Geologic Energy Storage
Links
- Document: Report (6.57 MB pdf) , HTML , XML
- Project Site: Geologic Energy Storage (html)
- Download citation as: RIS | Dublin Core
Introduction
As the United States transitions away from fossil fuels, its economy will rely on more renewable energy. Because current renewable energy sources sometimes produce variable power supplies, it is important to store energy for use when power supply drops below power demand. Battery storage is one method to store power. However, geologic (underground) energy storage may be able to retain vastly greater quantities of energy over much longer durations compared to typical battery storage. Geologic energy storage also has high flexibility; many different types of materials can be used to store chemical, thermal, or mechanical energy in a variety of underground settings. The U.S. Geological Survey (USGS) has the capability to research and assess possible domestic geologic energy storage resources to help prepare the United States for the future of renewable energy.
What is Geologic Energy Storage?
The term ‘geologic energy storage’ describes storing excess energy in underground settings such as rock formations. Storage of energy for later use is needed to supply seasonal demand, ensure strategic stockpiles, or provide baseload power when renewable energy sources are variable. Much of the technology for geologic energy storage is still undergoing research and development (Crotogino and others, 2017; Matos and others, 2019), although several industrial-sized underground storage projects are already operating in the United States and worldwide (fig. 1).
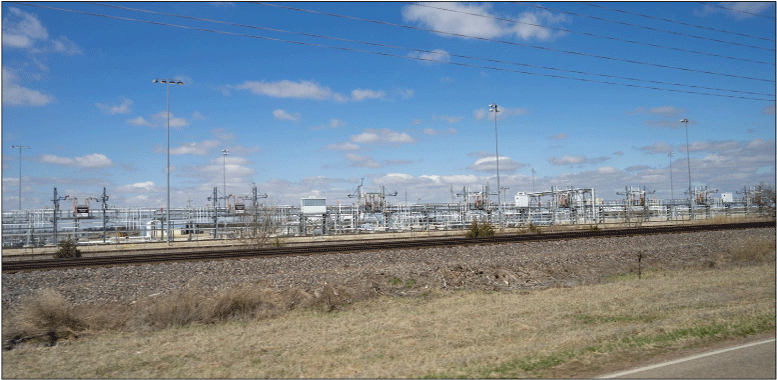
Example of above-ground infrastructure in Reno County, Kansas, for a natural gas storage cavern hundreds of feet deep in a salt formation. Photograph by Marc L. Buursink, U.S. Geological Survey.
-
Chemical methods, where energy is stored as potential energy in chemical bonds. These methods include storage of methane or natural gas, natural gas liquids, and hydrogen.
-
Mechanical methods, where energy is stored as potential energy using materials or fluids. These methods include compressed air energy storage, with constant or variable temperatures; gravity energy storage using suspended loads; and pumped hydroelectric energy storage.
-
Thermal methods, where energy is stored as a temperature difference in materials or fluids to be used later for heating, cooling, or industrial processes such as drying.
The methods, along with the different underground storage settings, are shown in figure 2.
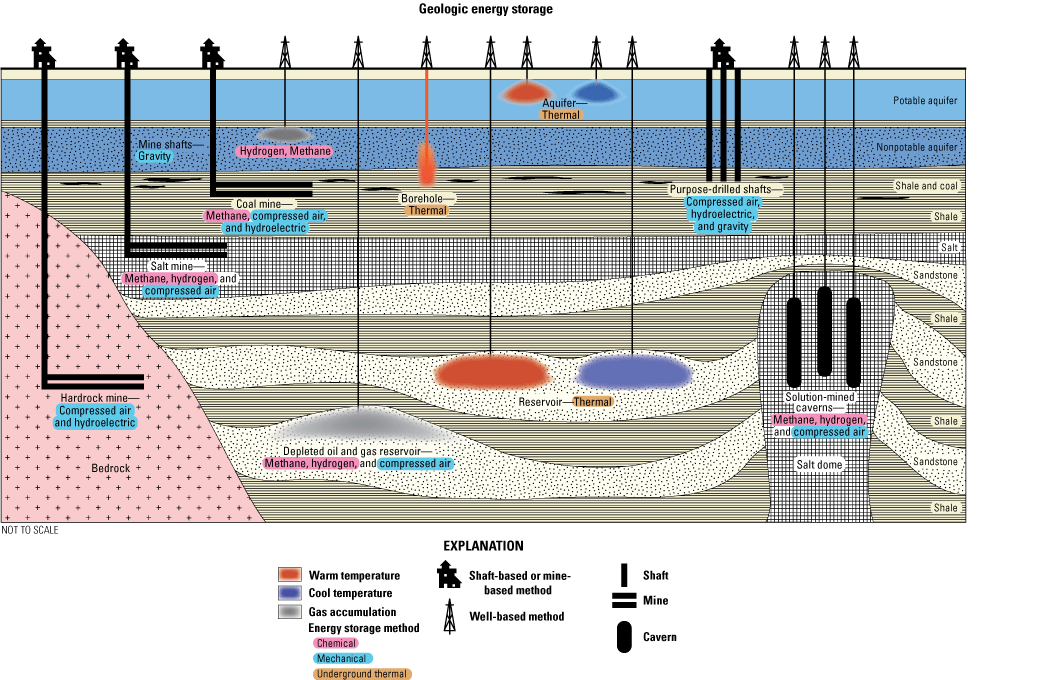
Schematic cross section showing examples of chemical, mechanical, and thermal geologic energy storage methods in potential underground settings in a sedimentary basin.
Some applications may use natural, permeable rock formations, but others rely on new or existing resource-extraction activities, such as mining or gas production.Different geologic settings for energy storage include the following:
-
Depleted or abandoned gas reservoirs;
-
Abandoned mine tunnels and shafts, both lined and unlined;
-
Purpose-drilled boreholes or shafts;
-
Mined caverns in salt formations; and
-
Freshwater or saline aquifers.
Most of these geologic settings could be used for more than one form of energy storage (fig. 2). Nevertheless, regulations enforced at the State and national level, particularly regarding geologic pore space ownership (Gresham and Anderson, 2011; Schremmer, 2021) and potential effects on groundwater for drinking (40 CFR part 144), may apply to these settings. These restrictions and grid infrastructure can affect site selection and economic risk.
Can Geologic Energy Storage Help with the Energy Transition?
Energy production and consumption in the United States is undergoing a transition from primarily fossil fuels to a mixture that includes greater shares of renewable sources and nuclear energy. Battery storage installations have a short start-up time to deliver power along with relatively short duration and small capacity. In comparison, geologic energy storage methods can retain vastly greater quantities of energy over much longer time periods (fig. 3), although power start-up times are not as fast (Aneke and Wang, 2016). An electrical grid that uses long duration energy storage projects with over 100 hours of stored power could result in the greatest reduction in electricity costs (Sepulveda and others, 2021). Geologic energy storage is a practical solution that can store 100 or more hours of energy. Batteries are primarily designed for storing electrical energy, but geologic storage methods have an advantage of being able to store chemical and thermal energy (for space heating, for example) directly without conversion to electricity. In addition, chemical and thermal energy storage would not depend on critical minerals used in batteries, which may be in short supply. All these characteristics make geologic energy storage an important approach for an energy transition in the United States.
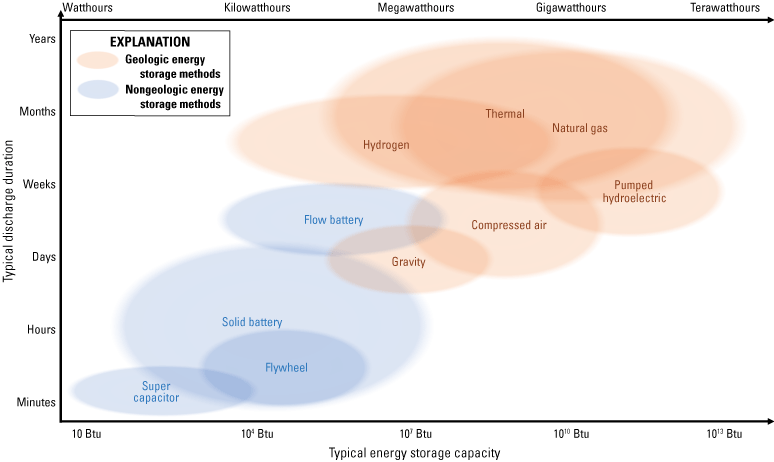
Graph of typical energy storage capacity compared to typical discharge duration for various geologic and nongeologic energy storage methods. Oval sizes are estimated based on current technology. Modified from Crotogino and others (2017) and Matos and others (2019). Btu, British thermal unit.
May Hydrogen be Stored Underground?
Hydrogen (either as a gas, liquid, or within another molecule like ammonia) may store a substantial amount of chemical energy. The subsequent use of that energy through electrical fuel cells or combustion is relatively clean compared to fossil fuel usage (Office of Energy Efficiency and Renewable Energy, 2022). Because of these clean applications, there is substantial research focused on hydrogen storage. Hydrogen may be stored underground primarily in solution-mined salt caverns and depleted gas reservoirs, though other storage settings are possible. Because the small hydrogen molecule easily travels through permeable rock and it can chemically interact with its surroundings, additional screening criteria are important when evaluating potential storage sites. These criteria include the sealing capacity of the cap rock above the storage formation, and potential geochemical reactions between the hydrogen and the host rock or in-place groundwater. Further considerations include underground microbial interactions, which could potentially convert some of the hydrogen into methane and lower the storage efficiency.
Hydrogen may be produced in different ways, including from renewable and nonrenewable energy sources. However, the resulting hydrogen produced from these methods is not expected to affect the amount of hydrogen that can be stored in a geologic setting.
How Can the U.S. Geological Survey Help with Energy Storage Research?
The USGS acquires and communicates scientific information needed to assess geologic energy resources. In 2018, a National Academies of Sciences, Engineering, and Medicine report (National Academies of Sciences, Engineering, and Medicine, 2018, p. 101) found that “Assessing the storage potential for various basins in the United States could become a new and strategically important priority for the ERP [U.S. Geological Survey Energy Resources Program].” For many years, USGS scientists have contributed to a growing body of knowledge on undiscovered hydrocarbon, geothermal energy, and carbon sequestration resources. The USGS has the ability to investigate geologic, geophysical, and geochemical characteristics of underground energy projects. This USGS expertise may be applied to assess potential domestic geologic energy storage resources. Any follow-on economic or engineering analysis may be considered after the assessment.
Initial work on a USGS assessment of geologic energy storage could focus on natural gas and hydrogen (chemical), compressed air and solid-mass gravity (mechanical), and geothermal energy (thermal) storage methods (table 1). Table 1 shows likely combinations of geologic energy storage methods and geologic settings for these initial assessments. Additional combinations of storage methods and geologic settings (fig. 2) could be assessed in the future to estimate total storage resources for a given area. For each geologic setting, the USGS would apply screening criteria, some of which may include storage depth, cavern spacing, host rock properties, and groundwater characteristics. These input parameters and any corresponding uncertainties may be quantified in a peer-reviewed resource assessment methodology developed by the USGS.
Table 1.
Summary of currently deployed or likely combinations of geologic energy storage methods and settings in the United States.[X, storage possible; —, not applicable]
Development of this assessment methodology could help with consistent delineation of storage capacities across the United States. Ultimately, results from a resource assessment by the USGS may inform public and private stakeholders and aid decisions by policymakers and tribal leaders in geographic areas of the United States that could support geologic energy storage.
References Cited
Aneke, M., and Wang, M., 2016, Energy storage technologies and real life applications—A state of the art review: Applied Energy, v. 179, p. 350–377, accessed November 10, 2022, at https://doi.org/10.1016/j.apenergy.2016.06.097.
National Academies of Sciences, Engineering, and Medicine, 2018, Future directions for the U.S. Geological Survey's Energy Resources Program: The National Academies Press, Washington, D.C., 156 p., accessed January 31, 2022, at https://doi.org/10.17226/25141.
Crotogino, F., Schneider, G.-S., and Evans, D.J., 2017, Renewable energy storage in geological formations: Proceedings of the Institution of Mechanical Engineers, Part A: Journal of Power and Energy, v. 232, no. 1, p. 100–114, accessed January 31, 2022, at https://doi.org/10.1177/0957650917731181.
Gresham, R.L., and Anderson, O.L., 2011, Legal and commercial models for pore-space access and use for geologic CO2 sequestration: University of Pittsburgh Law Review, v. 72, no. 4, accessed January 31, 2022, at https://doi.org/10.5195/lawreview.2011.170.
Matos, C.R., Carneiro, J.F., and Silva, P.P., 2019, Overview of large-scale underground energy storage technologies for integration of renewable energies and criteria for reservoir identification: Journal of Energy Storage, v. 21, p. 241–258, accessed January 31, 2022, at https://doi.org/10.1016/j.est.2018.11.023.
Office of Energy Efficiency and Renewable Energy, 2022, Hydrogen fuel basics—Hydrogen and Fuel Cell Technologies Office: U.S. Department of Energy web page, accessed November 10, 2022, at https://www.energy.gov/eere/fuelcells/hydrogen-fuel-basics.
Schremmer, J.A., 2021, Pore space property: Utah Law Review, vol. 2021, no. 1, article 1, p. 1–72, accessed January 31, 2022, at https://doi.org/10.26054/0D-SPTA-CFJW.
Sepulveda, N.A., Jenkins, J.D., Edington, A., Mallapragada, D.S., and Lester, R.K., 2021, The design space for long-duration energy storage in decarbonized power systems: Nature Energy, v. 6, no. 5, p. 506–516, accessed January 31, 2022, at https://doi.org/10.1038/s41560-021-00796-8.
For more information, contact the Energy Resources Program at AskEnergyProgram@usgs.gov or visit https://www.usgs.gov/centers/gemsc/science/geologic-energy-storage.
Suggested Citation
Buursink, M.L., Anderson, S.T., Brennan, S.T., Burns, E.R., Freeman, P.A., Gallotti, J.S., Lohr, C.D., Merrill, M. D., Morrissey, E.A., Plampin, M.R., and Warwick, P.D., 2023, Geologic energy storage: U.S. Geological Survey Fact Sheet 2022–3082, 4 p., https://doi.org/10.3133/fs20223082.
ISSN: 2327-6932 (online)
Publication type | Report |
---|---|
Publication Subtype | USGS Numbered Series |
Title | Geologic energy storage |
Series title | Fact Sheet |
Series number | 2022-3082 |
DOI | 10.3133/fs20223082 |
Year Published | 2023 |
Language | English |
Publisher | U.S. Geological Survey |
Publisher location | Reston, VA |
Contributing office(s) | Geology, Energy & Minerals Science Center |
Description | 4 p. |
Online Only (Y/N) | Y |
Additional Online Files (Y/N) | N |
Google Analytic Metrics | Metrics page |