others, 2021), and documenting cell-lysis techniques (Rosen and others, 2010). These consistent approaches and multiyear experiments poise USGS scientists to address the full range of variability encountered in the environment that can affect toxin production. By integrating USGS laboratory expertise with the USGS Water Mission Area’s existing real-time sensors, researchers could develop the capabilities to study and measure onsite metagenomics and metatranscriptomics, along with existing statistical capabilities to identify key environmental drivers of toxin production.
2.3.3. USGS Short- and Long-Term Science Opportunities
The opportunities (table 3) related to toxin drivers are long term and include many areas that are understudied. Primarily, opportunities are available to differentiate between the factors that cause HABs and those that cause synthesis and release of toxins. Research that characterizes the biosynthesis pathways of toxin production may help determine why toxins are produced. Additionally, studies of allelopathy and biological responses to toxins released by other algae, and studies of the effects of infection by phages, fungi, and bacteria, may help in this effort. Finally, long-term investments in data collection, technology, and other resources are needed to characterize changes in toxin trends over time, which may be related to changes in various drivers (for example, water temperature or clarity; Rose and others, 2016).
Table 3.
Scalable science opportunities to study fundamental processes that drive toxin production and release.2.4. Modeling and Predicting Harmful Algal Blooms and Toxins for Prevention, Early Warning, and Mitigation
Vision.—Scientists should have the fundamental understanding of HAB and toxin drivers to integrate mechanistic and statistical modeling to predict the risk of exposure to harmful algae and toxins.
2.4.1. Science Gaps
Understanding blooms, toxin concentrations, and associated health effects requires research on many complex processes over a range of temporal and spatial scales (Burford and others, 2020; Reynolds, 1998). Models are important tools to understand these processes (such as those driving occurrence, algal community composition, and toxin production) and to make decisions based on near-term forecasts or future scenarios (Chapra and others, 2017; Rousso and others, 2020). Choosing the appropriate models for a specific question and location of interest remains a challenge. Uncertainties propagate throughout a modeling framework, and defining sources of model uncertainty is an essential, and often overlooked, part of algal bloom and toxin occurrence models (Rousso and others, 2020). Models are often underpowered and overparameterized because of the lack of resources, time, or data. Characterizing the statistical power to achieve model certainty and breaking down mechanisms into subprocesses are needed.
Another challenge is characterizing the spatial and temporal scales of the interacting processes that affect algal bloom occurrence and toxin production. Scales of effect range from subcellular processes controlling algal growth and toxin production to global processes that drive weather phenomena. Models have been developed across this range of scales; however, models that connect organism-, local-, and broad-scale processes are generally lacking. Moreover, some environments, such as benthos and lotic ecosystems, are understudied relative to the water column of lakes and reservoirs (Graham and others, 2020; Charles and others, 2021).
Models that couple physicochemical and biological processes, watershed and waterbody processes, and processes across the freshwater to marine continuum are needed to advance our ability to forecast algal- and toxin-related events and predict outcomes under various management and climate scenarios. Field-deployed sensors, community science networks, remotely deployable instrumentation, and satellites can provide the required data types across a broad range of spatiotemporal scales. Using these measurement techniques and data types in models has lagged technological advances. Research is needed to determine how best to interpret and integrate these data streams into early warning systems, forecasts, and predictive models (Anderson and others, 2019; Stauffer and others, 2019; Burford and others, 2020).
A hybridized approach, with either a nested suite of models (Glibert and others, 2010) or ensemble modeling (where independent models work independently on the same outcome), may ultimately prove to be the most timely and useful in the near term, particularly in ecosystems experiencing HABs containing multiple organisms or toxins. Continued focus on mechanistic approaches is needed to improve the ability to understand and manage bloom and toxin extent, frequency, and magnitude to minimize associated harmful effects. Temporal and spatial model, prediction, and forecast scalability should be well described to understand the limits of models and forecasts.
Finally, the models and approaches used for algal biomass may not be appropriate for toxins, although mechanistic models for toxins might be linked or incorporated as modules into existing modeling frameworks to better understand the relation between algal growth and toxin production. Scientific advances in the processes driving potentially harmful species and strains and toxin production may help refinement of existing, and development of novel, modeling approaches. Progress on the science gaps and research needs described in other sections of this vision document, such as sample collection, study design, improved sensors, and laboratory measurement, may advance the development of robust models.
2.4.2. USGS Capabilities and Expertise
The USGS is poised to address many of the challenges related to HAB and toxin modeling. The long history and expertise in geospatial analysis and in correlative and mechanistic large-scale models will allow USGS researchers to further address issues related to HABs and toxins; for example, the empirical water-quality USGS Spatially Referenced Regression on Watershed Attributes (SPARROW) and the USGS Load Estimator (LOADEST) models have been used to investigate nutrient loads on a regional scale (Battaglin and others, 2009; García and others, 2016). USGS expertise in modeling large rivers is unparalleled, and this expertise can be expanded to solve issues related to harmful algae and toxins (Graham and others, 2020; Schmadel and others, 2024119). The USGS also has expertise in smaller scale models such as CE–Qual–W2, a mechanistic, two-dimensional model of water quality and hydrodynamics, which has been used to model temperature (Rounds and Stratton Garvin, 2022), nutrients (Rasmussen and Christensen, 2005), algal community dynamics (Smith and others, 2014; Smith and Kiesling, 2019), and other water-quality parameters in various waterbodies. These tools could be integrated with other HAB and toxin models to understand the processes that lead to HAB events.
The USGS also has well-established statistical expertise (Helsel and Hirsch, 1993), including analyses of water-quality trends (Ryberg and Vecchia, 2013; Oelsner and others, 2017; Nustad and Vecchia, 2020), atmosphere and climate trends (Hirsch and Ryberg, 2012), and biological trends (Prevéy, 2020). Additionally, the USGS has experience in integrating satellite imagery with surface properties (Titus and others, 2022), water quality (Petrakis and others, 2022), and HABs (Clark and others, 2017; Legleiter and Hodges, 2022). Combined with the long-term data portals and real-time water quality networks, the capabilities of many USGS scientists could be helpful for quantifying HABs and estimating toxin concentrations. Short-term ecological forecasting (Bradford and others, 2020) also has potential to improve early warning of HABs or toxins. The complexity of data needed will require a broad spectrum of expertise, from ecologists, hydrologists, statisticians, and modelers to climate scientists and economists. This talent is essential to advancing robust, reliable models for early warnings, forecasts, and predictions under various scenarios of change.
2.4.3. USGS Short- and Long-Term Science Opportunities
Data availability and modeling methodology improvements.—The USGS has an opportunity to clearly articulate the degree of operational success of existing models and to determine performance targets and evaluation procedures for future models in the short term (table 4). The fit-for-purpose concept can be used to determine appropriate targets and level of model complexity. For model and data readiness, the USGS could develop and validate automated data-cleaning approaches along with the development or improvement of existing data portals, which could include model storage, multivariate quality assurance, and output for biological, chemical, and physical data fit for modeling (including weather and remote sensing data). The USGS also could pursue more mechanistic models and ensemble or hybrid modeling approaches to clarify the subprocesses involved with a hierarchy for model inclusion over a range of temporal and spatial scales. Describing model, prediction, and forecast readiness should be an important part of transitioning research efforts into publicly available, robust applications with solid characterization of uncertainty and boundary condition testing.
Modeling and prediction of HABs.—Several long-term opportunities relate to the modeling and prediction of HABs, including a need to improve, validate, calibrate, and verify models of key factors concerning bloom lifecycles (for example, transport, species succession, and hypoxia associated with bloom decline). This could include three-dimensional models of bloom transport within and between waterbodies, such as the fresh-to-saline water interface or transport of toxins by wind. Additionally, the USGS is well poised to develop or improve models describing ecosystem dynamics including HAB contributions to food webs, nutrients, and elemental cycling from cyanobacteria and algae—and could use extensive USGS datasets, as well as other datasets (for example, the National Ecological Observing Network; National Science Foundation, 2019). Alternately, the USGS has an opportunity to study water-quality changes driven by HABs, such as HAB-driven light attenuation that mitigates aquatic vascular plant growth or sustainability, the effect of HABs on pH and subsequent water treatment, disinfection bioproducts, invasive species, and redox potential. Combining predictive modeling capability of biomass, toxins, exposures, health outcomes, and socioeconomic tradeoffs could be used to develop a decision support tool for human and animal health. Early warning indicator models of potentially harmful bloom events could be improved, including those that estimate biomass (Smith and Kiesling, 2019).
Modeling and prediction of toxins.—Although numerous models exist to predict HAB biomass or chlorophyll a, few predict toxins. Toxin-predicting models that do exist (Francy and others, 2015; Christensen and others, 2021) could be improved by incorporating more mechanistic components and comparisons to various health thresholds. Opportunities that are specific to toxins include the creation of thermodynamic and kinetic fate models for toxin formation, intracellular release, and natural attenuation. The USGS also could develop and improve thermodynamic and kinetic models for all toxin classes. To improve the ability to model and predict toxin occurrence or concentrations, the USGS has an opportunity to integrate real-time meteorology, high-frequency water temperature and dissolved oxygen depth profiles, and surface layer algal pigments, with high performance computing three-dimensional lake and reservoir hydrodynamic and water-quality models, mechanistic algal biomass models, and decision-support systems. Finally, long-term data for all categories are valuable for the modeling of HAB and toxin trends (sec. 2.2).
Table 4.
Scalable science opportunities to provide knowledge and models for prevention, early warning, and mitigation.2.5. Sampling, Monitoring, and Analytical Methods to Characterize Algal Blooms, Toxin Production, and Exposure
Vision.—Scientists should have the tools and advanced capabilities to be responsive to stakeholder needs by providing the knowledge to prevent, control, or mitigate harmful algae, algal blooms, and toxin exposure (for example, refer to sidebar 3).
Sidebar 3. A Technique to Identify Potentially Toxic Blooms
Detecting and identifying harmful algal blooms (HABs) by remote sensing techniques to determine the conditions leading to toxin production requires the integration of biological, geochemical, and high-resolution spectral data. U.S. Geological Survey researchers are addressing this challenge by studying the dynamics of HABs in lake systems around the United States, including Upper Klamath Lake in Oregon. After years of sample collection in these blooms, hyperspectral microscopy was integrated into this effort (fig. S2). Hyperspectral microscopy of individual cells and small assemblages may help researchers identify the organisms responsible for these HABs and improve the remote sensing tools to detect and track HAB occurrence at wide geographic scales.
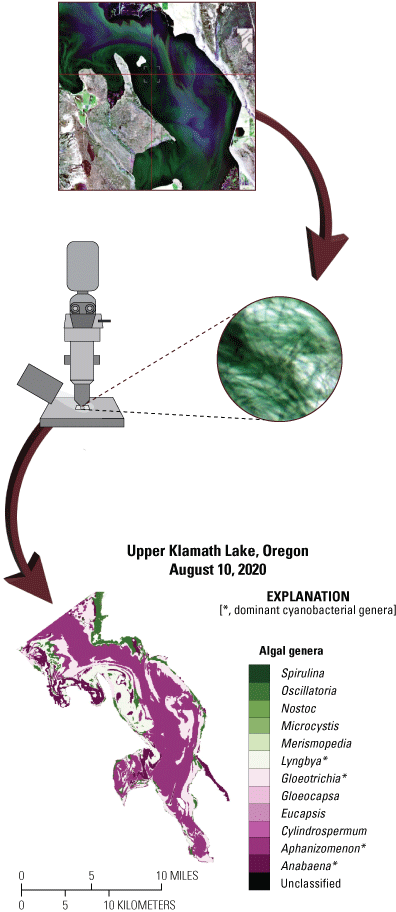
Microscope image of algae from Upper Klamath Lake at × 20 magnification was used to increase comparability with hyperspectral space-based sensors (Legleiter and others, 2022).
2.5.1. Science Gaps
Several gaps in sampling design and methods exist, particularly with respect to representative sampling for HABs. Cross-comparisons of data among studies can be a challenge, and blooms are highly variable (Foster and others, 2017). Representative sampling for HABs based on monitoring objectives or well-constructed study hypotheses will require consistent measurement of blooms, algae, and toxins. Emerging methods of sample and data collection, both field (including passive samplers) and remotely sensed, require validation augmented by robust laboratory methods. Ancillary and toxin data collection may require new or improved sensors, with clearly articulated methods for validation and ongoing verification, to measure parameters that do not currently (2024) exist.
For aquatic remote sensing to realize its full potential as a monitoring capability, a suite of high-quality and well-validated remote sensing products for coastal and inland waterbodies is needed. These data products should focus on not only detecting and retrieving algal biomass and toxins, but also characterizing the upper water columns for other physical, biological, and chemical properties that can aid in more reliable monitoring strategies for programs that prioritize algae sampling. Additionally, aquatic remote sensing science applications, particularly those focused on algae and cyanobacteria, could benefit from the higher spectral resolution sampling that imaging spectroscopy provides (Zolfaghari and others, 2022).
Identifying key organisms is difficult (Rosen and St. Amand, 2015); some identification methods, including visual counting, are prone to human error; and visual characterization is often not specific (for example, similar looking species may not be genetically related, or morphologies may exist that look quite different for closely related species; Anderson and others, 2005). Moreover, visual identification is inadequate for distinguishing toxic and nontoxic varieties of the same species (Medlin and Orozco, 2017) and many organisms cannot be successfully cultured in the laboratory.
Understanding of some understudied toxins has considerable gaps. Laboratory detection methods for many toxins need to be improved; and rapid, simple, and inexpensive assays are urgently needed for some common toxins such as cylindrospermopsin and anatoxin–a. More complex laboratory studies could identify the role of bacteria, viruses, and fungi in controlling or preventing HABs (for example, growth inhibition), including degradation of HAB toxins (Liu and others, 2020). Finally, a better understanding of the correlation between gene expression and toxicity may be accomplished through studies that use empirical environmental DNA (eDNA) as a way to understand how algal and microbial species ecology vary as a function of temperature, water chemistry, location within a bloom, and other factors.
2.5.2. USGS Capabilities and Expertise
The USGS is capable of addressing many of the identified gaps, particularly those related to sample collection, quality assurance, and analyzing water, sediment, and tissue. The USGS has a long history of documented sampling and data collection protocols that are regularly updated in the USGS National Field Manual (U.S. Geological Survey, 2018), including sections on sampling algal biomass indicators (sec. 7.4) and cyanobacteria (sec. 7.5). The USGS has guidelines for developing studies of cyanobacterial toxins and taste-and-odor compounds (Graham and others, 2008) and has a field and laboratory guide to identifying cyanobacteria and freshwater HABs for native communities (Rosen and St. Amand, 2015), both of which could be updated and expanded.
The USGS has a world-class, real-time water monitoring network, which includes water-quality and surrogate time-series data, that could be expanded to include more parameters related to HABs. USGS expertise in real-time data delivery (for example, USGS Water Quality Watch, https://waterwatch.usgs.gov/wqwatch/) could be improved to include additional fluorescence parameters and emerging toxin sensing capabilities. Improved sensing capabilities for toxins is an area for research and development that would be particularly helpful for predictive models. The network and other USGS advanced research computing capabilities could be used for compiling and integrating additional data sources to help improve data delivery of information related to HABs.
The USGS is moving towards developing and applying spectral analysis methods to retrieve and characterize HAB pigments and taxonomy at scales ranging from petri dishes to satellites. The USGS and the National Institute of Standards and Technology (NIST) are developing a hyperspectral microscopy capability to image HAB pigments and taxonomy at the cellular level, which has resulted in a rich new source of remote sensing HABs data to complement field sampling efforts. The USGS is situated in a strong position to contribute to the development and advancement of aquatic remote sensing science from several standpoints. First, the USGS already measures, monitors, analyzes, and manages national water resources. The Cyanobacteria Assessment Network (CyAN) project, which is a joint USGS, National Aeronautics and Space Administration (NASA), National Oceanic and Atmospheric Administration, and U.S. Environmental Protection Agency program focused on HABs, incorporates satellite remote sensing analytics, interpretation, and information for HABs rapid response, management, and decision making. These capabilities allow vast possibilities for USGS to address current HAB observational gaps by exploiting existing or designing new aquatic and hydrological measurement networks. These networks could be on site and automated and could be integrated with remote sensing techniques to measure water quality and algal biomass more effectively at a national scale. The USGS Core Science Systems Mission Area could be a substantial force for advancing basic and applied aquatic remote sensing HAB science through Landsat aquatic data product development (Crawford and others, 2023; Maciel and others, 2023) and HABs modeling and prediction. Looking ahead, the USGS could accelerate and expand its footprint in aquatic imaging spectroscopy more broadly through the Sustainable Land Imaging program research and development initiatives that leverages partnerships with NASA, as well as international governments that are operating or planning imaging spectroscopy missions (Turpie and others, 2023).
The USGS has a long history of developing and evaluating analytical methods, often in collaboration with academic institutions and other Federal agencies (for example, Foreman and others, 2021; Erwin and others, 2021). The USGS is particularly well suited for the development of toxin analysis methods in water and sediment at the USGS Kansas Organic Research Laboratory (Lawrence, Kansas), the National Water Quality Laboratory (Lakewood, Colorado), and other USGS laboratories across the United States. The USGS Ecosystems Mission Area has a history of analyzing and verifying biological tissue methods (for example, De La Cruz and others, 2022). Recent technological advancements within the USGS portfolio include bioinformatic (genomics) and chemoinformatic (metabolomics and proteomics) approaches to explain the processes of life, metabolism, biomarkers of exposure, and biomarkers of health effects. These and other resources set up the USGS to be responsive to the changing needs of our stakeholders and partners.
2.5.3. USGS Short- and Long-Term Science Opportunities
Study designs and sampling.—The USGS has several opportunities to enhance study design and sampling techniques (table 5). Studies on improving data consistency include the development of sampling schemes and metrics to characterize algal bloom heterogeneity horizontally and vertically within the water column as a function of waterbody type. Moreover, the USGS has an opportunity to define monitoring and hypothesis-driven representative sampling approaches and minimum methods validation criteria for field and laboratory data for a given waterbody type independent of salinity, season, or other variables that could affect sample collection. Research on improving sample splitting techniques is underway for phytoplankton and could be expanded to include periphyton and epiphyton. This research could enhance accuracy and precision with measurements by multiple methods. The USGS is actively researching the collection of data with passive samplers, measuring water properties with new and improved on-site sensors (such as fluorometric and chlorophyll sensors as surrogates for biomass or ground-to-space verification), and has an opportunity to develop capability for collecting water samples in difficult-to-access areas with autonomous drones. Opportunities, such as defining HAB features with measurable endpoints (for example, bloom initiation and growth phase), in addition to culturing microorganisms, may inform mechanisms of toxin production and explain favorable environmental conditions for overgrowth. Integrating data from local, state, and community science networks with documented protocols may be a way to increase involvement and collaboration for HABs issues.
Table 5.
Scalable science opportunities to advance capabilities for characterizing algal blooms, toxin production, and exposure.Remote sensing.—For remotely sensed data, short-term opportunities exist to determine the appropriate scope and scale of sampling frequency for ground-to-space or ground-to-airborne measurement verification (including leveraging multiple imaging sources). The aquatic remote sensing community currently is evaluating and conducting algorithm comparisons for removal of atmospheric effects (Pahlevan and others, 2021), working to converge on quantifying algorithm uncertainties for aquatic science applications, and developing prototype aquatic data products. Related opportunities exist to determine the frequency of cloud-free imagery and the minimum and maximum pixel resolution necessary to estimate HABs. Additional spectral bands that may improve algal assemblage characterization are being evaluated. The USGS could invest in improving response times for image collection by autonomous remote sensing (for example, aerial, on-water, submersible drones, and cameras) and could optimize and standardize image collection and quality from fixed camera or optical sensor installations. Another opportunity is to use light detecting and ranging (lidar) technology to remotely monitor algae at depth in water bodies, similar to work that has been done to characterize vertical distributions of freshwater cyanobacteria populations in Lake Erie (Moore and others, 2019). The USGS has long-term opportunities to expand or improve remote sensing products and algorithms for estimating parameters associated with algal bloom dynamics (for example, Secchi depth or turbidity, water surface temperature, water-quality constituents, aquatic plant coverage, and dissolved organic carbon). These opportunities may include machine learning techniques and the development of algorithms at pixel resolutions adequate for all waterbody types with multiple operational platforms (for example, USGS Landsat 8 or 9, or other appropriate emerging commercial or international hyperspectral platforms). Together, these techniques and algorithms could provide high-temporal imaging frequencies and the spatial resolution necessary to automatically exclude human-induced features (similar to Pahlevan and others, 2021) from waterbodies, such as boats, boat docks, and bridges. The USGS could also invest in the development of aquatic spectral libraries and an integrated portal for remote sensing data search and discovery, including methods to integrate imagery from multiple remote sensing sources for quantitative interpretation and decision support. Ideally, this portal would be able to match ground samples coincident or near-coincident to Earth-observing system overpass.
Analytical methods.—In terms of analytical improvements, the USGS has a short-term opportunity to develop, improve, and implement algal pigment measurement (phycocyanin, phycoerythrin, and other pigments) to validate and correlate with remote sensing applications for bloom characterization and trend evaluation in fresh and marine waters. Scientists also could expand nontarget approaches to capture all toxin classes and their congeners. Short-term opportunities exist to develop and (or) validate the mode of action assays to help identify unknown toxins, and to screen biosynthetic toxin precursors, metabolites, transformation, and treatment byproducts. Long-term opportunities related to analytical methods include harmonizing morphologic, phylogenic, and chemotaxonomic approaches, or polyphasic taxonomy, to identify and quantify phytoplankton (including picocyanobacteria, periphyton, and epiphyton). The USGS could also invest in the resources to develop and improve molecular genomic and transcriptomic techniques that identify and quantify phages, phage-associated Clustered Regularly Interspaced Short Palindromic Repeats deoxyribonucleic acid sequences (called “CRISPRs”), evidence of fungal infections in algae, and toxin production. This opportunity also could include establishing a molecular technique to distinguish live versus dead organisms and identifying toxic and nontoxic strains of cyanobacteria or algae to characterize blooms. The USGS could develop and validate biometric and chemometric approaches to measure up and down regulation of luxury element uptake, storage, and utilization processes, including the identification of intermediate biomolecules used in toxin production and their purpose.
3.1. Integrating Science and USGS Capabilities to Support Studies of HABs
The USGS has a workforce that includes scientists from a variety of fields and with specializations in many aspects of HAB research. These scientists include biologists, chemists, data scientists, ecologists, geographers, geologists, geochemists, hydrologists, numerical and process-based modelers, phycologists, physical scientists, social scientists, programmers, statisticians, and wildlife toxicologists. Specialty research ranges from the study of neurotoxins in remote areas to monitoring airborne contaminants to broad geospatial analysis capabilities (Christensen and others, 2021; Krall and others, 2023; Pearlman and others, 2019). The USGS draws on its wide-ranging interdisciplinary and transdisciplinary expertise, nationally consistent laboratory and field capabilities, Earth-observing systems, and computing capabilities (including artificial intelligence, and deep learning capabilities) to support needed data collection and research to test hypotheses related to HABs and associated benefits or harmful effects (for example, refer to sidebar 4). An interdisciplinary science approach can provide stakeholders with the tools and data they need to make decisions to identify, mitigate, and prevent HABs.
Wildlife have long been recognized as sentinels of pollution and other environmental stressors, and this is certainly the case for harmful algal bloom (HAB) events in the Chesapeake Bay region (fig. S3). Between 2000 and 2020, many Chesapeake Bay locations had recurring HAB events. The dominant algal toxins in Chesapeake drainage with harmful effects to avian wildlife are microcystins. Many mortality events were suspected to be related to HABs, and some incidents were coincidental with bloom events; however, few wildlife mortality incidents were definitively linked to HABs (Rattner and others, 2022).
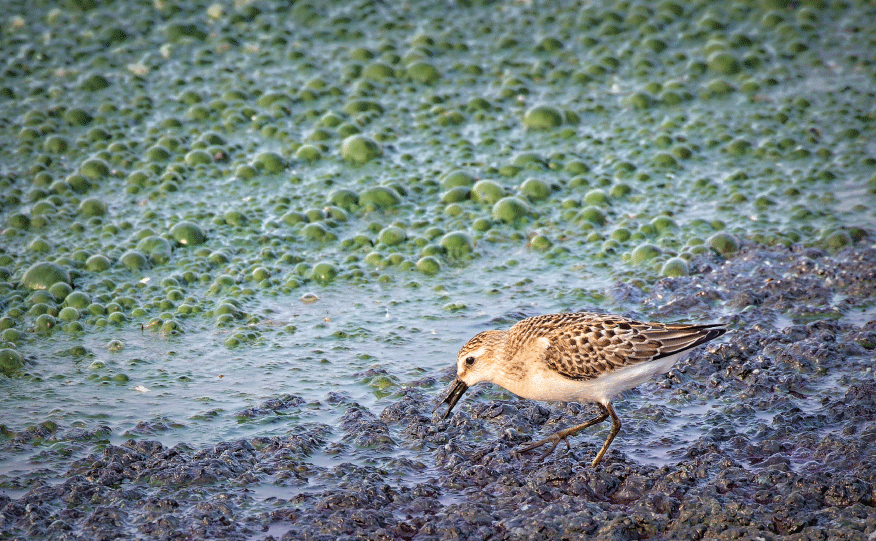
Calidris pusilla (Linnaeus, 1766) (semipalmated sandpiper) foraging in an algal bloom at Poplar Island, Chesapeake Bay, Maryland, on September 14, 2021. Photograph by Serguei V. Drovetski.
The USGS has documented capabilities related to many of the opportunities outlined herein. In terms of benefits and harmful effects of algae, the USGS has documented aquatic primary productivity research (Godwin and others, 2014; Hall and others, 2015), has documented substantial expertise on algae-driven hypoxia (Battaglin and others, 2009; Sullivan and others, 2010), and has developed tools to help managers select, implement, and evaluate natural resource management decisions (Alhassan and others, 2020; Graham and others, 2022; Pindilli and Loftin, 2022). USGS programs are able to integrate large-scale monitoring and assessment with focused smaller-scale studies of individual USGS centers that can help address drivers across a range of environmental gradients, evaluate emerging technologies, and identify novel challenges. The USGS measures, manages, and distributes hydrological, aquatic, biological, and chemical data records and a comprehensive suite of satellite data products that could be helpful for quantifying the effects of land use on hydrological and aquatic systems with respect to HABs. The USGS real-time water quality, fixed-site, and next generation water observing networks that include improved sensing capabilities particularly for toxins, along with well-established statistical expertise (Helsel and Hirsch, 1993; Hirsch and Ryberg, 2012; Nustad and Vecchia, 2020; Ryberg and Vecchia, 2013), could help determine trends in HAB-related data and support modeling and prediction efforts. The USGS is able to address gaps related to sample collection, quality assurance, and analyzing water, sediment, and tissue. Finally, the USGS has a long history of generating sampling and data-collection protocols (U.S. Geological Survey, 2018), and of developing analytical methods in collaboration with academic institutions and other Federal agencies. These and other resources set up the USGS to be responsive to the changing needs of its stakeholders and partners.
3.2. Conceptual Interdisciplinary Science Approach to Studying HABs
Individual groups of scientists in several USGS mission areas are studying HABs. An integrated workflow process has been suggested (Tokranov and others, 2021), which can be applied to HAB research (fig. 3). Briefly, interdisciplinary science can help address the topic areas in chapter 2: weighing the beneficial functions and harmful effects of algae or blooms (sec. 2.1); understanding the natural and anthropogenic drivers of algal blooms (sec. 2.2); understanding the fundamental processes that drive toxin production and release (sec. 2.3); and providing knowledge and models for prevention, early warning, and mitigation of algal blooms and their toxins (sec. 2.4). Advancing capabilities and tools to characterize algal blooms, toxin production, and exposure encompasses all areas of the integrated approach. The critical elements of the workflow for interdisciplinary science activities could include agreed-upon mechanisms for shared technical requirements, leveraging program resources, and collaborative design and implementation across many science centers. A workflow model that has Bureau support (work plans, project reviews, accountability, and coordinated communication) can leverage USGS resources and help ensure the success of interdisciplinary science activities related to HABs.
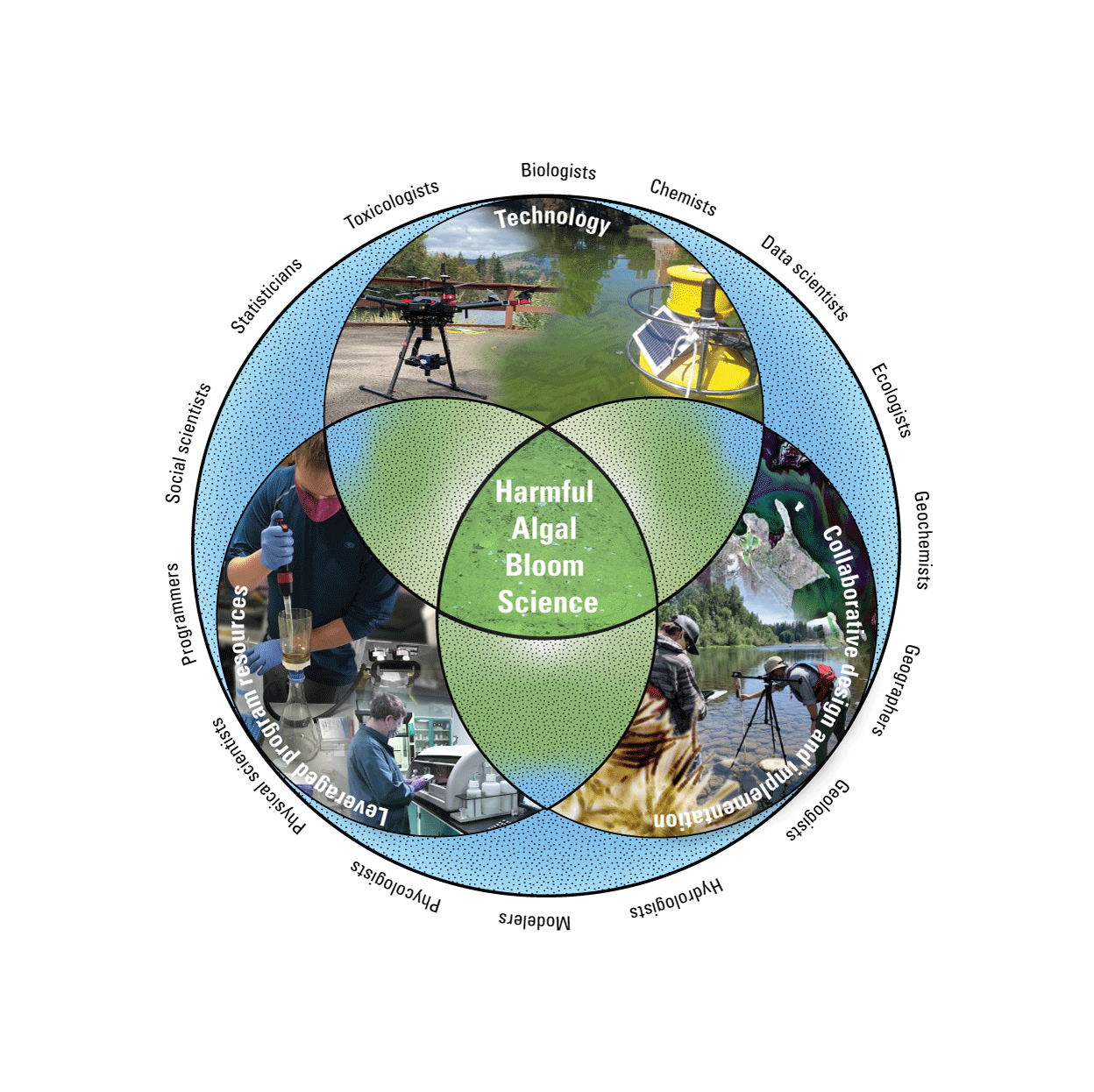
Iinterdisciplinary science areas, shared technology, leveraged program resources, and collaborative study design and implementation for a coordinated approach to the study of harmful algal blooms (HABs) and algal toxins.
Moreover, interdisciplinary science includes collaborative work with researchers and stakeholders outside the USGS. The USGS continues to collaborate with local and regional stakeholders, as well as with many Federal agencies as outlined in the Harmful Algal Bloom and Hypoxia Research and Control Act (Public Law 115–423), to coordinate and integrate HAB research in all Federal programs. The USGS will continue its interagency efforts to assist with the science needed to deploy new tools for remote sensing and event warning systems. On Federal lands, including National Park Service units and U.S. Fish and Wildlife Service refuges, the USGS is assisting with research, data management, and structured decision making. USGS is an active member agency of the Harmful Algal Bloom and Hypoxia Research and Control Act Interagency Working Group, and USGS scientists also collaborate with individual agencies on HAB research, such as the USGS-National Park Service Water-Quality Partnership program.
3.3. Conclusions
The opportunities documented here and discussed among the writing team, reviewers, and other USGS colleagues have been instrumental in establishing a strategic vision for HAB research with an interdisciplinary science approach. This strategic vision can guide the implementation of interdisciplinary science that provides water managers with data needed for decisions, helps build research partnerships, and is an information source for other HAB researchers. The interdisciplinary science activities guided by this vision can address issues at local, regional, and national scales and at varying timeframes as stakeholder needs evolve.
References Cited
Alhassan, M., Pindilli, E.J., Crowley, C.S.L., Shapiro, C.D., and Simon, B.M., 2020, The Interior Office of Policy Analysis supporting natural decisions—The role of economics at the U.S. Department of the Interior (DOI)—2018 DOI Economics Training Workshop: U.S. Geological Survey Open-File Report 2020–1044, 26 p., accessed May 9, 2023 at https://doi.org/10.3133/ofr20201044.
Anderson, C.R., Berdalet, E., Kudela, R.M., Cusack, C.K., Silke, J., O’Rourke, E., Dugan, D., McCammon, M., Newton, J.A., Moore, S.K., Paige, K., Ruberg, S., Morrison, J.R., Kirkpatrick, B., and others, 2019, Scaling up from regional case studies to a global harmful algal bloom observing system: Frontiers in Marine Science, v. 6, no. 250, p. 1–24. [Also available at https://doi.org/10.3389/fmars.2019.00250.]
Anderson, D.M., Fensin, E., Gobler, C.J., Hoeglund, A.E., Hubbard, K.A., Kulis, D.M., Landsberg, J.H., Lefebvre, K.A., Provoost, P., Richlen, M.L., Smith, J.L., Solow, A.R., and Trainer, V.L., 2021, Marine harmful algal blooms (HABs) in the United States—History, current status and future trends: Harmful Algae, v. 102, p. 101975. [Also available at https://doi.org/10.1016/j.hal.2021.101975.]
Anderson, D.M., Kulis, D.M., Keafer, B.A., Gribble, K.E., Marin, R., and Scholin, C.A., 2005, Identification and enumeration of Alexandrium spp. from the Gulf of Maine using molecular probes: Deep-Sea Research Part II—Topical Studies in Oceanography, v. 52, no. 19–21, p. 2467–2490. [Also available at https://doi.org/10.1016/j.dsr2.2005.06.015.]
Baselga-Cervera, B., García Balboa, C., Costas, E., and Lopez-Rodas, V., 2015, Why cyanobacteria produce toxins? Evolutionary game theory suggests the key: International Journal of Biology, v. 7, no. 1, p. 64–77. [Also available at https://doi.org/10.5539/ijb.v7n1p64.]
Battaglin, W.A., Aulenbach, B.T., Vecchia, A., and Buxton, H.T., 2009, Changes in streamflow and the flux of nutrients in the Mississippi-Atchafalaya River Basin, USA, 1980–2007: U.S. Geological Survey Scientific Investigations Report 2009–5164, 48 p. [Also available at https://doi.org/10.3133/sir20095164.]
Belnap, J., 2001, Biological soil crusts—Webs of life in the desert: U.S. Geological Survey Fact Sheet 065–01, 2 p. [Also available at https://doi.org/10.3133/fs06501.]
Benson, M.E., Smith, D.M., and Spaulding, S.A., 2021, Perspectives on the paleolimnology of the late Eocene Florissant lake from diatom and sedimentary evidence at Clare’s Quarry, Teller County, Colorado, USA in From saline to freshwater—The diversity of western lakes in space and time: Geological Society of America, v. 536. [Also available at https://doi.org/10.1130/2018.2536(10).
Blank, C.E., and Sánchez-Baracaldo, P., 2010, Timing of morphological and ecological innovations in the cyanobacteria—A key to understanding the rise in atmospheric oxygen: Geobiology, v. 8, no. 1, p. 1–23. [Also available at https://doi.org/10.1111/j.1472-4669.2009.00220.x.]
Bradford, J.B., Weltzin, J.F., McCormick, M., Baron, J., Bowen, Z., Bristol, S., Carlisle, D., Crimmins, T., Cross, P., and DeVivo, J., 2020, Ecological forecasting—21st century science for 21st century management: U.S. Geological Survey Open-File Report 2020–1073, 54 p. [Also available at https://doi.org/10.3133/ofr20201073.]
Buratti, F.M., Manganelli, M., Vichi, S., Stefaelli, M., Scardala, S., Testai, E., and Funari, E., 2017, Cyanotoxins—Producing organisms, occurrence, toxicity, mechanism of action and human health toxicological risk evaluation: Archives of Toxicology, v. 91, p. 1049–1130. [Also available at https://doi.org/10.1007/s00204-016-1913-6.]
Burford, M.A., Carey, C.C., Hamilton, D.P., Huisman, J., Paerl, H.W., Wood, S.A., and Wulff, A., 2020, Perspective—Advancing the research agenda for improving understanding of cyanobacteria in a future of global change: Harmful Algae, v. 91. [Also available at https://doi.org/10.1016/j.hal.2019.04.004.]
Buttke, D.E., Walker, A., Huang, I.-S., Flewelling, L., Lankton, J., Ballmann, A.E., Clapp, T., Lindsay, J., and Zimba, P.V., 2018, Green tree frog (Hyla Cinerea) and ground squirrel (Xerospermophilus Spilosoma) mortality attributed to inland brevetoxin transport at Padre Island National Seashore, Texas, USA, 2015: Journal of Wildlife Diseases, v. 54, no. 1, p. 142–146. [Also available at https://doi.org/10.7589/2017-01-018.]
Carey, C.C., Ewing, H.A., Cottingham, K.L., Weathers, K.C., Thomas, R.Q., and Haney, J.F., 2012, Occurrence and toxicity of the cyanobacterium Gloeotrichia echinulata in low-nutrient lakes in the northeastern United States: Aquatic Ecology, v. 46, no. 4, p. 395–409. [Also available at https://doi.org/10.1007/s10452-012-9409-9.]
Carmichael, W.W., and Boyer, G.L., 2016, Health impacts from cyanobacteria harmful algae blooms—Implications for the North American Great Lakes: Harmful Algae, v. 54, p. 194–212. [Also available at https://doi.org/10.1016/j.hal.2016.02.002.]
Castle, J.W., and Rodgers, J.H., 2009, Hypothesis for the role of toxin-producing algae in Phanerozoic mass extinctions based on evidence from the geologic record and modern environments: Environmental Geoscience, v. 16, no. 1, p. 1–23. [Also available at https://doi.org/10.1306/eg.08110808003.]
Chapra, S.C., Boehlert, B., Fant, C., Bierman, V.J., Henderson, J., Mills, D., Mas, D.M.L., Rennels, L., Jantarasami, L., Martinich, J., Strzepek, K.M., and Paerl, H.W., 2017, Climate change impacts on harmful algal blooms in U.S. freshwaters—A screening-level assessment: Environmental Science & Technology, v. 51, no. 16, p. 8933–8943. [Also available at https://doi.org/10.1021/acs.est.7b01498.]
Charles, D.F., Kelly, M.G., Stevenson, R.J., Poikane, S., Theroux, S., Zgrundo, A., and Cantonati, M., 2021, Benthic algae assessments in the EU and the US—Striving for consistency in the face of great ecological diversity: Ecological Indicators, v. 121, p. 107082. [Also available at https://doi.org/10.1016/j.ecolind.2020.107082.]
Christensen, V.G., and Khan, E., 2020, Freshwater neurotoxins and concerns for human, animal, and ecosystem health—A review of anatoxin-a and saxitoxin: Science of the Total Environment, v. 736, p. 139515. [Also available at https://doi.org/10.1016/j.scitotenv.2020.139515.]
Christensen, V.G., Stelzer, E.A., Eikenberry, B.C., Olds, H.T., LeDuc, J.F., Maki, R.P., Saley, A.M., Norland, J.E., and Khan, E., 2021, Cyanotoxin mixture models—Relating environmental variables and toxin co-occurrence to human exposure risk: Journal of Hazardous Materials. [Also available at https://doi.org/10.1016/j.jhazmat.2021.125560.]
Clark, J.M., Schaeffer, B.A., Darling, J.A., Urquhart, E.A., Johnston, J.M., Ignatius, A.R., Myer, M.H., Loftin, K.A., Werdell, P.J., and Stumpf, R.P., 2017, Satellite monitoring of cyanobacterial harmful algal bloom frequency in recreational waters and drinking water sources: Ecological Indicators, v. 80, p. 84–95. [Also available at https://doi.org/10.1016/j.ecolind.2017.04.046.]
Coffer, M.M., Schaeffer, B.A., Foreman, K., Porteous, A., Loftin, K.A., Stumpf, R.P., Werdell, P.J., Urquhart, E., Albert, R.J., and Darling, J.A., 2021, Assessing cyanobacterial frequency and abundance at surface waters near drinking water intakes across the United States: Water Research, v. 201, p. 117377. [Also available at https://doi.org/10.1016/j.watres.2021.117377.]
Cottingham, K.L., Ewing, H.A., Greer, M.L., Carey, C.C., and Weathers, K.C., 2015, Cyanobacteria as biological drivers of lake nitrogen and phosphorus cycling: Ecosphere, v. 6, no. 1, p. 1–19. [Also available at https://doi.org/10.1890/ES14-00174.1.]
Cox, P.A., Richer, R., Metcalf, J.S., Banack, S.A., Codd, G.A., and Bradley, W.G., 2009, Cyanobacteria and BMAA exposure from desert dust—A possible link to sporadic ALS among Gulf War veterans: Amyotrophic Lateral Sclerosis, v. 10, no. sup2, p. 109–117. [Also available at https://doi.org/10.3109/17482960903286066.]
Crawford, C.J., Roy, D.P., Arab, S., Barnes, C., Vermote, E., Hulley, G., Gerace, A., Choate, M., Engebretson, C., Micijevic, E., Schmidt, G., Anderson, C., Anderson, M., Bouchard, M., Cook, B., Dittmeier, R., Howard, D., Jenkerson, C., Kim, M., Kleyians, T., Maiersperger, T., Mueller, C., Neigh, C., Owen, L., Page, B., Pahlevan, N., Rengarajan, R., Roger, J.-C., Sayler, K., Scaramuzza, P., Skakun, S., Yan, L., Zhang, H.K., Zhu, Z., and Zahn, S., 2023, The 50-year Landsat collection 2 archive: Science of Remote Sensing, v. 8. [Also available at https://doi.org/10.1016/j.srs.2023.100103.]
Day, N.K., and Henneberg, M.F., 2023, Investigation of potential factors controlling benthic algae in the upper White River Basin, Colorado, 2018–21: U.S. Geological Survey Scientific Investigations Report 2023–5009, 30 p. [Also available at https://doi.org/10.3133/sir20235009.]
De La Cruz, S.E.W., Woo, I., Antonino, C.Y., Hall, L.A., Ricca, M.A., and Miles, A.K., 2022, Biological tissue data used to evaluate selenium hazards in the Salton Sea ecosystem (1984–2020): U.S. Geological Survey data release, accessed February 2023 at https://doi.org/10.5066/P9ECP7O0.
Demay, J., Bernard, C., Reinhardt, A., and Marie, B., 2019, Natural products from cyanobacteria—Focus on beneficial activities: Marine Drugs, v. 17, no. 6. [Also available at https://doi.org/10.3390/md17060320.]
Demoulin, C.F., Lara, Y.J., Cornet, L., François, C., Baurain, D., Wilmotte, A., and Javaux, E.J., 2019, Cyanobacteria evolution—Insight from the fossil record: Free Radical Biology and Medicine, v. 140, p. 206–223. [Also available at https://doi.org/10.1016/j.freeradbiomed.2019.05.007.]
Drake, J.L., Carpenter, E.J., Cousins, M., Nelson, K.L., Guido-Zarate, A., and Loftin, K., 2010, Effects of light and nutrients on seasonal phytoplankton succession in a temperate eutrophic coastal lagoon: Hydrobiologia, v. 654, no. 1, p. 177–192. [Also available at https://doi.org/10.1007/s10750-010-0380-y.]
Erwin, J.A., Fitak, R.R., and Culver, M., 2021, PumaPlex100—An expanded tool for puma SNP genotyping with low-yield DNA: Conservation Genetics Resources, v. 13, p. 341–343. [Also available at https://doi.org/10.1007/s12686-021-01206-7.]
Ferdous, U.T., and Yusof, Z.N.B., 2021, Insight into potential anticancer activity of algal flavonoids—Current status and challenges: Molecules, v. 26, no. 22. [Also available at https://doi.org/10.3390/molecules26226844.]
Foreman, W.T., Williams, T.L., Furlong, E.T., Hemmerle, D.M., Stetson, S.J., Jha, V.K., Noriega, M.C., Decess, J.A., Reed-Parker, C., and Sandstrom, M.W., 2021, Comparison of detection limits estimated using single- and multi-concentration spike-based and blank-based procedures: Talanta, v. 228, no. 122139. [Also available at https://doi.org/10.1016/j.talanta.2021.122139.]
Foster, G.M., Graham, J.L., Stiles, T.C., Boyer, M.G., King, L.R., and Loftin, K.A., 2017, Spatial variability of harmful algal blooms in Milford Lake, Kansas, July and August 2015: U.S. Geological Survey Scientific Investigations Report 2016–5168, 45 p. [Also available at https://doi.org/10.3133/sir20165168.]
Fraker, M.E., Aloysius, N.R., Martin, J.F., Keitzer, S.C., Dippold, D.A., Yen, H., Arnold, J.G., Daggupati, P., Johnson, M.V., Robertson, D.M., Sowa, S.P., White, M.J., and Ludsin, S.A., 2023, Agricultural conservation practices could help offset climate change impacts on cyanobacterial harmful algal blooms in Lake Erie: Journal of Great Lakes Research, v. 49, no. 1, p. 209–210. [Also available at https://www.sciencedirect.com/science/article/pii/S0380133022002593.]
Francy, D.S., Graham, J.L., Stelzer, E.A., Ecker, C.D., Brady, A.M.G., Struffolino, P., and Loftin, K.A., 2015, Water quality, cyanobacteria, and environmental factors and their relations to microcystin concentrations for use in predictive models at Ohio Lake Erie and inland lake recreational sites 2013–14: U.S. Geological Survey Scientific Investigations Report 2015–5120, 58 p. [Also available at https://doi.org/10.3133/sir20155120.]
García, A.M., Alexander, R.B., Arnold, J.G., Norfleet, L., White, M.J., Robertson, D.M., and Schwarz, G., 2016, Regional effects of agricultural conservation practices on nutrient transport in the Upper Mississippi River Basin: Environmental Science & Technology, v. 50, no. 13, p. 6991–7000. [Also available at https://doi.org/10.1021/acs.est.5b03543.]
Glibert, P.M., 2017, Eutrophication, harmful algae and biodiversity—Challenging paradigms in a world of complex nutrient changes: Marine Pollution Bulletin, v. 124, no. 2. [Also available at https://doi.org/10.1016/j.marpolbul.2017.04.027.]
Glibert, P.M., Allen, J.I., Bouwman, A.F., Brown, C.W., Flynn, K.J., Lewitus, A.J., and Madden, C.J., 2010, Modeling of HABs and eutrophication—Status, advances, challenges: Journal of Marine Systems, v. 83, no. 3–4, p. 262–275. [Also available at https://doi.org/10.1016/j.jmarsys.2010.05.004.]
Godwin, S.C., Jones, S.E., Weidel, B.C., and Solomon, C.T., 2014, Dissolved organic carbon concentration controls benthic primary production—Results from in situ chambers in north-temperate lakes: Limnology and Oceanography, v. 59, no. 6, p. 2112–2120. [Also available at https://doi.org/10.4319/lo.2014.59.6.2112.]
Gorney, R.M., Graham, J.L., and Murphy, J.C., 2023, The “H,” “A,” and “B” of a HAB—A definitional framework: Lake Line, v. 43, no. 2, 11 p. [Also available at https://pubs.usgs.gov/publication/70247093.]
Graham, J.L., Ziegler, A.C., Loving, B.L., and Loftin, K.A., 2012, Fate and transport of cyanobacteria and associated toxins and taste-and-odor compounds from upstream reservoir releases in the Kansas River, Kansas, September and October 2011: U.S. Geological Survey Scientific Investigations Report 2012–5129, 65 p. [Also available at https://doi.org/10.3133/sir20125129.]
Graham, J.L., Cebada Mora, G.M., Gorney, R.M., Ball, L.C., Mengelt, C., and Runge, M.C., 2022, A structured decision-making framework for managing cyanobacterial harmful algal blooms in New York State parks: U.S. Geological Survey Scientific Investigations Report 2022–5053. [Also available at https://doi.org/10.3133/sir20225053.]
Graham, J.L., Dubrovsky, N.M., and Eberts, S.M., 2016, Cyanobacterial harmful algal blooms and U.S. Geological Survey science capabilities: U.S. Geological Survey Open-File Report 2016–1174. [Also available at https://doi.org/10.3133/ofr20161174.]
Graham, J.L., Dubrovsky, N.M., Foster, G.M., King, L.R., Loftin, K.A., Rosen, B.H., and Stelzer, E.A., 2020, Cyanotoxin occurrence in large rivers of the United States: Inland Waters, v. 10, no. 1, p. 109–117. [Also available at https://doi.org/10.1080/20442041.2019.1700749.]
Graham, J.L., Foster, G.M., Williams, T.J., Mahoney, M.D., May, M.R., and Loftin, K.A., 2018, Water-quality conditions with an emphasis on cyanobacteria and associated toxins and taste-and-odor compounds in the Kansas River, Kansas, July 2012 through September 2016: U.S. Geological Survey Scientific Investigations Report 2018–5089, 55 p. [Also available at https://doi.org/10.3133/sir20185089.]
Graham, J.L., Loftin, K.A., Ziegler, A.C., and Meyer, M.T., 2008, Guidelines for design and sampling for cyanobacterial toxin and taste-and-odor studies in lakes and reservoirs: U.S. Geological Survey Scientific Investigations Report 2008–5038, 39 p. [Also available at https://doi.org/10.3133/sir20085038.]
Hall, R.O., Yackulic, C.B., Kennedy, T.A., Yard, M.D., Rosi-Marshall, E.J., Voichick, N., and Behn, K.E., 2015, Turbidity, light, temperature, and hydropeaking control primary productivity in the Colorado River, Grand Canyon: Limnology and Oceanography, v. 60, no. 2, p. 512–526. [Also available at https://doi.org/10.1002/lno.10031.]
Hallegraeff, G.M., Anderson, D.M., Belin, C., Bottein, M.Y.D., Bresnan, E., Chinain, M., Enevoldsen, H., Iwataki, M., Karlson, B., McKenzie, C.H., Sunesen, I., Pitcher, G.C., Provoost, P., Richardson, A., Schweibold, L., Tester, P.A., Trainer, V.L., Yñiguez, A.T., and Zingone, A., 2021, Perceived global increase in algal blooms is attributable to intensified monitoring and emerging bloom impacts: Communications Earth & Environment, v. 2, no. 1. [Also available at https://doi.org/10.1038/s43247-021-00178-8.]
Harris, T.D., Wilhelm, F.M., Graham, J.L., and Loftin, K.A., 2014, Experimental manipulation of TN:TP ratios suppress cyanobacterial biovolume and microcystin concentration in large-scale in situ mesocosms: Lake and Reservoir Management, v. 30, no. 1, p. 72–83. [Also available at https://doi.org/10.1080/10402381.2013.876131.]
Ramsdell, J.S., Anderson, D.M., and Glibert, P.M., eds., 2005, Harmful algal research and response—A national environmental science strategy 2005–2015: Washington, D.C., Ecological Society of America, 96 p. [Also available at https://www.whoi.edu/cms/files/HARRNESS_18189_23044.pdf.]
Hassan, S., Meenatchi, R., Pachillu, K., Bansal, S., Brindangnanam, P., Arockiaraj, J., Kiran, G.S., and Selvin, J., 2022, Identification and characterization of the novel bioactive compounds from microalgae and cyanobacteria for pharmaceutical and nutraceutical applications: Journal of Basic Microbiology, v. 62, no. 9, p. 999–1029. [Also available at https://doi.org/10.1002/jobm.202100477.]
Havens, K.E., and Paerl, H.W., 2015, Climate Change at a Crossroad for Control of Harmful Algal Blooms: Environmental Science & Technology, v. 49, no. 21, p. 12605–12606. [Also available at https://doi.org/10.1021/acs.est.5b03990.]
Heisler, J., Glibert, P.M., Burkholder, J.M., Anderson, D.M., Cochlan, W., Dennison, W.C., Dortch, Q., Gobler, C.J., Heil, C.A., Humphries, E., Lewitus, A., Magnien, R., Marshall, H.G., Sellner, K., Stockwell, D.A., Stoecker, D.K., and Suddleson, M., 2008, Eutrophication and harmful algal blooms—A scientific consensus: Harmful Algae, v. 8, no. 1, p. 3–13. [Also available at https://doi.org/10.1016/j.hal.2008.08.006.]
Hirsch, R.M., and Ryberg, K.R., 2012, Has the magnitude of floods across the USA changed with global CO2 levels?: Hydrological Sciences Journal, v. 57, no. 1, p. 1–9. [Also available at https://doi.org/10.1080/02626667.2011.621895.]
Holmes, R.R., Jr., Jones, L.M., Eidenshink, J.C., Godt, J.W., Kirby, S.H., Love, J.J., Neal, C.A., Plant, N.G., Plunkett, M.L., Weaver, C.S., Wein, A., and Perry, S.C., 2013, U.S. Geological Survey natural hazards science strategy—Promoting the safety, security, and economic well-being of the Nation: U.S. Geological Survey Circular 1383–F, 79 p. [Also available at https://doi.org/10.3133/cir1383F.]
Honey, J., and Ivis, D.O., 2023, U.S. Geological Survey Core Research Center—A gateway to subsurface discovery for geoscience research: Geological Society, London, v. 527, no. 1, p. 355–363. [Also available at https://doi.org/10.1144/sp527-2021-194.]
Howard, M.D.A., Kudela, R.M., Hayashi, K., Tatters, A.O., Caron, D.A., Theroux, S., Oehrle, S., Roethler, M., Donovan, A., Loftin, K.A., and Laughrey, Z.R., 2021, Multiple co-occurring and persistently detected cyanotoxins and associated cyanobacteria in adjacent California lakes: Toxicon, v. 192, p. 1–14. [Also available at https://doi.org/10.1016/j.toxicon.2020.12.019.]
Hudnell, H.K., 2010, The state of U.S. freshwater harmful algal blooms assessments, policy and legislation: Toxicon, v. 55, no. 5, p. 1024–1034. [Also available at https://doi.org/10.1016/j.toxicon.2009.07.021.]
Juracek, K.E., 2015, Occurrence and trends of selected nutrients, other chemical constituents, diatoms, and cyanobacteria in bottom sediment, Lake Maxinkuckee, northern Indiana: U.S. Geological Survey Scientific Investigations Report 2014–5199, 61 p. [Also available at https://doi.org/10.3133/sir20145199.]
Krall, A.L., King, K.A., Christensen, V.G., Stokdyk, J.P., Scudder-Eikenberry, B.C., and Stevenson, S.A., 2023, Creek and quarry water quality at Pipestone National Monument and pilot study of pathogen detection methods in waterfall mist at Winnewissa Falls, Pipestone, Minnesota, 2018–19: U.S. Geological Survey Scientific Investigations Report 2022–5122. [Also available at https://doi.org/10.3133/sir20225122.]
Kreiling, R.M., Thoms, M.C., Bartsch, L.A., Larson, J.H., and Christensen, V.G., 2020, Land use effects on sediment nutrient processes in a heavily modified watershed using structural equation models: Water Resources Research, v. 56, no. 7. [Also available at https://doi.org/10.1029/2019WR026655.]
Larson, J.H., Evans, M.A., Kennedy, R.J., Bailey, S.W., Loftin, K.A., Laughrey, Z.R., Femmer, R.A., Schaeffer, J.S., Richardson, W.B., Wynne, T.T., Nelson, J.C., and Duris, J.W., 2018, Associations between cyanobacteria and indices of secondary production in the western basin of Lake Erie: Limnology and Oceanography, v. 63, p. S232–S243.
Larson, J.H., Loftin, K., Stelzer, E.A., Costello, D.M., Bailey, S., Evans, M.A., Givens, C.E., and Fogarty, L.R., 2023, Role of trace metal co-limitation in cyanobacterial blooms of Maumee Bay (Lake Erie) and Green Bay (Lake Michigan): Journal of Freshwater Ecology, v. 38, no. 1. [Also available at https://doi.org/10.1080/02705060.2023.2222747.]
Laughrey, Z.R., Christensen, V.G., Dusek, R.J., Senegal, S., Lankton, J.S., Ziegler, T.A., Jones, L.C., Jones, D.K., Williams, B.M., Gordon, S., Clyde, G.A., Emery, E.B., and Loftin, K.A., 2021, A review of algal toxin exposures on reserved federal lands and among trust species in the United States: Critical Reviews in Environmental Science and Technology, v. 52, no. 23, p. 4284–4307. [Also available at https://doi.org/10.1080/10643389.2021.2010511.]
LeDuc, J.F., Christensen, V.G., and Maki, R.P., 2020, Rapid-assessment test strips—Effectiveness for cyanotoxin monitoring in a northern temperate lake: Lake and Reservoir Management, v. 36, no. 4, p. 444–453. [Also available at https://doi.org/10.1080/10402381.2020.1805531.]
Legleiter, C.J., and Hodges, S.W., 2022, Mapping benthic algae and cyanobacteria in river channels from aerial photographs and satellite images —A proof-of-concept investigation on the Buffalo National River, AR, USA: Remote Sensing, v. 14, no. 4, art. 953. [Also available at https://doi.org/10.3390/rs14040953.]
Legleiter, C.J., King, T.V., Carpenter, K.D., Hall, N.C., Mumford, A.C., Slonecker, T., Graham, J.L., Stengel, V.G., Simon, N., and Rosen, B.H., 2022, Spectral mixture analysis for surveillance of harmful algal blooms (SMASH)—A field-, laboratory-, and satellite-based approach to identifying cyanobacteria genera from remotely sensed data: Remote Sensing of Environment, v. 279, art. 113089. [Also available at https://doi.org/10.1016/j.rse.2022.113089.]
Ling, X., Keqiang, Z., Zijia, Z., Cui, Z., Delong, M., Li, W., Shaoxian, S., Sáncheze, R.M.T., and Farías, M.E., 2022, Rapid artificial biocrust development by co-inoculation of clay and cyanobacteria: Authorea, preprint posted March 23, 2022, accessed May 9, 2023 at https://doi.org/10.22541/au.164848873.33749259/v1.
Liu, Q., Zhang, Y., Wu, H., Liu, F., Peng, W., Zhang, X., Chang, F., Xie, P., and Zhang, H., 2020, A review and perspective of eDNA application to eutrophication and HAB control in freshwater and marine ecosystems: Microorganisms, v. 8, no. 3. [Also available at https://doi.org/10.3390/microorganisms8030417.]
Loftin, K.A., Clark, J.M., Journey, C.A., Kolpin, D.W., Van Metre, P.C., Carlisle, D., and Bradley, P.M., 2016a, Spatial and temporal variation in microcystin occurrence in wadeable streams in the southeastern United States: Environmental Toxicology and Chemistry, v. 35, no. 9, p. 2281–2287. [Also available at https://doi.org/10.1002/etc.3391.]
Loftin, K.A., Graham, J.L., Hilborn, E.D., Lehmann, S.C., Meyer, M.T., Dietze, J.E., and Griffith, C.B., 2016b, Cyanotoxins in inland lakes of the United States—Occurrence and potential recreational health risks in the EPA National Lakes Assessment 2007: Harmful Algae, v. 56, p. 77–90. [Also available at https://doi.org/10.1016/j.hal.2016.04.001.]
Maciel, D.A., Pahlevan, N., Barbosa, C.C.F., de Novo, E.M.L., Paulino, R.S., Martins, V.S., Vermonte, E., and Crawford, C.J., 2023, Validity of the Landsat surface reflectance archive for aquatic science—Implications for cloud-based analysis: Limnology and Oceanography Letters, v. 8, no. 6, p. 850–858. [Also available at https://doi.org/10.1002/lol2.10344.]
Maity, S., Guchhait, R., Chatterjee, A., and Pramanick, K., 2021, Co-occurrence of co-contaminants—Cyanotoxins and microplastics, in soil system and their health impacts on plant—A comprehensive review: Science of the Total Environment, v. 794, art. 148752. [Also available at https://doi.org/10.1016/j.scitotenv.2021.148752.]
Massey, I.Y., Yang, F., Ding, Z., Yang, S., Guo, J., Tezi, C., Al-Osman, M., Kamegni, R.B., and Zeng, W., 2018, Exposure routes and health effects of microcystins on animals and humans—A mini-review: Toxicon, v. 151, p. 156–162. [Also available at https://doi.org/10.1016/j.toxicon.2018.07.010.]
Medlin, L.K., and Orozco, J., 2017, Molecular techniques for the detection of organisms in aquatic environments, with emphasis on harmful algal bloom species: Sensors, v. 17, no. 5, art. 1184. [Also available at https://doi.org/10.3390/s17051184.]
Meriluoto, J., Spoof, L., and Codd, G., eds., 2017, Handbook of cyanobacterial monitoring and cyanotoxin analysis: West Sussex, United Kingdom, Wiley, 576 p. [Also available at https://doi.org/10.1002/9781119068761.]
Meyer, M.F., Topp, S.N., King, T.V., Ladwig, R., Pilla, R.M., Dugan, H.A., Eggleston, J.R., Hampton, S.E., Leech, D.M., Oleksy, I.A., Ross, J.C., Ross, M.R.V., Woolway, R.I., Yang, X., Brousil, M.R., Fickas, K.C., Padowski, J.C., Pollard, A.I., Ren, J., Zwart, J.A., 2024, National-scale remotely sensed lake trophic state from 1984 through 2020: Scientific Data, v. 11, p. 77. [Also available at https://doi.org/10.1038/s41597-024-02921-0.]
Miller, M.P., Kennen, J.G., Mabe, J.A., and Mize, S.V., 2012, Temporal trends in algae, benthic invertebrate, and fish assemblages in streams and rivers draining basins of varying land use in the south-central United States, 1993–2007: Hydrobiologia, v. 684, p. 15–33. [Also available at https://doi.org/10.1007/s10750-011-0950-7.]
Mize, S.V., and Demcheck, D.K., 2009, Water quality and phytoplankton communities in Lake Pontchartrain during and after the Bonnet Carré Spillway opening, April to October 2008, in Louisiana, USA: Geo-Marine Letters, v. 29, p. 431–440. [Also available at https://doi.org/10.1007/s00367-009-0157-3.]
Moore, T.S., Churnside, J.H., Sullivan, J.M., Twardowski, M.S., Nayak, A.R., McFarland, M.N., Stockley, N.D., Gould, R.W., Johengen, T.H., and Ruberg, S.A., 2019, Vertical distributions of blooming cyanobacteria populations in a freshwater lake from LIDAR observations: Remote Sensing of Environment, v. 225, p. 347–367. [Also available at https://doi.org/10.1016/j.rse.2019.02.025.]
Moorman, M.C., Fitzgerald, S.A., and Gurley, L.N., Rhoni-Aref, A., and Loftin, K.A., 2017, Water quality and bed sediment quality in the Albemarle Sound, North Carolina, 2012–14: U.S. Geological Survey Open-File Report 2016–1171, 46 p. [Also available at https://doi.org/10.3133/ofr20161171.]
National Science and Technology Council, 2017, Harmful algal blooms and hypoxia in the Great Lakes research plan and action strategy—An interagency report: Washington, D.C., Executive Office of the President of the United States, 103 p. [Also available at https://cdn.coastalscience.noaa.gov/page-attachments/research/Harmful%20Algal%20Blooms%20Report%20FINAL%20August.2017.pdf.]
National Science Foundation, 2019, National Ecological Observatory Network (NEON): Battelle, National Science Foundation website, accessed May 9, 2023 at https://www.neonscience.org/.
Nielsen, M.C., and Jiang, S.C., 2020, Can cyanotoxins penetrate human skin during water recreation to cause negative health effects?: Harmful Algae, v. 98, art. 101872. [Also available at https://doi.org/10.1016/j.hal.2020.101872.]
Nustad, R.A., and Vecchia, A.V., 2020, Water-quality trends for selected sites and constituents in the international Red River of the North Basin, Minnesota and North Dakota, United States, and Manitoba, Canada, 1970–2017: U.S. Geological Survey Scientific Investigations Report 2020–5079, 75 p. [Also available at https://doi.org/10.3133/sir20205079.]
Oelsner, G.P., Sprague, L.A., Murphy, J.C., Zuellig, R.E., Johnson, H.M., Ryberg, K.R., Falcone, J.A., Stets, E.G., Vecchia, A.V., Riskin, M.L., De Cicco, L.A., Mills, T.J., and Farmer, W.H., 2017, Water-quality trends in the nation’s rivers and streams, 1972–2012—Data preparation, statistical methods, and trend results (ver. 2.0, October 2017): U.S. Geological Survey Scientific Investigations Report 2017–5006, 136 p. [Also available at https://doi.org/10.3133/sir20175006.]
Paerl, H.W., and Huisman, J., 2009, Climate change—A catalyst for global expansion of harmful cyanobacterial blooms: Environmental Microbiology Reports, v. 1, no. 1, p. 27–37. [Also available at https://doi.org/10.1111/j.1758-2229.2008.00004.x.]
Pahlevan, N., Smith, B., Binding, C., Gurlin, D., Li, L., Bresciani, M., and Giardino, C., 2021, Hyperspectral retrievals of phytoplankton absorption and chlorophyll-a in inland and nearshore coastal waters: Remote Sensing of Environment, v. 253, art. 112200. [Also available at https://doi.org/10.1016/j.rse.2020.112200.]
Patiño, R., Christensen, V.G., Graham, J.L., Rogosch, J.S., and Rosen, B.H., 2023, Toxic algae in inland waters of the conterminous United States—A review and synthesis: Water (Basel), v. 15, no. 15, art. 2808. [Also available at https://doi.org/10.3390/w15152808.]
Pearlman, F., Lawrence, C.B., Pindilli, E.J., Geppi, D., Shapiro, C.D., Grasso, M., Pearlman, J., Adkins, J., Sawyer, G., and Tassa, A., 2019, Demonstrating the value of Earth observations—Methods, practical applications, and solutions—Group on Earth Observations side event proceedings: U.S. Geological Survey Open-File Report 2019–1033, 33 p. [Also available at https://doi.org/10.3133/ofr20191033.]
Peller, J., Nevers, M.B., Byappanahalli, M., Nelson, C., Ganesh Babu, B., Evans, M.A., Kostelnik, E., Keller, M., Johnston, J., and Shidler, S., 2021, Sequestration of microfibers and other microplastics by green algae, Cladophora, in the US Great Lakes: Environmental Pollution, v. 276, art. 116695. [Also available at https://doi.org/10.1016/j.envpol.2021.116695.]
Petrakis, R.E., Soulard, C.E., Waller, E.K., and Walker, J.J., 2022, Analysis of surface water trends for the conterminous United States using MODIS satellite data, 2003–2019: Water Resources Research, v. 58, no. 6, art. e2021WR031399, 24 p. [Also available at https://doi.org/10.1029/2021wr031399.]
Pindilli, E.J., and Loftin, K., 2022, What’s it worth? Estimating the potential value of early warnings of cyanobacterial harmful algal blooms for managing freshwater reservoirs in Kansas, United States: Frontiers in Environmental Science, v. 10, p. 1–9. [Also available at https://doi.org/10.3389/fenvs.2022.805165.]
Prevéy, J.S., 2020, Climate change—Flowering time may be shifting in surprising ways: Current Biology, v. 30, no. 3, p. R112–R114. [Also available at https://doi.org/10.1016/j.cub.2019.12.009.]
Rasmussen, P.P., and Christensen, V.G., 2005, Hydrologic and water-quality conditions in the Kansas River, northeast Kansas, November 2001–August 2002, and simulation of ammonia assimilative capacity and bacteria transport during low flow: U.S. Geological Survey Scientific Investigations Report 2005–5188, 111 p. [Also available at https://doi.org/10.3133/sir20055188.]
Rattner, B.A., Wazniak, C.E., Lankton, J.S., McGowan, P.C., Drovetski, S.V., and Egerton, T.A., 2022, Review of harmful algal bloom effects on birds and implications for avian wildlife in the Chesapeake Bay region: Harmful Algae, v. 120, art. 102319. [Also available at https://doi.org/10.1016/j.hal.2022.102319.]
Reynolds, C.S., 1998, What factors influence the species composition of phytoplankton in lakes of different trophic status?: Hydrobiologia, v. 369, p. 11–26. [Also available at https://doi.org/10.1023/A:1017062213207.]
Richer, R., Banack, S.A., Metcalf, J.S., and Cox, P.A., 2015, The persistence of cyanobacterial toxins in desert soils: Journal of Arid Environments, v. 112, part B, p. 134–139. [Also available at https://doi.org/10.1016/j.jaridenv.2014.01.023.]
Roberts, V.A., Vigar, M., Backer, L., Veytsel, G.E., Hilborn, E.D., Hamelin, E.I., Vanden Esschert, K.L., Lively, J.Y., Cope, J.R., Hlavsa, M.C., and Yoder, J.S., 2020, Surveillance for harmful algal bloom events and associated human and animal illnesses—One Health Harmful Algal Bloom System, United States, 2016–2018: Morbidity and Mortality Weekly Report, v. 69, no. 50, p. 1889–1894. [Also available at https://doi.org/10.15585/mmwr.mm6950a2.]
Romanok, K.M., Kolpin, D.W., Meppelink, S.M., Argos, M., Brown, J.B., Devito, M.J., Dietze, J.E., Givens, C.E., Gray, J.L., Higgins, C.P., Hladik, M.L., Iwanowicz, L.R., Loftin, K.A., McCleskey, R.B., McDonough, C.A., Meyer, M.T., Strynar, M.J., Weis, C.P., Wilson, V.S., and Bradley, P.M., 2018, Methods used for the collection and analysis of chemical and biological data for the Tapwater Exposure Study, United States, 2016–17: U.S. Geological Survey Open-File Report 2018–1098, 79 p. [Also available at https://doi.org/10.3133/ofr20181098.]
Rose, K.C., Winslow, L.A., Read, J.S., and Hansen, G.J.A., 2016, Climate-induced warming of lakes can be either amplified or suppressed by trends in water clarity: Limnology and Oceanography Letters, v. 1, no. 1, p. 44–53. [Also available at https://doi.org/10.1002/lol2.10027.]
Rosen, B.H., Davis, T.W., Gobler, C.J., Kramer, B.J., and Loftin, K.A., 2017, Cyanobacteria of the 2016 Lake Okeechobee and Okeechobee Waterway harmful algal bloom: U.S. Geological Survey Open-File Report 2017–1054, 34 p. [Also available at https://doi.org/10.3133/ofr20171054.]
Rosen, B.H., and St. Amand, A., 2015, Field and laboratory guide to freshwater cyanobacteria harmful algal blooms for Native American and Alaska Native communities: U.S. Geological Survey Open File Report 2015–1164, 44 p. https://doi.org/10.3133/ofr20151164.]
Rosen, B.H., Loftin, K.A., Smith, C.E., Lane, R.F., and Keydel, S.P., 2010, Microphotographs of cyanobacteria documenting the effects of various cell-lysis techniques: U.S. Geological Survey Open-File Report 2010–1289, 203 p. [Also available at https://doi.org/10.3133/ofr20101289.]
Rounds, S.A., and Stratton Garvin, L.E., 2022, Tracking heat in the Willamette River system, Oregon: U.S. Geological Survey Scientific Investigations Report 2022–5006, 47 p. [Also available at https://doi.org/10.3133/sir20225006.]
Rousso, B.Z., Bertone, E., Stewart, R., and Hamilton, D.P., 2020, A systematic literature review of forecasting and predictive models for cyanobacteria blooms in freshwater lakes: Water Research, v. 182, art. 115959. [Also available at https://doi.org/10.1016/j.watres.2020.115959.]
Ryberg, K.R., and Chanat, J.G., 2022, Climate extremes as drivers of surface-water-quality trends in the United States: Science of the Total Environment, v. 809. [also available at https://doi.org/10.1016/j.scitotenv.2021.152165.]
Ryberg, K.R., and Vecchia, A.V., 2013, seawaveQ—An R package providing a model and utilities for analyzing trends in chemical concentrations in streams with a seasonal wave (seawave) and adjustment for streamflow (Q) and other ancillary variables: U.S. Geological Survey Open-File Report 2020–1082, 13 p. [Also available at https://doi.org/10.3133/ofr20131255.]
Sanyal, S., Chakrabarti, B., Prasanna, R., Bhatia, A., Kumar, S.N., Purakayastha, T.J., Joshi, R., and Sharma, A., 2022, Influence of cyanobacterial inoculants, elevated carbon dioxide, and temperature on plant and soil nitrogen in soybean: Journal of Basic Microbiology, v. 62, no. 10, p. 1216–1228. [Also available at https://doi.org/10.1002/jobm.202200046.]
Schmadel, N.M., Harvey, J.W., Choi, J., Stackpoole, S.M., Graham, J.L., and Murphy, J.C., 2024, River control points for algal productivity revealed by transport analysis: Geophysical Research Letters, v. 51, no. 5. [Also available at https://doi.org/10.1029/2023GL105137.]
Smith, E.A., and Kiesling, R.L., 2019, Updates to the Madison Lake (Minnesota) CE–QUAL–W2 water-quality model for assessing algal community dynamics: U.S. Geological Survey Scientific Investigations Report 2019–5124, 35 p. [Also available at https://doi.org/10.3133/sir20195124.]
Smith, E.A., Kiesling, R.L., Galloway, J.M., and Ziegeweid, J.R., 2014, Water quality and algal community dynamics of three sentinel deepwater lakes in Minnesota utilizing CE–QUAL–W2 models: U.S. Geological Survey Scientific Investigations Report 2014–5066, 73 p. [Also available at https://doi.org/10.3133/sir20145066.]
Stauffer, B.A., Bowers, H.A., Buckley, E., Davis, T.W., Johengen, T.H., Kudela, R., McManus, M.A., Purcell, H., Smith, G.J., Vander Woude, A., and Tamburri, M.N., 2019, Considerations in harmful algal bloom research and monitoring—Perspectives from a consensus-building workshop and technology testing: Frontiers in Marine Science, v. 6, p. 1–18. [Also available at https://doi.org/10.3389/fmars.2019.00399.]
Stelzer, E.A., Loftin, K.A., and Struffolino, P., 2013, Relations between DNA- and RNA-based molecular methods for cyanobacteria and microcystin concentration at Maumee Bay State Park Lakeside Beach, Oregon, Ohio, 2012: U.S. Geological Survey Scientific Investigations Report 2013–5189, 9 p. [Also available at https://doi.org/10.3133/sir20135189.]
Sullivan, A.B., Snyder, D.M., and Rounds, S.A., 2010, Controls on biochemical oxygen demand in the upper Klamath River, Oregon: Chemical Geology, v. 269, no. 1–2, p. 12–21. [Also available at https://doi.org/10.1016/j.chemgeo.2009.08.007.]
Tatters, A.O., Smith, J., Kudela, R.M., Hayashi, K., Howard, M.D., Donovan, A.R., Loftin, K.A., and Caron, D.A., 2021, The tide turns—Episodic and localized cross-contamination of a California coastline with cyanotoxins: Harmful Algae, v. 103, art. 102003. [Also available at https://doi.org/10.1016/j.hal.2021.102003.]
Terrio, B.P.J., Ostrodka, L.M., Loftin, K.A., Good, G., and Holland, T., 2013, Initial results from a reconnaissance of cyanobacteria and associated toxins in Illinois, August–October 2012: U.S. Geological Survey Open-File Report 2013–1019, 4 p. [Also available at https://doi.org/10.3133/ofr20131019.]
Titus, T.N., Wynne, J.J., Jhabvala, M.D., and Cabrol, N.A., 2022, Using near–surface temperature data to vicariously calibrate high-resolution thermal infrared imagery and estimate physical surface properties: MethodsX, v. 9, art. 101644, 19 p. [Also available at https://doi.org/10.1016/j.mex.2022.101644.]
Tokranov, A.K., Bradley, P.M., Focazio, M.J., Kent, D.B., LeBlanc, D.R., McCoy, J.W., Smalling, K.L., Steevens, J.A., and Toccalino, P.L., 2021, Integrated science for the study of perfluoroalkyl and polyfluoroalkyl substances (PFAS) in the environment—A strategic science vision for the U.S. Geological Survey: U.S. Geological Survey Circular 1490, 50 p. [Also available at https://doi.org/10.3133/cir1490.]
Trevino-Garrison, I., Dement, J., Ahmed, F.S., Haines-Lieber, P., Langer, T., Ménager, H., Neff, J., Van Der Merwe, D., and Carney, E., 2015, Human illnesses and animal deaths associated with freshwater harmful algal blooms—Kansas: Toxins, v. 7, no. 2, p. 353–366. [Also available at https://doi.org/10.3390/toxins7020353.]
Turpie, K.R., Casey, K.A., Crawford, C.J., Guild, L.S., Kieffer, H., Lin, G., Kokaly, R., Shrestha, A., Anderson, C., Chandra, S.N., Green, R., Hook, S., Lukashin, C., and Thome, K., 2023, Calibration and validation for the surface biology and geology (SBG) mission concept—Recommendations for a multi-sensor system for imaging spectroscopy and thermal imagery: Journal of Geophysical Research—Biogeosciences, v. 128, no. 9. [Also available at https://doi.org/10.1029/2023JG007452.]
U.S. Geological Survey, 2018, National field manual for the collection of water-quality data: U.S. Geological Survey Techniques and Methods, book 9, accessed May 9, 2023 at https://www.usgs.gov/mission-areas/water-resources/science/national-field-manual-collection-water-quality-data-nfm.
Vadeboncoeur, Y., Moore, M.V., Stewart, S.D., Chandra, S., Atkins, K.S., Baron, J.S., Bouma-Gregson, K., Brothers, S., Francoeur, S.N., Genzoli, L., Higgins, S.N., Hilt, S., Katona, L.R., Kelly, D., Oleksy, I.A., Ozersky, T., Power, M.E., Roberts, D., Smits, A.P., Timoshkin, O., Tromboni, F., Vander Zanden, M.J., Volkova, E.A., Waters, S., Wood, S.A., and Yamamuro, M., 2021, Blue waters, green bottoms—Benthic filamentous algal blooms are an emerging threat to clear lakes worldwide: Bioscience, v. 71, no. 10, p. 1011–1027. [Also available at https://doi.org/10.1093/biosci/biab049.]
Van Dolah, F.M., 2000, Marine algal toxins—Origins, health effects, and their increased occurrence: Health Perspectives, v. 108, p. 133–141. [Also available at https://www.ncbi.nlm.nih.gov/pmc/articles/PMC1637787/pdf/envhper00310-0137.pdf.]
Zanchett, G., and Oliveira-Filho, E.C., 2013, Cyanobacteria and cyanotoxins—From impacts on aquatic ecosystems and human health to anticarcinogenic effects: Toxins, v. 5, no. 10, p. 1896–1917. [Also available at https://doi.org/10.3390/toxins5101896.]
Zhang, L., Wang, Z., Weng, N., Gu, L., Sun, U., Huang, Y., Chen, Y., and Yang, Z., 2020, Mixotrophic Ochromonas addition improves the harmful Microcystis-dominated phytoplankton community in in situ microcosms: Environmental Science & Technology, v. 54, no. 7, p. 4609–4620. [Also available at https://doi.org/10.1021/acs.est.9b06438.]
Zuellig, R.E., Graham, J.L., Stelzer, E.A., Loftin, K.A., and Rosen, B.H., 2021, Cyanobacteria, cyanotoxin synthetase gene, and cyanotoxin occurrence among selected large river sites of the conterminous United States, 2017–18: U.S. Geological Survey Scientific Investigations Report 2021–5121, 22 p., [Also available at https://doi.org/10.3133/sir20215121.]
Zolfaghari, K., Pahlevan, N., Binding, C., Gurlin, D., Simis, S.G.H., Verdu, A.R., Li, L., Crawford, C.J., Vanderwoude, A., Errera, R., Zastepa, A., and Duguay, C.R., 2022, Impact of spectral resolution on quantifying cyanobacteria in lakes and reservoirs—A machine-learning assessment: IEEE Transactions on Geoscience and Remote Sensing, v. 60, p. 1–20. [Also available at https://doi.org/10.1109/TGRS.2021.3114635.]
Glossary
allelopathy
Allelopathy is the chemical inhibition of one organism by another.
algal toxin
Algal toxins are produced by algae (including cyanobacteria or other organisms commonly called algae). Scientists use the more specific term “cyanotoxins” for toxins produced by cyanobacteria.
anthropogenic
Environmental change originating from human activity.
benthic/benthos
Benthic is a term used to describe organisms (or benthos) at the bottom of a waterbody.
bioaccessibility
Bioaccessiblity refers to the proportion of an ingested chemical that can be used by an organism.
bioinformatic
Bioinformatic approaches are those that develop and use tools for understanding complex biological data.
biosynthesis
Biosynthesis is the production of molecules withing living organisms or cells.
chemoinformatic
Chemoinformatic approaches combine chemistry, computer science, and information science techniques to solve chemistry related problems.
chemotaxonomic
Chemotaxonomic refers to the classification of algae based on similarities or differences in biochemical composition.
cyanobacteria
Cyanobacteria are prokaryotic organisms that are true bacteria with algal characteristics capable of oxygenic photosynthesis. Some researchers use the terms “CyanoHAB” or “harmful cyanobacterial bloom (HCB)” to distinguish cyanobacterial blooms from other types of potentially harmful algal blooms.
genomics
Genomics is an interdisciplinary field of biology focusing on the structure, function, and mapping genomes (chromosomes in a microorganism).
epiphyton
Epiphyton are algae or other organisms that grow on plants.
eutrophication
Eutrophication occurs when a body of water receives an excessive nutrient load, particularly phosphorus and nitrogen.
lotic
Lotic describes an environment of rapidly moving water.
mechanistic
A mechanistic model is based on fundamental laws, including physical and biochemical principles.
metabolomics
Metabolomics is the study of the metabolites inside an organism or cell.
metagenomics
Metagenomics is the study the genomic (DNA and RNA) content, identifying which microbes are present within a water sample or other material.
metatranscriptomics
Metatranscriptomics is a set of techniques used to study gene expression of microbes in a water sample or other material.
metabolites
A metabolite is a substance formed from a living organism in order to maintain life.
microplastics
Microplastics are very small pieces of plastic, some so small that they cannot be seen without a microscope.
morphologic
Morphologic approaches identify algae based on form or structure.
periphyton
Periphyton are algae or other organisms attached to plants, rocks, or objects projecting above the bottom sediments.
phylogenic
Phylogenic approaches identify organisms based on the relationship among them.
polyphasic
Using multiple approaches to identifying algae is referred to as polyphasic taxonomy.
polysaccharides
Polysaccharides are carbohydrates with molecules that consist of sugar molecules bonded together.
proteomics
Proteomics is the study of proteins within an organism or cell and their functions.
trophic gradient
Trophic gradient refers to the range of varying amounts of living biological material in a waterbody.
Appendix 1. Select U.S. Geological Survey Publications Related to Harmful Algal Blooms (HABs) or Algal Toxins, 2013–2024
For more information about this publication, contact:
USGS Water Resources Mission Area
12201 Sunrise Valley Drive
Reston, VA 20192
For additional information, visit: https://www.usgs.gov/mission-areas/water-resources
Publishing support provided by the Rolla and Sacramento Publishing Service Centers
Disclaimers
Any use of trade, firm, or product names is for descriptive purposes only and does not imply endorsement by the U.S. Government.
Although this information product, for the most part, is in the public domain, it also may contain copyrighted materials as noted in the text. Permission to reproduce copyrighted items must be secured from the copyright owner.
Suggested Citation
Christensen, V.G., Crawford, C.J., Dusek, R.J., Focazio, M.J., Fogarty, L.R., Graham, J.L., Journey, C.A., Lee, M.E., Larson, J.H., Stackpoole, S.M., Mazzei, V., Pindilli, E.J., Rattner, B.A., Slonecker, T., McSwain, K.B., Reilly, T.J., and Lopez, A.E., 2024, Interdisciplinary science approach for harmful algal blooms (HABs) and algal toxins—A strategic science vision for the U.S. Geological Survey: U.S. Geological Survey Circular 1520, 39 p., https://doi.org/10.3133/cir1520.
ISSN: 2330-5703 (online)
Publication type | Report |
---|---|
Publication Subtype | USGS Numbered Series |
Title | Interdisciplinary science approach for harmful algal blooms (HABs) and algal toxins—A strategic science vision for the U.S. Geological Survey |
Series title | Circular |
Series number | 1520 |
DOI | 10.3133/cir1520 |
Year Published | 2024 |
Language | English |
Publisher | U.S. Geological Survey |
Publisher location | Reston, VA |
Contributing office(s) | Earth Resources Observation and Science (EROS) Center, Kansas Water Science Center, National Wildlife Health Center, New Jersey Water Science Center, New York Water Science Center, South Atlantic Water Science Center, Upper Midwest Water Science Center, Toxics Substances Hydrology Program |
Description | Report: vi; 39 p.; Appendix |
Online Only (Y/N) | Y |
Google Analytic Metrics | Metrics page |