Haliaeetus leucocephalus (Bald Eagle) and Aquila chrysaetos (Golden Eagle) Mortality and Exposure to Lead, Mercury, and Anticoagulant Rodenticides in Eight Western and Midwestern States, 2014–17
Links
- Document: Report (2.10 MB pdf) , HTML , XML
- Data Release: USGS data release —USGS National Wildlife Health Center necropsy and contaminant results for bald and golden eagles collected in 8 States from January 1, 2014, through December 31, 2017, to determine cause of illness/death and lead, mercury, and anticoagulant rodenticide exposure
- Download citation as: RIS | Dublin Core
Acknowledgments
Funding for this project was provided by U.S. Geological Survey Science Support Program/Quick Response Program, U.S. Fish and Wildlife Service Golden Eagle Working Group and the U.S. Geological Survey National Wildlife Health Center. The following U.S. Fish and Wildlife Service and State agency staff were instrumental in the collection and submission of specimens for diagnostic evaluation: Kim Dickerson, Jessica Johnson, Lourdes Mena, Amanda Ciurej, David Rouse, Stephanie Graham, Gibran Suleiman, John Miesner, Kevin Downs, and Karen Fox (Colorado Parks and Wildlife).
We are grateful to the entire diagnostic and epidemiology teams at the National Wildlife Health Center for expertise and Todd Katzner and Patricia Ortiz of the U.S. Geological Survey Forest and Rangeland Ecosystem Science Center who processed liver samples for lead analysis and provided expert consultation.
Abstract
The U.S. Geological Survey National Wildlife Health Center measured environmental contaminants in Haliaeetus leucocephalus (bald eagles) and Aquila chrysaetos (golden eagles) to evaluate dietary exposure to lead, mercury, and anticoagulant rodenticides (AR), all of which were identified by U.S. Fish and Wildlife Service as a priority issue of concern for Mountain Prairie Region 6. Data were needed to inform U.S. Fish and Wildlife Service regional programs aimed at reducing exposure to these substances. Carcasses of bald eagles (n=172) and golden eagles (n=142) collected from North and South Dakota, Montana, Wyoming, Colorado, Utah, Nebraska, and Kansas between 2014 and 2017 were assessed for cause of death and liver lead, mercury, and AR level concentrations. Trauma, electrocution, and lead poisoning were the three leading causes of death, affecting 51 percent, 21 percent, and 20 percent of eagles, respectively. Trauma was the leading cause of death for both species, whereas lead poisoning was the second leading cause of death for bald eagles (31 percent) and was only diagnosed as the cause of death in 7 percent of golden eagles. Elevated lead concentrations within the range of subclinical or clinical poisoning (greater than [>] 2 milligrams per kilogram [mg/kg] wet weight) were present in 25 percent of eagles tested, including 36 percent of bald eagles and 11 percent of golden eagles. No association was detected among lead exposure and trauma, electrocution, or infectious disease. Mercury concentrations were considered high (>80 mg/kg dry weight) for only 2 percent of bald eagles and no golden eagles. Brodifacoum was the most common AR detected, present in 56 percent of eagles, including 70 percent of bald eagles and 39 percent of golden eagles; however, death was not directly attributed to AR toxicosis in any case. Results of this study provide baseline data on common causes of mortality and threats to eagles in Region 6 from lead, mercury, and rodenticide exposure and can be used to inform management decisions.
Introduction
As top predators, eagles are susceptible to ingesting contaminated prey and are excellent biosentinels for environmental toxins (Leith and others, 2010). Numerous studies have documented lead poisoning in Haliaeetus leucocephalus (bald eagles) and Aquila chrysaetos (golden eagles; Wayland and Bollinger, 1999; Stauber and others, 2010; Bedrosian and others, 2012; Warner and others, 2014; Slabe and others, 2022). Mercury and anticoagulant rodenticide (AR) poisoning of eagles has also been reported (Weech and others, 2003; Mierzykowski and others, 2013; U.S. Environmental Protection Agency [EPA], 2016).
Bald and golden eagles are protected from poisoning in accordance with laws administered by the U.S. Fish and Wildlife Service (USFWS) including the Bald and Golden Eagle Protection Act (16 U.S.C. §668 et seq.). Bald eagles were delisted under the Endangered Species Act (16 U.S.C. §1531 et seq.) in 2007; however, the post delisting plan (USFWS, 2009a) requires periodic monitoring of populations and potential limiting factors for 20 years. Since delisting, contaminant studies have been done on bald eagles collected from New England (Mierzykowski and others, 2013; for lead and mercury) and the Great Lakes region (Warner and others, 2014; for lead); however, similar contaminant assessments are lacking for bald and golden eagles in the States of North and South Dakota, Montana, Wyoming, Colorado, Utah, Nebraska, and Kansas (fig. 1). In addition to lead and mercury, ARs used in these States may put eagles at a high risk for unintentional poisonings.
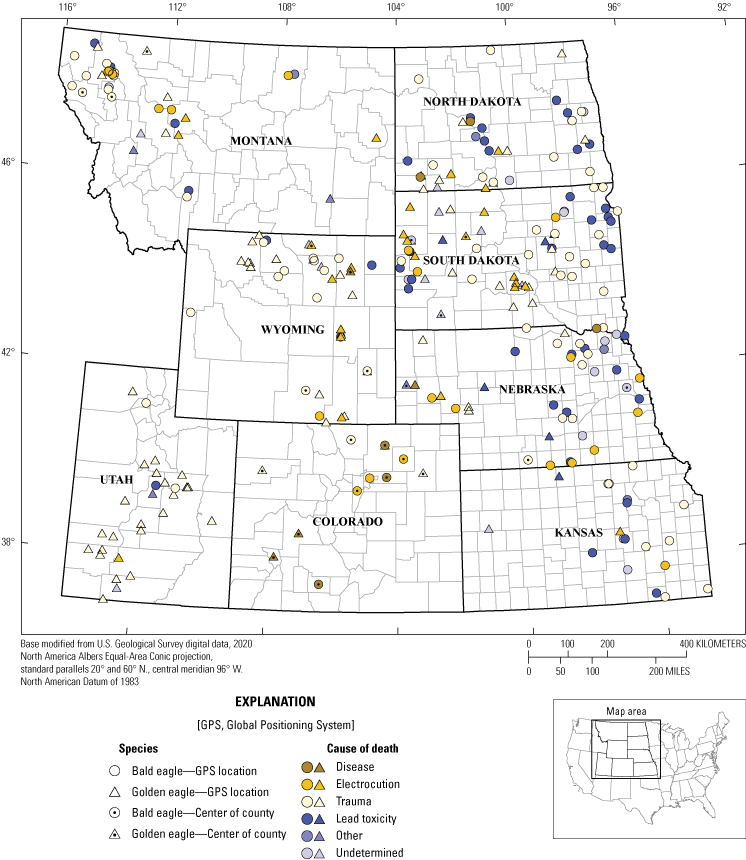
Cause of death determinations for 161 Haliaeetus leucocephalus (bald eagles) and 127 Aquila chrysaetos (golden eagles) with actual, estimated, or county-level locations in eight States from 2014 to 2017. Note: the data point for eagles that only had county-level location information recorded (n=41) was placed in the centroid of the county. Specimens with no recorded county-level information (n=26) were not included.
Although monitoring indicates that bald eagle populations are continuing to increase (USFWS, 2016a), it is important to evaluate wildlife poisoning events that may cause harm and be preventable; for example, lead poisoning of bald eagles from ingestion of lead shot can cause wing drooping, lethargy, emaciation, and blindness (Pattee and others, 1981). Although lead poisoning of eagles is widespread (Slabe and others, 2022), replacement of lead shot with nontoxic shot has been reported as effective at decreasing lead concentrations in avian scavengers (Avery and Watson, 2009;Parish and others, 2009;Kelly and others, 2011). Golden eagles have not been federally listed under the Endangered Species Act; however, populations in the Western United States might be gradually declining in some conservation regions (Millsap and others, 2013; USFWS, 2016a); thus, understanding regional contaminant risks may be crucial in recovering and maintaining migratory golden eagle populations. In this study, we investigated bald and golden eagle mortalities in eight States of the midwestern and western United States to document causes of death and identify toxins that may pose threats to their populations. All U.S. Geological Survey data collected for this study are available in a data release (Bodenstein and Lankton, 2023).
Eagles and Lead
Ingestion of lead-contaminated waterfowl carcasses by bald eagles was a primary consideration for the 1991 ban on lead shot for waterfowl hunting in the United States (USFWS, 1986). A 16-year retrospective study from 1980 to 1995 determined that average concentrations of lead in bald and golden eagle blood samples had decreased after 1991; however, the prevalence of lead poisoning in eagles did not change (Kramer and Redig, 1997). Thus, despite the 1991 ban on lead shot for hunting waterfowl, lead exposure and poisoning in eagles has been an ongoing concern (Cruz-Martinez and others, 2012; Russell and Franson, 2014).
Before and after the 1991 ban on lead shot for waterfowl hunting, several studies documented lead toxicity in bald and golden eagles. The U.S. Geological Survey National Wildlife Health Center (NWHC) diagnosed lead poisoning in 16 percent of 484 bald eagles and 5 percent of 68 golden eagles examined 1975–2013 (Russell and Franson, 2014). Examination of bald eagle carcasses from three upper midwestern States (Iowa, Minnesota, and Wisconsin) during a single winter (2011–12) revealed lethal lead concentrations (in other words, greater than [>] 6 milligrams per kilogram [mg/kg] wet weight [ww]) in 40 percent of 58 carcasses and detectable lead concentrations in another 20 percent (Warner and others, 2014). In another report, 14 percent of 127 bald eagles from New England had concentrations of lead indicative of lead poisoning (in other words, >30 mg/kg dry weight [dw]; Mierzykowski and others, 2013). A more recent analysis reports high concentrations of chronic (as much as 47 percent) and acute (as much as 35 percent) lead poisoning in both eagle species across the United States (Slabe and others, 2022), with the potential for long-term population suppression (Hanley and others, 2022; Slabe and others, 2022). Lead bullets used for big game hunting may be a primary exposure pathway for dietary lead in eagles (Stauber and others, 2010; Bedrosian and others, 2012) and the use of nonlead ammunition can reduce lead exposure in these species (Bedrosian and others, 2012); however, recreational and pest management shooting of Cynomys spp. (prairie dogs) and Sciuridae spp. (ground squirrels) with lead ammunition is common across the western United States and exposure to lead through scavenging of these carcasses also poses a potential exposure pathway (Pauli and Buskirk, 2007; Herring and others, 2016). McTee and others (2017) determined that 66 percent of Urocitellus columbianus (Columbian ground squirrels), a common prey species for golden eagles, contained lead fragments after being shot with lead bullets. The shot carcasses of Spermophilus richardsonii (Richardson’s ground squirrels), another common prey species for golden eagles, were also determined to be a source of lead exposure, meaning that raptors scavenging these carcasses may be at high risk of lead poisoning (Knopper and others, 2006).
Eagles and Mercury
Mercury is a naturally occurring element that can be highly toxic and mobile in the environment. Inorganic mercury is emitted more by anthropogenic than natural sources (Nriagu, 1989 as cited by Shore and others, 2011), largely because of coal-fired electricity generation (Streets and others, 2009; Dziok and others, 2021). Human activities have increased atmospheric concentrations of mercury by 3–5 fold during the last 150 years (Eagles-Smith and others, 2014). When inorganic mercury enters an aquatic ecosystem, it can undergo a process known as methylation resulting in the formation of methylmercury (MeHg). Methylation of mercury within the water column and sediments of surface waters is the most important biogeochemical process from an ecotoxicological perspective (Wiener and others, 2003, Scheuhammer and others, 2008). The methylated form of mercury is typically the most stable, bioavailable, and toxic form of mercury to wildlife (Shore and others, 2011). Mercury accumulated in fish and at higher trophic levels of aquatic food webs is almost entirely MeHg and is readily bioaccumulated and transferred in food webs and can biomagnify to high concentrations in predatory fish and wildlife (Wiener and others, 2003); thus, eagles may be exposed to mostly MeHg when they consume prey. The liver is where mercury is demethlylated, and the liver of fish and mammalian prey can accumulate methylmercury to harmful concentrations. Usually, MeHg is more than 50 percent of the total mercury measured in bald eagle livers (Weech and others, 2003;Mierzykowski and others, 2013); however, observations of elevated concentrations of total mercury in the liver are not sufficient for diagnosing methylmercury intoxication because of demethylation and the subsequent formation of MeHg complexes with selenium that have relatively low toxicity (Wiener and others, 2003).
Concentrations of mercury above toxicity thresholds have been reported in bald eagle blood (Carlson and others, 2012), feather (Bechard and others, 2009), and liver samples (Weech and others, 2003; Mierzykowski and others, 2013). Although tissue-based effect thresholds have not been derived for eagles, a synopsis of data available for other avian species indicates that liver concentrations >2 (experimental) and >20 (field) mg/kg ww may be indicative of adverse reproductive effects and death (Shore and others, 2011). A study that tested blood samples from 64 nestling bald eagles in Montana and Wyoming reported that mercury contamination had become more widespread from 1991 to 2007, whereas the prevalence of lead contamination during that period was unchanged (Harmata and Oakleaf, 1992; Carlson and others, 2012).
Eagles and Rodenticides
Several types of ARs have been used in the United States to control rodents including prairie dogs and ground squirrels in western States. Poisoned animals can take days to weeks to become moribund and are more susceptible to predation (Cox and Smith, 1992; C.A. Yoder, U.S. Department of Agriculture, unpub. data, 2008; these data are not currently available from the U.S. Department of Agriculture. Please contact the U.S. Department of Agriculture for further information). Large field applications of ARs have been known to attract raptors to the easy-to-capture prey (Vyas and others, 2017).
In 2012, two first generation anticoagulant rodenticides (FGARs), trade names Rozol TM (active ingredient chlorphacinone) and Kaput TM (active ingredient diphacinone), were registered to poison Cynomys ludovicianus (black-tailed prairie dogs), resulting in large scale field applications that may individually cover thousands of acres of grassland/prairie dog habitat (U.S. Environmental Protection Agency [EPA], 2016). Studies have recently demonstrated that FGARs are more toxic than the EPA’s “moderately toxic” classification (Rattner and others, 2012;Vyas, 2013) and raptors may be especially sensitive to these compounds when multiple doses are consumed on multiple days (Rattner and Mastrota, 2018). Acute diphacinone toxicity tests indicate that Falco sparverius (American kestrels) are more than 20 times more sensitive than Colinus virginianus (northern bobwhite) and more than 30 times more sensitive than Anas platyrhynchos (mallards), two test species required by the EPA for pesticide registration (Rattner and others, 2010, 2011). Little is known about golden eagle sensitivity to ARs, although golden eagles fed muscle from diphacinone-treated sheep exhibited extreme weakness, ataxia (lack of muscle control), and hemorrhages (Savarie and others, 1979).
In addition to FGAR prairie dog baits, second generation anticoagulant rodenticides (SGARs) are commonly used to poison small mammals, including Mus spp. (mice) and Rattus spp. (rats) around buildings (Kelly Registration Systems, 2019). Compared to FGARs, the SGARs are more persistent in tissues (Erickson and Urban, 2004) and are also highly toxic to animals that consume poisoned prey; however, like FGARs, it can take several days for rodents to die after they consume a lethal dose (Cox and Smith, 1992). Furthermore, unlike FGARs, residues of SGARs in tissues can persist for months, increasing the risk of multiple feedings and secondary toxicity to predators (Rattner and Mastrota, 2018). Concentrations of the SGAR brodifacoum in Mustela erminea (stoats) were greater during the three months after the removal of baits than during the poison operation (Murphy and others, 1998); thus, SGARs appear to pose the greatest risk among ARs to predatory and scavenging birds (Erickson and Urban, 2004;Rattner and Mastrota, 2018).
Accipitridae (hawks) and Strigidae (owls) have succumbed to SGAR poisoning (Hegdal and Colvin, 1988; Murray and Tseng, 2008; Albert and others 2010) as these species are known to forage on the rodents that SGARs target. Concentrations of brodifacoum greater than 100–200 micrograms per kilogram (µg/kg) in Tyto alba (barn owls) have been associated with clinical signs of rodenticide poisoning and increased mortality (Shore and others, 2002); however, liver brodifacoum concentrations associated with toxicity can differ among species, and it is unclear if these criteria would apply to other raptor species (Thomas and others, 2011; Christensen and others, 2012;Nakayama and others, 2019).
Unlike the numerous reports on lead and mercury exposure, few reports have evaluated eagle exposure to ARs in the United States. In one report assessing liver AR residues and related mortality in 116 bald and 17 golden eagles, detectable sublethal concentrations were reported in 83 percent of bald eagles and 77 percent of golden eagles assessed and AR toxicosis was determined to be the cause of death in 4 percent of eagles (Niedringhaus and others, 2021). Similarly, a review of AR poisoning of nontarget animals by Nakayama and others (2019) determined that 80 percent of 25 bald eagles tested had detectible concentrations of ARs, with 19 of those detects being SGARs. Bald eagle mortality from ingesting chlorophacinone-poisoned prairie dogs has been reported in Nebraska and South Dakota (EPA, 2016). Although less is known regarding prevalence and effects of AR exposure in golden eagles, the availability of poisoned prey may exacerbate local golden eagle population declines (Golden and Gober, 2010).
Methods
USFWS and NWHC staff coordinated with North and South Dakota, Montana, Wyoming, Colorado, Utah, Nebraska, and Kansas State wildlife conservation officers to obtain opportunistically collected free-ranging bald and golden eagle carcasses during 2014–17. Additional carcasses of free-ranging eagles that had been stored frozen from 2011 to 2013 were obtained for this study from these same States. Relevant field history including species, date, actual or estimated global positioning system coordinates, or county-level locations within each State were recorded as available for each eagle collected.
Sample Criteria and Cause of Death Determination
Necropsies were done either at NWHC or, for specimens originating in Colorado, by Colorado Parks and Wildlife using similar criteria. A subset of carcasses meeting NWHC diagnostic case submission criteria (which includes birds that had available field history described previously, had not been frozen >6 months, and had not spent >72 hours in rehabilitation) were handled as diagnostic service cases and received comprehensive postmortem examinations done by board-certified veterinary pathologists and ancillary laboratory analysis to determine the cause of death (COD). If carcasses were in good postmortem condition, frozen for >6 months, had at least county-level location and the date of field recovery known, they were sent to NWHC but handled as special study cases, receiving gross postmortem examination and tissue collection for contaminant analysis for this study. COD was attempted on these cases based on gross postmortem examination only. In a few cases, no postmortem exam was performed, and tissues were collected in the field by USFWS staff for contaminant analysis. Carcasses in this group were placed into appropriate COD categories after contaminant analysis was completed, if possible, based on field history or other information; for example, if the COD was suspected or confirmed as gunshot in the field, it was placed in the trauma category or after contaminant analysis confirmed lead poisoning (>10 mg/kg ww) it was placed in the lead poisoning category.
For this study, COD is defined as the most important factor contributing to death based on a combination of the field history, gross and (or) microscopic assessment, and ancillary test results as available. Testing of lead, mercury, and rodenticides specifically for this study were not used to make a COD determination for diagnostic cases. Results of lead, mercury, and AR testing done at Michigan State University (MSU) Veterinary Diagnostic Laboratory were considered in COD determination for special study cases and field sampled specimens, as no ancillary testing was otherwise performed. For diagnostic cases submitted to the NWHC or Colorado Parks and Wildlife where lead poisoning was suspected as the COD, liver lead testing was done at the NWHC or Colorado State University, respectively. Rodenticide testing was also performed on two diagnostic cases as part of the COD determination process. In both cases, separate samples of liver were obtained for inclusion in this study. For the purpose of this study, diagnostic, special study cases, and field sampled specimens were divided into six simplified COD categories for analysis: (1) “trauma” for eagles with COD confirmed or suspected as a result of trauma including vehicle collision, intraspecies aggression, entrapment, gunshot, blunt force, or euthaniasia or death in captivity because of severity of trauma; (2) “electrocution” for eagles with COD confirmed or suspected as a result of electrocution; (3) “disease” for eagles with COD confirmed or suspected as a result of disease or other toxin not part of this study; (4) “lead toxicity” for eagles with COD confirmed or suspected of lead toxicity based on a combination of liver lead concentrations, postmortem lesions, and field history (Franson and Pain, 2011); (5) “other” for eagles that had other causes of death such as drowning, neoplasia, or emaciation/starvation, not fitting into the other categories; and (6) “undetermined” for eagles when a COD could not be identified, such as when necropsy results did not indicate a specific COD or if carcasses were unsuitable for necropsy because of postmortem autolysis. Age for each eagle was categorized as either adult (including subadult) or juvenile based on plumage, gonad development, and presence or absence of thymus and bursa of Fabricius. Sex was determined from gonad morphology and categorized as male, female, or unknown. At necropsy, a minimum of 5 grams (g) of liver was collected, placed into whirl-pak bag, and archived frozen for AR analysis; another 5 g of liver was collected for lead and mercury analysis at MSU. The number of carcasses collected per State varied depending on availability of suitable specimens.
Lead and Mercury Analysis
Liver samples collected from carcasses examined during 2014 were analyzed at NWHC using microwave digestion and flame atomic absorption spectroscopy as part of the COD determination. Briefly, liver samples were prepared and digested using a MARS 6 microwave digestion system (CEM Corporation, Matthews, North Carolina) in accordance with manufacturer’s instructions. Once samples were digested, they were transferred to the atomic absorption spectrometer (Thermo Solaar M6, Thermo Scientific, Wilmington, Delaware) and run according to manufacturer instructions. The limit of detection (LOD) was 0.25 mg/kg ww. This same process was followed for liver samples collected for COD determination on diagnostic cases in which lead was suspected during gross examination from 2015 to 2017. This was done to provide a timely COD diagnosis to the natural resources managing agency that submitted the carcass, and the NWHC lead results were not used for analysis in this study unless no MSU results were available.
Liver samples collected from carcasses examined from 2015 to 2017 were sent to the U.S. Geological Survey Forest and Rangeland Ecosystem Science Center (FRESC) or MSU, where they were weighed wet, dried at 75 °C overnight in a drying oven, and then weighed dry (less than or equal to [≤] 0.2 g). Lead and mercury residue analysis was done on all dried samples at MSU, by inductively coupled plasma mass spectrometry (Agilent 7900 ICP–MS, Agilent Technologies, Santa Clara, California) in accordance with manufacturer’s instructions. Dry samples were then digested with 1–2 milliliter (mL) concentrated 69–70 percent nitric acid (Avantor Performance Materials, Center Valley Pennsylvania, type J.T. Baker American Chemical Society grade) reagent in 15-mL digestion vessels (Savillex, Eden Prairie, Minnesota) in a 95-°C oven overnight. The amount of acid added was relative to the weight of sample. Overnight digests were then diluted 1:100 in Millipore Filter (Burlington, Massachusetts) deionized water before analysis. Standard reference materials (SRMs) used as quality control for this process included National Institute of Standards and Technology (Gaithersburg, Maryland) SRM 1577c Bovine Liver, National Institute of Standards and Technology SRM 2976 Mussel, and an in-house maintained tissue spike control and a digest blank. Lead ww sample concentrations are calculated by multiplying the dw concentration in micrograms per gram by the dw to ww ratio for individual samples. The LOD for liver samples analyzed by MSU varies depending on sample mass but is generally less than (<) 0.5 mg/kg dw and <0.1 mg/kg ww for mercury and lead, respectively. Results for lead are reported as milligrams per kilogram ww and categorized following Franson and Pain (2011) as background (<2.0 mg/kg), subclinical poisoning (2.0 to <6.0 mg/kg), clinical poisoning (6 to 10 mg/kg), and severe clinical poisoning (>10 mg/kg). Unless otherwise noted, concentrations of mercury are reported herein as dw and ARs are reported as ww. Concentrations of mercury in liver are categorized as low (0 to <20 mg/kg dw), moderate (20 to <80 mg/kg dw), and high (>80 mg/kg dw) following Weech and others (2003).
Anticoagulant Rodenticide Analysis
For AR testing, liver tissue was analyzed at MSU using a panel that includes FGARs chlorophacinone, diphacinone, and warfarin and SGARs brodifacoum, bromadiolone, difenacoum, and difethialone. Anticoagulants were identified and quantitated in tissues by gradient high performance liquid chromatography (HPLC) with ultraviolet photodiode array (PDA) and fluorescence (FL) detection. Brodifacoum and difenacoum were screened and quantitated by FL detection in a basic mobile phase system and confirmed by PDA in an acidic mobile phase system. Chlorophacinone, difethialone, and diphacinone were screened, confirmed, and quantitated by PDA in basic mobile phase. Bromodiolone and warfarin were screened and quantitated by PDA in an acidic mobile phase system; bromodiolone was confirmed by FL detection in a basic mobile phase system, whereas warfarin was confirmed by HPLC-electrospray quadra-pole mass spectrometry. This method was also available to confirm the remaining analytes in difficult situations that may arise with interfering chromatographic peaks. Gas chromatography mass spectrometry was also available for warfarin confirmation if needed. The LODs for AR analytes are as follows: brodifacoum 0.002 mg/kg ww; bromadiolone 0.02 mg/kg ww; chlorophacinone 0.2 mg/kg ww; difethialone 0.07 mg/kg ww; diphacinone 0.2 mg/kg ww; difenacoum 0.02 mg/kg ww; and warfarin 0.02 mg/kg ww. Concentrations of ARs were reported below LOD when positive values within the instrument's limit of quantification were confirmed by the methods described previously (for example, difenacoum confirmend by mobile acidic phase HPLC with PDA). Concentrations of ARs in eagles are reported as presence/absence because no toxic threshold concentrations are available for these species; however, we noted AR concentrations in eagles that were above a potentially lethal range for barn owls (in other words, AR >100–200 µg/kg; Shore and others, 2002).
Logistic regression was used to assess associations among detection versus nondetection of lead, mercury, and brodifacoum with COD, age, and sex. All analyses were done in a Bayesian framework R (R Core Team, 2018) using the library “rjags” (Plummer, 2018). For the linear regression, values below the LOD were input in jags as 0–LOD using the “dinterval” function (Plummer, 2018). For logistic and linear models, “State” (Wyoming, Montana, South Dakota, etc.) was included as a random effect, and evidence of spatial autocorrelation was tested using Moran's I (Cliff and Ord, 1981). Small sample sizes (in other words, typically n<10) precluded significance testing for differences in COD among the States where eagles were collected.
Mortality and Exposure Results
A total of 314 eagles (172 bald eagles and 142 golden eagles) collected in the eight States during 2014–17 (table 1) was included in this study. Age categories included 193 (61 percent) adult eagles, 106 (34 percent) juveniles, and 15 (5 percent) unknown age. At least gross postmortem examinations to evaluate COD were done on 295 specimens (174 diagnostic cases and 121 special study).
Table 1.
Breakdown of Haliaeetus leucocephalus and Aquila chrysaetos ages and how carcasses were handled for this study.[Data are summarized from Bodenstein and Lankton (2023)]
Cause of Death
Postmortem examination to evaluate COD was attempted on 295 carcasses. A COD was suspected or determined for 269 carcasses (91 percent), including 152 (88 percent) of 172 bald eagles and 117 (82 percent) of 142 golden eagles (tables 2 and 3); COD for the remainder of the birds was undetermined. Of those carcasses where COD was determined, trauma was the leading cause of mortality in both species, accounting for 138 (51 percent) of 269 eagle deaths, including 70 (46 percent) of 152 bald eagles and 68 (58 percent) of 117 golden eagles (tables 2 and 3). Electrocution was the second leading COD, affecting 56 (21 percent) of 269 birds (24 [16 percent] and 32 [27 percent] of 152 bald and 117 golden eagles, respectively).
Table 2.
Cause of death determination results for 172 Haliaeetus leucocephalus collected from eight States, 2014–17.[Data are summarized from Bodenstein and Lankton (2023)]
Table 3.
Cause of death determination results for 142 Aquila chrysaetos collected from eight States, 2014–17.[Data are summarized from Bodenstein and Lankton (2023)]
Lead toxicity was the third leading COD overall, with 55 (20 percent) of 269 birds affected; however, it was the second leading COD (47 [31 percent]) after trauma in 152 bald eagles (table 2). Only 8 (7 percent) of 117 golden eagles examined were determined to have died of lead poisoning (table 3). Disease and toxins (other than lead) caused the death of 10 eagles (6 bald, 4 golden). Ten eagles (5 bald, 5 golden) had other causes of mortality such as drowning, emaciation, starvation, and neoplasia. Cause of death could not be determined for 45 eagles (20 bald, 25 golden). Eagles that had a postmortem examination attempted to determine COD and at least county-concentration location are summarized in figure 1.
Lead Results
A total of 299 of 314 eagle carcasses collected had liver available to measure concentrations of lead (table 4). Overall, 75 (25 percent) of 299 eagles tested had some concentration of lead poisoning (in other words, liver lead >2 mg/kg ww; Franson and Pain, 2011). When compared by species, 60 (36 percent) of 166 bald eagles had evidence of lead poisoning compared to 15 (11 percent) of 133 golden eagles. Eagles that had liver analyzed for lead exposure and at least county-concentration location are summarized in figure 2.
Table 4.
Categorized liver lead concentrations for 166 Haliaeetus leucocephalus and 133 Aquila chrysaetos collected from eight States, 2014–17.[Data are summarized from Bodenstein and Lankton (2023). <, less than; >, greater than; gray shading in the columns for liver concentrations from 2 to <10 denotes liver lead concentrations that exceed clinical or subclinical thresholds for lead poisoning in raptors (Franson and Pain, 2011)]
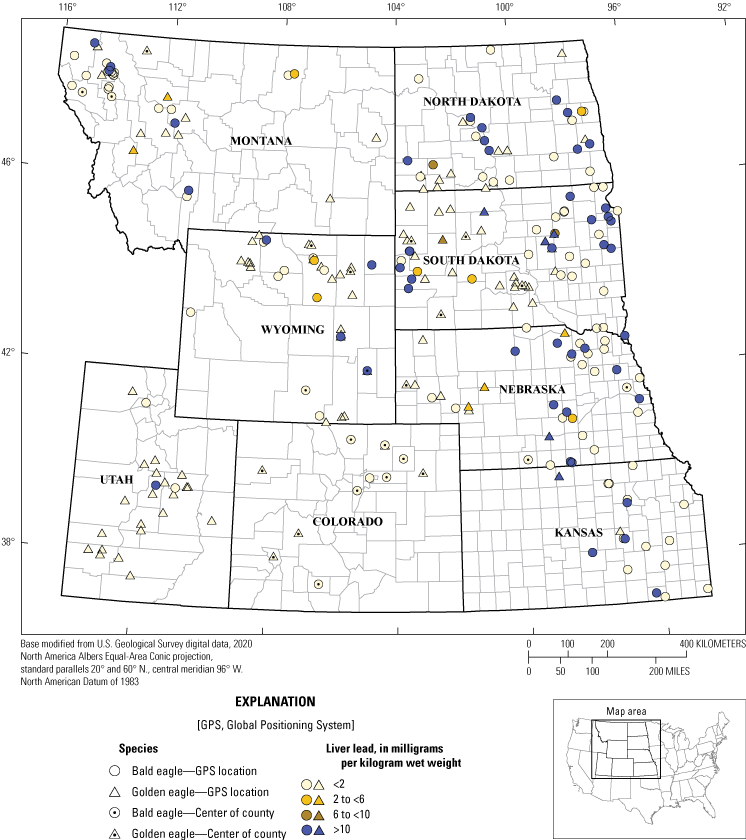
Lead concentration test results for 155 Haliaeetus leucocephalus (bald eagles) and 118 Aquila chrysaetos (golden eagles) with actual, estimated, or county-level locations from eight States from 2014 to 2017. Note: the data point for eagles that only had county-level location information recorded (n=40) was placed in the centroid of the county. Specimens with no recorded county-level information (n=26) were not included.
Lead concentrations were indicative of severe clinical lead toxicity (>10 mg/kg ww; Franson and Pain, 2011) in 56 (19 percent) of eagles tested: 50 (30 percent) of 166 bald eagles and 6 (4.5 percent) of 133 golden eagles (table 4). The highest lead concentration was 95.57 mg/kg ww, which was detected in an adult female bald eagle collected in Nebraska. Only three eagles (1 percent) had liver lead concentrations within the range indicative of clinical lead poisoning (in other words, 6–10 mg/kg ww; Franson and Pain, 2011). Causes of death determined for these 3 birds were trauma (2) and lead toxicity (1).
Subclinical lead toxicity concentrations (2.0 to <6.0 mg/kg ww) were detected in 16 (5 percent) of 299 eagles. Causes of death assigned to eagles in this category were trauma (9), undetermined (3), other (2), electrocution (1), and lead toxicity (1). Of the remaining 224 eagles, lead was detected at background concentrations (<2.0 mg/kg) in 125 eagles, and 99 eagles had no lead detected to the LOD (<0.25 mg/kg). Of the 138 birds that died because of trauma, 130 were tested for lead, and 117 of these eagles had concentrations of lead in the liver in the background range (<2.0 mg/kg ww). For eagles with trauma as the primary COD, five bald eagles and four golden eagles had lead concentrations in the subclinical range. In the clinical range there were four bald eagles with trauma as the primary COD. Three of 56 eagles that died because of electrocution also had toxic liver lead concentrations indicating severe clinical lead toxicity (>10 mg/kg ww).
Regression analyses indicated that adult bald eagles had liver lead concentrations that were about 52 percent higher than juveniles, and male bald eagles had lead concentrations that were about 28 percent lower than females (table 5); however, differences among adults and juveniles and males and females were not statistically significant (all estimated differences overlapped zero; table 5). Lead concentrations did not differ among bald eagles diagnosed with trauma, electrocution, infectious disease, or other COD (except lead poisoning; table 5), indicating no association between these causes of death and liver lead concentrations. For golden eagles, adult lead concentrations were about 81 percent higher than juveniles (table 5). Male golden eagles also had liver lead concentrations about 18 percent lower than females; however, this difference was not statistically significant. Golden eagles dying of causes other than lead toxicity had lead concentrations approximately 92–94 percent lower than eagles dying of lead poisoning.
Table 5.
Percent change estimates in lead concentrations by age, sex, and cause of death from Haliaeetus leucocephalus and Aquila chrysaetos from eight States, 2014–17.[Data are summarized from Bodenstein and Lankton (2023). %, percent; vs., versus; COD, cause of death; other, includes everything other than trauma, lead, and electrocution]
For bald and golden eagles combined, odds of an adult eagle dying of lead poisoning were 11 times greater than a juvenile (table 6). Odds of a male eagle dying of lead poisoning are about half that of a female (table 6). Bald eagles were nine times more likely to die of lead poisoning than golden eagles (table 6). These differences were statistically significant (in other words, odds ratios did not overlap one). Results for whether eagles had high concentrations of lead >10 mg/kg ww versus below 10 mg/kg ww were similar (table 6).
Table 6.
Odds ratios for eagle lead cause of death count and high liver lead concentration by age, sex, and species.[Data are summarized from Bodenstein and Lankton (2023). COD, cause of death; vs., versus; bald, Haliaeetus leucocephalus (bald eagle); golden, Aquila chrysaetos (golden eagle); >, greater than; <, less than]
Mercury Results
Liver mercury concentrations were available for 277 eagles in the study: 159 bald eagles and 118 golden eagles (table 7). For bald eagles, three (2 percent) birds had high concentrations of mercury (in other words, >80 mg/kg dw). Eleven (7 percent) bald eagles had moderate mercury concentrations that ranged from 20.85 to 78.16 mg/kg. Low concentrations (<20 mg/kg) were determined in the remaining 145 bald eagles. No golden eagles had high liver mercury concentrations. The remaining 117 (99 percent) golden eagles had low mercury exposure (<20 mg/kg). Eagles that had liver analyzed for mercury exposure and at least county-concentration location are summarized in figure 3.
Table 7.
Categorized concentrations of mercury in liver tissue of 159 Haliaeetus leucocephalus and 118 Aquila chrysaetos collected from eight States, 2014–17.[Data are summarized from Bodenstein and Lankton (2023). <, less than; >, greater than]
Overall, adult eagles were more likely to have detections of mercury than juveniles, and female eagles were more likely to have higher concentrations than males (table 8). Adult eagles had overall higher mercury concentrations than juvenile eagles, and bald eagles had overall higher concentrations than golden eagles (table 8).
Table 8.
Odds ratios for detection versus nondetection of mercury as a function of age, sex, and species and effect sizes from linear regression models of mercury concentration as a function of age, sex, and species.[Data are summarized from Bodenstein and Lankton (2023). vs., versus; bald, Haliaeetus leucocephalus (bald eagle); golden, Aquila chrysaetos (golden eagle); %, percent]
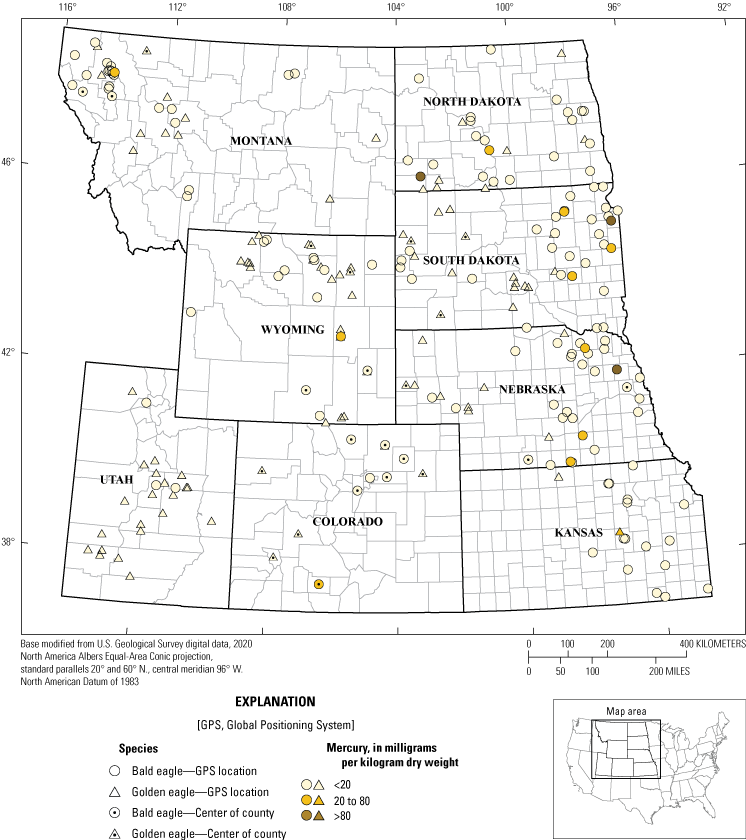
Mercury concentration test results for 148 Haliaeetus leucocephalus (bald eagles) and 103 Aquila chrysaetos (golden eagles) with actual, estimated, or county-level locations collected from eight States, 2014–17. Note: the data point for eagles that only had county-level location information recorded (n=39) was placed in the centroid of the county. Specimens with no recorded county-level information (n=26) were not included.
Anticoagulant Rodenticide Results
A total of 298 eagles (165 bald eagles and 133 golden eagles) had liver samples tested for ARs. Of the two FGARs included in the panel, chlorophacinone was not detected in any eagles tested; diphacinone was detected once, in a juvenile golden eagle from Wyoming. Warfarin was not detected in liver tissues of birds tested.
Unlike FGARs, SGARs (mostly brodifacoum) were frequently detected in liver tissue of bald and golden eagles. Brodifacoum was detected in 166 (56 percent) of 298 eagles tested: 114 (70 percent) bald eagles and 52 (39 percent) golden eagles (table 9). Eagles that had liver analyzed for lead exposure and at least county-concentration location are summarized in figure 4. Concentrations of brodifacoum ranged from 0.002 to 2.64 mg/kg ww. Of the leading causes of mortality, 54 percent of trauma, 46 percent of electrocution, and 89 percent of lead toxicity cases had evidence of exposure to brodifacoum. Kansas had the highest proportion of bald eagles (15 of 16) with detectable concentrations of brodifacoum.
Table 9.
Categorized concentrations of brodifacoum in liver tissue of 165 Haliaeetus leucocephalus and 133 Aquila chrysaetos collected from eight States, 2014–17.[Data are summarized from Bodenstein and Lankton (2023). <, less than; >, greater than]
In bald and golden eagles, the odds of brodifacoum detections and the concentration of brodifacoum in liver were statistically higher in adult eagles versus juvenile eagles and in bald eagles versus golden eagles (table 10). Moran’s I and spatial variograms indicated no spatial patterns in brodifacoum concentrations.
Table 10.
Odds ratios for detection versus nondetection of brodifacoum as a function of age, gender, and eagle species, and effects sizes for brodifacoum concentrations as a function of age, gender, and eagle species.[Data are summarized from Bodenstein and Lankton (2023). vs., versus; bald, Haliaeetus leucocephalus (bald eagle); golden, Aquila chrysaetos (golden eagle); %, percent]
Other less common SGAR detections included difenacoum (number of eagles [n]=3), difethialone (n=1), and bromadiolone (n=1). The four eagles with detectable difenacoum or bromadiolone also had detectable concentrations of brodifacoum.
In total, 9 (5 percent) of 165 bald eagles and 3 (2 percent) of 133 golden eagles were determined to have liver concentrations of brodifacoum in a potentially lethal range (>0.2 mg/kg ww) reported for barn owls (Shore and others, 2002); however, 10 of these eagles had CODs other than AR poisoning and two had undetermined CODs.
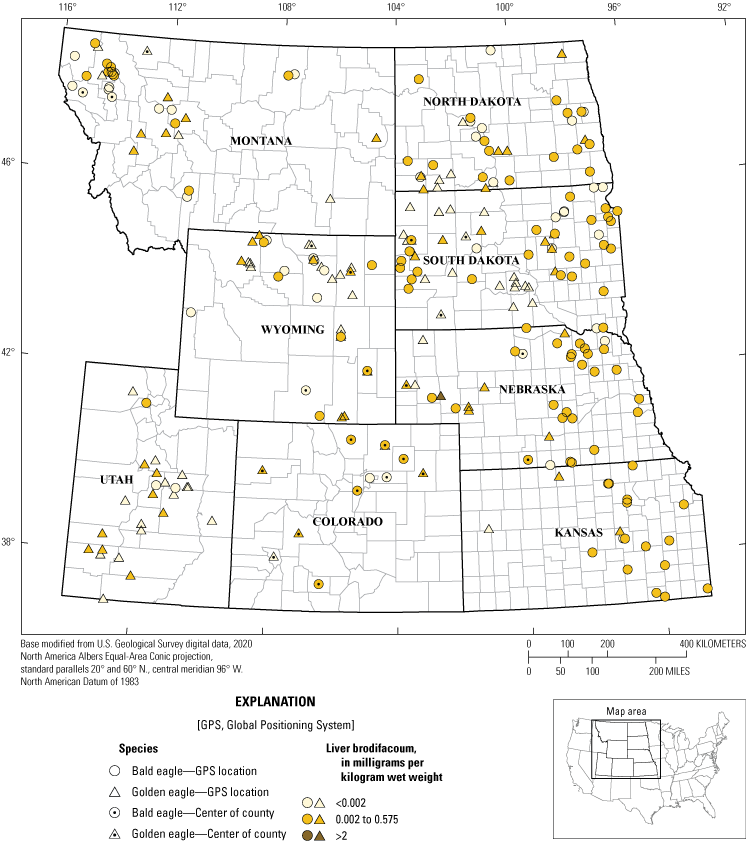
Brodifacoum concentration test results for 154 Haliaeetus leucocephalus (bald eagles) and 118 Aquila chrysaetos (golden eagles) with actual, estimated, or county-level locations from eight States, 2014–17. Note: the data point for eagles that only had county-level location information recorded (n=37) was placed in the centroid of the county. Specimens with no recorded county-level information (n=26) were not included.
Evaluation of Causes of Death and Contaminant Exposure
Our study objective was to evaluate COD and contaminant exposure (lead, mercury, and ARs) in suitable eagle remains from eight States for which data on eagle COD and contaminant exposure were lacking. Of those eagles that were necropsied for COD determination, the leading causes of death were trauma, lead poisoning, and electrocution. Our results correspond with those of previous reports, with trauma and poisoning (including by lead) identified as the two leading causes of death for 2,980 bald eagles and 1,427 golden eagles from 1975 to 2013 (Russell and Franson, 2014) and trauma (including electrocution) identified as the most common cause of death in a survey of more than 300 bald and golden eagles submitted to the Southeastern Cooperative Wildlife Disease Study from 2014 to 2018 (Niedringhaus and others, 2021).
The frequency of elevated liver lead concentrations in birds in this report (36 and 11 percent for bald and golden eagles, respectively) is similar to those previously reported in national surveys (Slabe and others, 2022). In our study, adult female bald eagles tended to have the highest lead concentrations, were more likely to be diagnosed with lead as a COD and were more likely to have liver lead concentrations indicative of severe clinical lead poisoning (in other words, liver lead >10 mg/kg ww). The foraging behavior and diets of bald eagles within the study area may make them more susceptible to lead poisoning than golden eagles. Golden eagles do not frequently feed on carrion; their primary diet of small mammals (for example, Leporidae [rabbits], ground squirrels, and prairie dogs) are often directly captured (Buehler, 2000;Kochert and others, 2002). Bald eagles are attracted to hunting areas to scavenge hunter-shot deer (Bedrosian and others, 2012; Cruz-Martinez and others, 2012); furthermore, bald eagles, unlike golden eagles, often feed on fish (McEwan and Hirth, 1980). Fish-eating bald eagles can be at risk of lead exposure by ingesting lead sinkers and fishing tackle (Scheuhammer and Norris, 1996; Bruggeman and others, 2018). We did not evaluate diet as part of this study and although bald eagles can be piscivorous, they are opportunistic predators and carrion scavengers, and their food preferences may differ geographically and temporally (Buehler, 2000).
Field evidence and previous studies, which included radiographs and postmortem examinations, strongly indicate that ingestion of bullet fragments is a contributor to elevated concentrations of lead in eagle tissues (Kramer and Redig, 1997; Johnson and others, 2007; Hunt and others, 2009; Stauber and others, 2010; Kelly and others, 2011; Cruz-Martinez and others, 2012; Bellinger and others, 2013; Mierzykowski and others, 2013; Warner and others, 2014; McTee and others, 2017; Harris, 2018; Pain and others, 2009, 2019). The higher frequency of lead poisoning in bald eagles (36 percent) in this study than golden eagles (11 percent) is likely because bald eagles are more prone to feeding on lead-shot game and varmit (for example, Canis latrans [coyote], prairie dogs), perhaps combined with additional exposure to lead from the ingestion of lead fishing tackle when consuming fish.
The propensity for lead poisoning in our sampled female bald eagles compared to males may be because of differences in size. Female bald eagles are typically larger than their male counterparts and are approximately 20 percent heavier (Bortolotti, 1984). This difference in size likely contributes to females being more successful at supplanting males from carrion (Garcelon, 1990). Age can also be a factor for supplanting success (Garcelon, 1990), and all 120 adult bald eagles had measurable concentrations of liver lead whereas only 39 (85 percent) of 46 juvenile bald eagles had detectable liver lead concentrations. The lower prevalence of liver lead detection in juvenile eagles may also be related to less time for exposure and accumulation in younger birds.
In addition to mortality from exposure to lethal concentrations, lead acts as a nonspecific poison affecting all body systems resulting in a wide range of sublethal effects in animals (Franson and Pain, 2011). Sublethal effects of lead exposure in birds includes reduced blood hemoglobin concentrations, anemia, and immunosuppression (Franson and Pain, 2011); moreover, lead poisoning disrupts normal liver and kidney functioning and disrupts the central and peripheral nervous systems (USFWS, 2013). These sublethal effects can be subtle and may contribute to mortality indirectly or decrease an eagle’s ability to produce viable offspring (fitness); thus, our eagle COD determinations are likely an underestimate of the harmful effects of lead on eagles.
Our results indicate that mercury exposure does not appear to be an issue for golden eagles from our study area; however, in bald eagles, high concentrations (>80 mg/kg dw) were detected in 3 adults, and 11 had moderate (20–80 mg/kg dw) concentrations, indicating potential effects from mercury. The more frequent and higher concentrations of mercury in bald than golden eagles are likely because of differences in diet, with bald eagles tending to eat more fish. Although bald eagles may be less susceptible to mercury poisoning than other avian species considered to be at high risk of mercury exposure (Evers, 2018; Scheuhammer and others, 2008), monitoring of mercury in bald eagles may be useful to further evaluate the observed upward trend of mercury accumulation (Carlson and others, 2012). Mercury biomagnification in aquatic food webs often results in high concentrations of mercury in fish-eating birds (Wiener and others, 2003). Bald eagles with exposure to mercury from fish ingestion is a concern given that 49 percent of the sampled population of lakes in the United States have fish with concentrations of mercury above the EPA’s human health criterion of 0.3 mg/kg ww (EPA, 2001). Because eagles are top predators and mercury biomagnifies between trophic levels, this 0.3 mg/kg ww criterion may be protective of bald eagles only if it is applied to the highest trophic level fish (Russell, 2003).
Female bald eagles tended to have the highest concentrations of mercury in liver, including the two highest in our study (312 and 145 mg/kg dw). These two high mercury concentrations in bald eagles from our study are greater than concentrations of mercury in liver samples from 89 bald eagles collected across Canada in the 1990s (0.5–104 mg/kg dw; Scheuhammer and others, 2008) or 16 adult bald eagles from Florida in the 1990s (maximum liver mercury concentration of approximately 42.07 mg/kg dw; Wood and others, 1996). Average concentrations of total mercury in bald eagles from our study (11.6±2.3 mg/kg dw, n=159) were similar to those reported for 82 bald eagles from British Columbia (11.8±1.7 mg/kg dw, n=82, Weech and others, 2003) and higher than reported for bald eagles from the Great Lake States (7.97±0.9 mk/kg dw, n=135, Rutkiewicz and others, 2011); however, bald eagles in Maine had similar average concentrations of mercury (13.5±2.6 mg/kg dw, n=127; Mierzykowski and others, 2013) compared to those detected in our study.
Brodifacoum was the most common AR identified in eagle livers in our study, detected in 56 percent of eagles tested, including 70 percent of bald eagles and 39 percent of golden eagles. The frequency of brodifacoum exposure in eagles from our study, compared to other anticoagulant rodenticides, is likely because of its frequency of use and toxicokinetics, which makes it more common and persistent in animal tissues (Nakayama and others, 2019). Brodifacoum and other SGARs are considerably more persistent in animal tissues than are FGARs, and bioaccumulation may increase whole-body residues with repeat feedings on contaminated carcasses (Erickson and Urban, 2004). Measured liver retention in rats ranged from a 350-day half-life for brodifacoum to 90 days for diphacinone (Erickson and Urban, 2004). A recent review of anticoagulant poisoning (Nakayama and others, 2019) reported detection rates for ARs in bald eagles similar to those reported in this study, with brodifacoum detected in 18 of 24 (75 percent) bald eagles compared to no detections of chlorophacinone or diphacinone. In another recent report, the prevalence of brodifacoum exposure was slightly higher, with 85 percent of of bald eagles and 65 percent of golden eagles tested between 2014 and 2018 having detectable concentrations of brodifacoum (Niedringhaus and others, 2021).
Exposure to FGARs in our study area was only detected once (diphacinone concentration of 0.30 mg/kg ww) in a golden eagle that died from electrocution in Wyoming. Eagles that die from exposure to FGARs have been found in or near prairie dog towns (EPA, 2016), but their carcasses may not be detected in remote locations.
The high number of brodifacoum detections compared to other SGARs (bromadiolone, difethialone, and difenacoum) may result from its lower LOD in our study (in other words, <0.002 for brodifacoum compared to 0.02 mg/kg for bromadiolone and difenacoum and 0.07 mg/kg for difethialone), its more frequent use, and (or) its greater persistence in tissues. Brodifacoum has a greater potential hepatic half-life (350 days) than bromadiolone (318 days), difenacoum (128 days), or difethialone (126 days; EPA, 2007). Out of 4,891 nontarget animals tested for ARs, brodifacoum had the highest detection rate (31 percent) followed closely by bromadiolone at 30 percent (Nakayama and others, 2019); however, in the United Kingdom, bromadiolone was the most frequently detected SGAR in Milvus milvus (red kites) at 52.2 percent compared to 30.4 percent for brodifacoum (Walker and others, 2008). The frequency of use for SGARs in our study area is unknown, although all the SGARs detected in this study are registered for use on similar pest species (rats and mice; Kelly Registration Systems, 2019).
In South Dakota, 18 rodenticides are registered for use with brodicacoum as the active ingredient (Kelly Registration Systems, 2019). Pests targeted for poisoning by brodifacoum products, according to the product labels, include several species of the family Muridae spp. (mice, rats, and woodrats). These small mammal species are not known to make up the bulk of a bald eagle’s diet, which consists mostly of fish (McEwan and Hirth, 1980); thus, feeding on the primary targets of these rodenticides is not likely the primary exposure pathway for bald eagles (Nakayama and others, 2019). Instead, exposure through ingestion of nontarget animals (for example, rabbits, Procyonidae spp. [raccoons]) may be an important exposure pathway to eagles (Nakayama and others, 2019), and tertiary exposure may happen through consumption of larger contaminated carcasses including coyotes and Canidae spp. (foxes; Erickson and Urban, 2004). Tertiary exposure to SGARs has been reported for other species including canids that fed on Suidae spp. (wild pigs) known to scavenge Didelphis virginiana (Virginia opossums) poisoned by the SGAR brodifacoum (Eason and others, 1999). Although brodificoum was frequently detected in eagle livers, the effect to health remains unknown. Despite the frequency of detection, in no case was death directly attributed to AR poisoning. Studies that track raptor survival in the wild that include an emphasis on the dose-response relations among hepatic AR concentrations and both hemorrhage and mortality would be beneficial to better understand AR poisoning in raptors (Walker and others, 2008;Nakayama and others, 2019;Rattner and Harvey, 2021).
Summary
In conclusion, we report that opportunistically recovered Haliaeetus leucocephalus (bald eagle) and Aquila chrysaetos (golden eagle) remains from eight western and midwestern States died most frequently from trauma, electrocution, and poisonings. Lead poisoning is a problem in this region for bald and golden eagles and especially for adult female bald eagles that are likely exposed when scavenging prey contaminated with lead bullet fragments or lead fishing tackle. Bald and golden eagles in the region are also exposed to potentially lethal concentrations of anticoagulant rodenticides (ARs), although more studies would be beneficial to further evaluate effects to eagles from AR exposure. Mercury exposure also is not uncommon for bald eagles documented in this region, and although mercury exposure was not identified as frequently as lead poisoning or AR exposure, it may become more of a concern in the future.
References Cited
Albert, C.A., Wilson, L.K., Mineau, P., Trudeau, S., and Elliott, J.E., 2010, Anticoagulant rodenticides in three owl species from western Canada, 1988–2003: Archives of Environmental Contamination and Toxicology, v. 58, no. 2, p. 451–459. [Also available at https://doi.org/10.1007/s00244-009-9402-z.]
Avery, D., and Watson, R.T., 2009, Regulation of lead-based ammunition around the world, in Watson, R.T., Fuller, M., Pokras, M., and Hunt. G., eds., Ingestion of Lead from Spent Ammunition—Implications for Wildlife and Humans Conference, Boise, Idaho, May 12–15, 2008, Proceedings: Boise, Idaho, The Peregrine Fund, p. 161–168. [Also available at https://doi.org/10.4080/ilsa.2009.0115.]
Bechard, M.J., Perkins, D.N., Kaltenecker, G.S., and Alsup, S., 2009, Mercury contamination in Idaho bald eagles, Haliaeetus leucocephalus: Bulletin of Environmental Contamination and Toxicology, v. 83, no. 5, p. 698–702. [Also available at https://doi.org/10.1007/s00128-009-9848-8.]
Bedrosian, B., Craighead, D., and Crandall, R., 2012, Lead exposure in bald eagles from big game hunting, the continental implications and successful mitigation efforts: PLoS One, v. 7, no. 12, p. e51978, accessed December 2022 at https://doi.org/10.1371/journal.pone.0051978.
Bellinger, D.C., Bradman, A., Burger, J., Cade, T.J., Cory-Slechta, D.A., Doak, D., Finkelstein, M., Flegal, A.R., Fry, M., Green, R.E., Hu, H., Jacobs, D.E., Johnson, C., Kelly, T., Kosnett, M., Landrigan, P.J., Lanphear, B., Mielke, H.W., Newton, I., Pokras, M.A., Poppenga, R.H., Redig, P.T., Rideout, B.A., Risebrough, R.W., Scheuhammer, T., Silbergeld, E., Smith, D.R., Strupp, B., Thomas, V.G., and Wright, R., 2013, Health risks from lead-based ammunition in the environment—A consensus statement of scientists: University of California, Santa Cruz Microbiology and Environmental Toxicology Department, 7 p., accessed December 2022 at https://escholarship.org/uc/item/6dq3h64x.
Bodenstein, B.L., and Lankton, J.S., 2023, USGS National Wildlife Health Center necropsy and contaminant results for bald and golden eagles collected in 8 States from January 1, 2014, through December 31, 2017, to determine cause of illness/death and lead, mercury, and anticoagulant rodenticide exposure: U.S. Geological Survey data release, https://doi.org/10.5066/P9F3ZPD7.
Bortolotti, G.R., 1984, Criteria for determining age and sex of nestling bald eagles: Journal of Field Ornithology, v. 55, no. 4, p. 467–481. [Also available at https://sora.unm.edu/sites/default/files/journals/jfo/v055n04/p0467-p0481.pdf.]
Bruggeman, J.E., Route, W.T., Redig, P.T., and Key, R.L., 2018, Patterns and trends in lead (Pb) concentrations in bald eagle (Haliaeetus leucocephalus) nestlings from the western Great Lakes region: Ecotoxicology, v. 27, no. 5, p. 605–618. [Also available at https://doi.org/10.1007/s10646-018-1933-5.]
Buehler, D.A., 2000, Bald eagle (Haliaeetus leucocephalus; ver. 2.0), in Poole, A.F., and Gill, F.B., eds., The birds of North America: Ithaca, N.Y., Cornell Lab of Ornithology, accessed December 2022 at https://doi.org/10.2173/bna.506.
Carlson, J.T., Harmata, A.R., and Restani, M., 2012, Environmental contaminants in nestling bald eagles produced in Montana and Wyoming: The Journal of Raptor Research, v. 46, no. 3, p. 274–282. [Also available at https://doi.org/10.3356/JRR-11-68.1.]
Christensen, T.K., Lassen, P., and Elmeros, M., 2012, High exposure rates of anticoagulant rodenticides in predatory bird species in intensively managed landscapes in Denmark: Archives of Environmental Contamination and Toxicology, v. 63, no. 3, p. 437–444. [Also available at https://doi.org/10.1007/s00244-012-9771-6.]
Cox, P., and Smith, R.H., 1992. Rodenticide ecotoxicology—Pre-lethal effects of anticoagulants on rat behaviour, in Borrecco, J.E., and Marsh, R.E., eds., Proceedings of the Fifteenth Vertebrate Pest Conference 1992, Newport Beach, Calif., March 3–5, 1992: New Beach, Calif., University of California, Davis, 165–170 p., accessed December 2022 at https://digitalcommons.unl.edu/vpc15/.
Cruz-Martinez, L., Redig, P.T., and Deen, J., 2012, Lead from spent ammunition—A source of exposure and poisoning in bald eagles: Human-Wildlife Interactions, v. 6, no. 1, p. 94–104. [Also available at https://doi.org/10.26077/sdgs-cs63.]
Dziok, T., Bury, M., Bytnar, K., and Burmistrz, P., 2021, Possibility of using alternative fuels in Polish power plants in the context of mercury emissions: Waste Management (New York, N.Y.), v. 126, p. 578–584. [Also available at https://doi.org/10.1016/j.wasman.2021.03.053.]
Eagles-Smith, C.A., Willacker, J.J., and Flanagan Pritz, C.M., 2014, Mercury in fishes from 21 national parks in the Western United States—Inter- and intra-park variation in concentrations and ecological risk: U.S. Geological Survey Open-File Report 2014–1051, 54 p., accessed December 2022 at https://doi.org/10.3133/ofr20141051.
Eason, C.T., Milne, L., Potts, M., Morriss, G., Wright, G.R.G., and Sutherland, O.R.W., 1999, Secondary and tertiary poisoning risks associated with brodifacoum: New Zealand Journal of Ecology, v. 23, no. 2, p. 219–224. [Also available at https://newzealandecology.org/nzje/2078.pdf.]
Erickson, W., and Urban, D., 2004, Potential risks of nine rodenticides to birds and nontarget mammals—A comparative approach: Washington, D.C., U.S. Environmental Protection Agency, 230 p., accessed December 2022 at https://www.fluoridealert.org/wp-content/pesticides/EPA-HQ-OPP-2006-0955-0005.pdf.
Evers, D.C., 2018, The effects of methylmercury on wildlife—A comprehensive review and approach for interpretation, inDellasala, D.A., and Goldstein, M.I., eds., Encyclopedia of the Anthropocene: Oxford, England, Elsevier, p. 181–194. [Also available at https://doi.org/10.1016/B978-0-12-809665-9.09985-7.]
Franson, C.J., and Pain, D.J., 2011, Lead in birds, inBeyer, N.W., and Meador, J.P., eds., Environmental contaminants in biota—Interpreting tissue concentrations: Boca Raton, Fla., CRC Press, p. 563–594. [Also available at https://digitalcommons.unl.edu/cgi/viewcontent.cgi?article=1984&context=usgsstaffpub.]
Garcelon, D.K., 1990, Observations of aggressive interactions by bald eagles of known age and sex: The Condor, v. 92, no. 2, p. 532–534. [Also available at https://doi.org/10.2307/1368256.]
Golden, N.H., and Gober, J., 2010, Prairie dogs, pesticides, and protected species—Concerns for anticoagulant use in a sensitive ecosystem, in Trimm, R.M., and Eagerstone, K.A., eds., Proceedings of the 24th Vertebrate Pest Conference, Sacramento, Calif., February 22–25, 2010: Sacramento, Calif., University of California, Davis, p. 181–185, accessed December 2022 at https://escholarship.org/content/qt2tp6g6mn/qt2tp6g6mn_noSplash_c23d9ff939ecad96ab47c5c3691fc101.pdf?t=pj62po.
Hanley, B.J., Dhondt, A.A., Forzán, M.J., Bunting, E.M., Pokras, M.A., Hynes, K.P., Dominguez-Villegas, E., and Schuler, K.L., 2022, Environmental lead reduces the resilience of bald eagle populations: The Journal of Wildlife Management, v. 86, no. 2. [Also available at https://doi.org/10.1002/jwmg.22177.]
Harmata, A.R., and Oakleaf, B., 1992, Bald eagles of the greater Yellowstone ecosystem—An ecological study with emphasis on the Snake River, Wyoming: Cheyenne, Wyoming, Wyoming Game and Fish Department 272 p., accessed December 2022 at https://ia803007.us.archive.org/10/items/baldeaglesingrea00harm/baldeaglesingrea00harm.pdf.
Harris, E., 2018, Full of lead—How bullets are poisoning eagles [Jim Briggs and Fernando Arruda, original score and sound design; The Center for Investigative Report and PRX co-producers]: Reveal podcast, 00:14:46, posted April 5, 2018, accessed December 2022 at https://www.revealnews.org/episodes/full-of-lead-how-bullets-are-poisoning-eagles/.
Hegdal, P.L., and Colvin, B.A., 1988, Potential hazard to eastern screech-owls and other raptors of brodifacoum bait used for vole control in orchards: Environmental Toxicology and Chemistry, v. 7, no. 3, p. 245–260. [Also available at https://doi.org/10.1002/etc.5620070309.]
Herring, G., Eagles-Smith, C.A., and Wagner, M.T., 2016, Ground squirrel shooting and potential lead exposure in breeding avian scavengers: PLoS One, v. 11, no. 12, p. e0167926, accessed December 2022 at https://doi.org/10.1371/journal.pone.0167926.
Hunt, W.G., Watson, R.T., Oaks, J.L., Parish, C.N., Burnham, K.K., Tucker, R.L., Belthoff, J.R., and Hart, G., 2009, Lead bullet fragments in venison from rifle-killed deer—potential for human dietary exposure: PLoS One, v. 4, no. 4, p. e5330, accessed December 2022 at https://doi.org/10.1371/journal.pone.0005330.
Johnson, C.K., Vodovoz, T., Boyce, W.M., and Mazet, J.A.K., 2007, Lead exposure in California condors and sentinel species in California: Wildlife Health Center, University of California, Davis, 36 p., accessed December 2022 at https://www.biologicaldiversity.org/species/birds/California_condor/pdfs/Johnson-et-al-2007-DFG-Report.pdf.
Kelly Registration Systems, 2019, South Dakota pesticide registration search: Kelly Registration Systems database, accessed December 2022 at http://www.kellysolutions.com/SD/.
Kelly, T.R., Bloom, P.H., Torres, S.G., Hernandez, Y.Z., Poppenga, R.H., Boyce, W.M., and Johnson, C.K., 2011, Impact of the California lead ammunition ban on reducing lead exposure in golden eagles and turkey vultures: PLoS One, v. 6, no. 4, p. e17656, accessed December 2022 at https://doi.org/10.1371/journal.pone.0017656.
Knopper, L.D., Mineau, P., Scheuhammer, A.M., Bond, D.E., and McKinnon, D.T., 2006, Carcasses of shot Richardson’s ground squirrels may pose lead hazards to scavenging hawks: The Journal of Wildlife Management, v. 70, no. 1, p. 295–299. [Also available at https://doi.org/10.2193/0022-541X(2006)70[295:COSRGS]2.0.CO;2.]
Kochert, M.N., Steenhof, K., McIntyre, C.L., and Craig, E.H., 2002 Golden eagle (Aquila chrysaetos; ver. 2.0). in Poole, A.F., and Gill, F.B., eds., The birds of North America: Ithaca, N.Y., Cornell Lab of Ornithology, 44 p., accessed December 2022 at https://birdsoftheworld.org/bow/managed-content/bna-pdfs/BNA-684-goleag.pdf.
Kramer, J.L., and Redig, P.T., 1997, Sixteen years of lead poisoning in eagles, 1980–95—An epizootiologic view: The Journal of Raptor Research, v. 31, no. 4, p. 327–332. [Also available at https://sora.unm.edu/sites/default/files/journals/jrr/v031n04/p00327-p00332.pdf.]
Leith, K.F., Bowerman, W.W., Wierda, M.R., Best, D.A., Grubb, T.G., and Sikarske, J.G., 2010, A comparison of techniques for assessing central tendency in left-censored data using PCB and p,p′DDE contaminant concentrations from Michigan’s Bald Eagle Biosentinel Program: Chemosphere, v. 80, no. 1, p. 7–12. [Also available at https://doi.org/10.1016/j.chemosphere.2010.03.056.]
McEwan, L.C., and Hirth, D.H., 1980, Food habits of the bald eagle in north-central Florida: The Condor, v. 82, no. 2, p. 229–231. [Also available at https://doi.org/10.2307/1367485.]
McTee, M., Young, M., Umansky, A., and Ramsey, P., 2017, Better bullets to shoot small mammals without poisoning scavengers: Wildlife Society Bulletin, v. 41, no. 4, p. 736–742. [Also available at https://doi.org/10.1002/wsb.822.]
Mierzykowski, S.E., Todd, C.S., Pokras, M.A., and Oliveira, R.D., 2013, Lead and mercury levels in livers of bald eagles recovered in New England: U.S. Fish and Wildlife Service Maine Field Office special project report FY13–MEFO–2–EC, 26 p., accessed December 2022 at https://legislature.vermont.gov/Documents/2016/WorkGroups/House%20Fish%20and%20Wildlife/Bills/H.460/Witness%20Testimony/H.460~Dr.%20Mark%20Potras~Lead %20and%20Mercury%20Levels%20in%20Livers%20of%20Bald%20Eagles%20Recovered%20in%20New%20England~5-8-2015.pdf.
Millsap, B.A., Zimmerman, G.S., Sauer, J.R., Nielson, R.M., Otto, M., Bjerre, E., and Murphy, R., 2013, Golden eagle population trends in the western United States—1968–2010: The Journal of Wildlife Management, v. 77, no. 7, p. 1436–1448. [Also available at https://doi.org/10.1002/jwmg.588.]
Murphy, E.C., Clapperton, B.K., Bradfield, P.M.F., and Speed, H.J., 1998, Brodifacoum residues in target and non-target animals following large-scale poison operations in New Zealand podocarp-hardwood forests: New Zealand Journal of Zoology, v. 25, no. 4, p. 307–314. [Also available at https://doi.org/10.1080/03014223.1998.9518160.]
Murray, M., and Tseng, F., 2008, Diagnosis and treatment of secondary anticoagulant rodenticide toxicosis in a red-tailed hawk (Buteo jamaicensis): Journal of Avian Medicine and Surgery, v. 22, no. 1, p. 41–46. [Also available at https://doi.org/10.1647/2007-012R.1.]
Nakayama, S.M.M., Morita, A., Ikenaka, Y., Mizukawa, H., and Ishizuka, M., 2019, A review—Poisoning by anticoagulant rodenticides in non-target animals globally: The Journal of Veterinary Medical Science, v. 81, no. 2, p. 298–313. [Also available at https://doi.org/10.1292%2Fjvms.17-0717.]
Niedringhaus, K.D., Nemeth, N.M., Gibbs, S., Zimmerman, J., Shender, L., Slankard, K., Fenton, H., Charlie, B., Dalton, M.F., Elsmo, E.J., Poppenga, R., Millsap, B., and Ruder, M.G., 2021, Anticoagulant rodenticide exposure and toxicosis in bald eagles (Haliaeetus leucocephalus) and golden eagles (Aquila chrysaetos) in the United States: PLoS One, v. 16, no. 4, p. e0246134, accessed December 2022 at https://doi.org/10.1371/journal.pone.0246134.
Nriagu, J.O., 1989, A global assessment of natural sources of atmospheric trace metals: Nature, v. 338, no. 6210, p. 47–49. [Also available at https://doi.org/10.1038/338047a0.]
Pain, D.J., Fisher, I.J., and Thomas, V.G., 2009, A global update of lead poisoning in terrestrial birds from ammunition sources, in Watson, R.T., Fuller, M., Pokras, M.A., and Hunt, G., eds., Ingestion of lead from spent ammunition—Implications for Wildlife and Humans Conference, Boise, Idaho, May 12–15, 2008, Proceedings: Boise, Idaho, The Peregrine Fund, p. 99–118. [Also available at https://doi.org/10.4080/ilsa.2009.0108.]
Pain, D.J., Mateo, R., and Green, R.E., 2019, Effects of lead from ammunition on birds and other wildlife—A review and update: Ambio, v. 48, no. 9, p. 935–953. [Also available at https://doi.org/10.1007/s13280-019-01159-0.]
Parish, C.N., Hunt, W.G., Feltes, E., Sieg, R., and Orr, K., 2009, Lead exposure among a reintroduced population of California Condors in northern Arizona and southern Utah, in Watson, R.T., Fuller, M., Pokras, M., and Hunt, W.G., eds., Ingestion of lead from spent ammunition—Implications for Wildlife and Humans Conference, Boise, Idaho, The Peregrine Fund: Boise, Idaho, The Peregrine Fund, p. 259–264. [Also available at https://doi.org/10.4080/ilsa.2009.0217.]
Pattee, O.H., Wiemeyer, S.N., Mulhern, B.M., Sileo, L., and Carpenter, J.W., 1981, Experimental lead-shot poisoning in bald eagles: The Journal of Wildlife Management, v. 45, no. 3, p. 806–810. [Also available at https://doi.org/10.2307/3808728.]
Pauli, J.N., and Buskirk, S.W., 2007, Recreational shooting of prairie dogs—A portal for lead entering wildlife food chains: The Journal of Wildlife Management, v. 71, no. 1, p. 103–108. [Also available at https://doi.org/10.2193/2005-620.]
Plummer, M., 2018, Rjags—Bayesian graphical models using MCMC (ver. 4–8): R Foundation for Statistical Computing software release, accessed December 2022 at https://CRAN.R-project.org/package=rjags.
R Core Team, 2018, R—A language and environment for statistical computing: R Foundation for Statistical Computing web page, accessed December 2022 at https://www.r-project.org/.
Rattner, B.A., and Harvey, J.J., 2021, Challenges in the interpretation of anticoagulant rodenticide residues and toxicity in predatory and scavenging birds: Pest Management Science, v. 77, no. 2, p. 604–610. [Also available at https://doi.org/10.1002/ps.6137.]
Rattner, B.A., Horak, K.E., Warner, S.E., Day, D.D., and Johnston, J.J., 2010, Comparative toxicity of diphacinone to northern bobwhite (Colinus virginianus) and American kestrels (Falco sparverius), in Vertebrate Pest Conference, 24th, Sacramento, Calif., February 2225, 2010, Proceedings: Sacramento, Calif., Wildlife Damage Management, p. 145–152. [Also available at https://doi.org/10.5070/V424110380.]
Rattner, B.A., Horak, K.E., Warner, S.E., Day, D.D., Meteyer, C.U., Volker, S.F., Eisemann, J.D., and Johnston, J.J., 2011, Acute toxicity, histopathology, and coagulopathy in American kestrels (Falco sparverius) following administration of the rodenticide diphacinone: Environmental Toxicology and Chemistry, v. 30, no. 5, p. 1213–1222. [Also available at https://doi.org/10.1002/etc.490.]
Rattner, B.A., Horak, K.E., Lazarus, R.S., Eisenreich, K.M., Meteyer, C.U., Volker, S.F., Campton, C.M., Eisemann, J.D., and Johnston, J.J., 2012, Assessment of toxicity and potential risk of the anticoagulant rodenticide diphacinone using Eastern screech-owls (Megascops asio): Ecotoxicology (London, England), v. 21, no. 3, p. 832–846. [Also available at https://doi.org/10.1007/s10646-011-0844-5.]
Rattner, B.A., and Mastrota, F.N., 2018, Anticoagulant rodenticide toxicity to non-target wildlife under controlled exposure conditions, in van den Bring, N.W., Elliott, J.E., Shore, R.F., and Rattner, B.A., eds., Anticoagulant rodenticides and wildlife: Cham, Switzerland, Springer International Publishing AG, Emerging Topics in Ecotoxicology series, v. 5, p. 45–86. [Also available at https://doi.org/10.1007/978-3-319-64377-9_3.]
Russell, R.E., and Franson, J.C., 2014, Causes of mortality in eagles submitted to the National Wildlife Health Center 1975–2013: Wildlife Society Bulletin, v. 38, no. 4, p. 697–704. [Also available at https://doi.org/10.1002/wsb.469.]
Rutkiewicz, J., Nam, D.-H., Cooley, T., Neumann, K., Padilla, I.B., Route, W., Strom, S., and Basu, N., 2011, Mercury exposure and neurochemical impacts in bald eagles across several Great Lakes states: Ecotoxicology (London, England), v. 20, no. 7, p. 1669–1676. [Also available at https://doi.org/10.1007/s10646-011-0730-1.]
Savarie, P.J., Hayes, D.J., McBride, R.T., and Roberts, J.D., 1979, Efficacy and safety of diphacinone as a predacide, inKenaga, E.E., ed., Avian and mammalian wildlife toxicology: Philadelphia, Pa., American Society for Testing Materials, p. 69–79. [Also available at https://doi.org/10.1520/STP35992S.]
Scheuhammer, A.M., and Norris, S.L., 1996, The ecotoxicology of lead shot and lead fishing weights: Ecotoxicology (London, England), v. 5, no. 5, p. 279–295. [Also available at https://doi.org/10.1007/BF00119051.]
Scheuhammer, A.M., Basu, N., Burgess, N.M., Elliott, J.E., Campbell, G.D., Wayland, M., Champoux, L., and Rodrigue, J., 2008, Relationships among mercury, selenium, and neurochemical parameters in common loons (Gavia immer) and bald eagles (Haliaeetus leucocephalus): Ecotoxicology (London, England), v. 17, no. 2, p. 93–101. [Also available at https://doi.org/10.1007/s10646-007-0170-0.]
Shore, R.F., Malcolm, H.M., Weinberg, C.L., Turk, A., Horne, J.A., Dale, L., Wyllie, I., and Newton, I., 2002, Wildlife and pollution—1999/2000 annual report: Joint Nature Conservation Committee report no. 321, 20 p., accessed December 2022 at https://pbms.ceh.ac.uk/sites/default/files/Wildlife_annual_report_02.pdf.
Shore, R.F., Pereira, M.G., Walker, L.A., and Thompson, D.R., 2011, Mercury in nonmarine birds and mammals, inBeyer, W.N., Heinz, G.H., and Redmon-Norwood, A.W., eds., Environmental contaminants in wildlife—Interpreting tissue concentrations (2d ed.): Boca Raton, Fla., Lewis Publishers, p. 609–626. [Also available at https://doi.org/10.1201/b10598-19.]
Slabe, V.A., Anderson, J.T., Millsap, B.A., Cooper, J.L., Harmata, A.R., Restani, M., Crandall, R.H., Bodenstein, B., Bloom, P.H., Booms, T.L., Buchweitz, J., Culver, R.C.E., Dickerson, K., Domenech, R., Dominguez-Villegas, E., Driscoll, D., Smith, B.W., Lockhart, M.J., McRuer, D., Miller, T.A., Ortiz, P.A., Rogers, K., Schwarz, M., Turley, N., Woodbridge, B., Finkelstein, M.E., Triana, C.A., DeSorbo, C.R., and Katzner, T.E., 2022, Demographic implications of lead poisoning for eagles across North America: Science, v. 375, no. 6582, p. 779–782. [Also available at https://doi.org/10.1126/science.abj3068.]
Stauber, E., Finch, N., Talcott, P.A., and Gay, J.M., 2010, Lead poisoning of bald (Haliaeetus leucocephalus) and golden (Aquila chrysaetos) eagles in the US inland Pacific northwest region—An 18-year retrospective study—1991–2008: Journal of Avian Medicine and Surgery, v. 24, no. 4, p. 279–287. [Also available at https://doi.org/10.1647/2009-006.1.]
Streets, D.G., Zhang, Q., and Wu, Y., 2009, Projections of global mercury emissions in 2050: Environmental Science & Technology, v. 43, no. 8, p. 2983–2988. [Also available at https://doi.org/10.1021/es802474j.]
Thomas, P.J., Mineau, P., Shore, R.F., Champoux, L., Martin, P.A., Wilson, L.K., Fitzgerald, G., and Elliott, J.E., 2011, Second generation anticoagulant rodenticides in predatory birds—Probabilistic characterisation of toxic liver concentrations and implications for predatory bird populations in Canada: Environment International, v. 37, no. 5, p. 914–920. [Also available at https://doi.org/10.1016/j.envint.2011.03.010.]
U.S. Environmental Protection Agency [EPA], 2001, Water quality criterion for the protection of human health—Methylmercury—Final: U.S. Environmental Protection Agency Office of Water report EPA–R–01–001, 308 p., accessed December 2022 at https://nepis.epa.gov/Exe/ZyPDF.cgi/20003UU4.PDF?Dockey=20003UU4.PDF.
U.S. Environmental Protection Agency [EPA], 2007, [Difenacoum] pesticide fact sheet: U.S. Environmental Protection Agency, Office of Prevention, Pesticide and Toxic Substance fact sheet, 34 p., accessed December 2022 at https://www3.epa.gov/pesticides/chem_search/reg_actions/registration/fs_PC-011901_01-Sep-07.pdf.
U.S. Environmental Protection Agency [EPA], 2016, In the matter of—Mr. David Meyer, Flasher, North Dakota, respondent—Proceedings under section 7003 of the Solid Waste Disposal Act, as amended, 42 U.S.C. § 6901 et seq., 42 U.S.C. § 6973: U.S. Environmental Protection Agency Administrative Order, Region 8, docket no. RCRA–8–2016–0003, 19 p., accessed December 2022 at https://yosemite.epa.gov/OA/rhc/EPAAdmin.nsf/Filings/C1E7625EAD6A09A485257FA1001BC0C1/$File/RCRA-08-2016-0003%20AO.pdf.
U.S. Fish and Wildlife Service [USFWS], 1986, Migratory bird hunting—Nontoxic shot approval procedures: Federal Register, v. 51, no. 225, p. 42098–42107, accessed December 2022 athttps://archives.federalregister.gov/issue_slice/1986/11/21/42098-42107.pdf.
U.S. Fish and Wildlife Service [USFWS], 2013, California condor (Gymnogyps californianus) 5-year review—Summary and evaluation: U.S. Fish and Wildlife Service, 64 p., accessed December 2022 at https://biologistshandbook.com/wp-content/uploads/2018/01/5-Year-Review-2013.pdf.
U.S. Fish and Wildlife Service [USFWS], 2016a, Bald and golden eagles—Population demographics and estimation of sustainable take in the United States, 2016 update: U.S. Fish and Wildlife Service, 115 p., accessed December 2022 at https://www.fws.gov/sites/default/files/documents/bald-and-golden-eagles-status-report-and-sustainable-take.2016.pdf.
Vyas, N.B., 2013, Untested pesticide mitigation requirements—Ecological, agricultural, and legal implications: The Drake Journal of Agricultural Law, v. 18, no. 2, p. 335–348. [Also available at https://aglawjournal.wp.drake.edu/wp-content/uploads/sites/66/2016/09/agVol18No2-Vyas.pdf.]
Vyas, N.B., Kuncir, F., and Clinton, C.C., 2017, Influence of poisoned prey on foraging behavior of ferruginous hawks: American Midland Naturalist, v. 177, no. 1, p. 75–83. [Also available at https://www.jstor.org/stable/44840302.]
Walker, L.A., Shore, R.F., Turk, A., Pereira, M.G., and Best, J., 2008, The predatory bird monitoring scheme—Identifying chemical risks to top predators in Britain: Ambio, v. 37, no. 6, p. 466–471. [Also available at https://doi.org/10.1579/0044-7447(2008)37[469:TPBMSI]2.0.CO;2.]
Warner, S.E., Britton, E.E., Becker, D.N., and Coffey, M.J., 2014, Bald eagle lead exposure in the Upper Midwest: Journal of Fish and Wildlife Management, v. 5, no. 2, p. 208–216. [Also available at https://doi.org/10.3996/032013-JFWM-029.]
Wayland, M., and Bollinger, T., 1999, Lead exposure and poisoning in bald eagles and golden eagles in the Canadian prairie provinces: Environmental Pollution, v. 104, no. 3, p. 341–350. [Also available at https://doi.org/10.1016/S0269-7491(98)00201-2.]
Weech, S.A., Wilson, L.K., Langelier, K.M., and Elliott, J.E., 2003, Mercury residues in livers of bald eagles (Haliaeetus leucocephalus) found dead or dying in British Columbia, Canada (1987–1994): Archives of Environmental Contamination and Toxicology, v. 45, no. 4, p. 562–569. [Also available at https://doi.org/10.1007/s00244-003-0237-8.]
Wood, P.B., White, J.H., Steffer, A., Wood, J.M., Facemire, C.F., and Percival, H.F., 1996, Mercury concentrations in tissues of Florida bald eagles: The Journal of Wildlife Management, v. 60, no. 1, p. 178–185. [Also available at https://doi.org/10.2307/3802053.]
Conversion Factors
International System of Units to U.S. customary units
Temperature in degrees Celsius (°C) may be converted to degrees Fahrenheit (°F) as follows:
°F=(1.8×°C)+32.
Supplemental Information
Concentrations of chemical constituents in solid materials (tissues) are given in milligrams per kilogram (mg/kg), micrograms per gram (μg/g), or nanograms per kilogram (ng/kg) wet weight.
Abbreviations
AR
anticoagulant rodenticide
dw
dry weight
COD
cause of death
EPA
U.S. Environmental Protection Agency
FGAR
first generation anticoagulant rodenticide
FL
fluorescence
HPLC
high performance liquid chromatography
LOD
limit of detection
MeHg
methylmercury
MSU
Michigan State University
NWHC
National Wildlife Health Center
PDA
photodiode array
SGAR
second generation anticoagulant rodenticides
SRM
standard reference material
USFWS
U.S. Fish and Wildlife Service
ww
wet weight
For more information about this publication, contact:
Director, USGS National Wildlife Health Center
6006 Schroeder Road
Madison, WI 53711
608–270–2400
For additional information, visit: https://www.usgs.gov/centers/nwhc
Publishing support provided by the Rolla Publishing Service Center
Disclaimers
The findings and conclusions in this report are those of the authors and do not necessarily represent the views of the U.S. Fish and Wildlife Service.
Any use of trade, firm, or product names is for descriptive purposes only and does not imply endorsement by the U.S. Government.
Although this information product, for the most part, is in the public domain, it also may contain copyrighted materials as noted in the text. Permission to reproduce copyrighted items must be secured from the copyright owner.
Suggested Citation
Bodenstein, B.L., Lankton, J.S., Russell, R.E., and Schwarz, M.S., 2023, Haliaeetus leucocephalus (bald eagle) and Aquila chrysaetos (golden eagle) mortality and exposure to lead, mercury, and anticoagulant rodenticides in eight western and midwestern States, 2014–17: U.S. Geological Survey Open-File Report 2023–1016, 23 p., https://doi.org/10.3133/ofr20231016.
ISSN: 2331-1258 (online)
Study Area
Publication type | Report |
---|---|
Publication Subtype | USGS Numbered Series |
Title | Haliaeetus leucocephalus (bald eagle) and Aquila chrysaetos (golden eagle) mortality and exposure to lead, mercury, and anticoagulant rodenticides in eight western and midwestern States, 2014–17 |
Series title | Open-File Report |
Series number | 2023-1016 |
DOI | 10.3133/ofr20231016 |
Year Published | 2023 |
Language | English |
Publisher | U.S. Geological Survey |
Publisher location | Reston, VA |
Contributing office(s) | National Wildlife Health Center |
Description | Report: vii, 23 p.; Data Release |
State | Colorado, Kansas, Montana, Nebraska, North Dakota, South Dakota, Utah, Wyoming |
Online Only (Y/N) | Y |
Google Analytic Metrics | Metrics page |