An Inventory of Three-Dimensional Geologic Models—U.S. Geological Survey, 2004–22
Links
- Document: Report (9.68 MB pdf) , HTML , XML
- Related Work: USGS 3D Geologic Model Inventory
- Data Release: USGS data release - Spatial data from An Inventory of U.S. Geological Survey Three-Dimensional Geologic Models, Volume 1, 2004–2022
- Download citation as: RIS | Dublin Core
Acknowledgments
This study was funded by the U.S. Geological Survey (USGS) National Cooperative Geologic Mapping Program. We thank Geoff Cromwell and Marina Marcelli (USGS) for sharing preliminary results from a similar model inventory activity being conducted within the USGS Water Resources Mission Area as part of the “National Extent Hydrogeologic Framework” project. This manuscript was improved by thoughtful peer reviews by Geoff Phelps and Wayne Belcher, both of the USGS.
Abstract
A database of spatial footprints and characteristics of three-dimensional geological models that were constructed by the U.S. Geological Survey between 2004 and 2022 was compiled as part of ongoing development of subsurface geologic information by the U.S. Geological Survey National Cooperative Geologic Mapping Program. This initial inventory resulted in the compilation of 38 three-dimensional geological models that vary widely in their spatial extent, the type and purpose of the model, the number of subsurface units characterized by the model, and the software platforms used to create the model. This Data Report provides the scientific rationale and explanation of the contents of a companion USGS digital data release of spatial data and attributes associated with each three-dimensional model.
Introduction
U.S. Geological Survey (USGS) science-planning documents (Gundersen and others, 2011; Bristol and others, 2013) and decadal strategic plans for the USGS National Cooperative Geologic Mapping Program (NCGMP; Brock and others, 2021) call for geologic mapping across the Nation to become increasingly three-dimensional (3D). The plan for nationwide 3D geologic mapping has been endorsed by the Association of American State Geologists (Allison, 2014). Increases in Congressional appropriations in Federal fiscal years 2020, 2021, and 2022 directed the NCGMP to launch and build “Phase Three of the National Geologic Map Database” using language related to a nationwide geologic synthesis proposed by Soller and Berg (2003). For example, House Report 116–100 mandated a national geologic synthesis “to bring together detailed national and continental-resolution 2D [two-dimensional] and 3D [three-dimensional] information produced throughout the USGS and by federal and state partners” (U.S. Congress, 2019, p. 48–49). In response to Congressional direction and funding, the NCGMP launched the U.S. GeoFramework Initiative to deliver a digital national geologic map and 3D geologic framework model of the United States (Shelton and others, 2022).
The strategic plans and enabling legislation (U.S. Congress, 2019) provide the impetus for a focused effort within the NCGMP to compile, synthesize, and distribute 3D geologic data at varying scales. The project is a potentially huge undertaking involving a compilation of vast quantities of disparate subsurface data. One natural starting point is the inventory and cataloging of salient attributes from 3D geologic models, which use and aggregate large amounts of 3D geologic data. However, the creation of such an inventory is hampered by the distributed nature of subsurface investigations by USGS researchers and a general lack of cataloging and archiving of 3D geologic models and subsurface products. U.S. Geological Survey scientific activities are organized around major topics or Mission Areas that align with distinct science themes (USGS, 2007; Gundersen and others, 2011). Typically, 3D geological modeling supports research and project work within a specific USGS Mission Area. As a result, 3D modeling activities are decentralized and results are released on a project-by-project basis in various ways (Sweetkind and others, 2019). Subsurface data from the various USGS Mission Areas are typically released either on the USGS ScienceBase Data Catalog (https://www.sciencebase.gov/catalog/) or through the USGS Publications Warehouse website (https://pubs.usgs.gov/). The USGS Water Resources Mission Area previously hosted a node of the National Spatial Data Infrastructure (Federal Geographic Data Committee, 2020) in which to store geologic model results and model-relevant subsurface datasets; as of 2023, these data have been migrated to the USGS ScienceBase Data Catalog (https://www.sciencebase.gov/catalog/). Certain specific USGS 3D realizations, such as the generation of 3D subduction zone geometries, are hosted in their own topical repositories (https://github.com/usgs/slab2).
The purpose of this Data Report, companion digital data release (Sweetkind and Zellman, 2022), and webapp (https://apps.usgs.gov/3d_geologic_model_inventory/index.html) is to begin a part of the national-scale work under the U.S. GeoFramework Initiative (Shelton and others, 2022) by inventorying, for the first time, the location and attributes of 3D geologic models constructed by the USGS across all USGS Mission Areas for models where digital data were released. This inventory assembles, in catalog form, the location, spatial extent, and salient characteristics of previously published USGS 3D geologic models where digital model results were released. The inventory includes citations of the original model publications and datasets so that interested users may find and use these products. This initial inventory is not intended to be an archive of subsurface data and maps that underpin—or of the digital elements that constitute—3D geologic models. It is anticipated that this inventory will be incrementally expanded to include, for example, 3D geologic models funded or produced by other Federal agencies, State geological surveys, or academic institutions. Through the expansion of scope and periodic updates of content as new models are released, the inventory is intended to serve as a comprehensive and persistent record of 3D geologic models produced throughout the United States.
What is a 3D Geologic Model?
The definition of the term “3D geologic model” drives the scope of the investigation and defines what studies are and are not included in the inventory. The broadest definition of 3D geologic model includes any instance in which subsurface geology is conceptualized through interpolation, extrapolation, or modeling of observed datasets; such conceptualizations include models of rock properties, such as permeability or seismic velocity, or geophysically based models where a subsurface horizon is modeled based on interpretation and numerical inversion of a potential field response (Culshaw, 2005; Turner and others, 2021). Using a narrower, and more useful definition, 3D geologic models are digital representations of the Earth’s subsurface that use data models and computer techniques to represent geo-objects (for example, strata, structures, nonstratified geological bodies, and rock type) in 3D and depict, in a volumetric sense, information that geologic maps portray at the Earth’s surface (Turner, 2005; Apel, 2006). Such models are typically built with software that use 3D topological data models and special interpolation methods (Apel, 2006; Keefer and others, 2011; Aswar and Ullagaddi, 2017a). Geological models generally include various types of digital-input data; geological rules for how geologic units or model elements interact; and human- and computer-based rules, methods, and geologic reasonings for interpolating, extrapolating, and modeling soft and hard data.
The terms “3D geological mapping” and “3D geological modeling” are used somewhat interchangeably in the geological literature. Although some authors advocate for exclusive use of the term “3D geological mapping” (Phelps and others, 2008) and there have been attempts at standardization of terminology (Berg and Keefer, 2004), both terms are commonly used (Berg and others, 2011; MacCormack and others, 2019). For this inventory, we chose 3D geologic model as the descriptive term for all digital 3D representations of subsurface geology and did not attempt to distinguish between 3D maps and 3D models during the inventory process.
Digital 3D geologic models may be subdivided into various classes based on many factors:
-
(1) How the Earth is conceptualized, for example, as boundary representation models that model a geo-object by defining its boundaries (Apel, 2006) as compared with representations that model a geo-object as a continuous field through space, such as a specific lithology or geologic formation.
-
(2) The choice of data model, such as all geologic models that are represented as relational databases.
-
(3) Models that have volumetric topology compared to models that do not.
-
(4) The technical implementation of a model, such as all models created in ArcGIS.
-
(5) The purpose of a geologic model, for example, models designed to support seismic hazards analysis by portraying the geometry of crustal-scale faults.
-
(6) The information attributes of the model, such as models that depict a rock property, for example, porosity, as compared to models that depict classed lithology categories (Keefer and others, 2011; Aswar and Ullagaddi, 2017a, b; Russell and others, 2019; Turner and others, 2021).
Digital 3D geologic models can also be classified as either “explicit models” that fill 3D volumes through interpolation among, and extrapolation beyond, abundant subsurface geologic input data or “implicit models” where geologic concepts are formalized within the modeling software; both classifications differ from stochastic models that rely on geostatistical approaches (Russell and others, 2019; Turner and others, 2021).
For this inventory, models were classified as either (1) “geo-object models,” also called boundary representation models, wherein the 3D volume is subdivided into a set of subregions defined by stratigraphic surfaces and structures or (2) “geocellular models” that subdivide the 3D volume through voxel-based, volume-element, methods (Keefer and others, 2011; Aswar and Ullagaddi, 2017b). The USGS 3D geologic models are generally explicit, data-driven boundary-based models where the emphasis is on defining geologic layering. Voxel-based properties models are less commonly produced by the USGS and stochastic models are quite rare. We chose not to inventory stochastic 3D models where the model volumes are filled based on a series of rules because the USGS rarely performs such modeling exercises (Cronkite-Ratcliff and Phelps, 2014) and none to date have resulted in available digital datasets.
In addition to defining the types of 3D geologic models in this inventory, it is important to list the types of subsurface data and models that were not considered in the inventory:
Purely conceptual models.—These models include block diagrams that may be drawn by hand.
Cross sections.—Cross sections conceptualize the subsurface and involve the use of geologic rules.
Structure contour and isopach maps.—Contour maps of subsurface data can be important input components of 3D models. Contour maps combine point-input data and interpolation methods to create an interpretation of a continuous surface but are not considered here as 3D geologic models.
Geophysically based models.—Models that combine seismic reflection or potential field data with assumptions of the spatial distribution of seismic velocity, density, or magnetization to produce two-dimensional and 3D realizations of the subsurface.
This inventory covers the period from 2004, the date of the earliest published model with publicly available digital data (Pantea and Cole, 2004), to 2022. The USGS was involved in 3D geologic modeling prior to 2004, such as at Yucca Mountain, Nevada, the Death Valley region of Nevada and California (D’Agnese and others, 1997), and in the Rio Grande basin, New Mexico (Cole and others, 1999), but those reports were not included because no digital data were released.
Numerical process models, such as geological process models that predict the potential location and magnitude of earthquakes or hydrologic process models that predict changes in groundwater quantity and movement, may include some representation of the Earth’s subsurface as part of their numerical structure. Such models may contain layering schemes that have a basis in subsurface geologic units (for example, Pool and others, 2011; Hanson and others, 2014), but a representation of the subsurface geologic framework is not created or computed separately as a digital 3D geologic model that serves as input to the process model. The geologically relevant features from USGS numerical process models were not included in this model inventory, although including these features is likely a worthwhile future activity.
The USGS National Crustal Model is being developed to assist with earthquake-hazard estimates in the USGS National Seismic Hazard Model (Boyd, 2019a, b, 2020). A 3D geologic framework is one of the primary components of the USGS National Crustal Model; to date, a 3D geologic framework has been constructed for the western United States (Shah and Boyd, 2018; Boyd, 2019a, b). The framework is based on 1:250,000- to 1:1,000,000-scale State geologic maps, modeled depths of multiple subsurface unit boundaries, and extrapolation of rock type in the subsurface using nearest-neighbor interpolation techniques. Because the USGS National Crustal Model will span the conterminous United States, a model-bounding polygon was not created, and this small-scale model was not included in the current inventory.
Methodology
This section described the methods used to find published USGS 3D geologic models and the model compilation approach in creating model-related spatial data and associated nonspatial attributes.
Description of Search Process
The USGS 3D geologic model inventory had its genesis as two USGS-focused chapters in synopsis volumes of 3D geological mapping and modeling activities in geological survey organizations (Jacobsen and others, 2011; Sweetkind and others, 2019). In these chapters, the authors pooled their knowledge of models they created or their colleagues created. Inventory activities were expanded using keyword searches of USGS publications, new publication announcements released by the USGS Water Resources Mission Area, and searches of the following data repositories: the USGS Publications Warehouse (https://pubs.usgs.gov/), the USGS ScienceBase Data Catalog (https://www.sciencebase.gov/catalog/), the U.S. Government’s open data site (https://data.gov/), the NCGMP’s National Geologic Map Database catalog (https://ngmdb.usgs.gov/ngmdb/ngmdb_home.html), the USGS Central Energy Resources Science Center’s Assessments of Undiscovered Oil and Gas Resources website (https://www.usgs.gov/centers/central-energy-resources-science-center/science/united-states-assessments-undiscovered-oil), and the USGS Water Resources Mission Area National Spatial Data Infrastructure node (https://water.usgs.gov/lookup/getgislist).
The initial inventory was primarily limited to internally funded USGS studies, which eliminated models that were funded by other Federal agencies, such as geothermal investigations funded by the U.S. Department of Energy (Siler and others, 2019, 2021). One significant exception was the inclusion of the 3D geologic framework of the Death Valley regional flow system in Nevada and California. This framework model was funded by the U.S. Department of Energy and constructed within the USGS Yucca Mountain Project by USGS geologists and hydrologists. It is anticipated that this inventory will be revised as new USGS models are published and expanded to include 3D models created by other Federal agencies, State geological surveys, or academic institutions.
Compilation Approach
Information about each 3D geologic model was standardized by (1) creating a model-bounding polygon using a geographic information system (GIS) to show the map extent of each model in a common geographic projection, (2) compiling publication source information in a single nonspatial table linked to the spatial footprints through a unique identifier, and (3) developing a common set of model attributes that generally describe each model and provide a means of comparing salient model characteristics.
The standardized information was assembled as a USGS digital data release (Sweetkind and Zellman, 2022), in which spatial extents of the 3D geologic models (as polygon features) are attributed with unique identifiers. These identifiers link the spatial data to nonspatial tables that define the data sources used and describe various aspects of each published model. The nonspatial DataSources table within the data release includes full citation and uniform resource locator (URL) address for published model reports and any digital model data released as a separate publication. The nonspatial ModelAttributes table within the data release classifies the type of model and uses several classification schemes; identifies the model purpose and originating agency; and describes the spatial extent, depth, and number of layers included in each model. These attributes are described in the “Model Attributes Table” section. A companion USGS webapp (https://apps.usgs.gov/3d_geologic_model_inventory/index.html) allows a user to interrogate and filter the data by category or attribute.
Model-Bounding Polygons
The spatial extent or bounding polygon of each model was created in various ways, depending on the content of the original dataset, including georeferencing an index map from the publication, digitizing a polygon, downloading a boundary file from the source publication’s digital data, creating a polygon boundary from the extent of the model grid, and using a GIS to create a closed polygon that surrounds a series of structure contour lines, or, for county-based models, downloading a county boundary from standard source libraries of spatial data. Model-bounding polygons were attributed with a short, unique model name, a DataSourceID attribute that links the polygon to entries in the nonspatial DataSources table, and a brief note on how the bounding polygon was created.
DataSources Table
The DataSources table provides the full-text citation and URL for each published element related to the model. Some 3D geologic models are described in two publications—a formal model report and a report that released the digital-input data and model output—whereas for other models, data are available through links on the model report’s publication page on the USGS Publications Warehouse website. The DataSources table includes a short, unique model name that provides the link to the model spatial extent, publication links, and a notes field for aspects specific to each entry.
Model Attributes Table
The Model Attributes table includes fields that characterize the models in terms of type, size, and overall purpose. These 10 attributes highlight important commonalities or distinctions among the models.
ModelType
ModelType characterizes the general class of 3D model that was constructed. We followed the designation of Apel (2006) and assigned subsurface geological models to one of two classes: (1) surface-based approaches, or boundary representation models, where the boundaries of geobodies are surfaces that subdivide the model volume and (2) volume-based approaches, or geocellular models, where a grid of blocks are created and attributed according to geologic unit (Keefer and others, 2011; Aswar and Ullagaddi, 2017a; Turner and others, 2021). In boundary representation models, the interior of each geobody is generally considered spatially homogeneous and the geobody properties are described by means of files or tables. However, boundary representation models are not limited to such approaches and interior geobody properties may be allowed to vary, such as with mathematical formulas that specify a property gradation across the volume.
GeologyType
GeologyType describes how geology is represented or aggregated within the model. Surface-based or stratigraphic models are categorized as either “geology,” where the modeled surfaces represent stratigraphic contacts that can generally be correlated to contacts mapped on surface geologic maps, or “hydrogeology,” where the modeled surfaces represent known or inferred boundaries within the rock mass that separate intervals with differing abilities to store and transmit water. Volume-based properties models are categorized as “lithology”; the attribute is applied to models that interpolate subsurface lithology through a 3D volume. Other types of properties models, such as porosity or seismic velocity models, may exist, but only lithology models were used in the initial inventory.
Topology
Topology characterizes the spatial organization of the model in the subsurface. In their simplest form, the subsurface interfaces within a boundary representation model can be created as a series of noninteracting surfaces, such as an ordered set of structure contour maps or a raster grid, which has one surface for each boundary of interest. Surfaces that can be stacked in 3D without any particular regard to how the surfaces interact are classified as “open” models. 3D geologic models that enforce topological rules on boundary representations in a mathematically rigorous way to simulate geologic conditions, such as erosion and onlap, are classified as “sealed” geologic models (Caumon and others, 2004, 2009; Aswar and Ullagaddi, 2017a). Geocellular models do not explicitly incorporate geologic boundaries as objects but can implicitly store boundaries as the relationship between adjacent cells of differing geologic unit designation. Because of the implicit nature of the topology, we classified geocellular models as “open” models.
GeomaterialsModeled
GeomaterialsModeled characterizes the part of the subsurface stratigraphic section being modeled. In general, models are subdivided into one of two classes. The first class of models are attributed as “consolidated rocks”; these models focus primarily on consolidated rocks, either because consolidated rocks are the principal interest in the geologic province being modeled (Pantea and Cole, 2004; Hudson and others, 2016), or the model volume extends to great depth, like models that portray crustal-scale faults (Phelps and others, 2008). The second class is attributed as “basin fill,” and used for those models primarily concerned with the thickness and character of poorly consolidated rocks, either as part of groundwater studies in basin-filling sediments and sedimentary rocks (Sweetkind, 2017; Cromwell and Matti, 2022), or studies of glacial deposits (Bayless and others, 2017). In models attributed as “basin fill,” consolidated rocks at the base of the model are typically undivided and not modeled as distinct units. Some regional-scale models include unconsolidated to poorly consolidated deposits and consolidated rock units (Faunt and others, 2010; Cederberg and others, 2011).
Purpose
Purpose characterizes the general purpose for which the 3D model was constructed. USGS 3D geological mapping efforts typically occur on a project-by-project basis and 3D geologic models are often purpose-built. In the USGS Water Resources Mission Area, 3D geologic models are built to support water-resource assessments and for aquifer delineation and characterization (Evenson and others, 2013). The USGS Energy and Mineral Resources Mission Area uses 3D geologic models to facilitate an improved understanding of geologic processes and support resource assessments (Ferrero and others, 2013). The USGS Core Science Systems Mission Area, which includes the NCGMP, uses 3D geologic models to inform studies of the Earth’s complex processes (Bristol and others, 2013).
CreatingOrg
CreatingOrg captures the USGS Program or Mission Area within the USGS that is associated with the model. 3D modeling typically supports research and project work within a specific USGS Program or Mission Area (USGS, 2007; Gundersen and others, 2011). In future revisions to the inventory, the attribute can be expanded to include models from other Federal agencies, State geological surveys, or academic institutions.
ModelingPlatform
ModelingPlatfrom characterizes the software platform in which the model was created. In many modeling projects, data management, data preprocessing, and model visualization tasks require hybrid approaches where several software platforms are used (Kaufmann and Martin, 2008; Aswar and Ullagaddi, 2017a, c; Turner and others, 2021). This attribute is used as an attempt to capture the software platform used for the main part of the 3D processing and computation of geological models.
ModelArea and ModelDepth
ModelArea is the area, in square kilometers, of the spatial extent of the model domain. The spatial extent is defined by a bounding polygon that is based on the vertical projection of the model region onto the Earth’s surface. ModelDepth defines the general depth, in meters, of the model base below land surface. The attributed depth value, which is a shorthand way of defining the depth extent of the model, is used as a general comparator between geologic models.
NumberofLayers
NumberofLayers is used to characterize the number of geologic subdivisions present in a model. For boundary representation models, where the boundaries of geobodies are stratigraphic surfaces that subdivide the model volume, the attribute value is the number of modeled geologic or hydrologic units. For geocellular models that distribute lithologic data throughout a volume, the attribute value is the number of lithologic classes the model was broken into. The term “layers” could be generalized to “geobody” in future inventory iterations to better account for complex nonstratigraphic models.
Results
This initial inventory resulted in 38 3D geologic models constructed by the USGS across all Mission Areas (fig. 1; Sweetkind and Zellman, 2022). In aggregate, the spatial extent of these 3D models covers 3,932,126 square kilometers (km2), which is approximately 49 percent of the total area of the contiguous United States (8,080,464 km2). This extent is skewed by the presence of a single model that covers the entire glaciated region of the northern conterminous United States (fig. 1; Bayless and others, 2017). The median spatial extent for each model is 4,170 km2, which is a more representative descriptor of 3D model coverage than the total area.
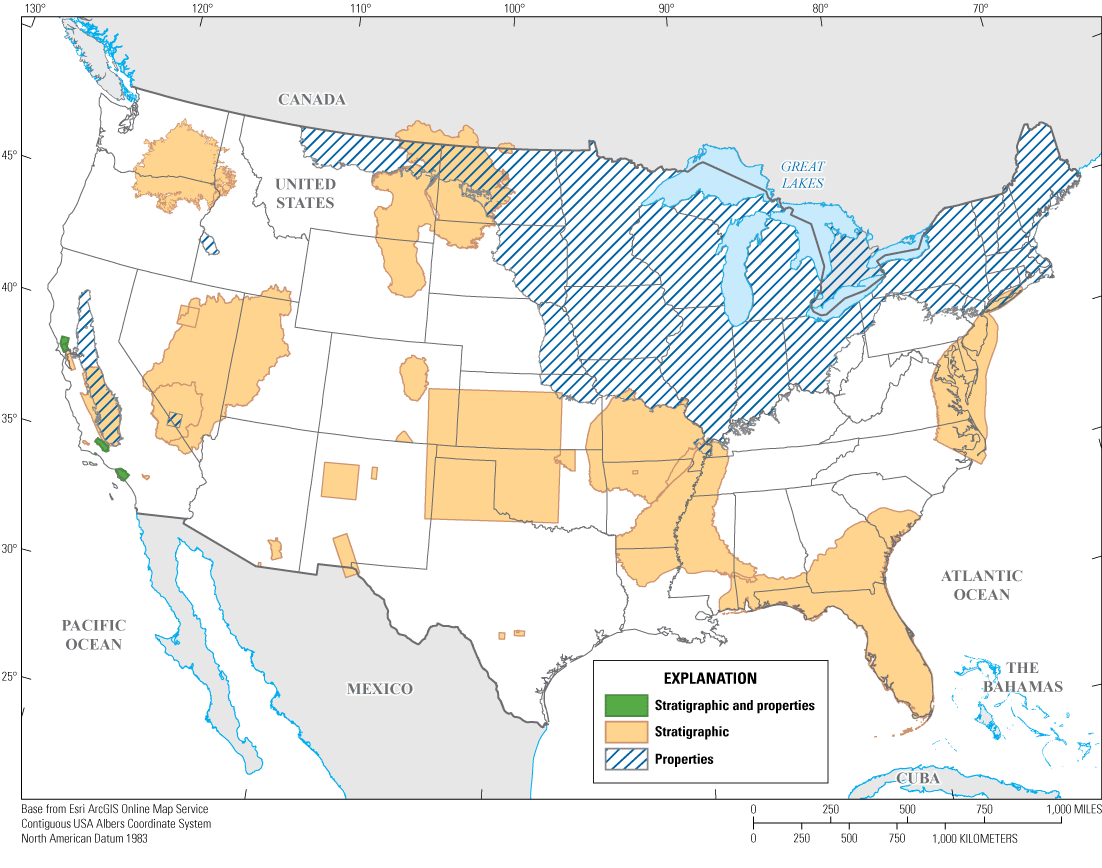
Spatial extent of inventoried U.S. Geological Survey three-dimensional geological models in the conterminous United States symbolized by model type (polygon boundary data from sources in Sweetkind and Zellman [2022]).
Model report or 3D model data release publication dates used in this inventory are from 2004 to 2022; USGS Mission Areas produced between 0 and 3 of these publications per year (fig. 2). The USGS Water Resources Mission Area was the most prolific publisher of 3D models during this time (n=23), followed by the USGS NCGMP (n=15), the USGS Energy Resources Program (n=2), the USGS Minerals Resources Program (n=1), and the USGS–U.S. Department of Energy Yucca Mountain Project (n=1). The USGS has produced 3D geologic framework model reports from a series of investigations at Yucca Mountain, Nev., the Nevada Nuclear Security Site (formerly the Nevada Test Site), Nev., and the surrounding Death Valley regional groundwater flow system in Nevada and California (D’Agnese and others, 1997; Belcher and Sweetkind, 2010; Belcher and others, 2017; Halford and Jackson, 2020). These investigations were combined into a single entry, shown as the USGS–U.S. Department of Energy Yucca Mountain Project (fig. 2), that corresponds to the only digital data release from a 3D geologic framework model for this region (Faunt, 2006).

The number of three-dimensional geologic models that each U.S. Geological Survey (USGS) Mission Area and the USGS–U.S. Department of Energy (DOE) Yucca Mountain Project published by year (2004–22). NCGMP, National Cooperative Geologic Mapping Program.
Model Type
Twenty-nine of the 3D geologic framework models are stratigraphic models that depict geologic (n=14) or hydrogeologic (n=15) information (fig. 3). Four 3D models are properties models that use lithologic information from driller’s logs or interpretations from downhole electric logs to develop 3D textural models of grain-size variability. In five of the modeled regions, both a stratigraphic and properties model was created. The USGS Water Resources Mission Area and NCGMP produced the only properties models included in this inventory (fig. 3).
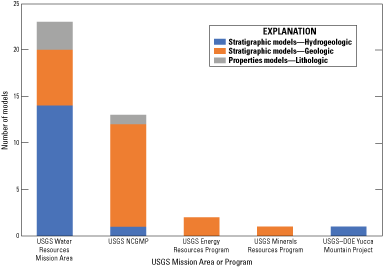
The number of three types of three-dimensional geologic models created by U.S. Geological Survey (USGS) Mission Areas or Programs. Models created jointly by more than one USGS Mission Area are plotted in the columns for separate Mission Areas such that the total number of models plotted is greater than the 38 inventoried models. NCGMP, National Cooperative Geologic Mapping Program; DOE, U.S. Department of Energy.
Model Purpose
Most of the 3D geologic framework models were created to support water-related studies, including aquifer delineation and characterization and water resource assessments (n=34). Other purposes include oil and gas assessments (n=2), minerals systems research (n=1), and geologic hazards (n=1).
Model Size
The 3D geologic models range in size from 30 to 1,582,460 km2 and the modeled volumes are divided into 1−27 layers (fig. 4). The model with the greatest area is not shown in figure 4 because this model is a one-layer properties model that covers the entire glaciated region of the northern conterminous United States (Bayless and others, 2017). Apart from this outlier, models with the smallest areas have a wide range in the number of layers represented in the model (3–22), whereas larger models with modeled area between 100,000 and 300,000 km2 generally have between 5 and 10 layers (fig. 4).
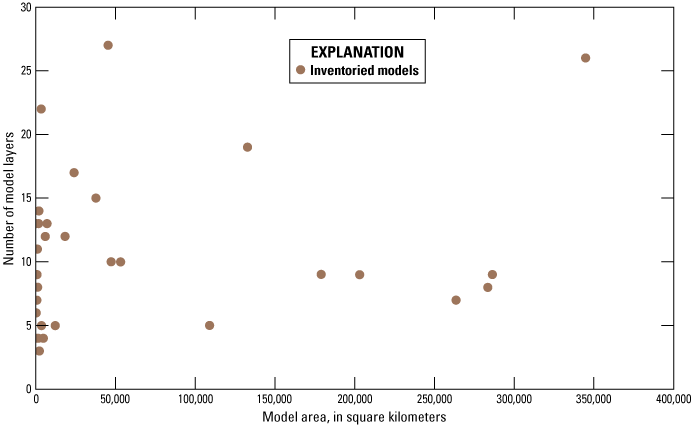
Relationship between the number of modeled layers and model area, in square kilometers, for inventoried three-dimensional geologic models. The one-layer properties model of Bayless and others (2017) that has an area of 1,582,460 square kilometers is not included on the plot to better display the relations among the remaining model data points.
Software Used
For 3D geologic model construction, EarthVision was used for 16 models, Esri GIS software was used for 10 models, RockWare was used for 5 models, and Roxar was used for 1 model. A hybrid of software platforms was used for the construction of 5 models: RockWare and Esri GIS (n=3), Stratamodel and Esri GIS (n=1), and EarthVision and Esri GIS (n=1). Esri GIS software was used for models in a wide range of areal dimensions (2,214–1,582,460 km2) and was more commonly used in models that cover large areas (fig. 5). EarthVision was primarily used for models that cover small areas (30–7,047 km2) apart from two outlier models of larger areal dimension (37,692 and 344,777 km2). RockWare was primarily used for models that cover small to midsize areas (3,407–23,931 km2) apart from one outlier with a much larger areal extent (286,308 km2). Hybrid models were used to model small to midsize areas (749–45,319 km2). The single model constructed with Roxar software was for a model of a small area (1,101 km2) and is not shown on figure 5.
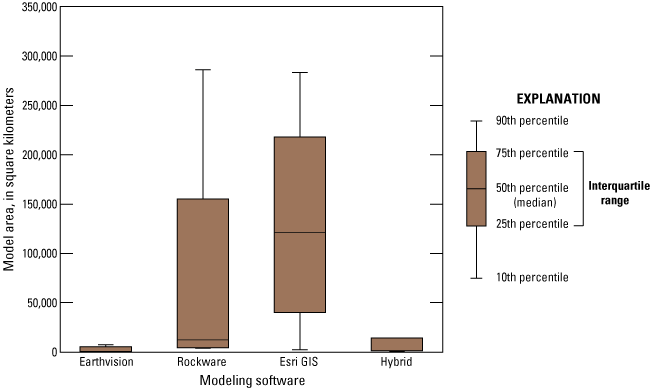
Relationship between type of modeling software used and the modeled area, in square kilometers. The lines extending from either side of the box set a boundary beyond which any data would be considered outliers. The one-layer properties model of Bayless and others (2017) that has an area of 1,582,460 square kilometers is not included on the plot as an outlier point to better display the relations among the remaining model data points. GIS, geographic information system.
Discussion
The 38 inventoried 3D geologic models represent a small sample size, and the apparent trends described in the “Results” section are likely heavily affected by the scope and purpose of individual USGS projects and the preferences of individual researchers. It is difficult to discuss any trends that may have programmatic or scientific significance until a larger sample set is available. This initial inventory is intended as one of the first steps toward a greater programmatic effort from the NCGMP to compile, synthesize, and distribute 3D geologic data, information, and models at varying scales (Brock and others, 2021). A logical next step is to inventory the 3D geologic models created by other Federal agencies, State geological surveys, and academic institutions. The structured nature of the data compiled in this inventory (Sweetkind and Zellman, 2022) is intended to facilitate future efforts to keep the inventory current and expand its scope as new 3D geologic models are added. Future activities may involve extracting geologic data, such as the elevation of formation tops, from these inventoried models and integrating the results into national-scale 3D maps and models.
Summary
This inventory assembles, in catalog form, the spatial footprint and salient characteristics of previously published U.S. Geological Survey (USGS) three-dimensional (3D) geological models where digital model output was released. Nonspatial data include citations of the original model publications and published model datasets so that users may easily find and access these products. The inventoried models vary widely in their spatial extent, type, purpose, number of characterized subsurface units, and software platforms. The result is a database of 38 spatial footprints and nonspatial tables with characteristics of 3D geologic models constructed by the USGS across all Mission Areas that have publication dates spanning from 2004 to 2022. This Data Report provides the scientific rationale for inventory and cataloging activities of published 3D geologic models. It is anticipated that this inventory may be expanded and periodically updated to serve as a definitive record of 3D geologic models produced throughout the USGS.
References Cited
Allison, L., 2014, AASG resolution on future directions of geologic mapping: State Geologists, June 20, 2014, accessed August 25, 2023, at https://stategeologists.blogspot.com/2014/06/.
Apel, M., 2006, From 3d geomodelling systems towards 3d geoscience information systems—Data model, query functionality, and data management: Computers and Geosciences, v. 32, no. 2, p. 222–229. [Also available at https://doi.org/10.1016/j.cageo.2005.06.016.]
Aswar, D.S., and Ullagaddi, P.B., 2017a, An overview 3-D geological modelling part I—Basics of 3-D geological modelling: International Journal of Latest Engineering and Management Research, v. 2, no. 11, p. 1–14, accessed March 19, 2021, at http://www.ijlemr.com/v2-i11.html.
Aswar, D.S., and Ullagaddi, P.B., 2017b, An overview of 3-D geological modelling part II. Summary of major 3-D geological modelling methodologies: International Journal of Latest Engineering and Management Research, v. 2, no. 11, p. 15–27, accessed August 25, 2023, at http://www.ijlemr.com/v2-i11.html.
Aswar, D.S., and Ullagaddi, P.B., 2017c, An overview of 3-D geological modelling, part III software applications in 3-D geological modelling: International Journal of Latest Engineering and Management Research, v. 2, no. 11, p. 28–38, accessed August 25, 2023, at http://www.ijlemr.com/v2-i11.html.
Bayless, E.R., Arihood, L.D., Reeves, H.W., Sperl, B.J.S., Qi, S.L., Stipe, V.E., and Bunch, A.R., 2017, Maps and grids of hydrogeologic information created from standardized water-well drillers’ records of the glaciated United States: U.S. Geological Survey Scientific Investigations Report 2015–5105, 34 p. [Also available at https://doi.org/10.3133/sir20155105.]
Belcher, W.R., and Sweetkind, D.S., eds., 2010, Death Valley regional groundwater flow system, Nevada and California—Hydrogeologic framework and transient groundwater flow model: U.S. Geological Survey Professional Paper 1711, 398 p. [Also available at https://doi.org/10.3133/pp1711.]
Belcher, W.R., Sweetkind, D.S., Faunt, C.C., Pavelko, M.T., and Hill, M.C., 2017, An update of the Death Valley regional groundwater flow system transient model, Nevada and California: U.S. Geological Survey Scientific Investigations Report 2016–5150, 74 p., 1 pl. [Also available at https://doi.org/10.3133/sir20165150.]
Berg, R.C., and Keefer, D.A., 2004, Commentary—Three-dimensional geologic modeling—Challenging our terminology and our understanding of geologic maps, in Three-dimensional geological mapping for groundwater applications—Workshop extended abstracts: Illinois State Geological Survey Open-File Series 2004–8, p. 4–9, accessed March 6, 2023, at https://library.isgs.illinois.edu/Pubs/pdfs/ofs/2004/ofs2004-08.pdf.
Berg, R.C., Mathers, S.J., Kessler, H., and Keefer, D.A., eds., 2011, Synopsis of current three-dimensional geological mapping and modeling in geological survey organizations: Illinois State Geological Survey Circular 578, 104 p., accessed March 6, 2023, at https://www.ideals.illinois.edu/items/43483.
Boyd, O.S., 2019a, 3D geologic framework for use with the U.S. Geological Survey National Crustal Model, phase 1—Western United States: U.S. Geological Survey Open-File Report 2019–1081, 36 p. [Also available at https://doi.org/10.3133/ofr20191081.]
Boyd, O.S., 2019b, 3D geologic framework for use with the U.S. Geological Survey National Crustal Model, phase 1—Western United States: U.S. Geological Survey data release, https://doi.org/10.5066/P9SBQENM.
Boyd, O.S., 2020, Calibration coefficients for the U.S. Geological Survey National Crustal Model and depth to water table: U.S. Geological Survey data release, https://doi.org/10.5066/P9GO3CP8.
Bristol, R.S., Euliss, N.H., Jr., Booth, N.L., Burkardt, N., Diffendorfer, J.E., Gesch, D.B., McCallum, B.E., Miller, D.M., Morman, S.A., Poore, B.S., Signell, R.P., and Viger, R.J., 2013, U.S. Geological Survey core science systems strategy—Characterizing, synthesizing, and understanding the critical zone through a modular science framework: U.S. Geological Survey Circular 1383–B, 33 p. [Also available at https://doi.org/10.3133/cir1383B.]
Brock, J., Berry, K., Faulds, J., Berg, R., House, K., Marketti, M., McPhee, D., Schmidt, K.M., Schmitt, J., Soller, D.R., Spears, D., Thompson, R.A., Thorleifson, H., and Walsh, G.J., 2021, Renewing the National Cooperative Geologic Mapping Program as the Nation’s authoritative source for modern geologic knowledge: U.S. Geological Survey Open-File Report 2021–1013, 10 p. [Also available at https://doi.org/10.3133/ofr20211013.]
Caumon, G., Lepage, F., Sword, C.H., and Mallet, J.-L., 2004, Building and editing a sealed geological model: Mathematical Geology, v. 36, no. 4, p. 405–424. [Also available at https://doi.org/10.1023/B:MATG.0000029297.18098.8a.]
Caumon, G., Collon-Drouaillet, P., Le Carlier de Veslud, C., Viseur, S., and Sausse, J., 2009, Surface-based 3D modeling of geological structures: Mathematical Geosciences, v. 41, p. 927–945. [Also available at https://doi.org/10.1007/s11004-009-9244-2.]
Cederberg, J.R., Sweetkind, D.S., Buto, S.G., and Masbruch, M.D., 2011, Appendix 1–Three-dimensional hydrogeologic framework, in Heilweil, V.M. and Brooks, L.E., eds., Conceptual model of the Great Basin carbonate and alluvial aquifer system: U.S. Geological Survey Scientific Investigations Report 2010–5193, p. 127–141. [Also available at https://doi.org/10.3133/sir20105193.]
Cole, J.C., Grauch, V.J.S., Hudson, M.R., Maldonado, F., Minor, S.A., Sawyer, D.A., and Stone, B.R., 1999, Three-dimensional geologic modeling of the middle Rio Grande basin, in Bartolino, J.R., ed., U.S. Geological Survey middle Rio Grande Basin Study—Proceedings of the third annual workshop, Albuquerque, New Mexico, February 24–25, 1999: U.S. Geological Survey Open-File Report 99–203, p. 25–26. [Also available at https://doi.org/10.3133/ofr99203.]
Cromwell, G., and Matti, J.C., 2022, Geology and hydrogeology of the Yucaipa groundwater subbasin, San Bernardino and Riverside Counties, California (ver. 1.1, May 2022): U.S. Geological Survey Scientific Investigations Report 2021–5129, 58 p. [Also available at https://doi.org/10.3133/sir20215129.]
Cronkite-Ratcliff, C., and Phelps, G.A., 2014, Stochastic modeling of a lava-flow aquifer system: U.S. Geological Survey Open-File Report 2014–1179, 18 p. [Also available at https://doi.org/10.3133/ofr20141179.]
Culshaw, M.G., 2005, From concept towards reality—Developing the attributed 3D geological model of the shallow subsurface: Quarterly Journal of Engineering Geology and Hydrogeology, v. 38, no. 3, p. 231–284. [Also available at https://doi.org/10.1144/1470-9236/04-072.]
D’Agnese, F.A., Faunt, C.C., Turner, A.K., and Hill, M.C., 1997, Hydrogeologic evaluation and numerical simulation of the Death Valley regional ground-water flow system, Nevada and California: U.S. Geological Survey Water-Resources Investigations Report 96–4300, 124 p. [Also available at https://pubs.usgs.gov/publication/wri964300.]
Evenson, E.J., Orndorff, R.C., Blome, C.D., Böhlke, J.K., Hershberger, P.K., Langenheim, V.E., McCabe, G.J., Morlock, S.E., Reeves, H.W., Verdin, J.P., Weyers, H.S., and Wood, T.M., 2013, U.S. Geological Survey water science strategy—Observing, understanding, predicting, and delivering water science to the Nation: U.S. Geological Survey Circular 1383–G, 49 p. [Also available at https://doi.org/10.3133/cir1383G.]
Faunt, C.C., 2006, Surface altitudes [and thicknesses] of hydrogeologic units used in the hydrogeologic framework and transient ground-water flow model of the Death Valley regional ground-water flow system, Nevada and California: U.S. Geological Survey data release, https://water.usgs.gov/GIS/metadata/usgswrd/XML/pp1711_hfm_top.xml.
Faunt, C.C., Sweetkind, D.S., and Belcher, W.R., 2010, Hydrogeologic framework model, chap. E of Belcher, W.R., and Sweetkind, D.S., eds., 2010, Death Valley regional ground-water flow system, Nevada and California—Hydrogeologic framework and transient ground-water flow model: U.S. Geological Survey Professional Paper 1711, p. 161–249, https://doi.org/10.3133/pp1711. [Supersedes USGS Scientific Investigations Report 2004–5205.]
Federal Geographic Data Committee, 2020, National spatial data infrastructure strategic plan—2021–2024: Reston, Va., Federal Geographic Data Committee, 17 p., accessed March 6, 2023, at https://www.fgdc.gov/nsdi-plan/nsdi-strategic-plan-2021-2024.pdf.
Ferrero, R.C., Kolak, J.J., Bills, D.J., Bowen, Z.H., Cordier, D.J., Gallegos, T.J., Hein, J.R., Kelley, K.D., Nelson, P.H., Nuccio, V.F., Schmidt, J.M., and Seal, R.R., 2013, U.S. Geological Survey energy and minerals science strategy—A resource lifecycle approach: U.S. Geological Survey Circular 1383–D, 37 p. [Also available at https://doi.org/10.3133/cir1383D.]
Gundersen, L.C.S., Belnap, J., Goldhaber, M., Goldstein, A., Haeussler, P.J., Ingebritsen, S.E., Jones, J.W., Plumlee, G.S., Thieler, E.R., Thompson, R.S., and Back, J.M., 2011, Geology for a changing world 2010–2020—Implementing the U.S. Geological Survey science strategy: U.S. Geological Survey Circular 1369, 68 p., accessed March 6, 2023, at https://pubs.usgs.gov/circ/circ1369/.
Halford, K.J., and Jackson, T.R., 2020, Groundwater characterization and effects of pumping in the Death Valley regional groundwater flow system, Nevada and California, with special reference to Devils Hole: U.S. Geological Survey Professional Paper 1863, 178 p. [Also available at https://doi.org/10.3133/pp1863.]
Hanson, R.T., Schmid, W., Faunt, C.C., Lear, J., and Lockwood, B., 2014, Integrated hydrologic model of Pajaro Valley, Santa Cruz and Monterey Counties, California: U.S. Geological Survey Scientific Investigations Report 2014–5111, 166 p., accessed March 6, 2023, at https://doi.org/10.3133/sir20145111.
Hudson, M.R., Smith, D.V., Pantea, M.P., and Becker, C.J., 2016, Geologic and geophysical models for Osage County, Oklahoma, and implications for groundwater resources: U.S. Geological Survey Scientific Investigations Report 2016–5067, 27 p. [Also available at https://doi.org/10.3133/sir20165067.]
Jacobsen, L.J., Glynn, P.D., Phelps, G.A., Orndorff, R.C., Bawden, G.W., and Grauch, V.J.S., 2011, U.S. Geological Survey—A synopsis of three-dimensional modeling, chap. 13 of Berg, R.C., Mathers, S.J., Kessler, H., and Keefer, D.A., eds., Synopsis of current three-dimensional geological mapping and modeling in geological survey organizations: Illinois State Geological Survey Circular 578, p. 69–79, accessed March 6, 2023 at https://www.ideals.illinois.edu/items/43483.
Kaufmann, O., and Martin, T., 2008, 3D geological modelling from boreholes, cross-sections and geological maps, application over former natural gas storages in coal mines: Computers and Geosciences, v. 34, no. 3, p. 278–290. [Also available at https://doi.org/10.1016/j.cageo.2007.09.005.]
Keefer, D.A., Kessler, H., Cave, M., and Mathers, S.J., 2011, Major mapping and modeling issues, chap. 2 of Berg, R.C., Mathers, S.J., Kessler, H., and Keefer, D.A., eds., Synopsis of current three-dimensional geological mapping and modeling in geological survey organizations: Illinois State Geological Survey Circular 578, p. 6–10, accessed March 6, 2023, at https://www.ideals.illinois.edu/items/43483.
MacCormack, K.E., Berg, R.C., Kessler, H., Russell, H.A.J., and Thorleifson, L.H., eds., 2019, 2019 synopsis of current three-dimensional geological mapping and modelling in geological survey organizations: Alberta Energy Regulator/Alberta Geological Survey, AER/AGS Special Report 112, 307 p., accessed March 6, 2023, at https://ags.aer.ca/publication/spe-112.
Pantea, M.P., and Cole, J.C., 2004, Three-dimensional geologic framework modeling of faulted hydrostratigraphic units within the Edwards aquifer, northern Bexar County, Texas: U.S. Geological Survey Scientific Investigations Report 2004–5226, 13 p., 1 CD–ROM. [Also available at https://doi.org/10.3133/sir20045226.]
Phelps, G.A., Graymer, R.W., Jachens, R.C., Ponce, D.A., Simpson, R.W., and Wentworth, C.M., 2008, Three-dimensional geologic map of the Hayward fault zone, San Francisco Bay region, California: U.S. Geological Survey Scientific Investigations Map 3045, accessed March 6, 2023, at https://pubs.usgs.gov/sim/3045/.
Pool, D.R., Blasch, K.W., Callegary, J.B., Leake, S.A., and Graser, L.F., 2011, Regional groundwater-flow model of the Redwall-Muav, Coconino, and alluvial basin aquifer systems of northern and central Arizona: U.S. Geological Survey Scientific Investigations Report 2010–5180, v. 1.1, 101 p., accessed March 6, 2023, at https://pubs.usgs.gov/sir/2010/5180/.
Russell, H.A.J., de Kemp, E., and MacCormack, K.E., 2019, Overview of geological survey organizations contributions on modelling approaches, chap. 3 of MacCormack, K.E., Berg, R.C., Kessler, H, Russell, H.A.J., and Thorleifson, L.H., eds., 2019 synopsis of current three-dimensional geological mapping and modelling in geological survey organizations: Alberta Energy Regulator/Alberta Geological Survey, AER/AGS Special Report 112, p. 7–18, accessed March 6, 2023, at https://ags.aer.ca/publication/spe-112.
Shah, A.K., and Boyd, O.S., 2018, Depth to basement and thickness of unconsolidated sediments for the western United States—Initial estimates for layers of the U.S. Geological Survey National Crustal Model: U.S. Geological Survey Open-File Report 2018–1115, 13 p. [Also available at https://doi.org/10.3133/ofr20181115.]
Shelton, J., Andrews, W., Colgan, J., Johnstone, S., Soller, D., Berg, R., Sweetkind, D., Zellman, K., Brock, J., and Ritzel, K., 2022, The National Cooperative Geologic Mapping Program’s U.S. GeoFramework Initiative—Delivering a digital database of geologic map information by 2030: U.S. Geological Survey Fact Sheet 2022–3079, 2 p. [Also available at https://doi.org/10.3133/fs20223079.]
Siler, D.L., Faulds, J.E., Glen, J.M.G., Hinz, N.H., Witter, J.B., Blake, K., Queen, J., and Fortuna, M., 2019, Three-dimensional geologic map of the southern Carson Sink, Nevada, including the Fallon FORGE area: U.S. Geological Survey Scientific Investigations Map 3437, 22-p. pamphlet. [Also available at https://pubs.er.usgs.gov/publication/sim3437.]
Siler, D.L., Faulds, J.E., Hinz, N.H., and Queen, J.H., 2021, Three-dimensional geologic map of the Brady geothermal area, Nevada: U.S. Geological Survey Scientific Investigations Map 3469, 2 sheets, 20-p. pamphlet, accessed March 6, 2023, at https://doi.org/10.3133/sim3469.
Soller, D.R., and Berg, T.M., 2003, The National Geologic Map Database project—Overview and progress, in Soller, D.R., ed., Digital mapping techniques ‘03—Workshop proceedings: U.S. Geological Survey Open-File Report 03–471, p. 57–77, accessed March 6, 2023, at https://doi.org/10.3133/ofr03471.
Sweetkind, D.S., 2017, Three-dimensional hydrogeologic framework model of the Rio Grande transboundary region of New Mexico and Texas, USA, and northern Chihuahua, Mexico: U.S. Geological Survey Scientific Investigations Report 2017–5060, 49 p. [Also available at https://doi.org/10.3133/sir20175060.]
Sweetkind, D., Graymer, R., Higley, D., and Boyd, O., 2019, Status of three-dimensional geological mapping and modelling activities in the U.S. Geological Survey, chap. 26 of MacCormack, K.E., Berg, R.C., Kessler, H., Russell, H.A.J., and Thorleifson, L.H., eds., 2019 synopsis of current three-dimensional geological mapping and modelling in geological survey organizations: Alberta Energy Regulator/Alberta Geological Survey, AER/AGS Special Report 112, p. 278–289, accessed March 6, 2023, at https://ags.aer.ca/publication/spe-112.
Sweetkind, D.S., and Zellman, K.L., 2022, Spatial data from an inventory of U.S. Geological Survey three-dimensional geologic models, volume 1, 2004–2022: U.S. Geological Survey data release, https://doi.org/10.5066/P9OFRNUN.
Turner, A.K., 2005, Challenges and trends for geological modelling and visualization: Bulletin of Engineering Geology and the Environment, v. 65, p. 109–127. [Also available at https://doi.org/10.1007/s10064-005-0015-0.]
Turner, A.K., Kessler, H., and van der Meulen, M.J., eds., 2021, Applied multidimensional geological modeling—Informing sustainable human interactions with the shallow subsurface: Hoboken, N.J., Wiley-Blackwell, 644 p. [Also available at https://doi.org/10.1002/9781119163091.]
U.S. Congress, 2019, Department of the Interior, environment, and related agencies appropriations bill, 2020: U.S. Congress H.R. 116–100, 237 p., accessed March 2, 2023, at https://www.congress.gov/congressional-report/116th-congress/house-report/100/1.
U.S. Geological Survey [USGS], 2007, Facing tomorrow’s challenges—U.S. Geological Survey science in the decade 2007–2017: U.S. Geological Survey Circular 1309, 67 p. [Also available at https://doi.org/10.3133/cir1309.]
Publishing support provided by the Science Publishing Network,
Denver Publishing Service Center
For more information concerning the research in this report, contact the
Center Director, USGS Geosciences and Environmental Change Science Center
Box 25046, Mail Stop 980
Denver, CO 80225
(303) 236-5344
Or visit the Geosciences and Environmental Change Science Center website at https://www.usgs.gov/centers/gecsc
Disclaimers
Any use of trade, firm, or product names is for descriptive purposes only and does not imply endorsement by the U.S. Government.
Although this information product, for the most part, is in the public domain, it also may contain copyrighted materials as noted in the text. Permission to reproduce copyrighted items must be secured from the copyright owner.
Suggested Citation
Sweetkind, D.S., and Zellman, K.L., 2023, An inventory of three-dimensional geologic models—U.S. Geological Survey, 2004–22: U.S. Geological Survey Data Report 1183, 13 p., https://doi.org/10.3133/dr1183.
ISSN: 2771-9448 (online)
Study Area
Publication type | Report |
---|---|
Publication Subtype | USGS Numbered Series |
Title | An inventory of three-dimensional geologic models—U.S. Geological Survey, 2004–22 |
Series title | Data Report |
Series number | 1183 |
DOI | 10.3133/dr1183 |
Year Published | 2023 |
Language | English |
Publisher | U.S. Geological Survey |
Publisher location | Reston VA |
Contributing office(s) | Geosciences and Environmental Change Science Center |
Description | Report: iv, 13 p.; Data Release |
Country | United States |
Online Only (Y/N) | Y |