Realizing the Potential of eDNA Biodiversity Monitoring Tools in the Marine Environment with Application to Offshore Renewable Energy
Links
- Document: Report (8.24 MB pdf) , HTML , XML
- Version History: Version History (650 B txt)
- Download citation as: RIS | Dublin Core
Offshore Renewable Energy and Biodiversity
The United States is planning to install 30 gigawatts of offshore wind energy by 2030. Offshore renewable energy development (ORED) is a critical component of global strategies to decarbonize the electricity sector and address the effects of climate change (U.S. Department of Energy, 2023a). However, placement and operation of ORED infrastructure is expected to alter the marine habitat for various fish and wildlife species. For example, new infrastructure and associated human activity offshore may overlap with animal migrations or foraging (Cook and others, 2018). The composition of biological communities can change, with species’ distribution shifts likely (Li and others, 2023). To avoid negative effects on fish and wildlife and their habitats, Federal agencies—including the National Oceanic and Atmospheric Administration, the U.S. Department of the Interior, the U.S. Department of Energy, as well as State agencies and others—need to understand how these developments may alter the marine environment and biodiversity. This information helps natural resource managers by supporting regulatory requirements and informing strategic resource management and conservation efforts. Efforts are underway to assess effects on species of conservation concern, which include species that have either demonstrated considerable decline in their populations, are naturally rare, or have a limited distribution.
eDNA in the Marine Environment
Federal and State agencies study and monitor natural resources offshore as part of their resource management practices. However, collecting biological monitoring data in ocean environments is challenging and costly owing to the natural diversity and complexity of marine communities (United Nations Educational, Scientific and Cultural Organization, 2017). Traces of genetic material (deoxyribonucleic acid [DNA] and ribonucleic acid [RNA]) from organisms, like fish, reptiles, and mammals, can be collected from the environment and analyzed to determine which species were likely present in sampled locations. Emerging technologies that integrate the collection of this environmental DNA (eDNA) and RNA (eRNA) (hereafter referred to as “eDNA”) with autonomous robotic samplers are being developed to enable critical insight about marine biodiversity from the relatively simple, fast, and inexpensive act of collecting a water sample, while reducing the need for expensive research vessels and personnel time (fig. 1). When eDNA sampling is automated with robots, comprehensive monitoring of genetic material from microbes to mammals can occur at a frequency and number of locations not otherwise feasible, providing the high resolution and more complete assessment of marine life needed for accurate monitoring. This allows scientists to track species in time and space to produce baseline genetic material information and assess potential changes in biodiversity.
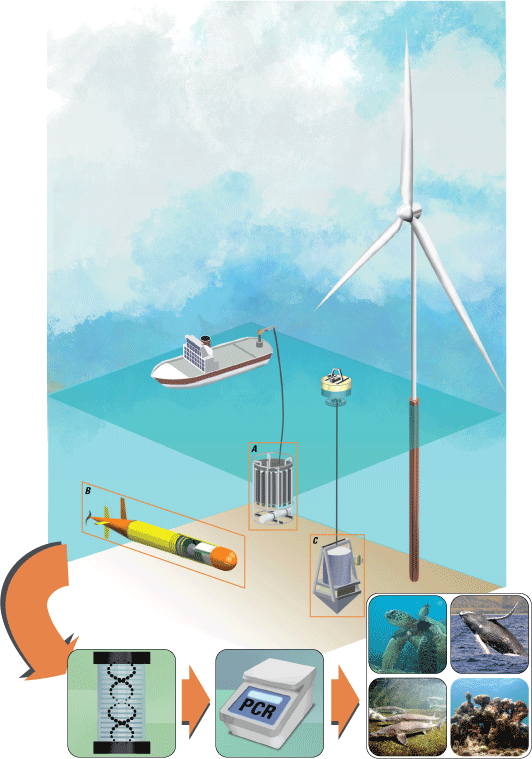
Schematic representation of sampling marine biodiversity via eDNA using current, (A) conductivity/temperature/density [CTD] deployment from ship, and experimental technologies, (B) autonomous underwater vehicles and (C) moored robotic samplers. Once water is filtered, eDNA analysis is performed, and data are analyzed to infer the presence of species.
Research and Development Needs
Like all emerging technologies, robotic eDNA sampling and data interpretation have unresolved knowledge gaps and limitations that must be overcome to realize the potential of eDNA as an effective biodiversity monitoring tool. Continued engineering advancements are needed in autonomous vehicles used for sampling to include eDNA collection at various water depths and for onboard processing. Key challenges with eDNA data interpretation include improving the accuracy of species identification from eDNA, linking eDNA observations to species presence at various sites that are sampled since eDNA can be transported away from its source organisms, determining where and when to deploy robotic eDNA samplers to optimize detections of multiple species of interest, and developing modeling approaches to detect changes in eDNA accurately and precisely over time and space.
eDNA Research and Development Roadmap
The USGS is uniquely positioned to leverage research and development (R&D) advances already achieved in their research programs to respond to these outstanding biodiversity monitoring needs for ORED. The USGS is a leader in the field of eDNA and has, among many additional topics, helped advance robotic eDNA samplers, has extensive experience working in the offshore environment, and has developed novel and actionable statistical methods and standards for monitoring applications (see sidebar 1, sidebar 2, and sidebar 3). The USGS is working with partners to respond to the R&D needs identified in this fact sheet and ensure alignment and coordination with the objectives of the national strategy on aquatic eDNA and associated implementation plans (Kelly and others, 2024). Advancing these key eDNA R&D needs is intended to result in better, faster, and less expensive use of robotic eDNA sampling for biodiversity monitoring applications and inform the development of new tools, like autonomous vehicles, onboard processing, and air eDNA sampling in conjunction with water sampling.
U.S. Geological Survey Expertise in eDNA Applicable to the Marine Environment
Sidebar 1. Marine eDNA Sampling and Metabarcoding Analyses
The U.S. Geological Survey (USGS) brings a wealth of expertise as a world leader in characterizing biological resources and related processes at, and beneath, the seafloor (for example, Brannock and others, 2023; U.S. Geological Survey, 2023; Cordes and others, 2024). As a part of ongoing studies by the National Oceanic and Atmospheric Administration (2023) and the U.S. Geological Survey (2023), the USGS has collected eDNA samples from the surface to the sea floor (60- to 4,500 meter-depth) using remotely operated vehicles and (ship-based and autonomous) conductivity temperature depth instruments to investigate the biological communities in living marine habitats. eDNA analyses provide critical information about species’ DNA presence at the sea floor and in the water column that link the deepwater food web to more productive surface waters. Coupled with environmental data, the eDNA data provide baseline characterization of biodiversity, help define water masses and food webs, and offer a detailed glimpse into ecosystem functioning.
Sidebar 2. Robotic eDNA Samplers
Programmable eDNA robots that can obtain samples at high frequencies across time and locations have the potential to serve as efficient, sensitive, and cost-effective tools for baseline biodiversity assessments as well as long-term monitoring following offshore renewable energy development (ORED). U.S. Geological Survey (USGS) researchers have completed proof-of-concept experiments where robotic eDNA samplers were installed at river streamgages to monitor for invasive mussels, introduced fishes, and human and fish pathogens (Sepulveda and others, 2020). They have documented that robotic eDNA samplers, which collected multiple samples a day for weeks to months, decreased false-negative detection rates and provided a more complete picture of biodiversity. USGS researchers have also deployed robotic eDNA samplers in autonomous underwater vehicles in the Great Lakes to monitor fish communities. USGS researchers are collaborating with the Monterey Bay Aquarium Research Institute to design, validate, and implement robotic eDNA samplers that are scalable, robust, and relatively easy to operate, and provide high-quality data for decision making. These new samplers are tailored for routine use and monitoring applications.
Sidebar 3. Study Design and Statistical Analysis for Actionable Science
The U.S. Geological Survey (USGS) has led the development of statistical (occupancy) models to address the challenges of imperfect species detection, which can result in some sites appearing to be unoccupied (false-negative) or occupied (false-positive) by a species when the opposite is true. Occupancy models solve these problems and produce unbiased estimates of occupancy and related parameters. They also provide insight on the location and timing of surveys required to achieve monitoring objectives, like achieving a specified precision for an occupancy estimate of marine mammals at offshore renewable energy development (ORED) sites. Occupancy models are applicable and informative to a broad range of ecological questions, including those in the marine environment, where imperfect species detection under the water’s surface is an inherent challenge. USGS scientists have led recent efforts to expand the use of occupancy models to eDNA data to minimize inference bias attributed to false-negative or false-positive detections, such as studies conducted on vulnerable manatee populations (Hunter and others, 2018). Software packages, such as EDNAOCCUPANCY (Dorazio and Erickson, 2018) and msocc (Stratton and others, 2020), developed by USGS scientists to fit eDNA data, are included in these efforts. Current USGS efforts on eDNA occupancy models include the incorporation of quantitative eDNA concentration data to better model occupancy of rare species using their equally rare eDNA and the development of both multispecies eDNA occupancy approaches and multimethod approaches to integrate eDNA data with other survey methods like acoustics, imagery, and trawl data.
Key eDNA Research and Development Advances Needed for Offshore Renewable Energy Development Applications
-
1. Validate robotic eDNA sampler use in the marine environment to monitor biodiversity changes in a cost-effective and reproducible way.
-
2. Assess the strengths and limitations of ecological inferences derived from eDNA sampling compared to other sampling approaches.
-
a. Make progress toward a complete, curated species DNA sequence reference database to identify species more accurately when using eDNA approaches.
-
b. Identify critical biotic and abiotic parameters that bias ecological inferences, like eDNA breakdown and movement.
-
c. Develop and validate eDNA modeling approaches to improve species occurrence and distribution inferences and allow comparison of results to other survey tools.
-
-
3. Develop analytical tools that quantify offshore fish and wildlife distributions for planning and monitoring ORED.
-
a. Develop and validate eDNA modeling advances and visualization tools to produce interactive distribution models for species of conservation concern.
-
b. Develop and validate multimethod approaches that harness the strengths of different survey tools, such as bioacoustics and microscopy, to enhance eDNA inferences. These models should provide superior information and enable comparisons among different field methods, ultimately enhancing the effectiveness of survey design.
-
Knowledge of the distribution of biological resources relative to ORED is critical to monitoring ocean health. eDNA offers a transformative tool to discover, map, monitor, and manage marine biodiversity and is a nimble and affordable environmental monitoring tool that could help streamline ORED assessments. Additional R&D investments are needed to facilitate the development of renewable energy resources. Similar to the advancements that satellite remote sensing provided for understanding and predicting weather and climate (Yang and others, 2013), eDNA can be a transformative technology for living resource monitoring.
References Cited
Brannock, P.M., Demopoulos, A.W.J., Landers, S.C., Waits, D.S., and Halanych, K.M., 2023, Metabarcoding analysis of meiobenthic biodiversity along the Gulf of Mexico continental shelf: Estuarine, Coastal and Shelf Science, v. 285, article 108303, accessed April 11, 2024, at https://doi.org/10.1016/j.ecss.2023.108303.
Cook, A.S.C.P., Humphreys, E.M., Bennet, F., Masden, E.A., Burton, N.H.K., 2018, Quantifying avian avoidance of offshore wind turbines—Current evidence and key knowledge gaps: Marine Environmental Research, v. 140, p. 278–288, accessed May 7, 2024, at https://doi.org/10.1016/j.marenvres.2018.06.017.
Cordes, E., Demopoulos, A.W.D., Bernard, B.B., Brooke, S., Brooks, J.M., Joye, S., Miksis-Olds, J., Quattrini, A., Sutton, T., and Wolff, G.A., 2024, Deepwater Atlantic habitats II—Continued Atlantic research and exploration in deepwater ecosystems with focus on coral, canyon, and seep communities, OCS Study BOEM 2024–012: Sterling, Va., U.S. Department of the Interior, Bureau of Ocean Energy Management, 638 p., accessed April 7, 2024, at https://espis.boem.gov/Final%20Reports/BOEM_2024-012.pdf.
Dorazio, R.M., and Erickson, R.A., 2018, Ednaoccupancy—An R package for multiscale occupancy modelling of environmental DNA data: Molecular Ecology Resources, v. 18, no. 2, p. 368–380, accessed March 2, 2024, at https://doi.org/10.1111/1755-0998.12735.
Hunter, M.E., Meigs-Friend, G., Ferrante, J.A., Kamla, A.T., Dorazio, R.M., Diagne, L.K.Luna, F., Lanyon, J.M., and Reid, J.P., 2018, Surveys of environmental DNA (eDNA)—A new approach to estimate occurrence in Vulnerable manatee populations: Endangered Species Research, v. 35, p. 101–111, accessed March 18, 2024, at https://doi.org/10.3354/esr00880.
Kelly, R.P., Lodge, D.M., Lee, K.N., Theroux, S., Sepulveda, A.J., Scholin, C.A., Craine, J.M., Andruszkiewicz Allan, E., Nichols, K.M., Parsons, K.M., Goodwin, K.D., Gold, Z., Chavez, F.P., Noble, R.T., Abbott, C.L., Baerwald, M.R., Naaum, A.M., Thielen, P.M., Simons, A.L., Jerde, C.L., Duda, J.J., Hunter, M.E., Hagan, J.A., Sarah Meyer, R., Steele, J.A., Stoeckle, M.Y., Bik, H.M., Meyer, C.P., Stein, E., James, K.E., Thomas, A.C., Demir-Hilton, E., Timmers, M.A., Griffith, J.F., Weise, M.J., and Weisberg, S.B., 2024, Toward a national eDNA strategy for the United States: Environmental DNA, v. 6, no. 1, article e432, accessed March 24, 2024, at https://doi.org/10.1002/edn3.432.
Li, C., Coolen, J.W.P., Scherer, L., Mogollón, J.M., Braeckman, U., Vanaverbeke, J., Tukker, A., and Steubing, B., 2023, Offshore wind energy and marine biodiversity in the North Sea—Life cycle impact assessment for benthic communities: Environmental Science & Technology, v. 57, no. 16, p 6455–6464, accessed May 7, 2024, at https://doi.org/10.1021/acs.est.2c07797.
National Oceanic and Atmospheric Administration, 2023, Gulf of Mexico mesophotic and deep benthic communities restoration: National Oceanic and Atmospheric Administration web page, accessed April 26, 2024, at https://coastalscience.noaa.gov/science-areas/restoration/gulf-mdbc-restoration/.
Sepulveda, A.J., Birch, J.M., Barnhart, E.P., Merkes, C.M., Yamahara, K.M., Marin, R., III, Kinsey, S.M., Wright, P.R., and Schmidt, C., 2020, Robotic environmental DNA bio-surveillance of freshwater health: Scientific Reports, v. 10, no. 1, article 14389, accessed March 25, 2024, at https://doi.org/10.1038/s41598-020-71304-3.
Stratton, C., Sepulveda, A.J., and Hoegh, A., 2020, msocc—Fit and analyse computationally efficient multi‐scale occupancy models in R: Methods in Ecology and Evolution, v. 11, no. 9, p. 1113–1120, accessed April 7, 2024, at https://doi.org/10.1111/2041-210X.13442.
United Nations Educational, Scientific and Cultural Organization, 2017, Global ocean science report—The current status of ocean science around the world: Paris, France, United Nations Educational, Scientific and Cultural Organization, accessed April 2, 2024, at https://sdgs.un.org/publications/global-ocean-science-report-current-status-ocean-science-around-world-18062.]
U.S. Department of Energy, 2023a, Advancing offshore wind energy in the United States—U.S. Department of Energy strategic contributions toward 30 gigawatts and beyond: U.S. Department of Energy, 67 p., accessed May 4, 2024, at https://www.energy.gov/sites/default/files/2023-03/advancing-offshore-wind-energy-full-report.pdf.
U.S. Department of Energy, 2023b, Offshore wind market report—2023 edition: U.S. Department of Energy, Office of Energy Efficiency & Renewable Energy, 121 p., accessed March 25, 2024, at https://www.energy.gov/sites/default/files/2023-09/doe-offshore-wind-market-report-2023-edition.pdf.
U.S. Geological Survey, 2023, Exploring biodiversity of the deep Hawaiian Pacific Ocean with seafloor mapping and eDNA technologies: U.S. Geological Survey web page, accessed April 26, 2024, at https://www.usgs.gov/special-topics/deep-sea-exploration%2C-mapping-and-characterization/science/exploring-biodiversity#overview.
Yang, J., Gong, P., Fu, R., Zhang, M., Chen, J., Liang, S., Xu, B., Shi, J., and Dickinson, R., 2013, The role of satellite remote sensing in climate change studies (corrected December 20, 2013): Nature Climate Change, v. 3, p. 875–883, accessed May 7, 2024, at https://doi.org/10.1038/nclimate1908.
For more information, contact:
Energy and Wildlife Program Specialist, Ecosystems Mission Area
U.S. Geological Survey
12201 Sunrise Valley Drive
Reston, VA 20192
or visit: https://www.usgs.gov/mission-areas/ecosystems.
By Adam Sepulveda, Cheryl Morrison, Maggie Hunter, and Mona Khalil
Photographs by U.S. Geological Survey except where noted.
Sidebar 2 photograph provided by the Monteray Bay Aquarium.
Figure 1 illustration by David Bruce, U.S. Geological Survey;
Figure 1 photographs by the U.S. Geological Survey except Atlantic sturgeon by Ryan Hagerty, U.S. Fish and Wildlife Service; and coral garden photograph by the National Oceanic and Atmospheric Administration.
Edited by Skylar N. Petrik, Baltimore Publishing Service Center.
Layout by David Bruce, Reston Publishing Service Center.
Disclaimers
Any use of trade, firm, or product names is for descriptive purposes only and does not imply endorsement by the U.S. Government.
Although this information product, for the most part, is in the public domain, it also may contain copyrighted materials as noted in the text. Permission to reproduce copyrighted items must be secured from the copyright owner.
Suggested Citation
Sepulveda, A., Morrison, C., Hunter, M., and Khalil, M., 2024, Realizing the potential of eDNA biodiversity monitoring tools in the marine environment with application to offshore renewable energy (ver. 1.1, June 2024): U.S. Geological Survey Fact Sheet 2024–3019, 6 p., https://doi.org/10.3133/fs20243019.
ISSN: 2327-6932 (online)
ISSN: 2327-6916 (print)
Publication type | Report |
---|---|
Publication Subtype | USGS Numbered Series |
Title | Realizing the potential of eDNA biodiversity monitoring tools in the marine environment with application to offshore renewable energy |
Series title | Fact Sheet |
Series number | 2024-3019 |
DOI | 10.3133/fs20243019 |
Edition | Version 1.0: May 31, 2024; Version 1.1: June 11, 2024 |
Year Published | 2024 |
Language | English |
Publisher | U.S. Geological Survey |
Publisher location | Reston, VA |
Contributing office(s) | Office of the AD Ecosystems |
Description | 6 p. |
Online Only (Y/N) | N |
Additional Online Files (Y/N) | N |
Google Analytic Metrics | Metrics page |