Alaska Focus Area Definition for Data Acquisition for Potential Domestic Sources of Critical Minerals in Alaska for Antimony, Barite, Beryllium, Chromium, Fluorspar, Hafnium, Magnesium, Manganese, Uranium, Vanadium, and Zirconium
Links
- Document: Report (14.7 MB pdf) , HTML , XML
- Chapters:
- Open-File Report 2019-1023-A - Focus Areas for Data Acquisition for Potential Domestic Sources of Critical Minerals—Rare Earth Elements
- Open-File Report 2019-1023-B - Focus Areas for Data Acquisition for Potential Domestic Resources of 11 Critical Minerals in the Conterminous United States, Hawaii, and Puerto Rico—Aluminum, Cobalt, Graphite, Lithium, Niobium, Platinum-Group Elements, Rare Earth Elements, Tantalum, Tin, Titanium, and Tungsten
- Open-File Report 2019-1023-C - Focus Areas for Data Acquisition for Potential Domestic Resources of 11 Critical Minerals in Alaska—Aluminum, Cobalt, Graphite, Lithium, Niobium, Platinum Group Elements, Rare Earth Elements, Tantalum, Tin, Titanium, and Tungsten
- Open-File Report 2019-1023-D - Focus Areas for Data Acquisition for Potential Domestic Resources of 13 Critical Minerals in the Conterminous United States and Puerto Rico—Antimony, Barite, Beryllium, Chromium, Fluorspar, Hafnium, Helium, Magnesium, Manganese, Potash, Uranium, Vanadium, and Zirconium
- Data Release: USGS data release - GIS, supplemental data table, and references for focus areas of potential domestic resources of 13 critical minerals in the United States and Puerto Rico—Antimony, barite, beryllium, chromium, fluorspar, hafnium, helium, magnesium, manganese, potash, uranium, vanadium, and zirconium
- Download citation as: RIS | Dublin Core
Acknowledgments
Funding for this work was provided by the Mineral Resources Program within the USGS and the Earth Mapping Resources Initiative. Melanie B. Werdon, Alaska Division of Geology & Geophysics, participated in workshops and provided data to complete the documentation of focus areas in Alaska. We thank USGS colleague Karen Kelley for her constructive review of this report.
Chemical Symbols
Ag
silver
Al
aluminum
As
arsenic
Au
gold
Bi
bismuth
Co
cobalt
Cr
chromium
Cs
cesium
Cu
copper
Ga
gallium
Ge
germanium
Hf
hafnium
In
indium
Li
lithium
Mg
magnesium
Mn
manganese
Mo
molybdenum
Nb
niobium
Ni
nickel
Pb
lead
Rb
rubidium
Re
rhenium
Sb
antimony
Sc
scandium
Sn
tin
Sr
strontium
Ta
tantalum
Te
tellurium
Ti
titanium
U
uranium
V
vanadium
W
tungsten
Zn
zinc
Zr
zirconium
Preface
Pursuant to Presidential Executive Order (EO) 13817 of December 20, 2017, “A Federal Strategy to Ensure Secure and Reliable Supplies of Critical Minerals” (82 FR 60835–60837), the Secretary of the Interior directed the U.S. Geological Survey (USGS), in coordination with other Federal agencies, to draft a list of critical minerals. The USGS developed a draft list of 35 critical minerals using a quantitative screening tool (S.M. Fortier and others, 2018, USGS Open-File Report 2018–1021, https://doi.org/10.3133/ofr20181021). The draft list of 35 minerals or mineral material groups deemed critical was finalized in May 2018 (83 FR 23295–23296), although the designation of “critical” will be reviewed at least every 3 years in accordance with the Energy Act of 2020 (Public Law 116–260, 134 Stat. 2565). A “critical mineral” is defined by EO 13817, section 2, as follows:
Definition. (a) A “critical mineral” is a mineral identified by the Secretary of the Interior pursuant to subsection (b) of this section to be (i) a non-fuel mineral or mineral material essential to the economic and national security of the United States, (ii) the supply chain of which is vulnerable to disruption, and (iii) that serves an essential function in the manufacturing of a product, the absence of which would have significant consequences for our economy or our national security.
Disruptions in supply chains may arise for any number of reasons, including natural disasters, labor strife, trade disputes, resource nationalism, and conflict.
EO 13817 noted that “despite the presence of significant deposits of some of these minerals across the United States, our miners and producers are currently limited by a lack of comprehensive, machine-readable data concerning topographical, geological, and geophysical surveys.”
In response to the need for information on potential domestic sources of these critical minerals, the USGS launched the Earth Mapping Resources Initiative (Earth MRI). The Earth MRI is a partnership between the U.S. Geological Survey, other Federal agencies, State geological surveys, and the private sector, and it is designed to acquire the national geologic framework information essential for identifying areas with potential for hosting the Nation’s critical mineral resources. The goal of the Earth MRI is to improve the geological, geophysical, and topographic mapping of the United States and to procure new data to stimulate mineral exploration to secure the Nation’s supply of critical minerals.
Abstract
Phase 3 of the Earth Mapping Resources Initiative (Earth MRI) focuses on geologic belts that are favorable for hosting mineral systems that could contain the critical minerals antimony, barite, beryllium, chromium, fluorspar, hafnium, magnesium, manganese, uranium, vanadium, and zirconium. Prior phases of the Earth MRI program in Alaska focused only on rare earth elements, aluminum, cobalt, graphite, lithium, niobium, platinum-group metals, tantalum, tin, titanium, and tungsten. An additional 11 critical minerals planed for future phases of Earth MRI (As, Bi, Cs, Ga, Ge, In, Re, Rb, Sc, Sr, Te) are considered prospective in these focus areas. Together, Alaska focus areas address 22 of the 35 minerals or mineral material groups presently deemed critical. This report describes the methodology and techniques utilized to define focus areas for future data acquisition in Alaska; the conterminous United States are covered in a separate report.
Focus areas are identified using a mineral systems framework, which accounts for all the possible tectonic and geologic settings where co-genetic mineral deposits may form. These deposits contain many commodities, including byproduct and critical minerals. Large system-scale processes may be evaluated using such a framework to determine the influence they play on critical mineral endowment within the deposits. Analyzing larger mineral systems provides an integrated and broad context to determine how and where critical minerals are sourced, transported, and deposited in geologic systems.
Statewide geological, geochemical, geophysical, and mineral occurrence datasets informed the delineation of focus areas in Alaska. For some mineral systems, previously published data-driven prospectivity analyses for critical mineral-bearing deposit types provided the basis for focus areas. We report a total of 22 new focus areas that are prospective for phase 3 critical minerals. These new focus areas represent four different mineral systems that are known or suspected to occur in Alaska. An additional 55 focus areas that were previously identified for phase 1 and phase 2 commodities were also identified as being prospective for phase 3 critical minerals. Collectively, 102 focus areas in Alaska have known or suspected potential for hosting phase 1, phase 2, and (or) phase 3 critical minerals. These focus areas represent 17 different mineral systems also containing critical minerals that are planned for consideration in future Earth MRI phases. Thus, the focus areas delineated herein, and in previous reports for Alaska, are comprehensive for all critical minerals as presently defined and may be used to guide the collection of new geologic, geochemical, and geophysical data in the region.
Introduction
The Earth Mapping Resources Initiative (Earth MRI) was launched by the U.S. Geological Survey (USGS) to bolster the national geologic and geophysical datasets required to document the potential for domestic sources of critical minerals (Day, 2019). This report describes the background data, sources, and methodology used to define broad focus areas for future data collection (geologic mapping, aeromagnetic and radiometric geophysical acquisition, and geochemical characterization) in Alaska (fig. 1). Geologic and geophysical data generated from this effort will improve the understanding of the mineral resources and framework geology throughout the state that are suspected or known to contain nonfuel mineral systems. This report focuses on mineral systems that have potential for phase 3 (Sb, barite [BaSO4], Be, Cr, fluorspar [CaF2], Hf, Mg, Mn, U, V, Zr) critical mineral enrichments (tables 1 and 2). Many of these mineral systems also have potential for phase 2 (Al, Co, graphite [C], Li, Nb, platinum group elements [PGEs], rare earth elements [REEs], Ta, Sn, Ti, and W) and (or) phase 1 (REE) critical mineral enrichments (table 2; Kreiner and Jones, 2020). Furthermore, select mineral systems also have potential for future phase (As, Bi, Cs, Ga, Ge, In, Re, Rb, Sc, Sr, Te) critical mineral enrichments (table 2). Thus, the focus areas delineated herein and in Kreiner and Jones (2020) represent all mineral systems that have potential to contain critical minerals and are known or suspected to exist in Alaska.
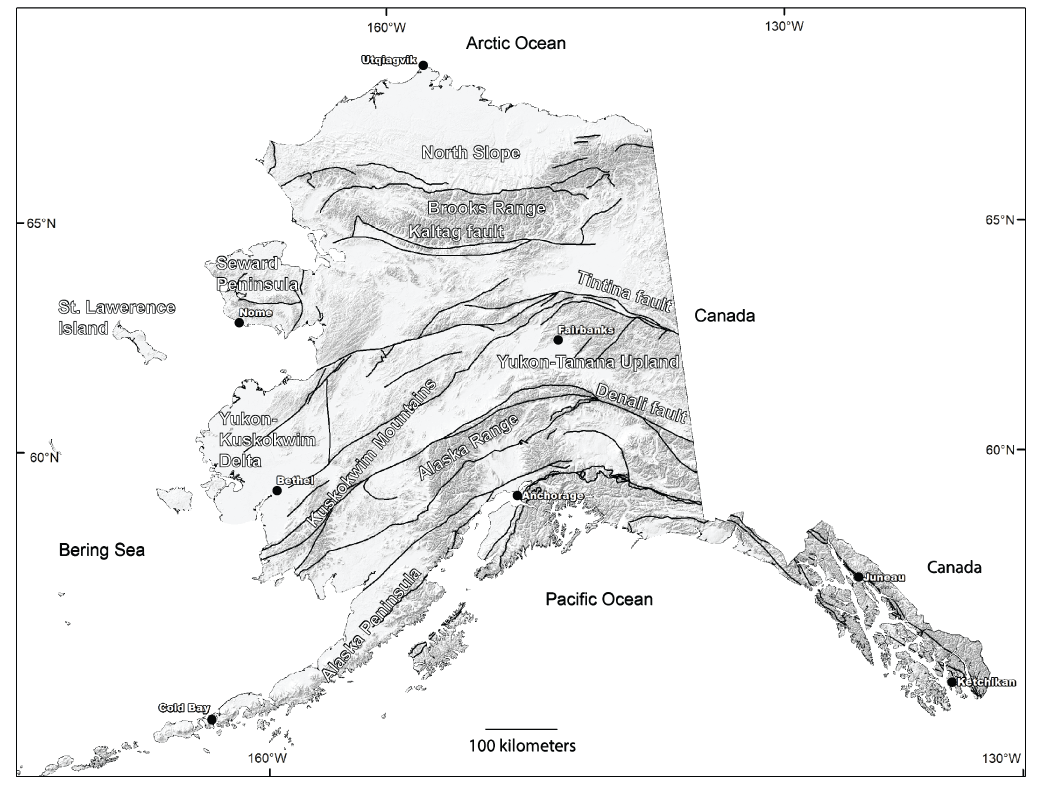
Geographical distribution of features, population centers, and major faults. Background is a shaded digital elevation model showing areas of high topography in darker shades. Major faults are solid black lines (from Wilson and others, 2015). Base map is from the National Elevation Dataset (NED), USGS.
Table 1.
Production data and applications of phase 3 critical minerals.[Production and consumption data from U.S. Geological Survey (2021); notable application examples from Fortier and others (2019). WH, data withheld to avoid disclosing company proprietary data; t, metric ton; lbs., pounds; <, less than; MgO, magnesium consumption; uranium data from table 8.2 of Energy Information Administration (2021) and World Nuclear Association (2021)]
Table 2.
Major and critical commodities in mineral systems of Alaska.[Abbreviations defined in list of Chemical Symbols. Phase 2 critical minerals are shown in italics. Phase 3 critical mineral commodities are shown in bold. Other critical commodities from Fortier and others (2018) are shown in plain text. PGEs, platinum group elements; REEs, rare earth elements; --, not applicable; IOA-IOCG, iron oxide-apatite-iron oxide copper gold]
Mineral system | Major commodity | Critical mineral commodities | Alaska prospectivity model | Earth MRI Focus Area Descriptions |
---|---|---|---|---|
Basin brine path | Lead, zinc, copper, silver | Cobalt, lithium, PGE, REE, tin, barite, germanium, gallium, indium, rhenium, vanadium, uranium | Carbonate-hosted Cu (-Co-Ag-Ge-Ga) deposits; sediment-hosted Pb-Zn deposits |
Phase 2 (Kreiner and Jones, 2020); Phase 3 (this study) |
Volcanogenic seafloor | Copper, gold, lead, zinc, silver | Cobalt, tin, antimony, barite germanium, gallium, indium, bismuth, tellurium, arsenic | Volcanogenic massive sulfide deposits* | Phase 3 (this study) |
Carlin-type | Gold | Antimony, arsenic | -- | Phase 3 (this study) |
Marine evaporite | Gypsum | Barite | -- | Phase 3 (this study) |
Placer | Gold, PGE | Niobium, PGE, REE, tantalum, tin, titanium, tungsten, zirconium, hafnium | Placer and paleo-placer gold deposits | Phase 2 (Kreiner and Jones, 2020) |
Climax-type | Molybdenum | Aluminum, niobium, tantalum, tin, tungsten, helium** | Sn-W-Mo (-Ta-In-fluorspar) deposits associated with specialized granites | Phase 2 (Kreiner and Jones, 2020) |
IOA-IOCG | Copper, gold | Cobalt, REE, antimony, uranium, arsenic | -- | Phase 2 (Kreiner and Jones, 2020) |
Mafic magmatic | PGE, chromium, nickel | Cobalt, PGE, titanium, chromium | PGE (-Co-Cr-Cu-Ni-Ti-V) deposits associated with mafic to ultramafic intrusive rocks | Phase 2 (Kreiner and Jones, 2020) |
Marine chemocline | Phosphorous, REE | REE, fluorspar, uranium | -- | Phase 2 (Kreiner and Jones, 2020) |
Magmatic REE | REE | Niobium, tantalum, REE, fluorspar, zirconium, uranium | REE-Th-Y-Nb (-U-Zr) deposits associated with peralkaline to carbonatitic intrusive rocks | Phase 2 (Kreiner and Jones, 2020) |
Orogenic and (or) reduced intrusion related | Gold | Tungsten, antimony, arsenic, tellurium, bismuth, rhenium, | Reduced intrusion related and orogenic gold | Phase 2 (Kreiner and Jones, 2020) |
Porphyry Cu-Mo-Au or alkalic porphyry | Copper, molybdenum, gold, lead, zinc, silver | Cobalt, bismuth, rhenium, PGE, tungsten, magnesium, manganese, tellurium, scandium, tungsten, uranium, vanadium, antimony | -- | Phase 2; Kreiner and Jones (2020) |
Porphyry Sn | Tin, tungsten | Lithium, niobium, tantalum, tin, tungsten, fluorspar, beryllium | Sn-W-Mo (-Ta-In-fluorspar) deposits associated with specialized granites | Phase 2 (Kreiner and Jones, 2020) |
Meteoric recharge | Uranium, vanadium | Uranium, vanadium, rhenium, scandium, REE, manganese, cobalt, PGE | Sandstone-hosted U (-V-Cu) deposits | Phase 2 (Kreiner and Jones, 2020) |
Metamorphic | Graphite | Graphite | -- | Phase 2 (Kreiner and Jones, 2020) |
The Alaska focus areas developed in this report were defined using a mineral systems framework (Hofstra and Kreiner, 2020). Datasets that contribute to the delineation of the focus area include the published and ongoing statewide geospatial prospectivity mapping (Karl and others, 2016, 2021; Kelley and others, 2021) and other relevant statewide datasets and publications (for example, Wilson and others, 2015; Granitto and others, 2019; Kreiner and others, 2020). Focus areas in Alaska are necessarily broad, due to substantial gaps in modern data coverage and quality across such a geologically complicated, large, and remote region. Where possible, mineral deposits that contain critical mineral enrichments identified in the Alaska Resource Data File (U.S. Geological Survey, 2008; ARDF; https://mrdata.usgs.gov/ardf/) are included within focus areas. Broad focus areas are drawn across regions of the state where no known mineral enrichments occur and include areas that exhibit key geological characteristics that are identified as important features of critical-mineral-bearing systems. Limited bedrock exposure across many regions of the state (for example, the North Slope and the Yukon-Kuskokwim delta, fig. 1) hampers the ability to collect appropriate data. Additionally, major fault systems (for example, Kaltag, Tintina, and Denali faults, fig. 1) juxtapose disparate geologic belts, which have been mapped at varying levels of detail, making interpretations across major faults difficult. The goal of Earth MRI is to acquire new geophysical, geologic, and geochemical data through mapping in these focus areas across Alaska to enable the USGS to evaluate the formation of and prospectivity for critical mineral deposits.
Criteria used to define the focus areas for the three regions of the conterminous United States are described in a companion report (Hammarstrom and others, 2022), which used a slightly different methodology and relied on additional datasets. These datasets include the USMIN Mineral Deposit Database, which is more complete and accurate for these regions than for Alaska (for example, Hammarstrom and others, 2022). Additionally, much of the conterminous United States has been studied in greater detail and experienced a rich history of geologic work leading to far greater information on the presence of known mineral occurrences, deposits, or mines that have current or past production. Thus, more detailed focus areas are drawn than can be done in Alaska, where a lack of knowledge and detailed data precludes such detailed analysis. In some cases, though, broader focus areas were developed to encompass regions containing mineral systems that have potential for phase 3 critical mineral enrichments in the absence of known deposits.
A related USGS data release (Dicken and others, 2021) provides geospatial data for all phase 3 focus areas in the U.S. and an associated data table that (1) summarizes what is known about the critical mineral potential of each focus area, (2) provides information on the extent and quality of the available geophysical and topographic coverages in each focus area, and (3) contains brief descriptions of data gaps that could be addressed through Earth MRI.
Phase 3 Critical Minerals
For earlier phases, Earth MRI selected and prioritized critical mineral commodities from the comprehensive list in Fortier and others (2018); these chosen commodities could reduce the import reliance on foreign sources, if a domestic discovery is made (Hammarstrom and Dicken, 2019; Hammarstrom and others, 2020; Kreiner and Jones, 2020). Critical minerals identified in phase 3 have high U.S. net import reliance and an increasing demand beyond the foreseeable domestic production. In addition, phase 3 critical minerals that are the focus of this report occur less often as principal commodities and, instead, are most commonly recovered as byproducts.
Following the selection of the phase 3 critical minerals (table 1), mineral systems were identified (table 2) that contain these commodities (Hofstra and Kreiner, 2020). Alaska contains multiple mineral systems that have the potential to host each of the phase 3 commodities, except for potash and helium. Potash is mined nearly exclusively from marine evaporite deposits (Hofstra and Kreiner, 2020) but has never been described in the few Alaska geologic belts that contain marine evaporites. Helium production from hard rock mineral deposits is principally from volcanogenic settings associated with highly evolved magmas in the Climax-type mineral system of Hofstra and Kreiner (2020). Despite some evidence of Climax-type molybdenum mineral systems in the state, no known helium resources have been described in those systems. Helium is presently recovered almost exclusively as a byproduct of natural gas production. Notwithstanding the petroleum wealth of Alaska, helium data in Alaska petroleum systems are sparse. Reported values are relatively low compared to other helium-bearing reservoirs in the United States (Pacheco and Ali, 2008). Accordingly, helium and potash were not considered during the development of the phase 3 focus areas in Alaska because they are not predicted to occur in Alaska as bedrock mineral deposits.
Alaska’s geologic belts meet the criteria for 14 distinct mineral systems that each have the potential to host one or more phase 3 critical mineral commodities (table 2). Mineral systems, for which new focus areas were developed, include basin brine path (barite), volcanogenic seafloor (barite, Sb), Carlin-type (Sb), and marine evaporite systems (barite) (table 2). Many of the mineral systems and focus areas that were delineated as part of phases 1 and 2 (Hammarstrom and Dicken, 2019; Kreiner and Jones, 2020) also contain phase 3 critical minerals. These mineral systems (and the phase 3 critical minerals they could contain) include placer (zirconium, hafnium), IOA-IOCG (Sb, U), mafic magmatic (Cr), marine chemocline (U), magmatic REE (fluorspar, Zr, U), orogenic (Sb), reduced intrusion-related gold (Sb), porphyry Cu-Mo-Au (Mg, Sb), porphyry Sn-W (fluorspar, Be), and meteoric recharge (U, V) (table 2). We do not replicate the previously published phase 1 and 2 focus areas in this report, but the phase 3 data release was updated to include them and an expanded list of critical minerals that they could contain (Dicken and others, 2021). Refer to Kreiner and Jones (2020) for detailed descriptions of phase 2 mineral systems that also are prospective for phase 3 critical commodities; refer to Dicken and others (2019) or Dicken and Hammarstrom (2020) for associated geospatial data.
Mineral Systems Approach
The following two paragraphs are repeated and summarized for the benefit of the reader from Kreiner and Jones (2020, p. 4). Mineral systems provide the framework that considers all geologic features that can influence or control the formation and preservation of a mineral deposit. Ore deposits, where potential economic concentrations of critical commodities could be, are the culmination of the geologic processes that constitute the mineral system. Mineral systems require the following: (1) an energy driver (topography, geothermal gradient in the crust, or magma); (2) a source of components (for example, metals) and fluid (melts, aqueous fluids, petroleum), and ligands (to complex components); (3) transport pathways (faults, fractures, or permeable lithologic units); and (4) a physical and (or) chemical trap (mixing of fluids, reduced host rocks, or boiling) (Hofstra and Kreiner, 2020). A productive mineral system must incorporate each of these criteria to generate a mineral deposit (Hofstra and Kreiner, 2020).
Mineral systems generally are characteristic of a single episode that typically occurs relatively late in an otherwise broad, potentially long-lived geotectonic setting. These systems can be evaluated on larger scales and commonly exhibit larger spatial footprints than a single mineral deposit. Due to this larger spatial and temporal scale, a single mineral system can result in multiple genetically related deposits that are mineralogically distinct. Furthermore, within a single mineral system, subtle variations in the fluid chemistry, source rocks, ligands, and lithologic setting can result in unique differences in the types of metals that can be transported or trapped in otherwise similar geotectonic settings. These subtle differences are responsible for the presence or absence of critical mineral enrichments, as byproducts in a system. Critical minerals are rarely the primary mineral commodity being explored and (or) produced in a mineral system, although exceptions include some deposits where the critical mineral is the principal commodity (REE, PGE, graphite, or uranium; Hofstra and Kreiner, 2020). Thus, understanding where, how, and why critical minerals are enriched in mineral systems is essential for most effectively predicting where undiscovered critical mineral resources are more likely to be (Hofstra and Kreiner, 2020).
Table 2 lists the mineral systems that have potential critical minerals and are known or suspected to be in Alaska. Some phase 2 mineral systems, delineated by Kreiner and Jones (2020), also contain phase 3 critical mineral commodities and are updated accordingly in table 2. Some mineral systems that contain critical commodities that are planned to be the focus of future Earth MRI phases are included in table 2. Table 2 also lists the deposit types for which statewide data-driven prospectivity analyses have been published or are in progress. Some mineral systems listed in table 2 have not yet been included in Alaska mineral prospectivity mapping (Karl and others, 2016), so focus areas for these mineral systems were identified through synthesis of published geological data and (or) ongoing geological research.
Data Sources
The development and delineation of focus areas in Alaska for phase 3 critical minerals relies on publicly available geological, geochemical, geophysical, and mineral occurrence datasets (U.S. Geological Survey, 2008; Wilson and others, 2015; Granitto and others, 2019). The description of the datasets and an overview of data-driven, GIS-based assessment methods can be found in Kreiner and Jones (2020). More detailed discussion of data-driven mineral prospectivity mapping in Alaska can be found in Karl and others (2016, 2021) and Jones and others (2015).
Delineation of Focus Areas
Data-driven, GIS-based analyses that map out mineral resource prospectivity across Alaska have already been published for numerous ore system types known or suspected to contain critical minerals (Jones and others, 2015; Karl and others, 2016, 2021; Kelley and others, 2021). These studies query characteristics relevant to the mineral system of interest in the geospatial geologic and geochemical datasets. A score is generated for each subwatershed (that is, watersheds that have 12-digit hydrologic unit codes and cover approximately 100 km2), and subwatersheds are classified based on scores that indicate high, medium, low, or unknown potential for that mineral system. Areas are drawn around regions containing high- to medium-potential subwatersheds for the mineral system of interest, and these areas are interpreted to be prospective for the associated mineral commodities. For mineral systems covered by published prospectivity models (Karl and others, 2016, 2021; Kelley and others, 2021), the phase 2 and phase 3 focus areas directly correspond to the areas outlining elevated prospectivity in the reports. For mineral systems that have not yet been analyzed utilizing the data-driven approach previously outlined, we qualitatively assessed available data to delineate the focus areas. For example, relevant mineral occurrences from the ARDF were combined with queries of key lithologic units in the Alaska geological map database (Wilson and others, 2015) to help identify possible volcanogenic seafloor, Carlin-type, and marine evaporite mineral systems. The mineral occurrences and prospective lithologies were overlain geospatially, and polygons were drawn around areas that contained permissive geological environments and mineral systems. Relevant data including information about the mineral systems, rationale for delineating each focus area, mineral occurrences and (or) prospects, any known production, potential for future critical mineral discovery, and key references were compiled in a database using the template outlined in table 3. The phase 3 focus area database is published separately as a USGS data release, which also contains geospatial data for each focus area (Dicken and others, 2021). The preliminary phase 3 focus areas were then reviewed by collaborators at the Alaska Division of Geology & Geophysical Surveys.
Table 3.
Focus area template containing criteria used to delineate focus areas for mineral systems in Alaska.[ARDF, Alaska Resource Data File; USMIN, U.S. Mineral Deposit Database; USGS, U.S. Geological Survey]
Topic | Explanation |
---|---|
Name of focus area | Descriptive geographic or geologic name |
Region | Alaska, West, Central, East |
Subregion | |
Mineral system | Select from table 1 |
Deposit type(s) | Select from table 1 |
Commodities | Mineral commodities associated with the focus area |
Identifier | A unique identifier for each focus area; some focus areas could be multipart |
States | States included in the focus area |
Basis for focus area | Short description of the main geologic criteria (basis) for delineating the area |
Production | Yes (when), no, or unknown |
Status of activity | Active mining, current or past exploration, unknown |
Estimated resources | Cite, if known |
Geologic maps that cover the area | Estimate of the percentage of the focus area covered by geologic mapping at different scales; cite specific references if applicable |
Geophysical data that cover the area | Types and quality of available data (aeromagnetic, gravity, radiometric, other) |
Favorable rocks and structures | Lithostratigraphic suitability for deposits; structures that could control mineralization |
Deposits | Name deposits within the focus area that have identified resources or past production |
Mineral occurrences | Summarize occurrences, if any, from USMIN, ARDF or other databases |
Geochemical evidence | Stream sediment, rock, soil indications, or associated commodities |
Geophysical evidence | Data that could indicate buried intrusions, extensions of known mineralization, structural controls |
Evidence from other sources | If applicable |
Comments | Author’s general comments on the focus area |
Cover thickness and description | Comment, if applicable. Otherwise, not applicable (NA) |
Selected references | Short reference (author[s], year) |
Authors | USGS and State Geological Surveys |
Geologic mapping and modeling needs | List geologic mapping needs |
Geophysical survey and modeling needs | List types of geophysical data needed and explain why |
Lidar | Give examples of utility of lidar for the focus area |
Mineral Systems
The following sections provide the background data describing the mineral systems that contain phase 3 critical minerals and were not previously described in Kreiner and Jones (2020). For each mineral system, we discuss the rationale for its inclusion and the general locations and geological characteristics of the associated focus areas in Alaska. The focus areas for each mineral system described herein are shown in figure 2.
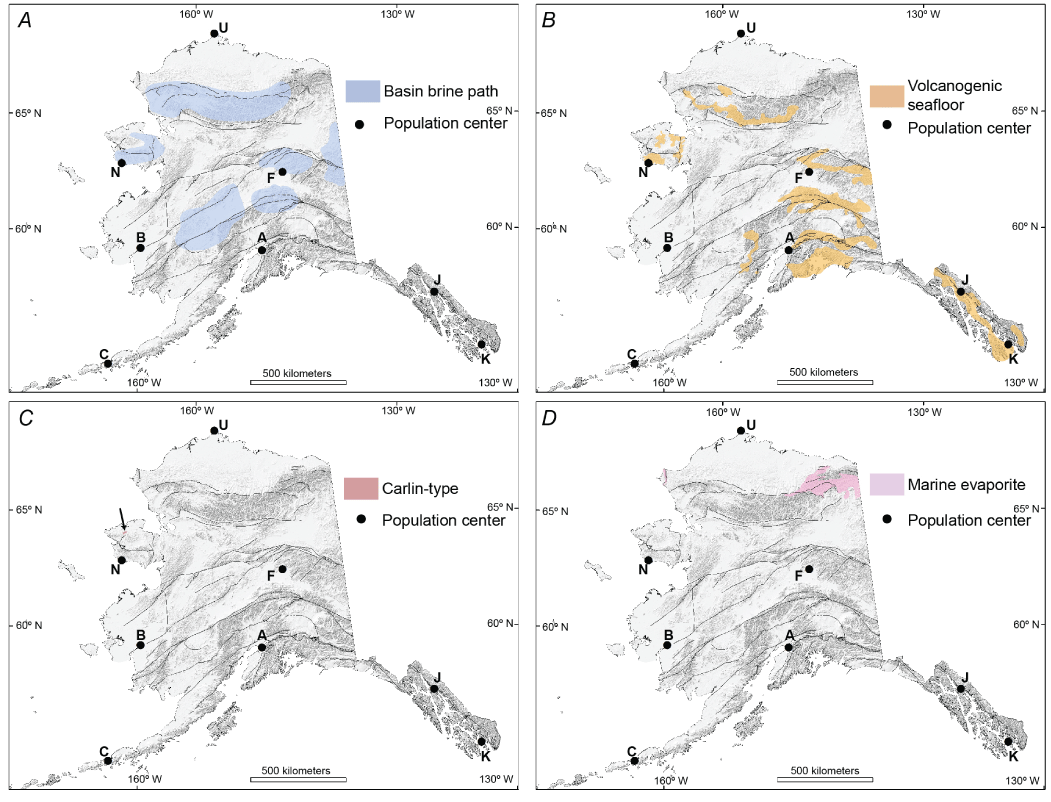
Mineral system focus areas in Alaska. A, is basin brine path mineral system focus areas, B, is volcanogenic seafloor mineral system focus areas, C, is Carlin-type mineral systems focus areas (arrow points to small focus area north of Nome), and D, is marine evaporite mineral system focus areas. The plates are all depicted using the same field of view and base map as figure 1. Base map is from the National Elevation Dataset (NED), USGS. [Population centers: U, Utqiagvik; N, Nome; F, Fairbanks; B, Bethel; A, Anchorage; C, Cold Bay; J, Juneau; K, Ketchikan.]
Basin Brine Path
Most basin brine path mineral systems were covered in phase 2 of Earth MRI (Kreiner and Jones, 2020). New focus areas were added during the current phase covering additional tectonic settings described below. For the readers benefit, the following description of the mineral system is repeated and summarized from Kreiner and Jones (2020, p. 7–8). Basin brine path mineral systems generally form through circulation of marine or terrestrial brines through permeable strata to upwelling and discharge sites where an ore deposit could form if appropriate conditions exist. The fluids are principally derived from evaporation of seawater resulting in high-salinity formational waters, or dissolution of seawater evaporites (halite, gypsum, and others) in the sedimentary sections, resulting in high-salinity basin brines (Emsbo, 2009; Hofstra and Kreiner, 2020). Fluids are circulated by topographic or tectonic drivers, ambient geothermal heat in the crust, or magma emplacement. Fluids will typically flow along lithologic contacts that have strong rheological contrast, flow through fault and fracture networks, or circulate in permeable lithologic units. Mineral deposits form in systems where (1) fluids were able to effectively scavenge metals and transport them as metal-chloride complexes along the flow paths, and (2) favorable traps exist to effectively reprecipitate the metals as ore minerals. Traps can be physical (temperature gradients, depressurization) or chemical (mixing of fluids, interaction with sulfide-bearing rocks, or others).
In general, basin brine path mineral systems are prospective for numerous critical minerals that can include Li, Sn, Co, PGE, REE, Ge, Ga, In, V, U, Re, Sc, barite, and Sr (table 2). However, local geologic controls will influence the style and geochemistry of the mineralizing system. In Alaska, we identified two general sedimentary environments in which basin brine path systems can form. Some focus areas outline strata that formed in foreland basins and have potential for Pb-Zn-Ag(-Co-Ge-Ga-In-Bi) MVT-style deposits. These deposits form where fluids flow along basement-carbonate contacts. Other focus areas identify strata that characterize passive margin settings, continental rifts, continental sag basins, and backarc basins. These sedimentary environments have potential for hosting clastic-dominated Pb-Zn deposits (Leach and others, 2010) and were previously known as sedimentary exhalative, or SEDEX, deposits.
The Red Dog Zn-Pb-Ag district in northwestern Alaska contains numerous shale-hosted Zn-Pb sulfide and barite deposits (Leach and others, 2004), and the orebodies in the district contain one of the world’s largest sources of zinc. The orebodies are contained in multiple thrust fault panels that offset the host Carboniferous Kuna Formation. Other strata that have similar lithologies, ages, and structural histories are known or expected to occur across the entire Brooks Range, which is outlined by our northernmost focus area for basin brine path mineral systems (fig. 2A; Brooks Range zinc belt; Leach and others, 2010; Kelley and others, 2021). Other regions that have potential for basin brine path minerals systems across the state include the Seward Peninsula, central Alaska Range, east-central Alaska, and areas underlain by the Farewell terrane in west-central Alaska (fig. 2A). These focus areas were updated from Kreiner and Jones (2020) to include newly published prospectivity maps of sediment-hosted Pb-Zn deposits (Kelley and others, 2021). These data-driven prospectivity maps were developed using geochemical data; the presence of appropriate lithologies in the stratigraphy that would permit formation of basin brines and provide sources of metals; the presence of potential host rocks (carbonaceous rocks for clastic-dominated deposits and carbonate rocks for MVT deposits); and the presence of known mineral occurrences that show alteration, mineralogy, and geochemical characteristics consistent with basin brine path mineral systems (Kelley and others, 2021). Critical mineral potential in these focus areas in Alaska includes Ga, Co, Ge, and In, and could include Sn. Some of the phase 3 focus areas overlap with focus areas previously delineated for phase 2 critical minerals, particularly those for sediment-hosted copper systems in the southern Brooks Range (for example, Bornite, Hitzman and others, 1986; Kreiner and Jones, 2020).
Volcanogenic Seafloor
Volcanogenic seafloor systems include a range of massive sulfide deposits that are strata-bound and formed at, or near, the seafloor in spatial, temporal, and genetic association with contemporaneous volcanism (Franklin and others, 2005; Shanks and Thurston, 2012). The principal fluid responsible for transporting metals and sulfur in the system is seawater that is modified, or evolved, by convection through hot volcanic rocks (Franklin and others, 2005). In areas where volcanogenic seafloor systems form in an arc setting, magmatic fluid inputs can play a key role in providing metals and sulfur to the system (de Ronde and others, 2005; Hannington and others, 2005). Metals that are present in a volcanogenic seafloor system largely reflect the host lithology and overall tectonic setting. Deposits dominated by mafic rocks are commonly characteristic of primitive oceanic backarcs or mature oceanic backarcs; they commonly have Zn-Cu-dominant signatures and lower lead (Hannington and others, 2005). In contrast, bimodal volcanic suites formed in incipient rifted supra-subduction oceanic arcs and (or) rifted supra-subduction epicontinental arcs have Zn-Pb-dominant signatures and lower Cu (Hannington and others, 2005). Gold content is variable and is typically controlled by a complex relationship between temperature, activity of sulfur, boiling, and precipitation mechanisms (Hannington and others, 2005). Critical minerals that could be enriched in volcanogenic seafloor systems variably include As, barite, Bi, Co, Ga, Ge, In, Mn, Sb, Sn, and Te (table 2).
Focus areas prospective for volcanogenic seafloor systems were developed based on an analysis that relied heavily on host stratigraphy as a primary component because of the stratigraphic nature of the deposits. The Alaska digital geologic map database (Wilson and others, 2015) was queried to identify units that host known volcanogenic seafloor deposits in the state. The Alaska Geochemical Database (version 3.0; Granitto and others, 2019) was also queried to identify rock and stream sediment samples that contained elevated Cu, Pb, Zn, Co, Cd, and Ag values because these elements are enriched in volcanogenic seafloor systems and help distinguish from other base and precious metal systems. The ARDF was also queried to identify records that contained keywords describing alteration and mineralization that are indicative of volcanogenic seafloor systems.
Across the state (fig. 2B), 13 focus areas are delineated that are prospective for volcanogenic seafloor mineral systems. Multiple focus areas contain active mines and (or) active exploration projects. The large focus area in southeastern Alaska contains the Greens Creek Ag-Au-Pb-Zn stratiform deposit that is being actively mined (Taylor and Johnson, 2010). The same focus area also includes the Palmer Cu-Zn-Au-Ag deposit to the northwest that is the focus of active exploration. In the southern Brooks Range, the east-west trending focus area contains the Ambler Cu-Zn-Pb deposit (Hitzman and others, 1986; Dicken and others, 2021). The remaining focus areas outline regions that have favorable geology and past and (or) present exploration for volcanogenic seafloor deposits in eastern interior Alaska, southcentral Alaska, the western Alaska Range, and the Seward Peninsula (fig. 2B; Dicken and others, 2021).
Carlin-type
Carlin-type mineral systems generally form in regions experiencing active extension of favorable stratigraphy in the presence of an elevated geothermal gradient. The only unequivocal Carlin-type mineral systems are found in Nevada, where favorable stratigraphy is characterized by a shelf-slope sequence of clastic and carbonate units deposited on the western margin of Laurentia (Cline and others, 2005). These sequences were overthrust by deeper marine siliciclastic and basaltic rocks during the Antler orogeny. Thrust faulting created steeply dipping fluid conduits and resulted in finer-grained, less permeable strata thrust over the more permeable carbonate-rich successions, creating stratigraphic “traps” for reactive fluids (Hofstra and Cline, 2000). The principal ore fluids in these systems are carbonic surface waters that convected through the upper crust and scavenged Au, As, Sb, Tl, Hg, and minor Ag from the pyritic carbonate rocks (Hofstra and Cline, 2000; Seedorff and Barton, 2004; Cline and others, 2005). Some authors argue for significant input of magma and (or) magmatic fluids in the formation of Carlin-type deposits (for example, Muntean and others, 2011). However, most deposits lack any clear spatial relationship to contemporaneous magmatism and do not have fluid compositions that indicate derivation from crystallizing magma (Seedorff and Barton, 2004).
Carlin-type mineral systems have not yet been recognized in Alaska. A series of occurrences in western Yukon Territory, Canada, have been described as Carlin-like (Pinet and others, 2018), and they are hosted in strata associated with the Selwyn basin that project toward the Alaska border. However, the prospective strata are truncated in western Yukon by the Tintina fault, a major regional structure that accommodated at least 430 kilometers of dextral displacement in the early Cenozoic (Gabrielse and others, 2006). It remains unclear if similar Selwyn basin strata exist south of the Tintina fault, and potentially correlative parts of the parautochthonous Yukon-Tanana assemblage in eastern Alaska have a much different, more intense structural and metamorphic history (Dusel-Bacon and others, 2006). Thus, we chose not to delineate a focus area for Carlin-type systems in eastern Alaska because of these discrepancies and associated uncertainty (fig. 2C). We did identify one focus area on the northwestern Seward Peninsula (fig. 2C) that is characterized by disseminated, fine-grained gold and arsenopyrite that are hosted in a carbonate and siliciclastic sequence. An associated mineral occurrence known as the Kelley Creek locality (ARDF #TE069) has been intermittently explored, and anomalous gold was encountered during surface exploration. Based on available information, the Kelley Creek occurrence and surrounding geology meet some criteria for Carlin-type mineral systems.
Marine Evaporite
Marine evaporite systems are characterized by closed or restricted epicontinental basins in arid to hyper-arid climatic zones (Raup 1991; Warren, 2010). Elements of interest in this environment are concentrated through continual evaporation with minimal fresh inputs, resulting in the increase of salinity. These deposits form at paleolatitudes equivalent to modern “horse latitudes” where seawater evaporation is at its maximum (Warren, 2010). Evaporation is particularly intense in areas where seawater is trapped in sub-sea level depressions, and evaporite minerals typically precipitate in order from gypsum or anhydrite to halite and, finally, sylvite.
Alaska is presently located at northern latitudes that are not favorable for evaporite formation. However, much of the state is underlain by exotic terranes accreted onto the western margin of North America in the Mesozoic and Cenozoic (Colpron and others, 2006; Colpron and Nelson, 2011). Some of these terranes are known or suspected to contain marine evaporite systems that formed prior to accretion. In particular, the Lisburne Group in northern Alaska contains marine chemocline systems that formed at much lower latitudes in the Paleozoic (for example, Dumoulin and others, 2013). The stratigraphy shows that the latest Mississippian climate became increasingly arid, resulting in the deposition of red beds in the upper Endicott Formation and anhydrite contained in the basal Lisburne. Anhydrite-bearing red beds also occur in the Prudhoe Bay region (Bird and Jordan, 1977). Quartz and calcite replacing evaporites are observed in the northeastern Brooks Range (Watts and others, 1994). Shallow-water facies of the Lisburne Group in the easternmost Brooks Range (fig. 2D) contain anhydrite and rare halite (Dumoulin and others, 2013). Seismic data indicate a fault-bounded evaporite basin with diapiric, pillow, and withdrawal structures in the lower Ellsemerian strata of the Lisburne Group (Sherwood and others, 2002) in far northwest Alaska (fig. 2D) and extending offshore into the Arctic Ocean.
Discussion
Statewide publicly available geologic and geochemical datasets combined with published data-driven geospatial mineral prospectivity analyses form the basis of Alaska Earth MRI focus areas (fig. 2A–D) defined for phase 3 critical minerals. A mineral systems approach is adopted to identify focus areas where they contain the necessary geological ingredients required to generate deposits that may contain critical minerals. Current and historical production of base, precious, and critical minerals occurs in some focus areas (for example, Red Dog), and others contain identified resources known to contain critical minerals (for example, Arctic), as delineated in Dicken and others (2021). Focus areas are broad and reflect gaps in existing or modern data coverages and quality, which are needed to refine mineral system outlines in a large, remote, and geologically complex state.
This report delineates 22 new Alaska Earth MRI phase 3 focus areas. The focus areas are listed in Dicken and others (2021) for the four identified mineral systems (fig. 2). An additional 55 focus areas that were previously defined for 10 mineral systems in Phase 2 and discussed in Dicken and Hammarstrom (2020) and Kreiner and Jones (2020) are also prospective for phase 3 critical minerals. As a result, these mineral systems are included in the following discussion and accompanying data release (Dicken and others, 2021). Collectively, phase 3 focus areas span large regions of the state and occur in multiple, diverse geological belts. To help prioritize new geological, geophysical, and geochemical data collection in Alaska, the authors mapped the number of phase 3 focus areas that occur within each 1:63,360-scale quadrangle in the state. Each 1:63,360-scale quadrangle contains at least one focus area to a maximum of nine (fig. 3). The resulting data (table 4) indicate that 1,979 (~66 percent) of the 3,011 quadrangles at a scale of 1:63,360 in the state contain at least one focus area that has potential for phase 3 critical mineral commodities. More than 400 quadrangles have six or more overlapping focus areas that are permissive or prospective for phase 3 critical minerals together with other primary and secondary commodities (table 2). We also considered all focus areas and associated mineral systems that have been developed through Earth MRI in Alaska (Dicken and others, 2019, 2021; Dicken and Hammarstrom, 2020; Kreiner and Jones, 2020) and mapped the number that occur within each 1:63,360 quadrangle in the state. In the state, 17 known or suspected mineral systems have the potential to contain one or more critical minerals (table 2). However, no quadrangles in the state contain an overlap of more than 12 of the 17 mineral systems. In Alaska, 2,081 quadrangles (1:63,360 scale) have evidence for at least one mineral system of interest, and 32 quadrangles exhibit evidence for as many as 12 overlapping mineral systems (fig. 4; table 5).
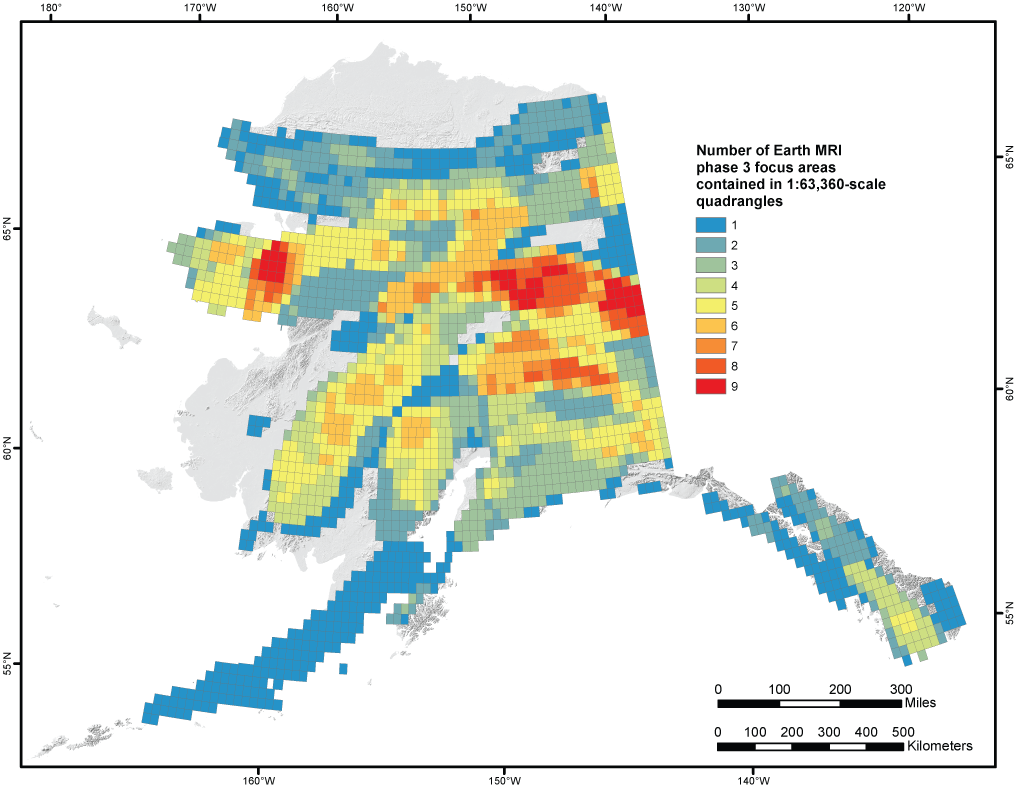
Overlap of mineral system focus areas for Earth MRI phase 3 in Alaska. The 1:63,360-scale quadrangles contain one or more phase 3 Earth MRI focus areas. Colors correspond to the number of focus areas the quadrangles contain; values range from 1 (blue) to 9 (red) (table 4).
Table 4.
Alaska 1:63,360-scale quadrangles containing one or more Earth Mapping Resources Initiative phase 3 focus areas.[Focus areas for the following mineral systems: basin brine path, Carlin-type, IOA-IOCG, mafic magmatic, magmatic REE, marine chemocline, meteoric recharge, orogenic, placer, porphyry Cu-Mo-Au, porphyry Sn, reduced intrusion-related, volcanogenic seafloor. See figure 3]
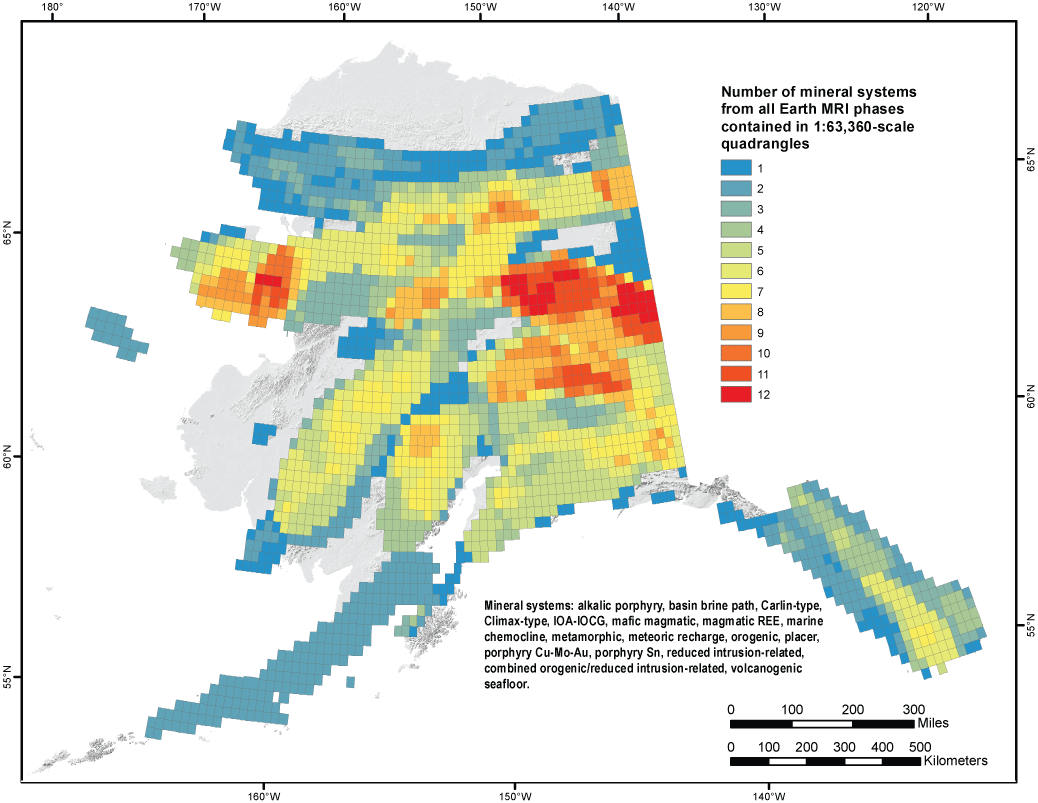
Overlap of mineral system focus areas for all Earth MRI phases in Alaska. The 1:63,360-scale quadrangles contain one or more Earth MRI focus areas of any phase. Colors correspond to the number of focus areas the quadrangles contain, and values range from 1 (blue) to 12 (red) (table 5).
Table 5.
Alaska 1:63,360-scale quadrangles containing one or more mineral systems from all Earth Mapping Resources Initiative phases.[Mineral systems: alkalic porphyry, basin brine path, Carlin-type, Climax-type, IOA-IOCG, mafic magmatic, magmatic REE, marine chemocline, marine evaporite, metamorphic, meteoric recharge, orogenic, placer, porphyry Cu-Mo-Au, porphyry Sn, reduced intrusion-related, combined orogenic/reduced intrusion-related, volcanogenic seafloor. See figure 4]
The mineral systems described herein and in Kreiner and Jones (2020) were identified in support of Earth MRI phase 1, 2, and 3 activities. They cover a broad range of critical mineral commodities listed in Fortier and others (2018). We also note that these same mineral systems account for all other nonfuel-related critical commodities that might be present in Alaska (table 2). Thus, we expect the focus areas delineated to date to suffice for any additional phases of Earth MRI planning and prioritization. Regions of the state where the greatest mineral system overlap occurs are the Yukon-Tanana upland and environs in east-central Alaska, the central and eastern Alaska Range, and parts of the Seward Peninsula (fig. 4). These and other areas, which have the most overlap of mineral systems containing critical minerals, are expected to have the highest potential for new discoveries. Prioritizing new data collection in these regions could be an efficient and effective way to develop a more complete and modern understanding of the deposit types and styles that are present, and how associated critical minerals are mobilized and concentrated in a variety of geologic environments. Note that some regions that have less overlap also contain significant known prospects or deposits (for example, the Pebble deposit, western Alaska Range and Bokan Mountain in southeast Alaska; Kreiner and Jones, 2020). Thus, there are areas with potential for a smaller number of mineral systems, but the mineral system(s) that are present were particularly productive given the geologic environment and history. In these cases, known but undeveloped and (or) undiscovered deposits or prospects might have more significant potential for new critical minerals either as primary products, coproducts, or byproducts despite occurring in regions with fewer overlapping systems. Therefore, considering the overlap of mineral systems together with the state of knowledge and modern data in any region is important when prioritizing areas for new mapping and data collection.
Summary
Alaska focus areas for phase 3 of Earth MRI, which include potential for antimony, barite, beryllium, chromium, fluorspar, hafnium, magnesium, manganese, uranium, vanadium, and zirconium, have been defined based on a data-driven, mineral systems approach that uses publicly available statewide datasets to map prospectivity for a variety of mineral deposit groups. The prospectivity maps and associated data delineate regions that are prospective for mineral systems that could contain critical minerals of current interest. In addition, the statewide prospectivity analyses identify key gaps in existing datasets that highlight the need for new data collection throughout Alaska. Prioritization of data acquisition through the Earth MRI program is informed by the data gaps highlighted in the prospectivity analyses.
Four additional mineral systems discussed here and 10 previously discussed mineral systems contain high prospectivity for Earth MRI phase 3 critical minerals in Alaska. Evaluation of phase 3 critical minerals has resulted in 22 new and 55 previous focus areas identified in regions that are favorable for mineral systems that could contain the critical minerals. In total, 102 focus areas and 17 mineral systems containing high prospectivity for critical minerals have been identified in Alaska. Evaluating the amount of spatial overlap at the quadrangle scale provides a useful tool for prioritizing regions for new Earth MRI geologic, geophysical, and geochemical data acquisition. These regions exhibit evidence for the greatest variety of prospective mineral systems.
References Cited
Bird, K.J., and Jordan, C.J., 1977, Lisburne Group (Mississippian and Pennsylvanian), potential major hydrocarbon objective of Arctic Slope, Alaska: AAPG Bulletin, v. 61, no. 9, p. 1493–1512. [Also available at http://doi.org/10.1306/C1EA45BD-16C9-11D7-8645000102C1865D.]
Cline, J.S., Hofstra, A.H., Muntean, J.L., Tosdal, R.M., and Hickey, K.A., 2005, Carlin-type gold deposits in Nevada—Critical geologic characteristics and viable models, in Hedenquist, J.W., Thompson, J.F.H., Goldfarb, R.J., and Richards, J.P., eds., Economic geology 100th anniversary volume: Littleton, Colorado, Society of Economic Geologists, Inc., p. 451–484. [Also available at http://doi.org/10.5382/AV100.15.]
Colpron, M., and Nelson, J.L., 2011, A Palaeozoic NW Passage and the Timanian, Caledonian and Uralian connections of some exotic terranes in the North American Cordillera: Geological Society of London Memoirs 35, p. 463–484. [Also available at http://doi.org/10.1144/M35.31.]
Colpron, M., Nelson, J.L., and Murphy, D.C., 2006, A tectonostratigraphic framework for the pericratonic terranes of the northern Canadian Cordillera, in Colpron, M., and Nelson, J.L., eds., Paleozoic evolution and metallogeny of pericratonic terranes at the ancient pacific margin of North America, Canadian, and Alaskan Cordillera: St. John’s, Newfoundland, Geological Association of Canada, p. 1–23.
Day, W.C., 2019, The Earth Mapping Resources Initiative (Earth MRI)—Mapping the Nation’s critical mineral resources: U.S. Geological Survey Fact Sheet 2019–3007, 2 p., accessed February 26, 2019, at http://doi.org/10.3133/fs20193007.
de Ronde, C.E.J., Hannington, M.D., Stoffers, P., Wright, I.C., Ditchburn, R.G., Reyes, A.G., Baker, E.T., Massoth, G.J., Lupton, J.E., Walker, S.L., Greene, R.R., Soong, C.W.R., Ishibashi, J., Lebon, G.T., Bray, C.J., and Resing, J.A., 2005, Evolution of a submarine magmatic-hydrothermal system—Brothers volcano, southern Kermadec Arc, New Zealand: Economic Geology and the Bulletin of the Society of Economic Geologists, v. 100, no. 6, p. 1097–1133. [Also available at http://doi.org/10.2113/gsecongeo.100.6.1097.]
Dicken, C.L., and Hammarstrom, J.M., 2020, GIS for focus areas of potential domestic resources of 11 critical minerals—Aluminum, cobalt, graphite, lithium, niobium, platinum group elements, rare earth elements, tantalum, tin, titanium, and tungsten: U.S. Geological Survey data release, accessed January 2021, at http://doi.org/10.5066/P95CO8LR.
Dicken, C.L., Hammarstrom, J.M., Woodruff, L.G., and Mitchell, R.J., 2021, GIS, supplemental data table, and references for focus areas of potential domestic resources of 13 critical minerals in the United States and Puerto Rico—Antimony, barite, beryllium, chromium, fluorspar, hafnium, helium, magnesium, manganese, potash, uranium, vanadium, and zirconium: U.S. Geological Survey data release, http://doi.org/10.5066/P9WA7JZY.
Dicken, C.L., Horton, J.D., San Juan, C.A., Anderson, A.K., Ayuso, R.A., Bern, C.R., Bookstrom, A.A., Bradley, D.C., Bultman, M.W., Carter, M.W., Cossette, P.M., Day, W.C., Drenth, B.J., Emsbo, P., Foley, N.K., Frost, T.P., Gettings, M.E., Hammarstrom, J.M., Hayes, T.S., Hofstra, A.H., Hubbard, B.E., John, D.A., Jones, J.V.,III, Kreiner, D.C., Lund, K., McCafferty, A.E., Merschat, A.J., Ponce, D.A., Schulz, K.J., Shah, A.K., Siler, D.L., Taylor, R.D., Vikre, P.G., Walsh, G.J., Woodruff, L.G., and Zurcher, L., 2019, GIS and data tables for focus areas for potential domestic nonfuel sources of rare earth elements: U.S. Geological Survey data release, accessed February 2021, at http://doi.org/10.5066/P95CHIL0.
Dumoulin, J.A., Johnson, C.A., Slack, J.F., Bird, K.J., Whalen, M.T., Moore, T.E., Harris, A.G., and O’Sullivan, P.B., 2013, Carbonate margin, slope, and basin facies of the Lisburne Group (Carboniferous–Permian) in northern Alaska, in Verwer, K., Playton, T., and Harris, P., eds., Deposits, Architecture, and Controls of Carbonate Margin, Slope, and Basin Systems: SEPM Society for Sedimentary Geology, v. 105, p. 211–236. [Also available at http://doi.org/10.2110/sepmsp.105.02.]
Dusel-Bacon, C., Hopkins, M.J., Mortensen, J.K., Dashevsky, S.S., Bressler, J.R., and Day, W.C., 2006, Paleozoic tectonic and metallogenic evolution of the pericratonic rocks of east-central Alaska and adjacent Yukon, in Colpron, M., and Nelson, J., eds., Paleozoic evolution and metallogeny of pericratonic terranes at the ancient Pacific margin of North America: Geological Association of Canada, Special Paper 45, p. 25–74.
Emsbo, P., 2009, Geologic criteria for the assessment of the sedimentary exhalative (sedex) Zn-Pb-Ag deposits: U.S. Geological Survey Open-File Report 2009–1209, 21 p., accessed February 2021, at http://doi.org/10.3133/ofr20091209.
Energy Information Administration, 2021, Nuclear Energy Overview—Uranium Overview: Monthly Energy Review, U.S. Energy Information Administration, accessed February 23, 2021, at https://www.eia.gov/totalenergy/data/monthly/pdf/sec8_5.pdf.
Fortier, S.M., Nassar, N.T., Lederer, G.W., Brainard, J., Gambogi, J., and McCullough, E.A., 2018, Draft critical mineral list—Summary of methodology and background information—U.S. Geological Survey technical input document in response to Secretarial Order No. 3359: U.S. Geological Survey Open-File Report 2018–1021, 15 p., accessed December 15, 2018, at http://doi.org/10.3133/ofr20181021.
Franklin, J.M., Gibson, H.L., Jonasson, I.R., and Galley, A.G., 2005, Volcanogenic massive sulfide deposits, in Hedenquist, J.W., Thompson, J.F.H., Goldfarb, R.J., and Richards, J.P., eds., Economic geology 100th anniversary volume: Littleton, Colorado, Society of Economic Geologists, Inc., p. 523–560.
Gabrielse, H., Murphy, D.C., Mortensen, J.K., Haggart, J.W., Enkin, R.J., and Monger, J.W.H., 2006, Cretaceous and Cenozoic dextral orogen-parallel displacements, magmatism, and paleogeography, north-central Canadian Cordillera. Paleogeography of the North American Cordillera—Evidence for and Against Large-Scale Displacements: Geological Association of Canada Special Paper 46, p. 255–276.
Granitto, M., Wang, B., Shew, N.B., Karl, S.M., Labay, K.A., Werdon, M.B., Seitz, S.S., and Hoppe, J.E., 2019, Alaska geochemical database version 3.0 (AGDB3)—Including “best value” data compilations for rock, sediment, soil, mineral, and concentrate sample media: U.S. Geological Survey Data Series 1117, 33 p., accessed January 2021, at http://doi.org/10.3133/ds1117.
Hammarstrom, J.H., and Dicken, C.L., 2019, Focus areas for data acquisition for potential domestic sources of critical minerals—Rare earth elements, chap. A of U.S. Geological Survey, Focus areas for data acquisition for potential domestic sources of critical minerals: U.S. Geological Survey Open-File Report 2019–1023–A, 11 p., accesses January 2021, at http://doi.org/10.3133/ofr20191023A.
Hammarstrom, J.M., Dicken, C.L., Day, W.C., Hofstra, A.H., Drenth, B.J., Shah, A.K., McCafferty, A.E., Woodruff, L.G., Foley, N.K., Ponce, D.A., Frost, T.P., and Stillings, L.L., 2020, Focus areas for data acquisition for potential domestic resources of 11 critical minerals in the conterminous United States, Hawaii, and Puerto Rico—Aluminum, cobalt, graphite, lithium, niobium, platinum-group elements, rare earth elements, tantalum, tin, titanium, and tungsten, chap. B of U.S. Geological Survey, Focus areas for data acquisition for potential domestic sources of critical minerals: U.S. Geological Survey Open-File Report 2019–1023–B, 67 p., accessed January 2021, at http://doi.org/10.3133/ofr20191023B.
Hammarstrom, J.M., Dicken, C.L., Hall, S., Brennan, S., Day, W.C., Hofstra, A.H., Drenth, B.J., Shah, A.K., McCafferty, A.E., Woodruff, L.G., Foley, N.K., Ponce, D.A., and Anderson, A.K., 2022, Focus areas for data acquisition for potential domestic resources of 13 critical minerals in the conterminous United States, and Puerto Rico—Antimony, barite, beryllium, chromium, fluorspar, hafnium, helium, magnesium, manganese, potash, uranium, vanadium, and zirconium, chap. D of U.S. Geological Survey, Focus areas for data acquisition for potential domestic sources of critical minerals: U.S. Geological Survey Open-File Report 2019–1023–D, 66 p, https://doi.org/10.3133/ofr20191023D.
Hannington, M.D., de Ronde, C.E.J., and Petersen, S., 2005, Sea-floor tectonics and submarine hydrothermal systems, in Hedenquist, J.W., Thompson, J.F.H., Goldfarb, R.J., and Richards, J.P., eds., Economic geology 100th anniversary volume: Littleton, Colorado, Society of Economic Geologists, Inc., p. 111–141.
Hitzman, M.W., Proffett, J.M., Jr., Schmidt, J.M., and Smith, T.E., 1986, Geology and mineralization of the Ambler District, northwestern Alaska: Economic Geology and the Bulletin of the Society of Economic Geologists, v. 81, no. 7, p. 1592–1618. [Also available at http://doi.org/10.2113/gsecongeo.81.7.1592.]
Hofstra, A.H., and Cline, J.S., 2000, Characteristics and models for Carlin-type gold deposits, chap. 5 of Hagemann, S.G. and Brown, P.E., eds., Gold in 2000, v. 13 of Hagemann, S.G. and Brown, P.E., eds., Reviews in economic geology: Littleton, Colorado, Society of Economic Geologists, Inc., p. 163–220.
Hofstra, A.H., and Kreiner, D.C., 2020, Systems-deposits-commodities-critical minerals table for the Earth Mapping Resources Initiative: U.S. Geological Survey Open-File Report 2020–1042, 24 p., accessed January 2021, at http://doi.org/10.3133/ofr20201042.
Jones, J.V., III, Karl, S.M., Labay, K.A., Shew, N.B., Granitto, M., Hayes, T.S., Mauk, J.L., Schmidt, J.M., Todd, E., Wang, B., Werdon, M.B., and Yager, D.B., 2015, GIS-based identification of areas with mineral resource potential for six selected deposit groups, Bureau of Land Management Central Yukon Planning Area, Alaska: U.S. Geological Survey Open-File Report 2015–1021, 78 p., 5 app., 12 pls., accessed January 2021, at http://doi.org/10.3133/ofr20151021.
Karl, S.M., Jones, J.V., III, and Hayes, T.S., eds., 2016, GIS-based identification of areas that have resource potential for critical minerals in six selected groups of deposit types in Alaska: U.S. Geological Survey Open-File Report 2016–1191, 99 p., 5 app., 12 pls., scale 1:10,500,000, accessed December 15, 2018, at http://doi.org/10.3133/ofr20161191.
Karl, S.M., Kreiner, D.C., Case, G.N., Labay, K.A., Shew, N.B., Granitto, M., Wang, B., and Anderson, E.D., 2021, GIS-based identification of areas that have resource potential for lode gold in Alaska: U.S. Geological Survey Open-File Report 2021–1041, 75 p., 9 pls, accessed March 2021, at http://doi.org/10.3133/ofr20211041.
Kelley, K.D., Graham, G.E., Labay, K.A., and Shew, N.B., 2021, GIS-based identification of areas that have resource potential for sediment-hosted Pb-Zn deposits in Alaska: U.S. Geological Survey Open-File Report 2020−1147, 37 p., 1 app., 2 pls., scale 1:10,500,000, accessed March 2021, at http://doi.org/10.3133/ofr20201147.
Kreiner, D.C., and Jones, J.V., III, 2020, Focus areas for data acquisition for potential domestic resources of 11 critical minerals in Alaska—Aluminum, cobalt, graphite, lithium, niobium, platinum group elements, rare earth elements, tantalum, tin, titanium, and tungsten, chap. C of U.S. Geological Survey, Focus areas for data acquisition for potential domestic sources of critical minerals: U.S. Geological Survey Open-File Report 2019–1023–C, 20 p., accessed March 2021, at http://doi.org/10.3133/ofr20191023C.
Kreiner, D.C., Jones, J.V., III, Kelley, K.D., and Graham, G.E., 2020, Tectonic and magmatic controls on the metallogenesis of porphyry deposits in Alaska, in Sharman, E.R., Lang, J.R., and Chapman, J.B., eds., Porphyry Deposits of the Northwestern Cordillera of North America—A 25-year update v. 57: Montreal, Quebec, Canadian Institute of Mining and Metallurgy Special, p. 134–175.
Leach, D.L., Marsh, E., Emsbo, P., Rombach, C.S., Kelley, K.D., and Anthony, M., 2004, Nature of hydrothermal fluids at the shale-hosted Red Dog Zn-Pb-Ag deposits, Brooks Range, Alaska: Economic Geology and the Bulletin of the Society of Economic Geologists, v. 99, no. 7, p. 1449–1480. [Also available at http://doi.org/10.2113/gsecongeo.99.7.1449.]
Leach, D.L., Taylor, R.D., Fey, D.L., Diehl, S.F., and Saltus, R.W., 2010, A deposit model for Mississippi Valley-type lead-zinc ores, chap. A of Mineral deposit models for resource assessment: U.S. Geological Survey Scientific Investigations Report 2010–5070–A, 52 p., accessed March 2021, at https://pubs.usgs.gov/sir/2010/5070/a/pdf/SIR10-5070A.pdf.
Muntean, J.L., Cline, J.S., Simon, A.C., and Longo, A.A., 2011, Magmatic-hydrothermal origin of Nevada’s Carlin-type gold deposits: Nature Geoscience, v. 4, no. 2, p. 122–127, accessed March 2021, at http://doi.org/10.1038/ngeo1064.
Pinet, N., Sack, P.J., Mercier-Langevin, P., Lavoie, D., Dubé, B., Lane, J., and Brake, V., 2018, Breccia styles and controls on carbonate replacement-type (‘Carlin-type’) gold zones, Rackla belt, east-central Yukon, v. 1 of Rogers, N., ed., Targeted Geoscience Initiative—2017 Report of Activities: Geological Survey of Canada Open File 8358, p. 163–168.
U.S. Geological Survey, 2008, Alaska resource data file, new and revised records, version 1.7: U.S. Geological Survey Open-File Report 2008–1225, 2605 p., accessed June 3, 2020, at https://ardf.wr.usgs.gov/ardf_data/1225.pdf.
U.S. Geological Survey, 2021, Mineral commodity summaries 2021: U.S. Geological Survey, 200 p., accessed March 15, 2021, at http://doi.org/10.3133/mcs2021.
Warren, J.K., 2010, Evaporites through time—Tectonic, climatic, and eustatic controls in marine and nonmarine deposits: Earth-Science Reviews, v. 98, no. 3-4, p. 217–268. [Also available at https://doi.org/10.1016/j.earscirev.2009.11.004.]
Watts, K.F., Harris, A.G., Carlson, R.C., Eckstein, M.K., Gruzlovic, P.D., Imm, T.A., Krumhardt, A.P., Lasota, D.K., Morgan, S.K., Enos, P., Goldstein, R.H., Dumoulin, J.A., and Mamet, B.L., 1994, Analysis of reservoir heterogeneities due to shallowing-upward cycles in carbonate rocks of the Upper Mississippian and Pennsylvanian Wahoo Limestone of northeastern Alaska: Department of Energy Open-File Report, Contract DOE/BC14471, 433 p.
Wilson, F.H., Hults, C.P., Mull, C.G., and Karl, S.M., comps., 2015, Geologic map of Alaska: U.S. Geological Survey Scientific Investigations Map 3340, pamphlet 196 p., 2 sheets, scale 1:1,584,000, accessed December 15, 2018, at http://doi.org/10.3133/sim3340.
World Nuclear Association, 2021, Uranium Production Figures, 2010–2019: World Nuclear Association website, accessed April 12, 2021, at https://www.world-nuclear.org/information-library/facts-and-figures/uranium-production-figures.aspx.
Publishing support provided by the U.S. Geological Survey
Science Publishing Network, Tacoma Publishing Service Center
For more information concerning the research in this report, contact the
Director, Alaska Science Center
U.S. Geological Survey
4210 University Drive
Anchorage, Alaska 99508
Disclaimers
Any use of trade, firm, or product names is for descriptive purposes only and does not imply endorsement by the U.S. Government.
Although this information product, for the most part, is in the public domain, it also may contain copyrighted materials as noted in the text. Permission to reproduce copyrighted items must be secured from the copyright owner.
Suggested Citation
Kreiner, D.C., Jones, J.V., III, and Case, G. N., 2022, Alaska focus area definition for data acquisition for potential domestic sources of critical minerals in Alaska for antimony, barite, beryllium, chromium, fluorspar, hafnium, magnesium, manganese, uranium, vanadium, and zirconium, chap. E of U.S. Geological Survey, Focus areas for data acquisition for potential domestic sources of critical minerals: U.S. Geological Survey Open-File Report 2019–1023, 19 p., https://doi.org/10.3133/ofr20191023E.
ISSN: 2331-1258 (online)
Study Area
Publication type | Report |
---|---|
Publication Subtype | USGS Numbered Series |
Title | Alaska focus area definition for data acquisition for potential domestic sources of critical minerals in Alaska for antimony, barite, beryllium, chromium, fluorspar, hafnium, magnesium, manganese, uranium, vanadium, and zirconium |
Series title | Open-File Report |
Series number | 2019-1023 |
Chapter | E |
DOI | 10.3133/ofr20191023E |
Year Published | 2022 |
Language | English |
Publisher | U.S. Geological Survey |
Publisher location | Reston, VA |
Contributing office(s) | Alaska Science Center Geology Minerals |
Description | Report: vii, 19 p.; Data Release |
Country | United States |
State | Alaska |
Online Only (Y/N) | Y |
Additional Online Files (Y/N) | N |
Google Analytic Metrics | Metrics page |