Potential Factors Controlling Benthic Algae in the Upper White River Basin, Colorado, 2018–21
Links
- Document: Report (6.12 MB pdf) , HTML , XML
- Related Works:
- USGS Scientific Investigations Report 2022-5112 Characterization of Streamflow and Nutrient Occurrence in the Upper White River Basin, Colorado, 1980–2020
- USGS Scientific Investigations Report 2023-5009 Investigation of Potential Factors Controlling Benthic Algae in the Upper White River Basin, Colorado, 2018–21
- Data Releases:
- USGS data release - Channel Characteristics, benthic algae, and water quality model data for selected sites in the upper White River Basin, Colorado, 2018-21
- USGS data release - USGS water data for the Nation: U.S. Geological Survey National Water Information System database
- Download citation as: RIS | Dublin Core
Introduction
Bottom-attached (benthic) algae grow on streambeds and use energy from sunlight to produce their own food through photosynthesis. Nuisance levels of benthic algae are becoming increasingly common in surface waters of the western United States (Oleksy and others, 2021) and can compromise aesthetic quality, limit recreational activities, block water infrastructure, and have negative effects on aquatic life (Dodds and others, 2009). In 2016, Colorado Parks and Wildlife identified Cladophora glomerata (green algae), a pervasive, nuisance aquatic algae, in the White River, located in western Colorado. In cooperation with the White River and Douglas Creek Conservation Districts, the Colorado River Basin Salinity Control Forum, and the Colorado River Water Conservation District, the U.S. Geological Survey (USGS) studied factors potentially controlling the occurrence of benthic algae (fig. 1) in the upper White River Basin (fig. 2) from 2018 through 2021. This fact sheet summarizes major findings of the study; detailed information is available in Day (2023) and Day and Henneberg (2023). Data are available in a USGS data release (Day and others, 2023).
For the USGS study, data collection and analysis were designed to test the following hypotheses:
-
1. streamflow-induced movement of the streambed during snowmelt runoff may change conditions that limit algal biomass, and
-
2. physical and chemical characteristics associated with streambed particle size, water temperature, light availability, and nutrient availability, will affect algal biomass.
Multiple approaches were used to assess nutrients and physical conditions in the upper White River Basin. Streamflow and nutrient data were retrieved from the USGS National Water Information System database (USGS, 2021) to characterize changes at water-quality sites monitored for more than 20 years. In addition, new data were collected at 20 water-quality sites (fig. 2) during the study period (2018–21). A linear mixed-effects model was used to evaluate the relative effect of different factors, on algal biomass.
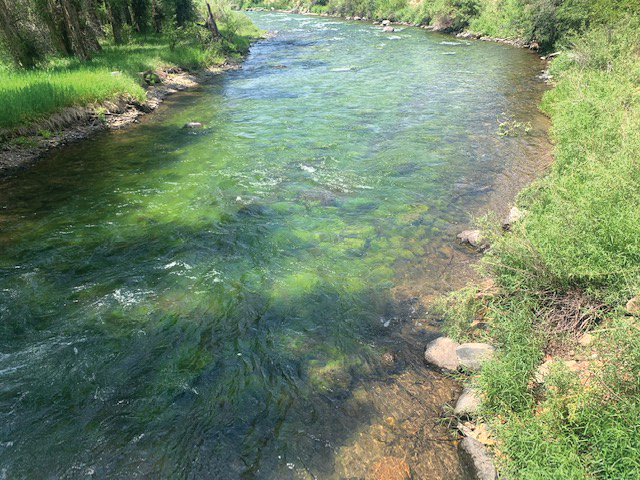
Benthic algae at North Fork White River at Buford, Colorado (water-quality site 8, fig. 2), in summer 2018. [Photograph by Natalie Day, U.S. Geological Survey.]
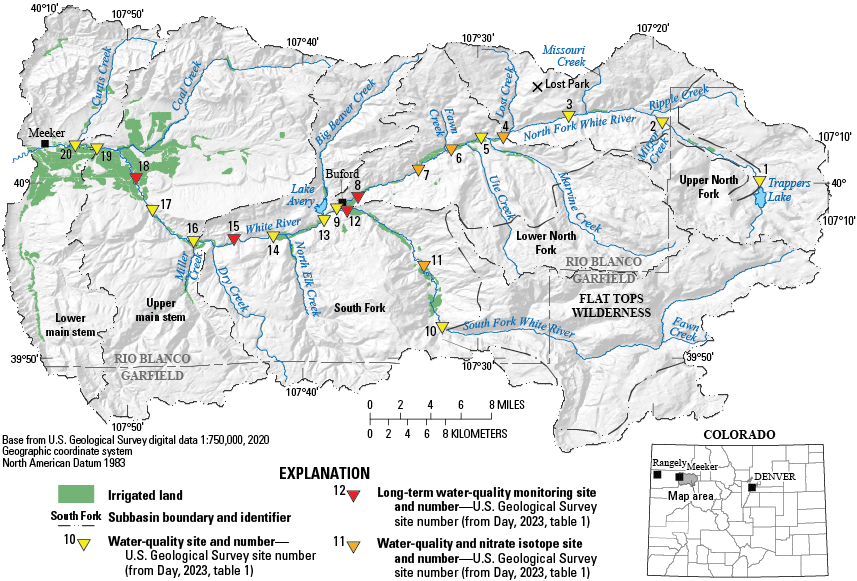
Location of the upper White River Basin, Colorado, with selected U.S. Geological Survey water-quality sites from Day (2023). Irrigated lands layer modified from Colorado Decision Support Systems (2020).
Benthic Algae in the Upper White River Basin
Benthic algal samples were collected during the summer from 2018 through 2021, and sampling targeted conditions when benthic algal biomass was the greatest. Algal biomass (chlorophyll-a) ranged between 0.7 and 309 milligrams per square meter (mg/m2), and the greatest median concentrations were measured at North Fork White River at Buford (site 8; 51 mg/m2) and at White River above Dry Creek (site 15; 50 mg/m2) (figs. 2 and 3; refer to table 1 from Day [2023] and Day and Henneberg [2023] for list of USGS site names and abbreviated site names and numbers). In general, algal biomass was greatest in 2018 (fig. 3D).
The types of algae present in the study area were characterized in 2020 and 2021. Four genera of filamentous green algae were identified: Cladophora, Stigeoclonium, Ulothrix, and Spirogyra. Many genera of cyanobacteria were present, including some capable of producing toxins and taste and odor compounds.
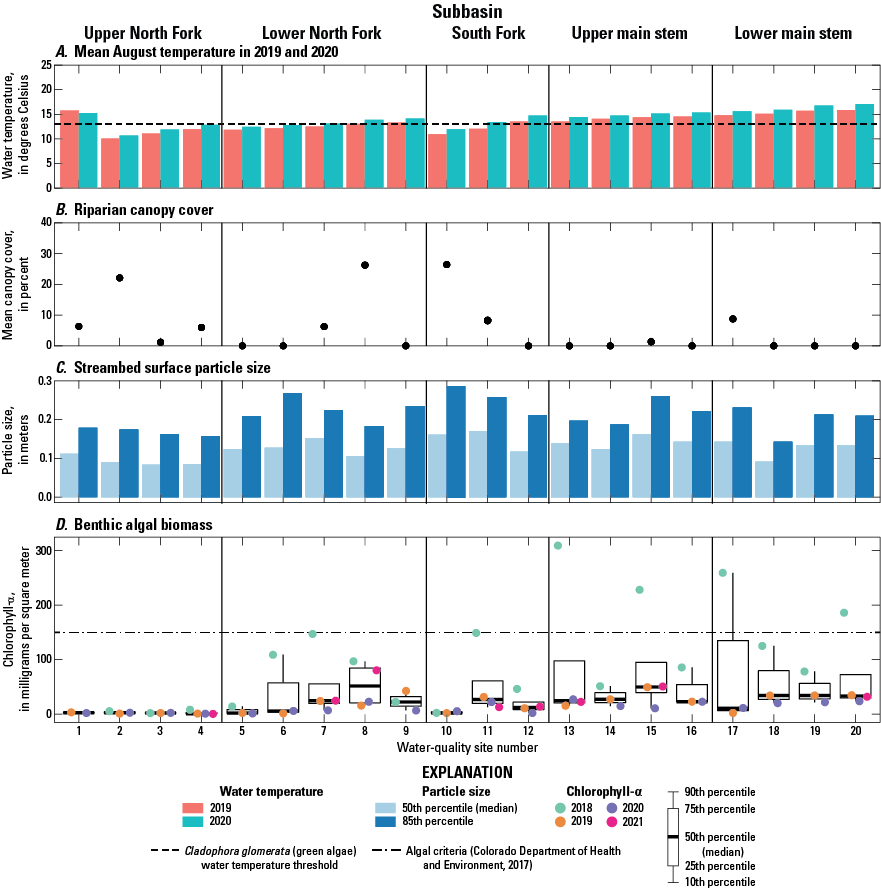
A, mean August water temperature in 2019 and 2020 (data from Hodge and Eyre, 2021); B, mean riparian canopy cover; C, streambed surface particle size; and D, chlorophyll-a (benthic algal biomass) at 20 water-quality sites in 5 subbasins of the upper White River Basin, Colorado. Graph from Day and Henneberg, 2023.
Streamflow
The seasonal timing, magnitude, and duration of high and low streamflows can affect the growth and accumulation of benthic algae (fig. 4). In the upper White River Basin, peak streamflow is driven by snowmelt runoff in late spring or early summer. High streamflow during spring runoff can reduce benthic algal abundance by physical disturbance through scouring or streambed reorganization (Power and Stewart, 1987). Streamflow also affects water-quality conditions such as water temperature, light availability, and nutrient loading and dilution (Rolls and others, 2012; Piggott and others, 2015; Schneider, 2015). Snowmelt-derived runoff is cold and has elevated turbidity, which limits light availability to benthic algae. Low streamflow during the summer can result in warmer temperatures favorable to algae. During years with earlier snowmelt runoff, warm water temperatures earlier in the summer can extend the growing season for algae, leading to higher accumulation of benthic algae (Schneider, 2015).
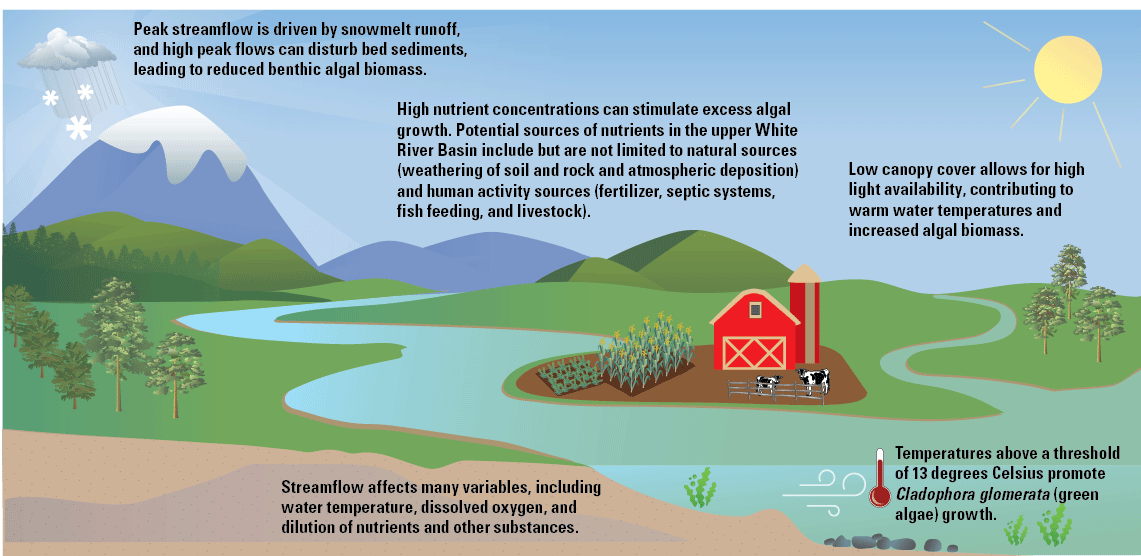
Potential factors controlling the growth of benthic algae in the upper White River Basin, Colorado, 2018–21.
Using streamflow data from 1980 through 2020, significant decreases in August and September streamflow were identified for White River above Coal Creek (site 18; fig. 2). Decreases in May and June streamflows and corresponding increases in April may indicate a shift toward earlier snowmelt runoff (Day, 2023).
Field measurements were used to assess if the streambed moved during peak runoff in 2018, 2019, and 2020 using the modified critical shear method (U.S. Department of Agriculture Forest Service, 2008). Results indicated movement of the median particle size at zero sites in 2018, seven sites in 2019, and two sites in 2020. Results from the linear mixedeffects model show larger median particle sizes were associated with greater algal biomass. Large particles provide stable areas for algae to establish and can increase algal settlement rates by slowing the velocity of oncoming water (Burkholder, 1996).
Nutrients
Nutrients, including nitrogen and phosphorus, are essential substances promoting the growth and accumulation of algae. Nutrients can occur in the environment through weathering of rock or soil, breakdown of organic material, atmospheric deposition, and human activities (Hem, 1985; Murphy and Sprague, 2019; Stets and others, 2020).
Data from 2000 through 2020 for North Fork White River at Buford, South Fork White River at Buford, White River above Dry Creek, and White River above Coal Creek (sites 8, 12, 15, and 18; fig. 2) were used to assess seasonal patterns and changes in nutrient concentrations and loads. Phosphorus concentrations and loads increased through time, while nitrogen concentrations and loads decreased. A relatively uniform increase in total phosphorus between the North and South Forks of the White River indicates the factors contributing to the increases may be regional in scope. In fact, similar increases in phosphorus were observed nearby in the Yampa River (Day, 2021). Changes in the relative availability of nitrogen to phosphorus, represented by the molar ratio of nitrogen to phosphorus, show conditions in the North and South Forks of the White River changed from colimited to nitrogen limited starting around 2012. Results from the linear mixed-effects model show that more nitrogen-limiting conditions (greater phosphorus availability) corresponded to greater algal biomass.
A synoptic sampling was completed in October 2020 to identify the relative magnitude of nutrient inputs from potential source areas. The results represent nutrient loading conditions at the time of sampling and are not meant to explain the long-term changes in nutrients throughout the basin. This effort identified the lower North Fork subbasin as having a large phosphorus yield (load divided by subbasin area). Loads of total phosphorus and orthophosphate were elevated at North Fork White River below Marvine Creek (site 5; fig. 2). An elevated total nitrogen concentration and load occurred between the two most downstream sites on the White River (water-quality sites 19 and 20, fig. 2), likely driven by inputs of organic nitrogen.
Water Temperature
Variation in the magnitude and timing of snowmelt runoff led to interannual variation in water temperature. In general, Cladophora glomerata growth is promoted at water temperatures above a threshold of 13 degrees Celsius (Graham and others, 1982). The threshold was exceeded by early to mid-June in 2018, 2020, and 2021. The threshold was not reached until July in 2019, reflecting high streamflow and an extended period of snowmelt runoff. Results from the linear mixed-effects model indicate higher water temperatures were associated with greater benthic algal biomass; all water-quality sites with mean August water temperatures below the Cladophora glomerata threshold (dashed line, fig. 3A) had consistently low algal biomass (fig. 3D). Water temperatures were warmer at downstream water-quality sites, corresponding with higher algal biomass.
Riparian Canopy Cover
Riparian canopy cover affects the amount of sunlight reaching surface water. Low canopy cover, and therefore high light availability, promotes warmer temperatures and increased algal biomass (Warnaars and others, 2007). Canopy cover at water-quality sites in the upper White River Basin was low and ranged from 0 to 26 percent (fig. 3B).
Conclusions
The broad spatial extent of algae indicates that the factors contributing to algal blooms are likely of basin-wide proportion rather than one sole factor. The frequency and severity of algal blooms in the upper White River Basin may be affected by long-term changes in nutrient availability and streamflow, specifically changes in the timing and magnitude of high and low streamflow. The effects of large peak streamflow, including movement of the streambed, may be the dominant control on the occurrence of algal blooms through years. Large, late, and long-lasting peak streamflow, such as in 2019, may limit algal blooms during the year in which they occur and into subsequent years, as evidenced by low algal biomass in 2019 and 2020, despite warm water temperatures, low summer streamflow, and lack of streambed movement in 2020. Without streambed disturbance, other factors such as nutrients and water temperature may have a larger effect on algal accumulation.
For More Information
-
Director, USGS Colorado Water Science Center
-
Box 25046, Mail Stop 415
-
Denver, CO 80225
-
For additional information, visit: https://www.usgs.gov/centers/colorado-water-science-center
References Cited
Colorado Decision Support Systems, 2020, Division 5 irrigated lands: Colorado’s Decision Support Systems, accessed June 2021, at https://cdss.colorado.gov/gis-data/division-5-colorado.
Colorado Department of Health and Environment, 2017, Water Quality Control Commission, Regulation no. 31—The basic standards and methodologies for surface water: Colorado Water Quality Control Commission, 230 p., accessed September 3, 2019, at https://www.colorado.gov/pacific/cdphe/clean-waternutrients.
Day, N.K., 2021, Input and output data from streamflow and water-quality regression models used to characterize streamflow and water-quality conditions in the Upper Yampa River Basin, Colorado, from 1992 to 2018: U.S. Geological Survey data release, https://doi.org/10.5066/P9L7S3NQ.
Day, N.K., Gidley, R.G., and Henneberg, M.F., 2023, Channel characteristics, benthic algae, and water quality model data for selected sites in the upper White River Basin, Colorado, 2018–21: U.S. Geological Survey data release, https://doi.org/10.5066/P9E82RMQ.
Dodds, W.K., Bouska, W.W., Eitzmann, J.L., Pilger, T.J., Pitts, K.L., Riley, A.J., Schloesser, J.T., and Thornbrugh, D.J., 2009, Eutrophication of U.S. freshwaters—Analysis of potential economic damages: Environmental Science & Technology, v. 43, p. 12−19, accessed June 2021, at https://doi.org/10.1021/es801217q.
Graham, J.M., Auer, M.T., Canale, R.P., and Hoffmann, J.P., 1982, Ecological studies and mathematical modeling of Cladophora in Lake Huron—4. Photosynthesis and respiration as functions of light and temperature: Journal of Great Lakes Research, v. 8, no. 1, p.100−111, accessed June 2021, at https://doi.org/10.1016/S0380-1330(82)71948-3.
Hem, J.D., 1985, Study and interpretation of the chemical characteristics of natural water, 3d. ed.: U.S. Geological Survey Water-Supply Paper 2254, accessed February 2020, at https://pubs.usgs.gov/wsp/wsp2254/.
Hodge, B.W., and Eyre, T., 2021, Stream temperatures in the forks and main stem of the White River in northwest Colorado (2019–2020): Trout Unlimited and Colorado Parks and Wildlife, accessed April 1, 2021, at https://wrcd-dccd.colorado.gov/sites/wrcd-dccd/files/documents/stream_temps_in_upper_white_river_-_hodge-eyre.pdf.
Murphy, J., and Sprague, L., 2019, Water-quality trends in US rivers—Exploring effects from streamflow trends and changes in watershed management: Science of the Total Environment, v. 656, p. 645–658, accessed March 2021, at https://doi.org/10.1016/j.scitotenv.2018.11.255.
Oleksy, I.A., Baron, J.S., and Beck, W.S., 2021, Nutrients and warming alter mountain lake benthic algal structure and function: Freshwater Science, v. 40, accessed March 2021, at https://doi.org/10.1086/713068.
Power, M.E. and Stewart, A.J., 1987, Disturbance and recovery of an algal assemblage following flooding in an Oklahoma stream: The American Midland Naturalist, v. 117, no. 2, p. 333–345, accessed August 2021, at https://www.jstor.org/stable/2425975?seq=1#metadata_info_tab_contents. https://doi.org/10.2307/2425975.
Rolls, R.J., Leigh, C., and Sheldon, F., 2012, Mechanistic effects of low-flow hydrology on riverine ecosystems—Ecological principles and consequences of alteration: Freshwater Science, v. 31, no. 4, p. 1163–1186, accessed April 2021, at https://doi.org/10.1899/12-002.1
Schneider, S.C., 2015, Greener rivers in a changing climate? Effects of climate and hydrological regime on benthic algal assemblages in pristine streams: Limnologica, v. 55, p. 21–32, accessed September 2021, at https://doi.org/10.1016/j.limno.2015.10.004.
Stets, Edward G., Sprague, L.A., Oelsner, G.P., Johnson, H.M., Murphy, J.C., Ryberg, Karen, Vecchia, A.V., Zuellig, R.E., Falcone, J.A., and Riskin, M.L., 2020, Landscape drivers of dynamic change in water quality of US rivers: Environmental Science & Technology. v. 54, no. 7, p. 4336−4343, accessed March 2021, at https://doi.org/10.1021/acs.est.9b05344.
U.S. Department of Agriculture Forest Service, 2008, Stream simulation—An ecological approach to providing passage for aquatic organisms at road-stream crossings, accessed May 2021, at https://www.fs.fed.us/eng/pubs/pdf/StreamSimulation/hi_res/%20FullDoc.pdf.
U.S. Geological Survey [USGS], 2021, USGS water data for the Nation: U.S. Geological Survey National Water Information System database, accessed March 20, 2021, at https://doi.org/10.5066/F7P55KJN.
Warnaars, T.A., Hondzo, M., and Power, M.E., 2007, Abiotic controls on periphyton accrual and metabolism in streams—Scaling by dimensionless numbers: Water Resources Research, v. 43, accessed March 2021, at https://doi.org/10.1029/2006WR005002.
Disclaimers
Any use of trade, firm, or product names is for descriptive purposes only and does not imply endorsement by the U.S. Government.
Although this information product, for the most part, is in the public domain, it also may contain copyrighted materials as noted in the text. Permission to reproduce copyrighted items must be secured from the copyright owner.
Suggested Citation
Gidley, R.G., Day, N.K., 2023, Potential factors controlling benthic algae in the upper White River Basin, Colorado, 2018–21: U.S. Geological Survey Fact Sheet 2023–3005, 4 p., https://doi.org/10.3133/fs20233005.
ISSN: 2327-6932 (online)
ISSN: 2327-6916 (print)
Study Area
Publication type | Report |
---|---|
Publication Subtype | USGS Numbered Series |
Title | Potential factors controlling benthic algae in the upper White River Basin, Colorado, 2018–21 |
Series title | Fact Sheet |
Series number | 2023-3005 |
DOI | 10.3133/fs20233005 |
Year Published | 2023 |
Language | English |
Publisher | U.S. Geological Survey |
Publisher location | Reston, VA |
Contributing office(s) | Colorado Water Science Center, Southwest Biological Science Center |
Description | Report: 4 p.; Data Release: 3 |
Country | United States |
State | Colorado |
Other Geospatial | Upper White River Basin |
Online Only (Y/N) | N |