Quality of Groundwater Used for Domestic Supply in the Modesto, Turlock, and Merced Subbasins of the San Joaquin Valley, California
Links
- Document: Report (6.3 MB pdf) , HTML , XML
- Data Release: USGS data release - Groundwater-quality data in the Modesto-Turlock-Merced Domestic-Supply Aquifer Study Unit, 2020-2021: Results from the California GAMA Priority Basin Project
- Download citation as: RIS | Dublin Core
Summary
More than 2 million Californians rely on groundwater from privately owned domestic wells for drinking-water supply. This report summarizes a water-quality survey of domestic and small-system drinking-water supply wells in the Modesto, Turlock, and Merced subbasins of the San Joaquin Valley where more than 78,000 residents are estimated to use privately owned domestic wells. Results indicate that inorganic and organic constituents in groundwater were respectively present above regulatory (maximum contaminant level, MCL) benchmarks for public drinking-water quality in 37 percent and 9 percent of the aquifer area used for domestic drinking-water supplies (herein, “domestic groundwater resources”; fig. 1).
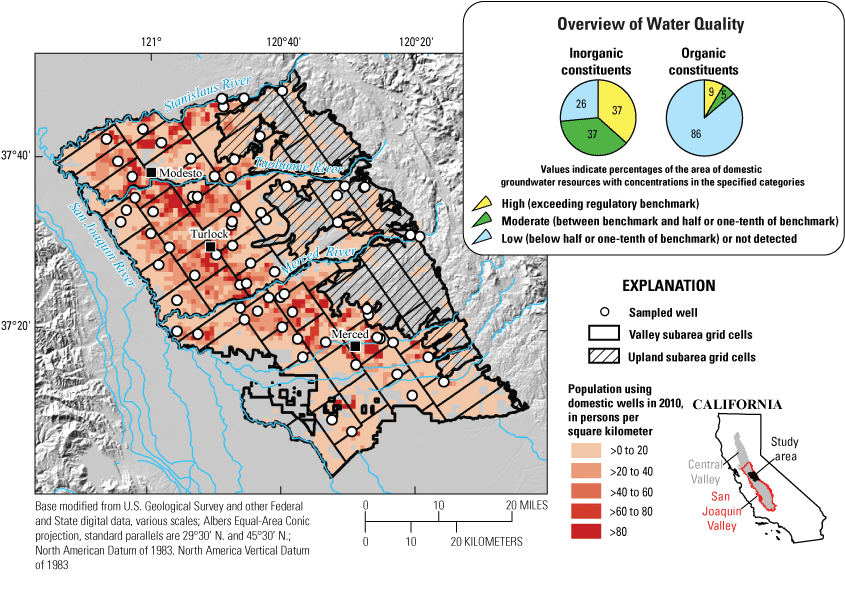
Modesto, Turlock, and Merced subbasins of the San Joaquin Valley showing estimated population using domestic wells, sampling grid cells for Valley and Upland study subareas, and location of wells sampled to characterize the quality of groundwater resources used for domestic drinking-water supply (Johnson and Belitz, 2019; Balkan and others, 2022). Overview of water-quality inset shows proportions of domestic groundwater resources in the study area having low, moderate, or high concentrations of inorganic and organic constituents with regulatory maximum contaminant level benchmarks.
The most prevalent inorganic constituents exceeding regulatory benchmarks were nitrate, uranium, and arsenic. The only organic constituents exceeding regulatory benchmarks were the fumigant constituents 1,2,3-trichloropropane (1,2,3-TCP) and 1,2-dibromo-3-chloropropane (DBCP), but the herbicides atrazine and simazine were detected at low concentrations below one-tenth of regulatory benchmarks in 30 percent of domestic groundwater resources. Total dissolved solids (TDS) and manganese exceeded aesthetic-based (secondary maximum contaminant level [SMCL]) benchmarks for drinking water in 3 percent and 13 percent of domestic groundwater resources, respectively. Per- and polyfluoroalkyl substances (PFAS) were detected in 23 percent of domestic groundwater resources, with 4 percent exceeding California state notification or response levels for specific compounds. Total coliform bacteria were detected in 20 percent of domestic groundwater resources.
Elevated concentrations of nitrate, uranium, TDS, and pesticides (fumigant constituents and herbicides) are related to agricultural land use and were typically present at shallow depths up to 75 meters below land surface. Agriculturally derived constituents were detected in wells screened below the Corcoran Clay Member of the Tulare Formation (herein, “Corcoran Clay”) in the southeastern part of the study area, where the Corcoran Clay tends to be shallower and thinner than in areas to the northwest. Nitrate, uranium, and TDS were most prevalent in the northwest part of the study area proximal to the valley trough where soils are poorly drained and agricultural land uses are predominantly grain, alfalfa, and dairy farms. Pesticides tended to occur in groundwater below coarse-grained surficial deposits and within a northwest to southeast trending band along the eastern extent of the Corcoran Clay that typically demarcates the western extent of well-drained soils associated with perennial orchard crops. Elevated concentrations of arsenic tended to occur west of this band in reducing groundwater but also sometimes co-occurred with elevated nitrate in oxic groundwater, most likely because of geochemical conditions in agriculturally affected groundwater that can enhance the mobility of arsenic from aquifer sediments.
Introduction
Approximately 85 percent of the residential population of California depends in some part on groundwater for drinking-water supply (Chappelle and others, 2017). To protect this vital resource, the California State Water Resources Control Board (SWRCB) created the Groundwater Ambient Monitoring and Assessment (GAMA) program in 2000. The purpose of GAMA is to comprehensively assess groundwater quality throughout California and enhance public access to groundwater-quality information and analysis. The U.S. Geological Survey implements the Priority Basin Project (PBP) of the GAMA program, which provides spatially unbiased assessments of groundwater resources used for drinking-water supply on a basin scale.
The first phase of the GAMA-PBP (2004–12) focused on groundwater wells used for public drinking-water supply (Belitz and others, 2015). Presently, the GAMA-PBP is assessing the status of groundwater resources used for domestic drinking-water supply (U.S. Geological Survey, 2018). Approximately 2 million California residents use domestic or small-system (with fewer than 15 service connections) wells for their drinking-water supply (California State Water Resources Control Board, 2015). Unlike public-supply wells that are routinely monitored for water quality by law, private domestic wells are not regulated for water quality, and much less is known about the quality of domestic drinking-water resources on a regional scale. Domestic wells tend to be drilled to shallower depths than public-supply wells and can provide important information on groundwater quality in shallower parts of aquifer systems that respond more rapidly to changes at the land surface compared to deeper production wells (Burow and others, 2008; Voss and others, 2019).
During 2020–21, the GAMA-PBP made a spatially unbiased survey of the quality of groundwater resources used for domestic drinking-water supply in the Modesto, Turlock, and Merced subbasins of the San Joaquin Valley (Balkan and others, 2022), where it is estimated that more than 78,000 residents use domestic wells for household drinking-water supplies (Johnson and Belitz, 2019). Groundwater subbasins (as defined by California Department of Water Resources, 2020) were separated into a “Valley” subarea, where domestic well density was greater, and an “Uplands” subarea of sparser domestic well density defined by the extent of Pliocene- to Pleistocene-aged alluvial sediments on the eastern boarder of the Central Valley (fig. 1; Saucedo and others, 2000). The respective subareas were subsequently divided into grid cells with the objective of sampling at least one well used for domestic or small-system supply per cell to assess groundwater quality across the total aquifer area used for domestic drinking-water supply in Valley and Upland subareas. The Valley subarea was divided into 52 equal-area grid cells of approximately 60 square kilometers (km2), and the Upland subarea was divided into 10 equal-area grid cells of 110 km2 to account for the coarser sampling resolution in the more sparsely populated Uplands. During 2020–21, the GAMA-PBP sampled a total of 71 domestic or small-system wells for water quality distributed across 46 Valley grid cells and 7 Upland grid cells (fig. 1; Balkan and others, 2022).
Hydrogeologic Setting
The study area covers about 4,300 km2 and is centrally located on the east side of the San Joaquin River Basin within California’s Central Valley (fig. 1), a highly productive agricultural region where large residential populations depend on groundwater for irrigation and drinking-water supply. The Central Valley is an asymmetric structural trough containing up to 10 kilometers (km) of marine and continental sediments and bordered to the east and west by the Sierra Nevada and Coast Ranges, respectively (Faunt, 2009). Study subbasins are bounded by three primary tributaries of the San Joaquin River (the Stanislaus, Tuolumne, and Merced Rivers) that drain primary watersheds of the Sierra Nevada to the east and are intensively managed for irrigation, flood control, and power generation (Phillips and others, 2015).
Average annual precipitation is about 330 millimeters (mm) and 370 mm in Valley and Upland subareas, respectively (PRISM Climate Group, 2015), and follows a distinct seasonal pattern with cool, wet winters and hot, dry summers. Land uses in the Valley subarea of the study area are primarily agricultural (about 70 percent) and urban (about 20 percent), transitioning to predominantly natural grassland in the Upland area to the east (about 75 percent; Multi-Resolution Land Characteristics Consortium, 2022). Primary crop types are almonds, walnuts, peaches, grapes, grain, corn, pasture, and alfalfa (Phillips and others, 2015). Groundwater is often used to supplement surface-water diversions for irrigation during the summer dry season and during extended regional droughts (Faunt, 2009).
Aquifer systems in the study area consist of overlapping alluvial fan sediments of Tertiary to Quaternary age deposited by tributaries of the San Joaquin River draining the Sierra Nevada (Burow and others, 2004). Alluvial aquifer systems in the study area tend to be unconfined close to the water table and transition to semi-confined conditions with depth because of the presence of abundant, discontinuous clay lenses in aquifer sediments which tend to fine downwards (Burow and others, 2004). A large, regionally extensive confining unit known as the Corcoran Clay Member of the Tulare Formation (herein, “Corcoran Clay”) is present in the eastern part of the study area at depths ranging from about 15 meters (m) in the southeast to 60 m in the northeast (Faunt, 2009). Aquifer systems below the Corcoran Clay consist of alluvial sediments and volcanic deposits of the upper Mehrten Formation, which extend down to the base of fresh water (about 200 m; Burow and others, 2004). Surficial Holocene flood-basin and dune deposits tend not to be saturated but can influence percolation rates (Burow and others, 2004).
Primary sources of groundwater recharge and discharge have shifted from precipitation and river seepage in predevelopment times to irrigation return flows and groundwater pumpage, respectively, because of intensive agricultural development in the region (Phillips and others, 2015). Development of the groundwater system has increased vertical groundwater flow into deeper parts of aquifers previously dominated by regional flow systems transmitting older groundwater laterally from the valley margins to discharge zones along its axial trough (Faunt, 2009). Long-screened production wells used for irrigation and municipal drinking-water supply tends to be deeper (about 85-m depths) than those used for domestic drinking-water supply (about 50 m), which are typically more susceptible to vertical migration of contaminants from the land surface (Burow and others, 2004, 2008).
Methods for Evaluating Groundwater Quality
The GAMA-PBP uses established benchmarks for public drinking water to contextualize groundwater quality from domestic wells, which is not regulated in California. This report focuses on water-quality constituents with regulatory, health-based maximum contaminant level (MCL) benchmarks. Constituents with aesthetic-based secondary maximum contaminant level (SMCL) benchmarks that affect groundwater color, taste, or odor are discussed but are not included in the overview of water-quality summary (fig. 1). Other constituents of interest with non-regulatory, health-based benchmarks (such as California state response levels or Federal health advisory levels) are discussed on a more limited basis and not included in the overview of water-quality summary (fig. 1). Water-quality benchmarks used in this report are compiled in Balkan and others (2022).
Concentrations of a given water-quality constituent exceeding its respective benchmark were considered “high.” Concentrations between the benchmark and one-half the benchmark (for inorganic constituents) or one-tenth the benchmark (for organic constituents) were considered “moderate.” Concentrations beneath the moderate threshold were considered “low.” The moderate classification indicates elevated concentrations below the benchmark and can help identify constituents of emerging concern in an aquifer system. A lower moderate threshold was set for organic constituents compared to inorganic constituents to be a more sensitive indicator of anthropogenic contamination. Organic constituents in groundwater primarily derive from human activities at the land surface, and a lower threshold effectively highlights parts of the aquifer area vulnerable to anthropogenic contamination as opposed to inorganic constituents that commonly occur at low concentrations in aquifer systems because of natural water-rock interactions (Levy and Fram, 2021). Non-detections for organic constituents were separated from detections at low concentrations to further highlight the presence of anthropogenic compounds in groundwater. Non-detections for inorganic constituents were grouped into the low classification because detection frequencies for inorganic constituents that are naturally present in aquifers at low concentrations can be more indicative of analytical method detection limits than environmental sources.
The gridded sampling design used in GAMA-PBP studies allows results to be reported as the proportion of aquifer area with water quality in a defined range (for example, exceeding an MCL or “high”). Wells sampled from individual grid cells represent a random sampling within a defined unit of the total aquifer area used for domestic drinking-water supply (herein, “domestic groundwater resources”). A spatial-weighting method was used to calculate the proportion of domestic groundwater resources with water quality within defined ranges (Belitz and others, 2010, 2015). Methods for spatially weighted calculations are described in detail in Levy and Fram (2021). Briefly, the proportion of wells in each sampled grid cell with low, moderate, or high concentrations for a given constituent was calculated, and an area-weighted average proportion was computed across all sampled grid cells. This prevents samples from multiple wells clustered within a given grid cell from over-representing aquifer conditions across a broader study area (Belitz and others, 2010, 2015).
Groundwater from 71 domestic or small-system wells selected for study was sampled and analyzed for a comprehensive suite of water-quality constituents and geochemical indicators as described by Balkan and others (2022). Groundwater samples were taken as close to the wellhead as possible and indicate the quality of ambient aquifer water before exposure to household plumbing or treatment systems. The median and interquartile range of sampled well depths were 61 m and 40–80 m, respectively, and the median well-screen length was 6 m (Balkan and others, 2022).
Overview of Water-Quality Results
This report focuses on water-quality constituents with regulatory benchmarks detected at moderate or high concentrations in the study area. Organic constituents with regulatory benchmarks that were frequently detected at low concentrations are also discussed. Results are presented separately for inorganic and organic constituents. Inorganic constituents include trace elements and nutrients, which are often present naturally at low concentrations in groundwater because of chemical interactions with aquifer materials. Organic constituents include volatile organic compounds (VOCs) and pesticides, which primarily originate from human activities at the land surface such as agriculture, urban land use, or industry. Inorganic and organic constituents were present at high concentrations exceeding an MCL benchmark in 37 percent and 9 percent of domestic groundwater resources, respectively (fig. 1). Inorganic and organic constituents were present at moderate concentrations below MCL benchmarks in 37 percent and 5 percent of domestic groundwater resources, respectively (fig. 1).
Inorganic Constituents
The most prevalent inorganic constituents of concern were nitrate, uranium, and arsenic, which were detected at concentrations exceeding respective MCLs (10 milligrams per liter [mg/L], 30 micrograms per liter [µg/L], and 10 µg/L) in 27 percent, 20 percent, and 10 percent of domestic groundwater resources (fig. 2). Nitrate is a nutrient that is typically present at high concentrations in study-area groundwater because of historic fertilizer applications at the land surface (Burow and others, 2008; Landon and others, 2011). Uranium and arsenic are trace elements that are naturally present in aquifer materials and can be mobilized into groundwater at high concentrations by geochemical processes affecting porewater alkalinity and reduction-oxidation (redox) conditions (Jurgens and others, 2010; Haugen and others, 2021).
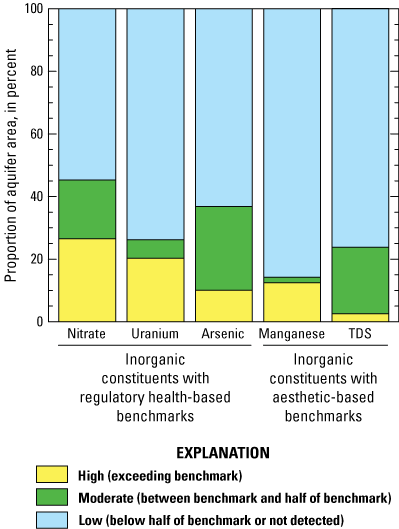
Proportion of domestic groundwater resources having selected inorganic constituents detected at low, moderate, or high concentrations. Abbreviation: TDS, total dissolved solids (Balkan and others, 2022).
Additional inorganic constituents with regulatory and non-regulatory benchmarks were detected at moderate or high concentrations with less prevalence. The nutrient nitrite and inorganic compound perchlorate were respectively detected at moderate and high concentrations in less than 2 percent of domestic groundwater resources. The trace elements selenium and barium were detected at moderate concentrations below their MCLs in 3 and 5 percent of domestic groundwater resources, respectively. The trace elements molybdenum, strontium, and vanadium were detected at moderate or high concentrations with respect to non-regulatory, health-based benchmarks in 2 to 5 percent of domestic groundwater resources.
Constituents with aesthetic-based benchmarks known as SMCLs are not included in the water-quality overview summary (fig. 1) and are considered separately to highlight factors affecting aesthetic properties of groundwater such as taste, color, or odor. Manganese is a trace metal that can affect the taste and color of groundwater but can also precipitate in pipes and cause clogging of household plumbing systems. Manganese was present above its SMCL of 50 µg/L in 13 percent of domestic groundwater resources (fig. 2). Manganese has also been associated with neurotoxicity in humans at higher doses; the U.S. Environmental Protection Agency’s non-regulatory Health Advisory Level for manganese (300 µg/L) was exceeded in 7 percent of domestic groundwater resources in the study area (California State Water Resources Control Board, 2021; Balkan and others, 2022).
Total dissolved solids (TDS) content is a measure of groundwater salinity and has recommended and upper SMCLs in the State of California of 500 mg/L and 1,000 mg/L, respectively, which were used as moderate and high thresholds for this study (Balkan and others, 2022). Total dissolved solids were measured at moderate and high concentrations in 21 percent and 3 percent of domestic groundwater resources, respectively (fig. 2). Chloride and sulfate are major ions with SMCL thresholds that can contribute to TDS but did not exceed benchmark thresholds and were only present at moderate concentrations in 3 percent and 1 percent of domestic groundwater resources, respectively. Bicarbonate was the largest ionic component of TDS in the study area, ranging from 24 percent to 94 percent of TDS by mass.
Organic Constituents
The only organic constituents exceeding regulatory MCL benchmarks were the fumigant byproduct 1,2,3-trichloropropane (1,2,3-TCP) and fumigant 1,2-dibromo-3-chloropropane (DBCP), which were detected at concentrations above respective MCLs (0.005 µg/L and 0.2 µg/L) in 9 percent and 2 percent of domestic groundwater resources (fig. 3). The 1,2,3-TCP and DBCP also were detected at low or moderate concentrations in 3 percent and 7 percent of domestic groundwater resources, respectively (fig. 3). The fumigant 1,2-dichloropropane (1,2-DCP) was only detected at low concentrations below one-tenth of its MCL of 5 µg/L in 10 percent of domestic groundwater resources. Fumigants are VOC pesticides that have historically been applied to perennial agricultural crops, such those found in vineyards or orchards, for nematode control in the San Joaquin Valley; 1,2,3-TCP was a manufacturing byproduct in fumigant mixtures (Burow and others, 2019). These three fumigant constituents were historically applied simultaneously or at alternate intervals and often co-occurred in groundwater samples. The State of California banned use of fumigants containing DBCP and 1,2-DCP in 1979 and 1984, respectively (Burow and others, 2019).
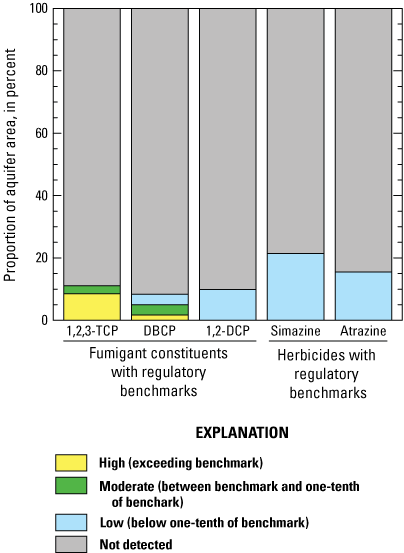
Proportion of domestic groundwater resources having selected organic constituents detected at low, moderate, or high concentrations. Abbreviations: 1,2,3-TCP, 1,2,3-trichloropropane; DBCP, 1,2-dibromo-3-chloropropane; 1,2-DCP, 1,2-dichloropropane (Balkan and others, 2022).
The herbicides atrazine and simazine were detected at low concentrations below one-tenth of their MCLs of 1 µg/L and 4 µg/L, respectively, in 16 percent and 21 percent (collectively 30 percent) of domestic groundwater resources (fig. 3). Herbicides are applied to a range of crop types in the Central Valley for weed control. Atrazine and simazine are recognized as reproductive toxins by the State of California (California Office of Environmental Health Hazard Assessment, 2016). Herbicides with non-regulatory benchmarks (hexazinone, metolachlor, and prometon) were collectively detected at low concentrations in 7 percent of domestic groundwater resources.
Additional VOCs with regulatory benchmarks detected with relative frequency in the study area included gasoline byproducts, solvents, and disinfection byproducts. The gasoline byproduct methyl tert-butyl ether (MTBE) was detected at low concentrations in 13 percent of domestic groundwater resources. Solvents with regulatory benchmarks (dichloromethane, carbon tetrachloride, trichloroethene, and 1,2-dichlorobenzene) were collectively detected at low and moderate concentrations in 5 percent and 1 percent of domestic groundwater resources, respectively. Disinfection byproducts with regulatory benchmarks (chloroform and bromodichloromethane) were collectively detected at low and moderate concentrations in 5 percent and 2 percent of domestic groundwater resources, respectively.
Microbial Indicators
Microbial indicator constituents can be used to evaluate the potential for bacterial contamination of groundwater from wastewater sources. In this study, groundwater samples were tested for the presence or absence of total coliform bacteria, Escherichia coli (E. coli), and Enterococci. Total coliform bacteria and Enterococci were detected in 20 percent and 2 percent of domestic groundwater resources, respectively. E. coli bacteria were not detected. Coliform bacteria are naturally present in soil and surface water but can also indicate fecal contamination, whereas E. coli and Enterococci are more specific indicators of fecal waste. Although total coliform and E. coli have MCLs in California under the Revised Total Coliform Rule (RTCR), they are not included in the overview of water-quality summary (fig. 1) because microbial MCL violations under the RTCR are based on repeat sampling, and synoptic survey results described in the current study are not sufficient to indicate MCL exceedances for these constituents (California State Water Resources Control Board, 2016).
Constituents of Special Interest—Per- and Polyfluoroalkyl Substances
Per- and polyfluoroalkyl substances (PFAS) are a family of human-made chemicals that are commonly used as surfactants in a variety of industrial processes and are persistent in the environment (Kent, 2021). Although regulatory benchmarks currently do not exist, California currently has health-based advisory “notification” and “response” levels in place for perfluorooctanoate (PFOA), perfluorooctanesulfonate (PFOS), and perfluorobutanesulfonate (PFBS). If a notification level is exceeded, the SWRCB recommends that water utilities inform consumers of health risks associated with a given water-quality constituent, and if the response level is exceeded, the SWRCB recommends the water source be taken out of service. Notification levels for PFOA, PFOS, and PFBS are 5.1, 6.5, and 500 nanograms per liter (ng/L), respectively; response levels are 10, 40, and 5,000 ng/L, respectively (California State Water Resources Control Board, 2022).
In this study, 9 out of 28 measured PFAS were detected. At least one PFAS was detected in 23 percent of domestic groundwater resources (fig. 4). Perfluorooctanoate was detected above the response level in 2 percent of domestic groundwater resources. PFOS was detected below the response level but above the notification level in 4 percent of domestic groundwater resources. The four PFAS shown in figure 4, (1) PFOA, (2) PFOS, (3) PFBS, and (4) perfluorohexanesulfonate (PFHxS), were each detected in greater than 5 percent of domestic groundwater resources. Five additional PFAS, (1) perfluoropentanoate (PFPeA), (2) perfluorobutanoate (PFBA), (3) perfluorohexanoate (PFHxA), (4) perfluoroheptanoate (PFHpA), and (5) perfluorononanoate (PFNA), were each detected in less than 5 percent of domestic groundwater resources.
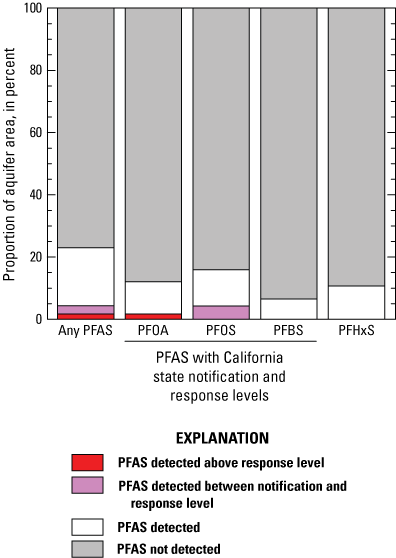
Proportion of domestic groundwater resources with detected per- and polyfluoroalkyl substances (PFAS). Other abbreviations: PFOA, perfluorooctanoate; PFOS, perfluorooctanesulfonate; PFBS, perfluorobutanesulfonate; PFHxS, perfluorohexanesulfonate (Balkan and others, 2022).
Factors that Affect Groundwater Quality
Groundwater quality can be influenced by anthropogenic factors, such as land use, that alter the chemistry of recharge waters as well as by natural interactions of groundwater with aquifer sediments. Geochemical conditions, often indicated by dissolved oxygen (DO) and pH, within an aquifer can determine its ability to attenuate contamination derived from human activities at the land surface. The following sections discuss decadal-scale effects of agricultural land use on groundwater chemistry and how geochemical conditions influence the mobility of contaminants within the aquifer system.
Effects of Agricultural Development
Elevated concentrations of nitrate, uranium, TDS, and pesticides (fumigants and herbicides) in study-area groundwater are related to legacy agricultural land use that has largely continued to present (Burow and others, 2008, 2019; Jurgens and others, 2010; Landon and others, 2011; Hansen and others, 2018). Burow and others (2008) indicated county-level fertilizer application rates to croplands in the region increased from 15 kilograms of nitrogen per hectare per year (kgN/ha/yr) in 1950 to 100 kgN/ha/yr in 2005. Elevated nitrate and pesticide levels in study-area groundwater have been attributed to penetration of legacy agricultural recharge into shallow parts of regional aquifers used for domestic supply during the latter part of the 20th century (Burow and others, 2008, 2019; Landon and others, 2011). Longer-term geochemical changes related to agricultural recharge affect groundwater TDS and uranium. Hansen and others (2018) found that increased carbon dioxide in recharge from crop respiration has accelerated plagioclase mineral weathering in soils leading to higher TDS in shallow groundwater over the past century. Increased carbon dioxide and mineral weathering in agriculturally affected groundwater has, in turn, increased bicarbonate concentrations in shallow groundwater leading to mobilization of uranium from aquifer sediments (Jurgens and others, 2010). Consequently, nearly two-thirds of the wells with nitrate exceeding the MCL also had uranium exceeding the MCL and moderately elevated TDS.
Patterns of agriculturally derived constituents detected in domestic-supply aquifers in this study were consistent with prior findings in the region. The highest concentrations of nitrate, uranium, and TDS co-occurred in agricultural areas to the west of Turlock and Merced proximal to the valley’s axial trough (figs. 5A–5C). In these areas, agricultural uses are predominantly grain, alfalfa, and dairy farms as opposed to higher elevation, comparatively well-drained soils to the east that support extensive almond orchards (Data Basin, 2022). Elevated (moderate to high) concentrations of nitrate, uranium, TDS and detections of pesticides (fumigant constituents or herbicides) with regulatory benchmarks were almost exclusively measured in wells of depths less than 75 m (fig. 6). Only one well with high 1,2,3-TCP and three wells with moderate nitrate had depths greater than 75 m.
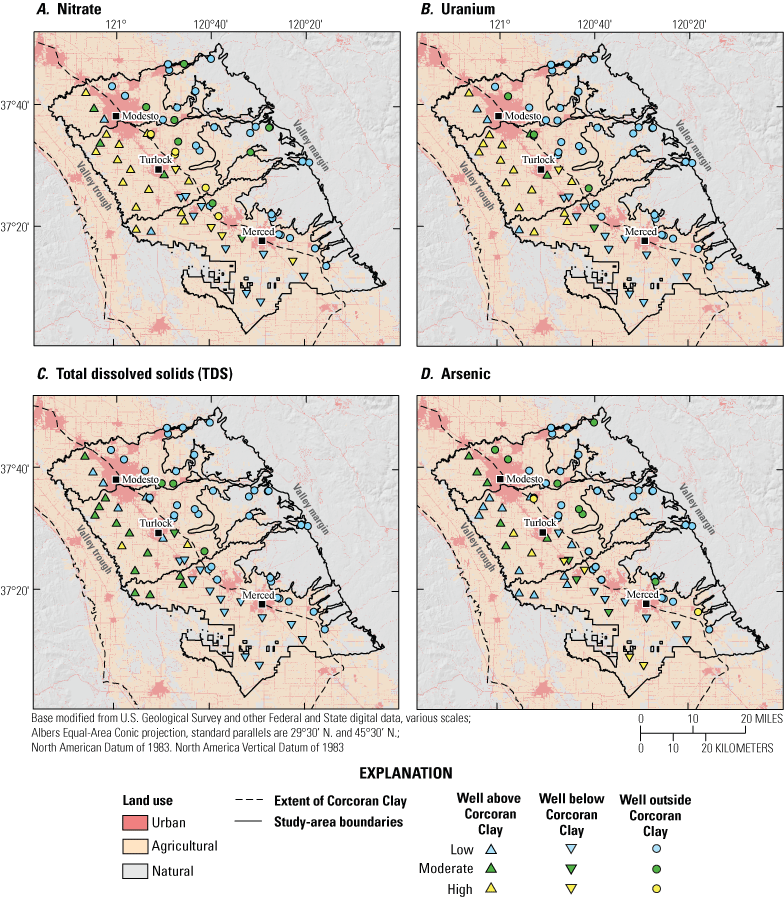
Study-area boundaries, land use, and position of wells with respect to the Corcoran Clay Member of the Tulare Formation (“Corcoran Clay”) having low, moderate, and high concentrations of A, nitrate; B, uranium; C, total dissolved solids (TDS); and D, arsenic (Faunt, 2009; Balkan and others, 2022; Multi-Resolution Land Characteristics Consortium, 2022).
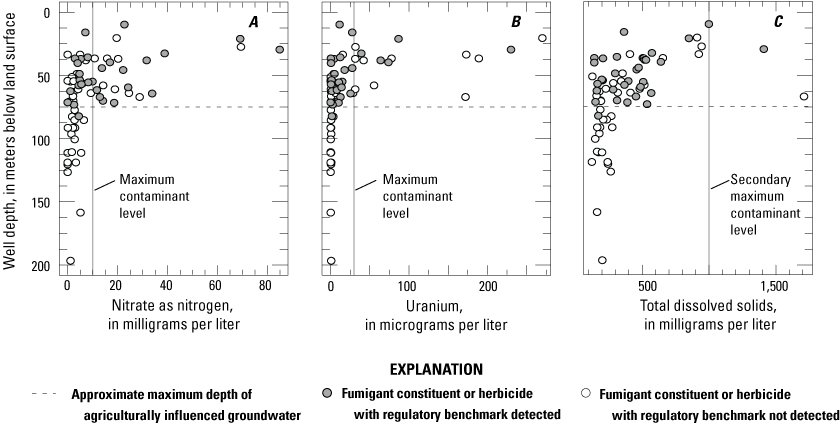
Relations of well depth to A, nitrate; B, uranium; and C, total dissolved solids, by detection of a fumigant constituent or herbicide with regulatory benchmark. Horizontal dashed line at 75 meters indicates the approximate maximum depth of agriculturally influenced groundwater (Balkan and others, 2022).
Well-screen position with respect to the Corcoran Clay was assessed using data from Faunt (2009). Wells were classified as being screened above, below, or outside the lateral extent of the Corcoran Clay. No well screens completely penetrated the estimated vertical extent of the Corcoran Clay. Wells were almost entirely screened above the Corcoran Clay in the western part of the Modesto and Turlock subbasins where the top of the member is deeper below the land surface (about 60 m) than it is to the southeast (fig. 5). Wells in this area also tend to be shallower because the water table is typically closer to the land surface with proximity to the valley’s axial trough (Landon and others, 2011). Wells were mostly screened below the Corcoran Clay in the western part of the Merced subbasin, where the member tends to be thinner and closer to the land surface (about 15 m). Moderate to high concentrations of nitrate were commonly measured in this area below the Corcoran Clay, indicating that the Corcoran Clay no longer fully acts as a confining layer because of ubiquitous penetration by boreholes, as has been noted in other studies (Williamson and others, 1989; Faunt, 2009; Landon and others, 2011). However, moderate to high concentrations of uranium and TDS were less prevalent in wells finished below the Corcoran Clay, indicating the interaction of agricultural recharge with aquifer minerals that produces elevated uranium and TDS may lag in deeper parts of the aquifer system compared to constituents like nitrate that are more mobile and have limited interactions with aquifer sediments (figs. 5A–5C).
Fumigant constituents and herbicides with regulatory benchmarks also were detected beneath the Corcoran Clay and tended to occur in a northwest to southeast trending band along the eastern extent of the member (fig. 7). This band typically demarcates the transition from deeper water tables and predominantly oxic groundwater conditions in the east to shallower water tables and reducing groundwater conditions to the west (Landon and others, 2011). Soils along and to the east of this band tend to be more well drained than soils to the west and are typically occupied by perennial orchard crops, such as almonds (Data Basin, 2022). Burow and others (2019) reported detections of pesticides were more common in oxic groundwater derived from agricultural return flows through coarse-grained surficial deposits associated with vineyards and orchards in the San Joaquin Valley.
Moderate to high concentrations of fumigants 1,2,3-TCP and DBCP were clustered in groundwater underlying surficial (top 15 m), coarse-grained Holocene dune deposits in the urban-agricultural corridor between Turlock and Merced (fig. 7A). Low concentrations of herbicides atrazine and simazine were also measured in this area and in shallow groundwater below agricultural lands to the northwest where surficial deposits are more fine-grained, indicating less attenuation of herbicides compared to fumigants in poorly drained soils or different spatial distributions of the use of fumigants and herbicides related to historic crop applications. Burow and others (1998) reported detections of herbicides atrazine and simazine in groundwater were broadly associated with corn, alfalfa, vegetable, vineyard, and orchard crop types in the San Joaquin Valley, whereas fumigants were more specifically associated with orchards or vineyards that are less common in poorly drained soils proximal to the valley trough.
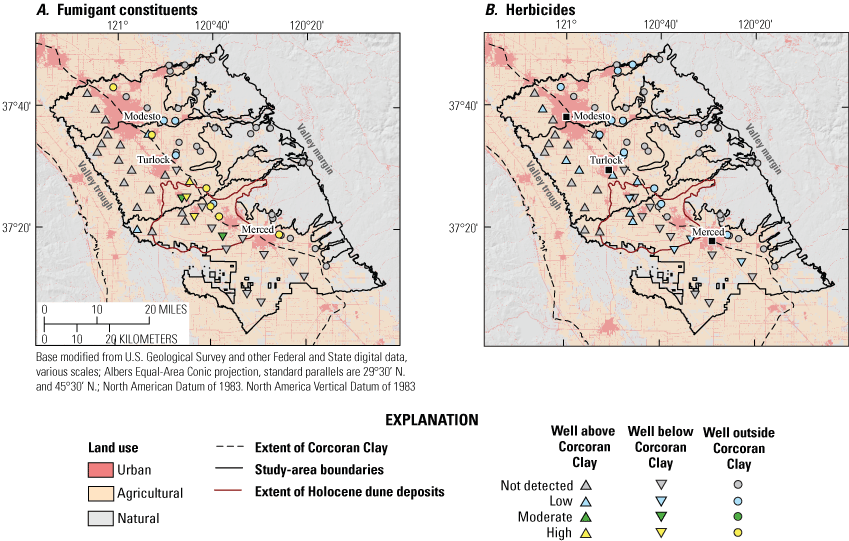
Study-area boundaries, land use, and position of wells with respect to the Corcoran Clay Member of the Tulare Formation (“Corcoran Clay”) and Holocene dune deposits having non-detections or low, moderate, and high concentrations of A, fumigant constituents with regulatory benchmarks; and B, herbicides with regulatory benchmarks (Saucedo and others, 2000; Faunt, 2009; Balkan and others, 2022; Multi-Resolution Land Characteristics Consortium, 2022).
Hydrogeochemical Evolution of the Aquifer System
Geochemical conditions in groundwater can affect contaminant mobility from aquifer materials. The concentration of dissolved oxygen (DO) in groundwater is an important indicator of redox conditions, which range from oxic (DO greater than 0.5 mg/L) to reducing (dissolved oxygen less than or equal to 0.5 mg/L). Dissolved oxygen is typically in equilibrium with the atmosphere in recharge but can be depleted along groundwater flowpaths by reactions with organic carbon or inorganic reductants (McMahon and Chapelle, 2008). Landon and others (2011) used relative lateral position from the valley margins to trough as a proxy for flowpath length and reported that reducing groundwater in the study area was mostly in the lower fifth of the valley with proximity to its axial trough (lateral positions less than 0.2; fig. 8) in old groundwater or young groundwater with high dissolved organic carbon near the water table.
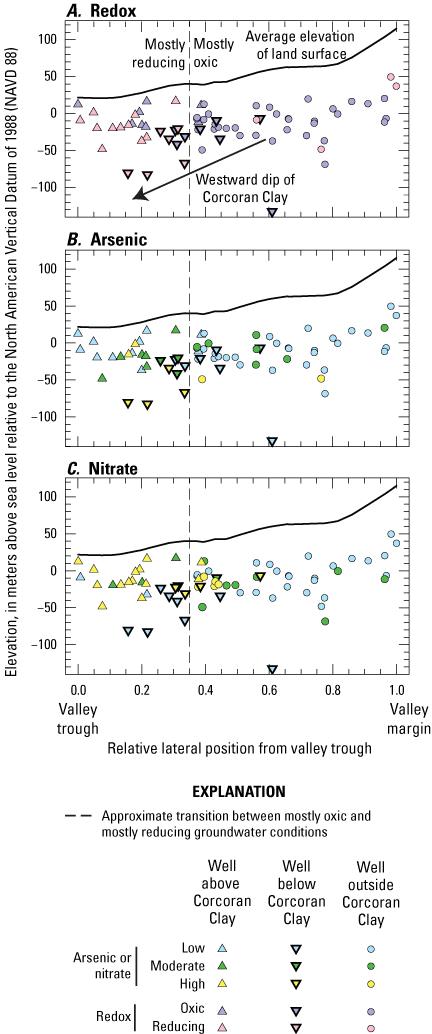
Transects showing relations of relative lateral position to the valley trough and elevation of well depths for A, groundwater redox conditions or wells having low, moderate, and high concentrations of B, arsenic, and C, nitrate, by well position with respect to the Corcoran Clay Member of the Tulare Formation (“Corcoran Clay”; Faunt, 2009; Balkan and others, 2022).
In this study, reducing conditions were more prevalent with proximity to the valley’s axial trough, similar to results presented in Landon and others (2011). However, in this study the transition to reducing conditions occurred at slightly greater lateral positions (less than 0.35) encompassing the lower third of the valley (fig. 8). This observed difference in lateral positions associated with reducing groundwater could result from Landon and others (2011) not incorporating parts of the Merced subbasin where the Corcoran Clay is shallower and extends further to the east. Although oxic water was detected below the shallowest part of the Corcoran Clay along its eastern extent, wells finished below the member transition to reducing conditions where it dips to the west between lateral positions of 0.2 and 0.35 (fig. 8). Additionally, Landon and others (2011) focused on public-supply wells, which tend to have longer screens that support mixing of deeper reducing groundwater with shallower oxic water within the wellbore.
Redox conditions in groundwater can exert strong controls on arsenic mobility and nitrate attenuation. Arsenic tends to be mobilized from aquifer sediments by microbially mediated dissolution of iron oxides and hydroxides that occur in reducing groundwater (typically in the absence of nitrate), or to a lesser degree desorption from surface-binding sites at basic pH values (Smedley and Kinniburgh, 2002). Nitrate can be attenuated by microbial denitrification in the subsurface if dissolved oxygen in contemporaneous recharge is consumed by heterotrophic respiration or if it moves into already anoxic parts of the aquifer system. However, denitrification can be limited by the availability of electron donors in groundwater (for example, organic carbon) and may not be sufficient to process large nitrate loads transmitted to aquifers within the study area by agricultural return flows (Landon and others, 2011).
As expected, results of this study indicated that moderate to high arsenic concentrations were most prevalent in reducing groundwater located proximal to the valley’s axial trough (lateral positions less than 0.35) and were highest in deep wells finished beneath the Corcoran Clay in this area (figs. 5D, 8A–8B, 9). The highest concentrations of arsenic and nitrate were measured in reducing and oxic groundwater, respectively (fig. 9). However, elevated (moderate to high) arsenic concentrations were also measured in 12 wells with oxic groundwater. Further, elevated nitrate concentrations were measured in nine wells with reducing groundwater. Oxic groundwater with elevated arsenic is likely a function of other geochemical controls on arsenic sorption to aquifer sediments; 9 of the 12 wells had basic pH greater than or equal to 7.5, a value above which arsenic tends to be less strongly bound to aquifer minerals. Groundwater phosphate derived from fertilizer also may displace arsenic from binding sites on aquifer minerals and cause elevated arsenic concentrations in oxic groundwater (Smedley and Kinniburgh, 2002). All but one well sampled in this study had groundwater phosphate concentrations exceeding 10 µg/L (Balkan and others, 2022), but there was no apparent relation of dissolved phosphate to arsenic indicating a variety of complex drivers affect arsenic availability in oxic groundwater. The presence of elevated nitrate in reducing water is consistent with the findings of Landon and others (2011) that denitrification may not be sufficient to attenuate large nitrate loads in study area groundwater.
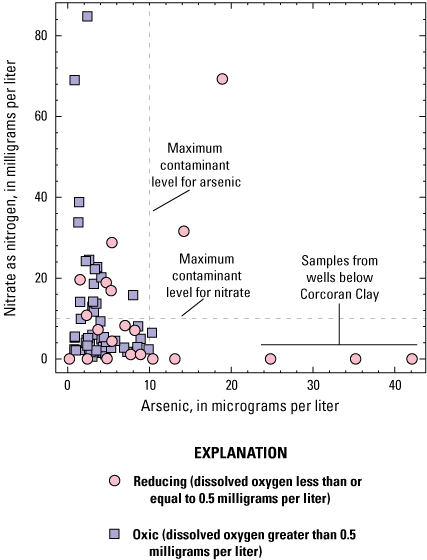
Relations of groundwater arsenic and nitrate by redox condition. The three samples with the highest arsenic concentrations were from wells screened beneath the Corcoran Clay Member of the Tulare Formation (“Corcoran Clay”; Balkan and others, 2022).
Although the highest concentrations of nitrate and arsenic occurred independently of each other, elevated concentrations of nitrate and arsenic co-occurred at 9 wells. Wells with co-occurring elevated concentrations of nitrate and arsenic were in the northwest part of the study area above or outside the extent of the Corcoran Clay at mostly shallow depths (less than 75 m; figs. 5A, 5D, 8B, 8C). Two-thirds of these wells had reducing water, with the remainder being oxic. These results indicate nitrate-laden recharge has mixed into reducing parts of aquifer systems proximal to the valley’s axial trough. Incursions of agricultural recharge driven by irrigation and groundwater pumpage may oxidize reducing parts of the aquifer system through time, in which case arsenic mobility will be primarily controlled by pH and the presence of agriculturally derived ligands such as phosphate (Haugen and others, 2021).
For More Information
Technical reports and hydrologic data collected for the GAMA Program may be obtained from
GAMA Project Chief
U.S. Geological Survey
California Water Science Center
6000 J Street, Placer Hall
Sacramento, CA 95819
Telephone number: (916) 278-3000
Acknowledgments
We thank the site owners and water purveyors for granting site access and allowing the U.S. Geological Survey to collect samples from their wells. Most of the funding for this work was provided by the California State Water Resources Control Board Groundwater Ambient Monitoring and Assessment Program. Additional funding was provided with U.S. Geological Survey Cooperative Matching Funds. This report is a product of the California State Water Resources Control Board Groundwater Ambient Monitoring and Assessment Program Priority Basin Project.
References Cited
Balkan, M., Levy, Z.F., Dupuy, D.I., Shelton, J.L., Watson, E., and Johnson, T.D., 2022, Groundwater-quality data in the Modesto-Turlock-Merced Domestic-Supply Aquifer Study Unit, 2020–2021—Results from the California GAMA Priority Basin Project: U.S. Geological Survey data release, https://doi.org/10.5066/P96R55KQ.
Belitz, K., Fram, M.S., and Johnson, T.D., 2015, Metrics for assessing the quality of groundwater used for public supply, CA, USA—Equivalent-population and area: Environmental Science & Technology, v. 49, no. 14, p. 8330–8338, available at https://doi.org/10.1021/acs.est.5b00265.
Belitz, K., Jurgens, B., Landon, M.K., Fram, M.S., and Johnson, T., 2010, Estimation of aquifer scale proportion using equal area grids—Assessment of regional scale groundwater quality: Water Resources Research, v. 46, no. 11, 14 p., available at https://doi.org/10.1029/2010WR009321.
Burow, K.R., Floyd, W.D., and Landon, M.K., 2019, Factors affecting 1,2,3-trichloropropane contamination in groundwater in California: Science of the Total Environment, v. 672, p. 324–334, available at https://doi.org/10.1016/j.scitotenv.2019.03.420.
Burow, K.R., Shelton, J.L., and Dubrovsky, N.M., 1998, Occurrence of nitrate and pesticides in ground water beneath three agricultural land-use settings in the Eastern San Joaquin Valley, California, 1993–1995: U.S. Geological Survey Water-Resources Investigations Report 97–42, 51 p., accessed June 23, 2022, at https://doi.org/10.3133/wri974284.
Burow, K.R., Shelton, J.L., and Dubrovsky, N.M., 2008, Regional nitrate and pesticide trends in ground water in the eastern San Joaquin Valley, California: Journal of Environmental Quality, v. 37, no. S5, p. S249–S263, available at https://doi.org/10.2134/jeq2007.0061.
Burow, K.R., Shelton, J.L., Hevesi, J.A., and Weissmann, G.S., 2004, Hydrogeologic characterization of the Modesto area, San Joaquin Valley California: U.S. Geological Scientific Investigations Report 2004–5232, 62 p., accessed June 23, 2022, at https://doi.org/10.3133/sir20045232.
California Office of Environmental Health Hazard Assessment, 2016, Atrazine, propazine, simazine and their chlorometabolites DACT, DEA and DIA listed effective July 15, 2016, as reproductive toxicants: California Office of Environmental Health Hazard Assessment website, accessed June 23, 2022, at https://oehha.ca.gov/proposition-65/crnr/atrazine-propazine-simazine-and-their-chlorometabolites-dact-dea-and-dia-0.
California Department of Water Resources, 2020, California’s groundwater bulletin 118, update 2020, 485 p., accessed June 23, 2022, at https://water.ca.gov/Programs/Groundwater-Management/Bulletin-118.
California State Water Resources Control Board, 2015, A guide for private domestic well owners: Groundwater Ambient Monitoring and Assessment Program, 22 p., accessed June 23, 2022, at https://www.waterboards.ca.gov/water_issues/programs/gama/docs/wellowner_guide.pdf.
California State Water Resources Control Board, 2016, Groundwater information sheet, bacterial indicators: Groundwater Ambient Monitoring and Assessment Program, 7 p., accessed June 23, 2022, at https://www.waterboards.ca.gov/gama/docs/coc_bacteria_indicators.pdf.
California State Water Resources Control Board, 2021, Drinking water notification level for Manganese: California State Water Resources Control Board website, accessed June 23, 2022, at https://www.waterboards.ca.gov/drinking_water/certlic/drinkingwater/Manganese.html.
California State Water Resources Control Board, 2022, PFAS—Per- and polyfluoroalkyl substances: California State Water Resources Control Board website, accessed June 23, 2022, at https://www.waterboards.ca.gov/pfas/.
Chappelle, C., Hanak, E., and Harter, T., 2017, Groundwater in California: Public Policy Institute of California, accessed June 23, 2022, at https://www.ppic.org/wp-content/uploads/JTF_GroundwaterJTF.pdf.
Data Basin, 2022, California augmented multisource landcover 2010: Data Basin website, accessed June 23, 2022, at https://databasin.org/datasets/f12a901528c0498ba63ca291b8e6627b/.
Faunt, C.C., ed., 2009, Groundwater availability of the Central Valley Aquifer, California: U.S. Geological Survey Professional Paper 1766, 225 p., accessed June 23, 2022, at https://doi.org/10.3133/pp1766.
Hansen, J.A., Jurgens, B.C., and Fram, M.S., 2018, Quantifying anthropogenic contributions to century-scale groundwater salinity changes, San Joaquin Valley, California, USA: Science of the Total Environment, v. 642, p. 125–136, accessed June 23, 2022, at https://doi.org/10.1016/j.scitotenv.2018.05.333.
Haugen, E.A., Jurgens, B.C., Arroyo-Lopez, J.A., and Bennett, G.L., V, 2021, Groundwater development leads to decreasing arsenic concentrations in the San Joaquin Valley, California: Science of the Total Environment, v. 771, 14 p., available at https://doi.org/10.1016/j.scitotenv.2021.145223.
Johnson, T.D., and Belitz, K., 2019, Domestic well locations and populations served in the contiguous U.S.—Datasets for decadal years 2000 and 2010: U.S. Geological Survey data release, accessed June 23, 2022, at https://doi.org/10.5066/P9FSLU3B.
Jurgens, B.C., Fram, M.S., Belitz, K., Burow, K.R., and Landon, M.K., 2010, Effects of groundwater development on uranium—Central Valley, California, USA: Groundwater, v. 48, no. 6, p. 913–928, accessed June 23, 2022, at https://doi.org/10.1111/j.1745-6584.2009.00635.x.
Kent, R.H., 2021, Sampling for per- and polyfluoroalkyl substances (PFAS) by the Groundwater Ambient Monitoring and Assessment Priority Basin Project: U.S. Geological Survey Fact Sheet 2021–3028, 4 p., accessed June 23, 2022, at https://doi.org/10.3133/fs20213028.
Landon, M.K., Green, C.T., Belitz, K., Singleton, M.J., and Esser, B.K., 2011, Relations of hydrogeologic factors, groundwater reduction-oxidation conditions, and temporal and spatial distributions of nitrate, Central-Eastside San Joaquin Valley, California, USA: Hydrogeology Journal, v. 19, p. 1203–1224, accessed June 23, 2022, at https://doi.org/10.1007/s10040-011-0750-1.
Levy, Z.F., and Fram, M.S., 2021, Status and understanding of groundwater quality in the northern Sierra Nevada foothills domestic-supply aquifer study units, 2015–17—California GAMA Priority Basin Project: U.S. Geological Survey Scientific Investigations Report 2021–5019, 120 p., accessed June 23, 2022, at https://doi.org/10.3133/sir20215019.
McMahon, P.B., and Chapelle, F.H., 2008, Redox processes and water quality of selected principal aquifer systems: Groundwater, v. 46, no. 2, p. 259–271, accessed June 23, 2022, at https://doi.org/10.1111/j.1745-6584.2007.00385.x.
Multi-Resolution Land Characteristics Consortium, 2022, NLCD 2011 Land cover (CONUS): Multi-Resolution Land Characteristics Consortium website, accessed April 20, 2022, at https://www.mrlc.gov/data/nlcd-2011-land-cover-conus.
Phillips, S.P., Rewis, D.L., and Traum, J.A., 2015, Hydrologic model of the Modesto Region, California, 1960–2004: U.S. Geological Survey Scientific Investigations Report 2015–5045, 69 p., accessed June 23, 2022, at https://doi.org/10.3133/sir20155045.
PRISM Climate Group, 2015, United States average annual precipitation, maximum and minimum temperature, 1981–2010: Oregon State University, PRISM Climate Group website, accessed November 1, 2019, at https://prism.oregonstate.edu/.
Smedley, P.L., and Kinniburgh, D.G., 2002, A review of the source, behavior and distribution of arsenic in natural waters: Applied Geochemistry, v. 17, p. 517–568, accessed June 23, 2022, at https://doi.org/10.1016/S0883-2927(02)00018-5.
U.S. Geological Survey, 2018, California Groundwater Ambient Monitoring and Assessment (GAMA) Program Priority Basin Project—Shallow aquifer assessment (ver. 1.1, September 2018): U.S. Geological Survey Fact Sheet 2012–3136, 2 p., accessed June 23, 2022, at https://pubs.usgs.gov/fs/2012/3136/.
Voss, S.A., Jurgens, B.C., Fram, M.S., and Bennett, G.L., V, 2019, Delineation of spatial extent, depth, thickness, and potential volume of aquifers used for domestic and public water-supply in the Central Valley, California: U.S. Geological Survey Scientific Investigations Report 2019–5076, 34 p., accessed June 23, 2022, at https://doi.org/10.3133/sir20195076.
Williamson, A.K., Prudic, D.E., and Swain, L.A., 1989, Ground-water flow in the Central Valley, California: U.S. Geological Survey Professional Paper 1401–D, 127 p., accessed June 23, 2022, at https://doi.org/10.3133/pp1401D.
Disclaimers
Any use of trade, firm, or product names is for descriptive purposes only and does not imply endorsement by the U.S. Government.
Although this information product, for the most part, is in the public domain, it also may contain copyrighted materials as noted in the text. Permission to reproduce copyrighted items must be secured from the copyright owner.
Suggested Citation
Levy, Z.F., Balkan, M., and Shelton, J.L., 2023, Quality of groundwater used for domestic supply in the Modesto, Turlock, and Merced Subbasins of the San Joaquin Valley, California: U.S. Geological Survey Open-File Report 2022-1116, 13 p., https://doi.org/10.3133/ofr20221116.
ISSN: 2331-1258 (online)
Study Area
Publication type | Report |
---|---|
Publication Subtype | USGS Numbered Series |
Title | Quality of groundwater used for domestic supply in the Modesto, Turlock, and Merced Subbasins of the San Joaquin Valley, California |
Series title | Open-File Report |
Series number | 2022-1116 |
DOI | 10.3133/ofr20221116 |
Year Published | 2023 |
Language | English |
Publisher | U.S. Geological Survey |
Publisher location | Reston, VA |
Contributing office(s) | California Water Science Center |
Description | Report: 13 p.; Data Release |
Country | United States |
State | California |
Other Geospatial | San Joaquin Valley |
Online Only (Y/N) | Y |
Google Analytic Metrics | Metrics page |