Forest Structure and Residual Tree Growth at the Northwest Gateway Project Area, Lassen Volcanic National Park, California
Links
- Document: Report (10 MB pdf) , HTML , XML
- Download citation as: RIS | Dublin Core
Acknowledgments
We thank the numerous field crews who collected field data during many years. Kelly Muth completed the laboratory work for cross-dating tree core samples. Nancy Nordensten, Rosemary Sherriff, and Lisa Rosenthal provided insightful comments on early versions of this report. This project was supported by the National Park Service and the U.S. Geological Survey’s Ecosystems Mission Area Western Ecological Research Center.
Abstract
Mechanical thinning and prescribed fire are common mitigation treatments to reduce fire hazards. However, these treatments are infrequently applied together within national parks. The Northwest Gateway project at Lassen Volcanic National Park is an exception to this pattern. Various thinning prescriptions were applied to the project area in 2014, with a subset of the area prescribed burned in 2018 and 2019. To determine responses to these treatments, we analyzed forest structure and fuels data across a network of long-term monitoring plots measured before treatments and in multiple years following treatments. Additionally, we assessed patterns in individual tree growth from cores taken from 101 individual yellow pines (ponderosa pine, Pinus ponderosa Douglas ex Lawson & C. Lawson, and Jeffery pine, P. jeffreyi Grev. & Balf.) within the project unit.
Basal area and stem density were reduced following thinning treatments for pole-sized (≤15-centimeter diameter at base height) and overstory trees (>15-centimeter diameter at base height), with sharper reductions in pole-sized trees. Proportional change in live basal area after thinning was highest for pole-size Abies, with more than 80-percent basal area and stem density removed on average. There were large reductions in pole-sized Pinus and Populus. However, Populus trees were not targeted for removal, suggesting that these trees died via other mechanisms. Thinning treatments also resulted in reductions in stand density index values and in surface fuel loading when followed by prescribed fire, particularly for small fuels size classes (such as litter/duff, 1-hour, and 10-hour fuels). Growth of individual residual yellow pine, measured in terms of annual basal area increment, indicated a strong growth release in the years following thinning treatments.
Taken together, these results indicate that forest restoration treatments at the Northwest Gateway project area were effective at reducing stand density and encouraging growth of residual Pinus. Interestingly, our results also indicated that although thinning followed by prescribed fire was most effective at reducing surface fuel loads, harvest techniques such as whole tree yarding may effectively reduce the accumulation of post-treatment residual fuels, especially when combined with hand piling and other targeted treatments.
Introduction
Following more than a century of fire exclusion in western coniferous forests in the U.S., land managers now expend substantial resources reducing fire hazards using mechanical thinning and prescribed fire (Schwilk and others, 2009; Ryan and others, 2013). Mechanical thinning and prescribed fire treatments are frequently applied sequentially to the same stand, with evidence that mechanical thinning alone is often less effective than combined treatments at reducing canopy and surface fuels that partially determine future fire behavior (Raymond and Peterson, 2005; Stephens and Moghaddas, 2005; Vaillant and others, 2009; Stephens and others, 2012; Lydersen and others, 2017). Regardless, the effectiveness of these treatments to reduce fire hazards declines over time, with the rate of decline varying with forest type (Chiono and others, 2012).
Despite the widespread use of mechanical thinning and prescribed fire, these treatments are rarely applied in old growth forests. Understanding the effects of treatments such as mechanical thinning and prescribed fire on old growth forests is critical because of their ecological and cultural importance (Abella and others, 2007 and references therein). Old growth is relatively scarce on the landscape and is often present in protected areas (such as parks and wilderness areas) where there may be fewer opportunities for direct management. In these situations, thinning treatments typically are reserved for areas with extensive infrastructure or other sites where even low risk of accidental fire escape is unacceptable. Because of the relative rarity of treatments in these areas, less is known about the effects of treatments in old growth stands, although some examples exist. Maloney and others (2008) reported that mechanical thinning and prescribed fire may increase the prevalence of bark-beetle mortality in old growth pine in the Sierra Nevada, at least in the short term. Other researchers have documented that thinning was most effective at reducing surface fuels in old growth when followed by prescribed fire (Innes and others, 2006). Cansler and others (2019) reported that fire can effectively reduce surface fuels in old growth, though their project area was burned during firefighting operations and the results may not be representative of prescribed fire.
Mechanical thinning and prescribed fire treatments can affect the growth of residual trees. Mechanical thinning typically increases the availability of light, water, and other resources, leading to increased growth in residual trees (Feeney and others, 1998), though subsequent prescribed burning may affect these conditions (Fajardo and others, 2007; Knapp and others, 2021, Zald and others, 2022). Fire may injure live trees following thinning (Perrakis and others, 2011; Hood and others, 2018), but these trees also may experience more open growing conditions following fire, which could lead to improved growth over time (Westlind and Kerns, 2021). Treatments also may create stand conditions that allow residual trees to better respond to climatic stress, such as increased drought resistance (Vernon and others, 2018; Low and others, 2021) and recovery (Sohn and others, 2016).
In this study, we use extensive forest plot inventories supplemented with tree-ring data to describe the effects of thinning treatments and prescribed fire on stand structure, surface fuel load, and residual tree growth in an old growth forest known locally as the Northwest Gateway (NWG) project in Lassen Volcanic National Park (LVNP). This study has three primary objectives:
-
1. Quantify the change in stand structure following the thinning treatment relative to the untreated areas, including differences at the species level. We expected that thinning and prescribed burning treatments would reduce stand density index (SDI), stand basal area (cumulative cross-sectional area of live stems), and stem density (number of trees per unit area), particularly for small trees.
-
2. Quantify the effects of thinning and prescribed fire treatments on surface fuel loading. We expected that surface fuel loads would increase immediately after the thinning treatment because of the creation of activity fuels (slash) and the redistribution of canopy fuels to the ground. We expected this increase to mainly be in fine fuels because thinning prescriptions (see “Treatments”) called for larger fuels to be removed by a combination of the harvesting methods and post-thin, pre-burn fuel treatments (such as hand piling). We expected that surface fuel loads would decrease following prescribed fire treatments because of the consumption of fine fuels.
-
3. Quantify the effects of thinning on the radial growth of residual pine trees (an index of tree vigor). We expected to see a growth release following treatment for trees in treated areas, with a smaller growth release for trees in the thin and burn treatment combination. Additionally, we expected the magnitude of the growth release to be dependent on local competition and annual variation in climate.
Methods
Study Area
The study was carried out in a mixed conifer forest within the NWG of LVNP, in a 540-acre area near Manzanita Lake in the northwest corner of the park (fig. 1). The project area surrounds high value National Park Service infrastructure. Historically, the project area is expected to have had a mean fire return interval of approximately 32 years (Taylor, 2000; Skinner, 2009). No major wildfires have burned in the project area since 1918 (Skinner, 2009). In the absence of frequent fires, surface fuel loads and tree densities have increased, favoring small, shade tolerant white fir (Abies concolor [Gordon & Glend.] Lindl. ex Hildebr.). Insect and disease mortality also has become increasingly common. Aspen (Populus tremuloides Michx.) was historically abundant in the project area, though quite rare today. The goals of the Northwest Gateway project included (1) reducing surface and canopy fuels, (2) restoring the aspen population, and (3) maintaining desired fire regimes. Common species in the project area include white fir, ponderosa pine, Jeffery pine, with incense cedar (Calocedrus decurrens [Torr.] Florin), and sugar pine (Pinus lambertiana Douglas) intermixed.
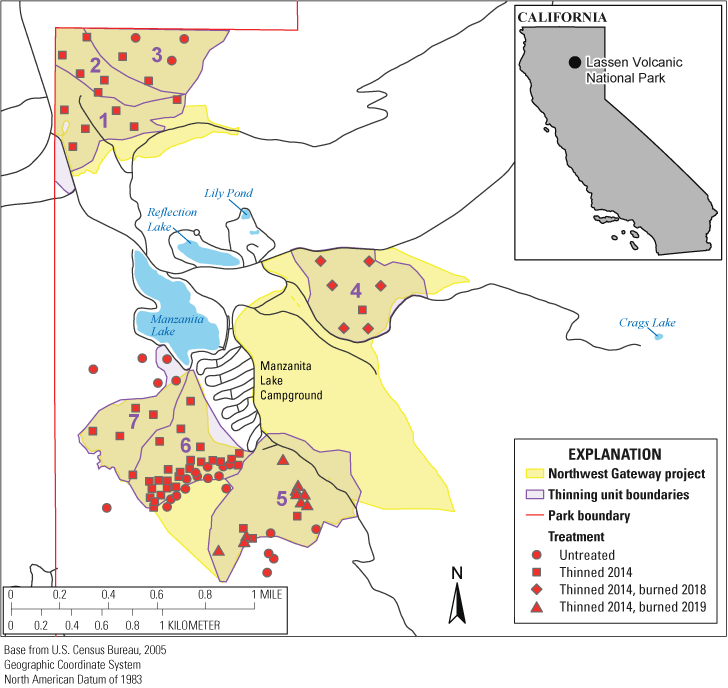
Northwest Gateway study area. Numbers represent treatment units, and points represent study plot locations, with shapes indicating treatment history. Note that project and thinning unit boundaries are approximate.
Treatments
Mechanical thinning treatments were carried out between January and April of 2014 (fig. 2). A combination of thinning prescriptions was applied throughout the project area. Whole tree yarding was used for tree removal in all prescriptions. Although the overall treated area is known, the boundaries of the specific treatment types within each unit were not recorded. Mechanical treatments were usually followed by hand piling of fuels. The hand piled fuels were burned during the winter of 2014–16 as conditions permitted (fig. 3). The mechanical thinning prescriptions are described in table 1.
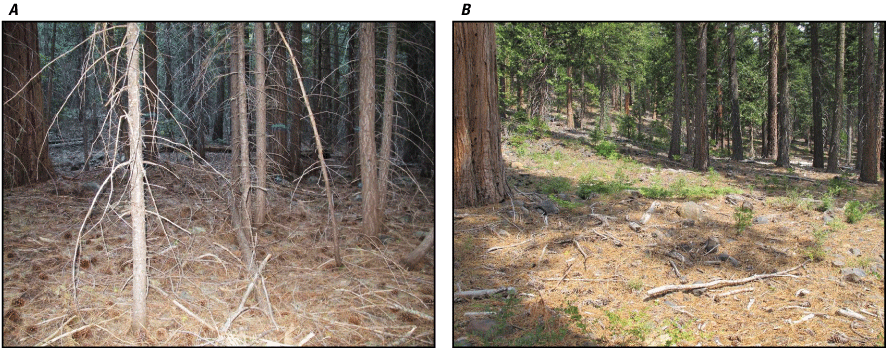
A, Forest conditions before treatment in 2012 and B, following treatment in 2020 at a representative site in the Northwest Gateway Project area (Unit 1, plot 102, east view). Photographs by Calvin Farris, National Park Service.
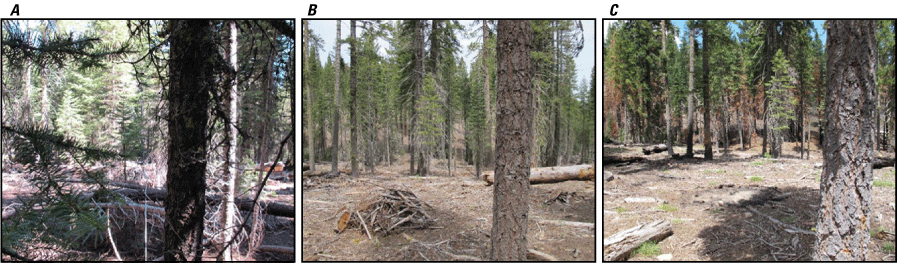
A, Forest conditions before treatment in 2012; B, following hand piling of slash fuels in 2015; and C, following burning of hand piles and broadcast prescribed burning in 2020 at a representative site in the Northwest Gateway Project area (Unit 5, plot 519, north view). Photographs by Calvin Farris, National Park Service.
Table 1.
Thinning treatment descriptions at the Northwest Gateway study area, Lassen Volcanic National Park.[m, meter; >, greater than; cm, centimeter, DBH, diameter at breast height]
The thinning prescriptions were applied across the study area using a designation by description approach (that is, conditional based on field reconnaissance; fig. 1). A subset of the thinned area was subsequently treated with prescribed broadcast burning, separate from the pile burns (Unit 4 in 2018 and parts of Unit 5 in 2019; see fig. 1). Different parts of the project area were burned in 2018 and 2019. Note that the thinning treatments in Unit 5 were only partially completed because of time constraints.
Field Data Collection
Stand Structure and Surface Fuels
Our dataset consists of a network of 90 long-term plots that were either thinned (n=47), thinned and broadcast burned (n=16), or untreated (n=27). All plots were established by the National Park Service (NPS) before the thinning and prescribed burn treatments. We measured trees and fuels in each plot before and after each thinning and prescribed burning treatment using one of five protocols, described above (table 2).
Table 2.
Plot types with treatments and sample dates.[CSE, common stand exam; FMH, Fire Monitoring Handbook]
-
• Rapid Assessment Fire Monitoring Handbook (FMH) Plots. These plots were randomly located 5×30 meters (m; 0.015 hectares [ha]) fixed area plots. Fuel loading, vegetation cover, and stand structure were sampled using standard FMH protocols, (National Park Service, 2003). A total of 15 plots were sampled: 5 untreated, 8 thinned, and 2 thinned and burned. Plots were sampled in 2005, 2006, 2011, 2014, 2015, 2016, 2017, and 2020.
-
• Aspen plots. These consisted of fixed 0.006-ha plots randomly located through various aspen groves in the project area. These plots consisted of a single 2- × 30-m fixed-area belt transect. A total of 38 plots were sampled: 16 untreated, 20 thinned, and 2 thinned and burned. Plots were sampled in 2012, 2014, 2016, 2017, and 2020.
-
• Common stand exam (CSE) plots. These plots used a point sampling approach and were systematically located on a Universal Transverse Mercator (UTM) grid. Basal area was measured using a standard wedge prism (basal area factor 40). A total of 32 plots were sampled: 6 untreated, 19 thinned, and 7 thinned and burned. Plots were sampled in 2012, 2015, 2017, 2018, 2019, and 2020.
-
• Meadow plots. These plots were randomly located 0.08-ha fixed area plots. Plot dimensions were 16×50 m. A total of five plots were sampled, all were thinned and burned. Plots were sampled in 2012, 2015, 2017, 2019, and 2020.
Stem diameter, species, and mortality status (live or dead) were recorded for all individual trees ≥2-centimeter (cm) diameter at base height (DBH; 1.37 m) in all fixed area plots (FMH, Aspen, and Meadow plots). Plot counts are given above. The variable radius common stand exam plots recorded live tree counts, tree species, and mortality status. At all plots, surface fuels were sampled with Brown’s fuels transects (Brown, 1974), which are used to estimate fuel load dry weight by size class. Woody fuels size classes include 1-hour (up to 0.635-cm diameter), 10-hour fuels (0.635–2.54 cm), 100-hour fuels (2.54–7.62 cm), and 1,000-hour fuels (>7.62 cm; Rothermel, 1972). Forest floor fuels (duff and litter) were measured by depth. All fuels transects originated from a permanently marked location with a recorded azimuth to facilitate repeated measurements. The number of Brown’s transects in each plot varied by plot design. The aspen plots had a single transect, whereas the FMH plots had two taken along a central axis. The Meadow plots each had six Brown’s transects arrayed in series along the 50-m plot edge. All data for stand structure and surface fuels were stored using the FEAT-FIREMON Integrated (FFI) monitoring software (Lutes and others, 2009).
We standardized all metrics to unit area to facilitate comparisons among sample designs. We included plot measurements where they collected the same observations, including stem density (count of live trees per hectare), basal area (summed cross-sectional area of live stems per hectare) and surface fuels (kilograms per square meter [kg/m2]). There was limited information on the specific treatment attributes of each plot. Therefore, we did not differentiate between specific thinning treatment prescriptions or prescribed fire attributes, such as burn severity or proportion of the plot that burned.
Growth
Cores were collected from live yellow pine (ponderosa or Jeffrey pine) >15-cm DBH that were an average of 60 m from selected study plots. Trees more than 30-cm DBH were preferentially selected where possible, within and outside of the treatment units. Cores were taken perpendicular to the direction of the slope at approximately 50-cm height. Where possible, trees were cored to pith or to 50 years of growth. We cored a total of 101 trees in two separate sample years. The initial sample of 68 trees were cored in 2017, with an additional sample of 33 trees cored in 2020. Six trees from the 2020 sample and two trees from the 2017 sample could not be crossdated and were not used in the analysis. Two cores were taken from 62 trees in the 2017 sample; annual growth was estimated by averaging the annual growth measurements for these cores. We recorded attributes of each cored tree, including DBH and competitor basal area, which was measured using a wedge prism (Basal Area Factor of 20 or 30). In total, 77 trees were in treated areas, and 24 were in untreated areas.
Climate Data
There is a weather station near the study site at Manzanita Lake. However, the available raw data had missing values, so we used precipitation estimates from TerraClimate (Abatzoglou and others, 2018), a 4-kilometer (km) resolution dataset (https://www.climatologylab.org/terraclimate.html, accessed July 23, 2021) available at monthly timesteps. We downloaded TerraClimate precipitation values for the Manzanita Lake weather station location for all years since 1960. There was good agreement between the available weather station values and the TerraClimate values for the station location, with a Pearson’s correlation of more than 0.9.
We calculated annual precipitation totals for the water year (October–September) for the years 1980–2019. To account for potential lag effects, we calculated a running mean for the two previous years, which has been reported to affect tree growth in LVNP (Wenderott and others, 2022).
Data Processing
Stand Structure
We exported the individual tree records for the NWG from FFI (Lutes and others, 2009). We classified all trees with DBH ≤15 cm as poles and all others as overstory trees. We calculated the live stem density and basal area per hectare by plot, species, and size class. To assess thinning treatment effects on forest stand structure, we used the most recent pre-treatment and the earliest survey following thinning (but before the application of prescribed fire) for each plot and calculated differences in stand basal area and trees per hectare. We did not have reliable pre-treatment data for individual trees where sampling was completed using a wedge prism (CSE plots, n=32 plots), so we did not use these plots to describe changes in stem density and basal area. We considered changes in two size classes (pole and overstory trees) for major genera (Abies, Pinus, and Populus) for each plot. We calculated the plot-level average proportional change in live basal area and stem density by size class and genus in each plot. We also calculated the SDI for each plot, then compared average SDI for each treatment unit using observations from immediately before and after the thinning treatments. Changes in stand structure were prescriptive; therefore, we only describe the data and did not perform formal statistical analysis.
We quantified changes in live crown base height measurements from the CSE plots, which were the only plots where it was measured. Individual trees were not tracked in these plots, so we summarized the average values by sample year and treatment status (untreated, before thinning, after thinning, and after burning).
Surface Fuels
We exported the NWG fuels report from FFI, which provides estimates of mean plot fuel load in kilograms per hectare (kg/ha) for all fuel particle size classes using equations and fuels constants from Brown (1974). We removed any observations where the records for all fuel size classes summed to 0 for a given observation (n=37), assuming that these observations were recorded in error. We were interested in treatment-mediated changes in surface fuels. To assess changes in fuels associated with treatments, we extracted the most recent pre- and post-treatment reads (2011–12 and 2019–20, respectively). Note that for some plots, this included the effects of prescribed burning. We used these data to calculate the difference in fuel loads between these intervals for each surface fuel size class.
The post-treatment observations we used in the analysis were recorded between 5 and 6 years following treatments, potentially allowing fuel accumulation, especially in smaller size classes (van Wagtendonk and Moore, 2010). Our estimates of fuel loading could be obscured by the lag between treatment and measurement; that is, we may have missed the full reduction in surface fuels following treatment because of rapid accumulation of new fuels. However, we examined the first post-thinning observations in preliminary analysis, which produced broadly similar results. We believe it is unlikely that new fuel additions have substantially changed our results.
Growth
We processed the tree cores using standard sample preparation procedures (Speer, 2010). We glued cores to wooden mounts and sanded with progressively finer sandpaper until the ring boundaries were clearly visible. We measured ring width using a calibrated color flatbed scanner and WinDENDRO software (Regent Instruments Canada Inc.).
We calculated bark thickness for each cored tree using the following equation from Zeibig-Kichas and others (2016):
wherebark thickness
is bark thickness, in millimeters (mm), and
dch
is tree diameter at the height of coring, in centimeters.
Bark thickness, as a contribution to stem diameter, was multiplied by two to account for bark on both sides of the tree. We converted the raw ring widths to basal area increment (BAI, square centimeters [cm2]) using inside bark core height diameters with the dplR package in R (Bunn, 2008). BAI uses a circular cross section assumption to characterize growth as the area of wood produced per year. We initially removed any growth records after 2017 to keep our analysis to the same time frame for each tree. However, this left only two reliable growth years post treatment for all trees, so we kept the entire record (up to and including 2019) where possible. We were primarily interested in the response of annual growth to treatments; therefore, we only considered growth years after 1980 in the analysis.
Analysis
We performed statistical analysis on changes in surface fuels and growth of cored trees. We used a Bayesian approach for all analyses. We fit all models using the brms (Bürkner, 2018) package in the R statistical programming environment (version 4.0.4, R Core Team, 2022). We fit all models with four chains that ran for a minimum of 2,000 iterations each. We assessed model convergence with trace plots of the Markov chain Monte Carlo (MCMC) chains, and examined convergence with rank plots of MCMC chains for all parameters with the R-hat convergence diagnostic () above 1.01. Model fit was assessed using posterior predictive checks (Gabry and others, 2019).
Surface Fuels
We modeled the change in surface fuel for each size class as a function of treatment (untreated, thinned, and thinned and burned) and pre-treatment fuel load using linear models. We calculated the change in surface fuels as the difference between post and pre-treatment fuel loads in each plot. We assigned 2014 as the treatment year for the untreated and thin-only plots and the burn year as the treatment year for the combined thin and burn plots. Differences less than 0 represent a loss of surface fuel, whereas positive values represent an increase in surface fuel. Values of 0 indicate no change. We set untreated as the reference condition for the treatment effect in all models. We used weakly informative priors to estimate parameters, following the normal distribution with a mean of 0 and a variance of 5. To see how treatments differed from each other, we calculated pairwise contrasts of the posteriors for each treatment.
Growth
We used a hierarchical generalized linear model (gamma errors with a log link) to estimate the response of growth to treatment and precipitation. We regressed annual BAI against treatment status (treated versus untreated) and precipitation. We allowed the intercept to vary by tree. Because treatment status is binary, we standardized precipitation by subtracting the mean and dividing by two standard deviations, which puts parameters on a common scale (Gelman, 2008). Because of temporal autocorrelation between years, we included a first order autoregressive term. We specified weakly informative priors to estimate parameters, following the normal distribution with a mean of 0 and a variance of 5.
We used a generalized linear model to investigate the effects of residual competitors on growth. Competitor basal area was measured post-treatment, so we averaged post-treatment (post 2014) BAI values for each tree. We fit two generalized linear models (gamma errors with a log link), one with treatment alone, and another with treatment and competitor basal area as the predictors. We assessed the change in treatment effect when adding competitor basal area, assuming that a loss of importance of treatment indicated that the effect of treatment was driven by changes in neighborhood competition. Where applicable, we used the same weakly informative priors as in the hierarchical model.
Results
Stand Structure
Average basal area and stem density following treatments were lower than pre-treatment baselines for pole and overstory trees (figs. 4, 5). The notable exception was in overstory Populus, which had no change before and after thinning.
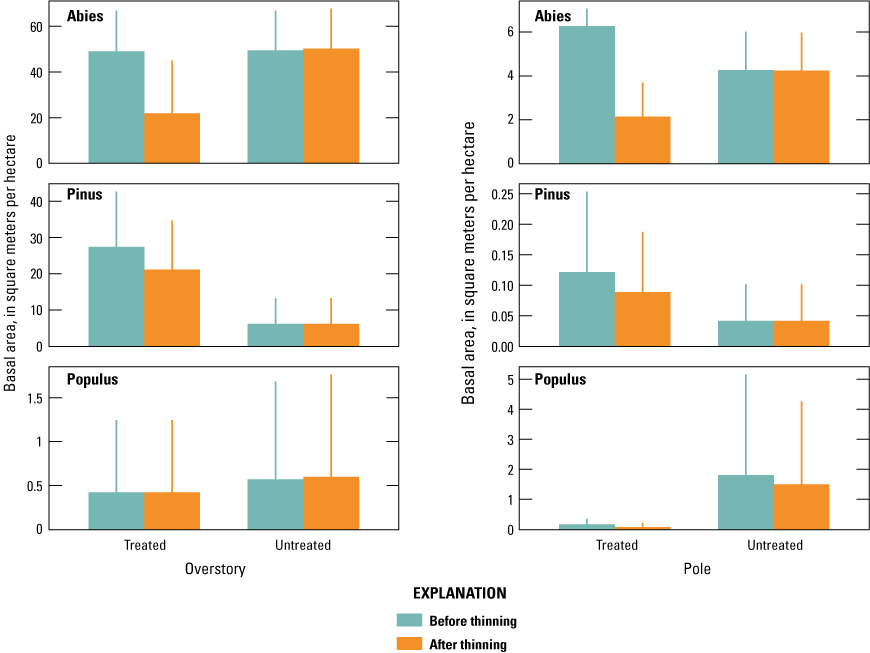
Average basal area (±1 standard error [SE]) for pre- and post-thinning reads by genus. Untreated plots were not thinned but measured during years comparable to the treated plots. The averages represent conditions before broadcast burning.
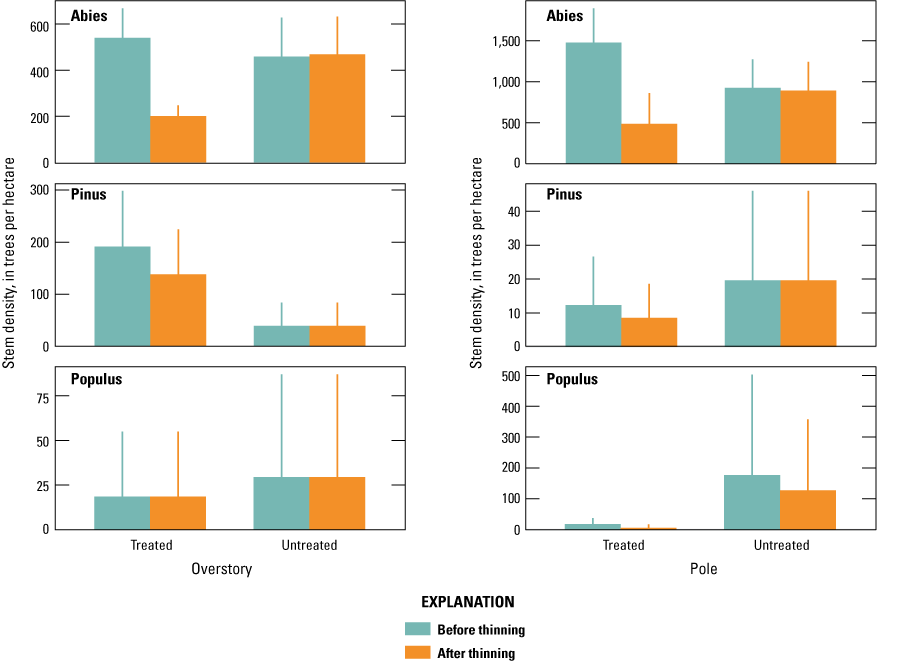
Average stem density (±1 SE) for pre- and post-thinning reads by genus. Untreated plots were not thinned but measured during years comparable to the treated plots. The averages represent conditions before broadcast burning.
Absolute and proportional change in live basal area after thinning was highest for pole-size Abies, with more than 80-percent basal area and stem density removed on average (figs. 4, 5). Overstory Abies basal area also saw substantial reductions averaging more than 60 percent (fig. 4). Pole-size Populus basal area was also reduced more than 60 percent on average. However, Populus trees were not targeted for removal, regardless of size, suggesting that these trees died via other mechanisms. This is consistent with observations of aspen mortality in the study area between 2006 and 2014. Compared to Abies, Pinus structural attributes changed much less on average after treatment. Pinus trees only were removed in areas of “radial release” for old growth legacy trees and in heavy infestations of mistletoe. Basal area was reduced 50 percent on average for pole-sized trees and 25 percent in overstory trees. Unsurprisingly, proportional basal area change was negligible for both size classes of all genera in the untreated plots, with average changes of less than 5 percent (fig. 6).
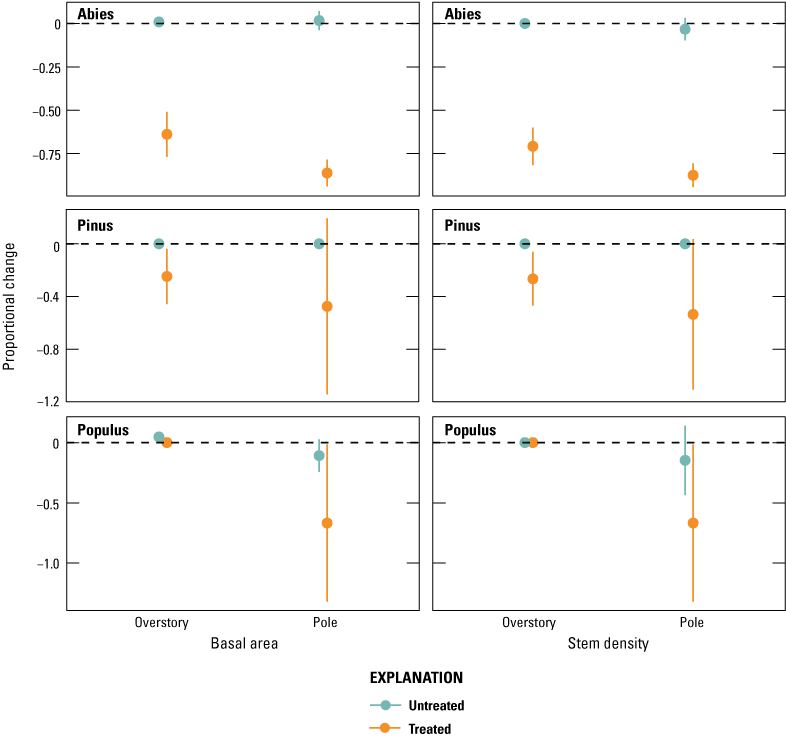
Average proportional change (points ±95-percent confidence intervals [CI]) for live basal area and stem density following treatment for treated and untreated plots by tree size class and genus. Confidence intervals were estimated using twice the standard error. The dashed horizontal line at 0 represents no change between pre- and post-treatment.
Average SDI and stem density was notably lower following thinning treatments in all treatment units (fig. 7). We did not observe notable changes in either average SDI or stem density in the untreated plots.
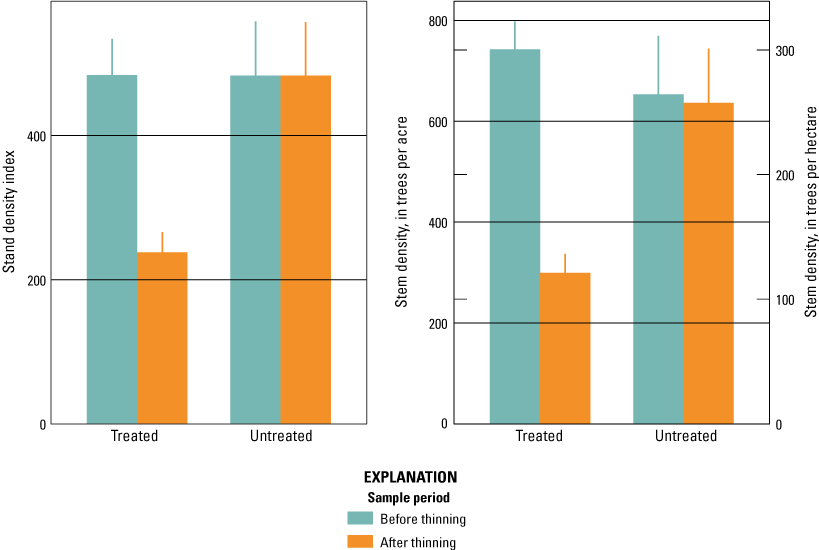
Average stand density index (SDI) and stem density (trees per acre, ±1 SE) for pre- and post-thinning reads.
Average live crown base height was lowest in the untreated plots (fig. 8). As expected, live crown base height increased following thinning and burning treatments, with the highest average values recorded following the burn treatments. Interestingly, live crown base height seemed to continue to increase over time following thinning treatments, even when no additional treatments were applied (data not shown).
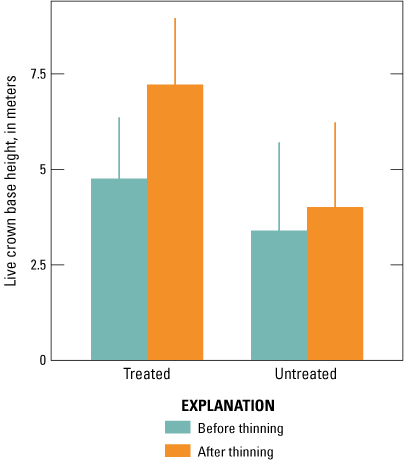
Average live crown base height (±1 SE) before and after thinning treatments.
Surface Fuels
Surface fuel loads were relatively unchanged between samples in untreated plots for most size classes, suggesting that fuels either remained stable or increased over time in the absence of treatment (fig. 9). There was little support for a consistent effect of thin-only treatments on fuel loads relative to the untreated reference condition (fig. 10). Model predictions indicated little observed change between treatment types, with nearly equal support for negative and positive values for all fuel size classes except for the one hour and litter/duff size classes. There was some support for decreased fuel loads following treatment relative to the untreated reference condition in the smaller size classes, though there was more uncertainty in the litter and duff class.
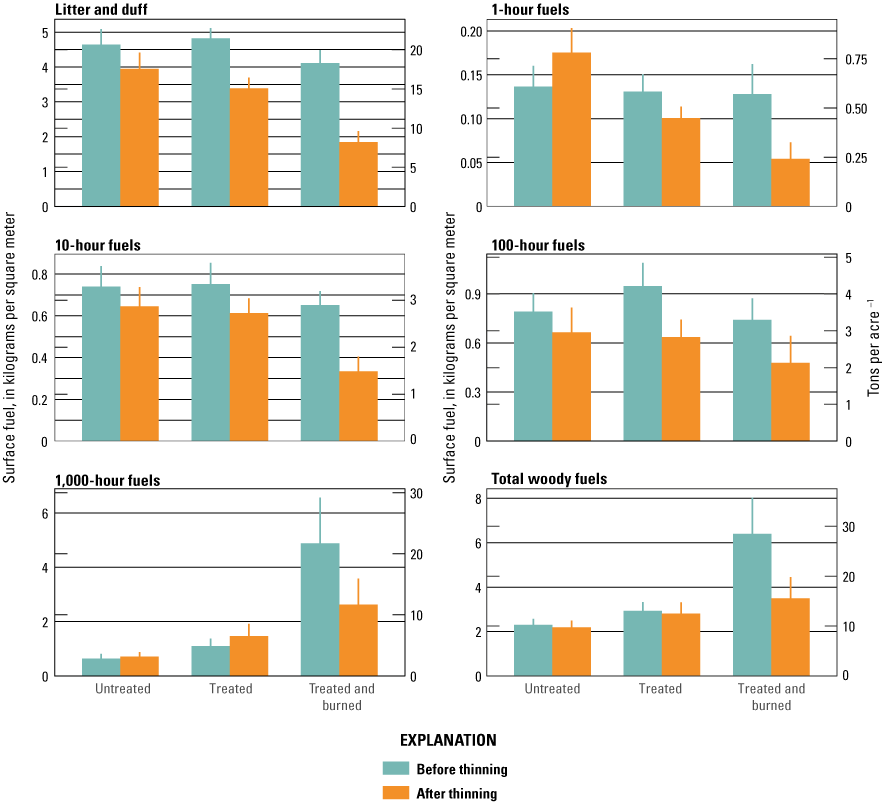
Mean fuel load (±1 SE) by size class for the last pre-treatment year and latest post treatment year. Pre- and post-treatment values for untreated plots were made from the sample year immediately preceding 2014 and the last sample year (range = 2019 to 2020). Note the varied x-axes scales.
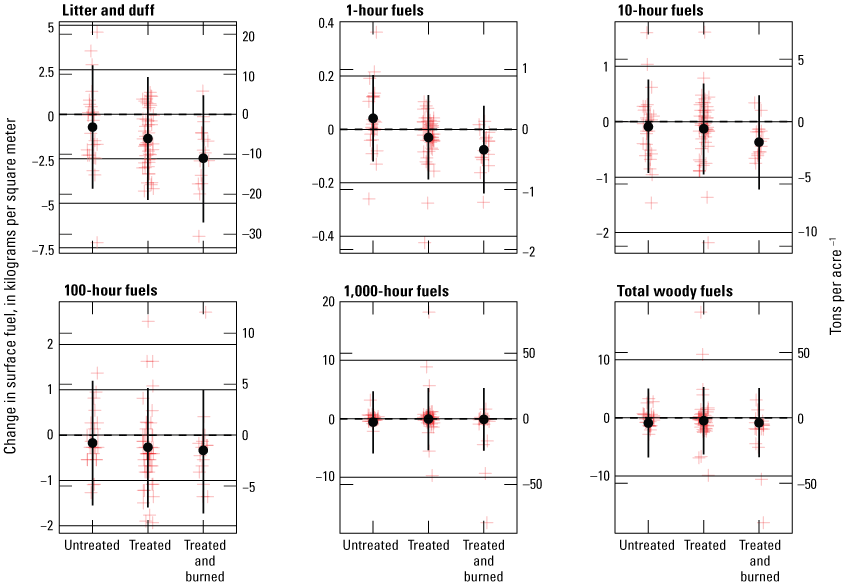
Median model predictions (points ±95-percent credible intervals) of the difference in fuel after treatment relative to pre-treatment loads for untreated and treated plots by fuel size class for the most recent year of observation. The red crosses are observed plot-level values. Positive differences can be interpreted as an increase in fuel load, whereas differences below zero indicate reduced fuel loads. The dashed horizontal line at 0 represents no change between pre- and post-treatment intervals. Note the varied y-axes scales.
In contrast, mechanical thinning followed by prescribed fire had a more consistent effect on subsequent fuel loading. Predicted post-treatment medians were below zero for all size classes except 1,000-hour and total woody fuels (fig. 10), with almost all support for a negative effect in the smaller size classes (litter/duff, 1-, and 10-hour fuels). Pairwise posterior contrasts (fig. 11) also indicated that there was fewer surface fuels following thin and burn treatments than thin-only treatments for the three smallest size classes. However, the models had much less support for a consistent effect in the other size classes, with posterior medians closer to zero and pairwise posterior contrasts suggesting considerable uncertainty in the direction of these effects. All models indicated that there is strong evidence that higher pre-treatment loads could result in large differences between pre- and post-treatment fuel mass, suggesting that areas with more initial fuels experienced a greater reduction in fuel load following treatment.
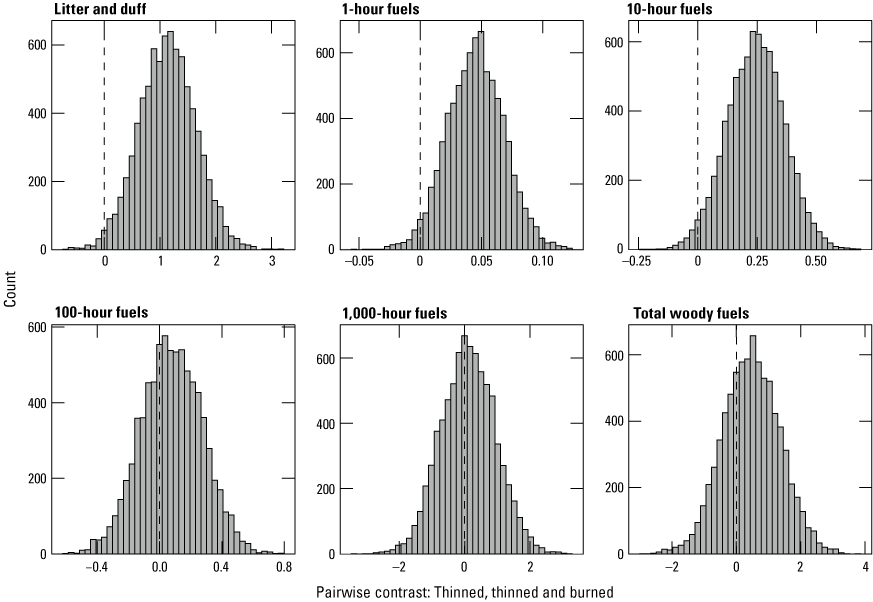
Pairwise posterior contrasts for the difference in fuels by treatment models. Contrasts show the posteriors for the estimated difference in means between thinned, and thinned and burned treatments. Values greater than 0 indicate fuel loads were higher after thinning treatments than combined thin and burn treatments. Subplots show the contrast for each fuel size class.
Growth
Plots of average BAI documented considerable growth differences in the post-treatment years for trees with and without thinning treatments (fig. 12). The model echoed these results, indicating good support for a positive effect of treatment on BAI (fig. 13). The model also had strong support for a positive effect of precipitation on growth, though it was weaker than the treatment effect.
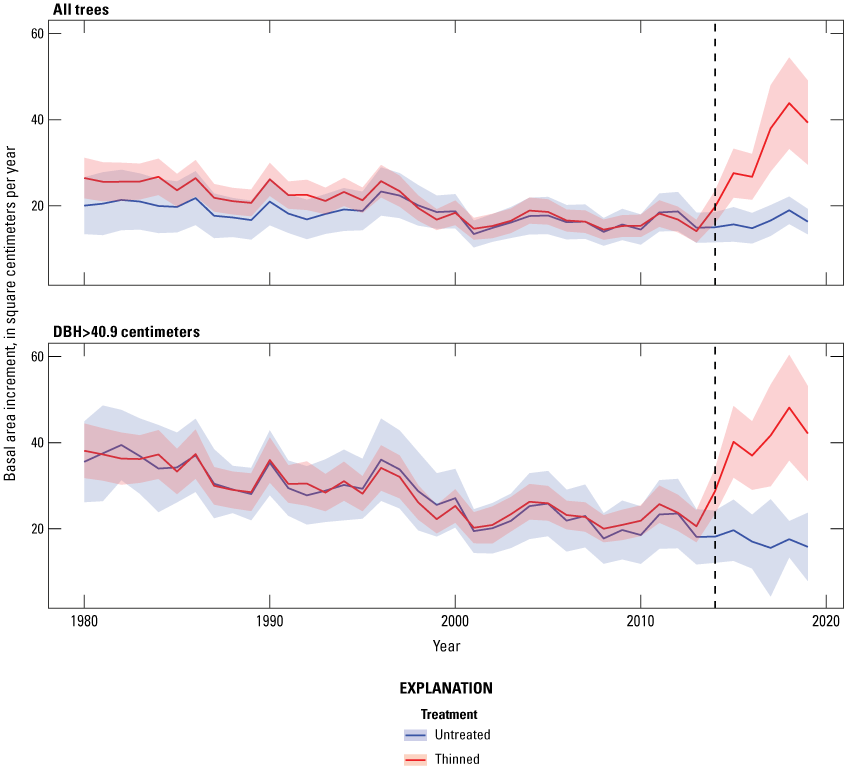
Average basal area increment (BAI; square centimeters per year [cm2/yr], ±95-percent confidence intervals) for treated and untreated Pinus trees from 1980 to 2019, for A, all trees and B, trees larger than the median diameter at breast height, 40.9-cm DBH. The treatment year is shown as a dotted vertical line.
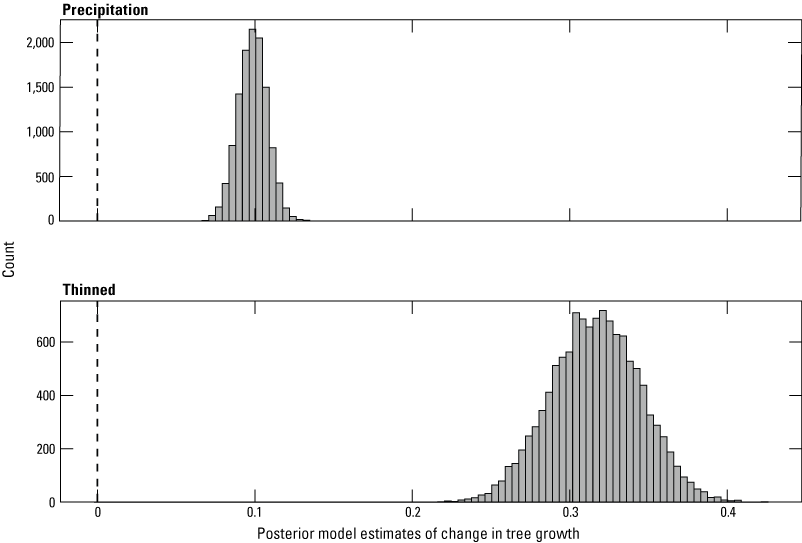
Histograms of the posteriors from the hierarchical tree growth model. Posteriors for the intercepts and autoregressive model term are not shown. The term ‘Thinned’ shows differences in thinned stands relative to unthinned stands.
Competition also seemed to affect growth. There was good support for a negative relationship between competitor basal area and average growth (fig. 14). Additionally, including competitor basal area in the model had a substantial moderating effect on the importance of the treatment variable, suggesting that the removal of competitors was largely responsible for the treatment effect.
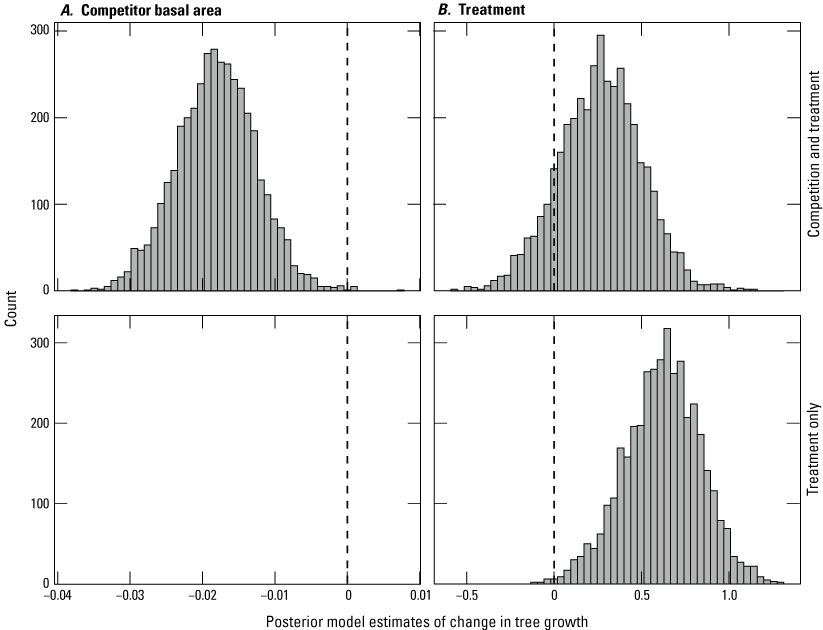
Histograms of the posteriors for the effect of A, competitor basal area; and B, treatment on average post-treatment BAI. Each row is a model; each column is a variable.
Conclusion
The Northwest Gateway treatments were largely successful in reducing stand-level stem density and basal area while having little measurable effects on surface fuel loading. However, there were indications that surface fuels were reduced from initial conditions where thinning was followed by prescribed fire. Combined with the reduction of canopy fuels and the positive growth response of residual trees following mechanical thinning, these results indicate that the restoration treatments successfully reduced fire hazards and may confer a greater resistance to future fire and drought for residual trees. However, actual outcomes during a fire will partly depend on other contingencies such as fire weather, suppression tactics and the timing of the fire relative to future maintenance treatments. This overall positive assessment is applicable to thinning treatments in general, and not specific thinning prescriptions. We did not have information regarding the specific thinning treatment that was applied to each plot, therefore we could not assess the effectiveness of specific prescription. These results are consistent with the intent of understanding the aggregate effects of all prescriptions across the study area rather than prescription-specific effects because each thinning prescription was developed to address different site-specific stand conditions.
Stand Structure
As expected, most of the changes in stand structure occurred in pole-size Abies, with more than 80 percent of the basal area removed for trees ≤15-cm DBH. This stands in stark contrast to the untreated plots, which indicated little change in basal area or stem density. The removal of small trees can reduce the available ladder fuels and raise the height to live crown, decreasing the probability of crown fire and subsequent mortality in larger trees (Stephens and Moghaddas, 2005). We observed increases in live crown base height following treatment where data was available.
The loss of small aspen following treatment was surprising, given that the preservation of aspen was a primary management goal of NWG. This is likely explained by two factors: (1) the degraded condition of remnant aspen within the high-density conifer stands that have overtopped them (losses have occurred continuously since monitoring began in 1998), and (2) the relatively old age of the last remaining aspen (which likely originated following a 1918 fire). Furthermore, an ice storm in 2006 weakened many trees by splitting and breaking trunks. However, the number of aspen in our sample was small to begin with (21 trees across all plots in the pre-treatment read), so the loss of only a few trees will create large proportional changes. Regardless, the small population size suggests that any loss may threaten the continued viability of the aspen population in the project area.
Surface Fuels
Though we expected some variability in the effect of treatment on surface fuels, we were surprised that mechanical thinning treatments seemed to produce limited changes in most surface fuel size classes in the absence of prescribed fire. Previous work has documented that mechanical treatments may increase surface fuel loads in the short term unless they are followed by prescribed fire (Raymond and Peterson, 2005). However, our results indicated little support for increasing fuels following mechanical thinning. The lack of a thinning effect on surface fuels may be a result of two independent mechanisms. First, trees were removed from the project area via whole tree yarding, which can be effective at limiting the amount of accumulated slash within the project units (Stephens and others, 2009). Second, there were subsequent mitigation measures applied after thinning, most notably the hand piling and burning of heavier pockets of residual fuels (fig. 3). These treatments are mentioned in the prescription, and post-treatment photographs suggested that they were widely applied across the project area. However, there are no systematic records available concerning the extent of these secondary treatments, so their overall effect is unquantifiable.
The combined thinning and burning treatment resulted in larger reductions in surface fuel loading than the thin-only treatment. These effects were best supported for the 1-hour, 10-hour, and litter and duff size classes. Reduced fuels in these size classes following burn treatments is unsurprising, because fuels in the smaller size classes are more easily consumed than their larger counterparts in prescribed fire (Knapp and others, 2005) and wildfire (Cansler and others, 2019). The two prescribed burns in this study were completed during cool conditions in October and were low intensity and patchy but were still locally effective at reducing surface fuel loading (figs. 2, 3).
The change in duff and litter depth after the combined thin and burn treatments may not be attributable to fuel consumption alone. Similar to our findings, Chiono and others (2012) documented that litter and duff were reduced following treatment, even in the absence of prescribed fire. Chiono and others (2012) postulated that litter and duff reductions following thin-only treatments may have been the result of fuel bed compaction or displacement by machinery. Fuel bed compaction was observed within the Northwest Gateway project, lending support to this phenomenon. There is uncertainty in our analysis because of the potential for misclassification and imprecision in the burn treatment specification. Plots were categorized as simply burned or not, with no additional information on whether the whole plot was burned, burn treatment effectiveness, or other attributes. It is possible, and perhaps likely, that some plots and their associated fuels transects were either unburned or burned at low enough intensity that fuels were largely unaffected. Assessing burn severity at such fine scales is beyond the scope of this work but represents an avenue for future research to understand mechanisms driving plot-level treatment effects.
There does not seem to be substantial differences in the effect of treatment on surface fuels in NWG and other projects that used whole tree harvesting methods in younger (non-old growth) stands (Stephens and others, 2009). These results suggest that similar treatments are likely to obtain similar results in mature versus old growth stand conditions.
Growth
As expected, we observed a strong growth release in Pinus following thinning treatments. This has important implications for the future persistence old growth legacy trees in this landscape because increased growth rates are correlated with vigor and lower rates of mortality (Dobbertin, 2005; Cailleret and others, 2019). Support for the post-treatment growth model including competitor basal area suggests that this release is likely because of reduced competition in treated area. These effects are consistent with prior research. Vernon and others (2018) reported that lower competition after thinning resulted in increased growth of Douglas-fir and ponderosa pine near Whiskeytown National Recreation Area, California. Fajardo and others (2007) reported that ponderosa pine growth increased following thin and combined thin and burn treatments, though the growth release was smaller in burned areas. We cored trees before the application of the burning treatment, so we were unable to characterize any effect of prescribed fire on growth at NWG. The relative effects of the two prescribed burns on growth was likely minimal given the cool prescription and patchy burns. There were few observations of extensive bole or crown scorch following the burns.
The effects of weather on annual growth are well established. Therefore, our finding that increased precipitation results in greater annual growth was unsurprising. Indeed, thinning treatments have been reported to lead to increased drought resistance (Vernon and others, 2018; Low and others, 2021), defined as the reduction or reversal of negative growth trends during periods of drought (Lloret and others, 2011). Additionally, annual precipitation was reported to positively affect growth for ponderosa pine and white fir in a separate study of prescribed fire effects also in LVNP (Wenderott and others, 2022). However, it is of some interest that the relative effect of precipitation on growth was weaker than the treatment effect. This phenomenon suggests that competition for other resources such as light and access to groundwater may have a greater effect on annual growth than interannual variability in local climate.
Our results demonstrate that thinning treatments were effective at reducing stand density and encouraging radial growth at the Northwest Gateway project area at Lassen Volcanic National Park. Our results also indicated that thinning combined with subsequent prescribed burning was the most effective at reducing surface fuel loads. Our observations seemed to indicate that harvest techniques such as whole tree yarding used at Lassen Volcanic National Park reduced the initial addition of post-treatment residual fuels, especially when combined with hand piling and other targeted treatments. This result was encouraging given the concern that thinning operations might increase surface fuel loading throughout the project area. Our results indicate that continued cycles of prescribed burning would maintain the current low surface fuel loadings and prevent the establishment of another dense cohort of fir in the understory.
Thinning treatments resulted in a growth release of large residual Pinus, indicating that even these well-established trees were affected by competition under pre-treatment conditions. The enhanced growth of the residual Pinus also indicates that these trees are more vigorous following treatments and may be more likely to survive future disturbances such as drought or bark beetle outbreaks, though some pine species may be more susceptable to beetles during sever outbreaks, especially following prescribed fire (Stephenson and others, 2019; Steel and others, 2021). The duration of this growth release will be dependent on environmental and biological conditions, including how stands are managed in the future.
Additionally, the post-treatment loss of small aspen underscored the importance of limiting mortality in small populations. Aspen are rare throughout the project area. Results indicate that continued monitoring may be necessary to determine if the treatments have been effective at preserving this population.
References Cited
Abatzoglou, J.T., Dobrowski, S.Z., Parks, S.A., and Hegewisch, K.C., 2018, TerraClimate, a high-resolution global dataset of monthly climate and climatic water balance from 1958–2015: Scientific Data, v. 5, 12 p. [Available at https://doi.org/10.1038/sdata.2017.191.]
Abella, S.R., Covington, W.W., Fulé, P.Z., Lentile, L.B., Sánchez Meador, A.J., and Morgan, P., 2007, Past, present, and future old growth in frequent-fire conifer forests of the western United States: Ecology & Society, v. 12, no. 2, article 16, 16 p. [Available at https://doi.org/10.5751/ES-02171-120216.]
Bunn, A.G., 2008, A dendrochronology program library in R (dplR): Dendrochronologia, v. 26, no. 2, p. 115–124. [Available at https://doi.org/10.1016/j.dendro.2008.01.002.]
Bürkner, P.-C., 2018, Advanced Bayesian multilevel modeling with the R package brms: The R Journal, v. 10, no. 1, p. 395–411. [Available at https://doi.org/10.32614/RJ-2018-017.]
Cailleret, M., Dakos, V., Jansen, S., Robert, E.M.R., Aakala, T., Amoroso, M.M., Antos, J.A., Bigler, C., Bugmann, H., Caccianaga, M., Camarero, J.-J., Cherubini, P., Coyea, M.R., Čufar, K., Das, A.J., Davi, H., Gea-Izquierdo, G., Gillner, S., Haavik, L.J., Hartmann, H., Hereş, A.-M., Hultine, K.R., Janda, P., Kane, J.M., Kharuk, V.I., Kitzberger, T., Klein, T., Levanic, T., Linares, J.-C., Lombardi, F., Mäkinen, H., Mészáros, I., Metsaranta, J.M., Oberhuber, W., Papadopoulos, A., Petritan, A.M., Rohner, B., Sangüesa-Barreda, G., Smith, J.M., Stan, A.B., Stojanovic, D.B., Suarez, M.-L., Svoboda, M., Trotsiuk, V., Villalba, R., Westwood, A.R., Wyckoff, P.H., and Martínez-Vilalta, J., 2019, Early-warning signals of individual tree mortality based on annual radial growth: Frontiers in Plant Science, v. 9, 14 p. [Available at https://doi.org/10.3389/fpls.2018.01964.]
Cansler, C.A., Swanson, M.E., Furniss, T.J., Larson, A.J., and Lutz, J.A., 2019, Fuel dynamics after reintroduced fire in an old-growth Sierra Nevada mixed-conifer forest: Fire Ecology, v. 15, article 16, 17 p. [Available at https://doi.org/10.1186/s42408-019-0035-y.]
Chiono, L.A., O’Hara, K.L., De Lasaux, M.J., Nader, G.A., and Stephens, S.L., 2012, Development of vegetation and surface fuels following fire hazard reduction treatment: Forests, v. 3, no. 3, p. 700–722. [Available at https://doi.org/10.3390/f3030700.]
Dobbertin, M., 2005, Tree growth as indicator of tree vitality and of tree reaction to environmental stress—A review: European Journal of Forest Research, v. 124, no. 4, p. 319–333. [Available at https://doi.org/10.1007/s10342-005-0085-3.]
Fajardo, A., Graham, J.M., Goodburn, J.M., and Fiedler, C.E., 2007, Ten-year responses of ponderosa pine growth, vigor, and recruitment to restoration treatments in the Bitterroot Mountains, Montana, USA: Forest Ecology and Management, v. 243, no. 1, p. 50–60. [Available at https://doi.org/10.1016/j.foreco.2007.02.006.]
Feeney, S.R., Kolb, T.E., Covington, W.W., and Wagner, M.R., 1998, Influence of thinning and burning restoration treatments on presettlement ponderosa pines at the Gus Pearson Natural Area: Canadian Journal of Forest Research, v. 28, no. 9, p. 1295–1306. [Available at https://doi.org/10.1139/x98-103.]
Gabry, J., Simpson, D., Vehtari, A., Betancourt, M., and Gelman, A., 2019, Visualization in Bayesian workflow: Journal of the Royal Statistical Society, v. 182, no. 2, p. 389–402. [Available at https://doi.org/10.1111/rssa.12378.]
Gelman, A., 2008, Scaling regression inputs by dividing by two standard deviations: Statistics in Medicine, v. 27, no. 15, p. 2865–2873. [Available at https://doi.org/10.1002/sim.3107.]
Hood, S.M., Varner, J.M., van Mantgem, P., and Cansler, C.A., 2018, Fire and tree death—Understanding and improving modeling of fire-induced tree mortality: Environmental Research Letters, v. 13, no. 11, 17 p. [Available at https://doi.org/10.1088/1748-9326/aae934.]
Innes, J.C., North, M.P., and Williamson, N., 2006, Effect of thinning and prescribed fire restoration treatments on woody debris and snag dynamics in a Sierran old-growth, mixed-conifer forest: Canadian Journal of Forest Research, v. 36, no. 12, p. 3183–3193. [Available at https://doi.org/10.1139/x06-184.]
Knapp, E.E., Bernal, A.A., Kane, J.M., Fettig, C.J., and North, M.P., 2021, Variable thinning and prescribed fire influence tree mortality and growth during and after a severe drought: Forest Ecology and Management, v. 479, 12 p. [Available at https://doi.org/10.1016/j.foreco.2020.118595.]
Knapp, E.E., Keeley, J.E., Ballenger, E.A., and Brennan, T.J., 2005, Fuel reduction and coarse woody debris dynamics with early season and late season prescribed fire in a Sierra Nevada mixed conifer forest: Forest Ecology and Management, v. 208, nos. 1–3, p. 383–397. [Available at https://doi.org/10.1016/j.foreco.2005.01.016.]
Lloret, F., Keeling, E.G., and Sala, A., 2011, Components of tree resilience—Effects of successive low-growth episodes in old ponderosa pine forests: Oikos, v. 120, no. 12, p. 1909–1920. [Available at https://doi.org/10.1111/j.1600-0706.2011.19372.x.]
Low, K.E., Collins, B.M., Bernal, A., Sanders, J.E., Pastor, D., Manley, P., White, A.M., and Stephens, S.L., 2021, Longer-term impacts of fuel reduction treatments on forest structure, fuels, and drought resistance in the Lake Tahoe Basin: Forest Ecology and Management, v. 479, 11 p. [Available at https://doi.org/10.1016/j.foreco.2020.118609.]
Lutes, D.C., Benson, N.C., Keifer, M., Caratti, J.F., and Streetman, S.A., 2009, FFI—A software tool for ecological monitoring: International Journal of Wildland Fire, v. 18, no. 3, p. 310–314. [Available at https://doi.org/10.1071/WF08083.]
Lydersen, J.M., Collins, B.M., Brooks, M.L., Matchett, J.R., Shive, K.L., Povak, N.A., Kane, V.R., and Smith, D.F., 2017, Evidence of fuels management and fire weather influencing fire severity in an extreme fire event: Ecological Applications, v. 27, no. 7, p. 2013–2030. [Available at https://doi.org/10.1002/eap.1586.]
Maloney, P.E., Smith, T.F., Jensen, C.E., Innes, J., Rizzo, D.M., and North, M.P., 2008, Initial tree mortality and insect and pathogen response to fire and thinning restoration treatments in an old-growth mixed-conifer forest of the Sierra Nevada, California: Canadian Journal of Forest Research, v. 38, no. 12, p. 3011–3020. [Available at https://doi.org/10.1139/X08-141.]
Perrakis, D.D.B., Agee, J.K., and Eglitis, A., 2011, Effects of prescribed burning on mortality and resin defenses in old growth ponderosa pine (Crater Lake, Oregon)—Four years of post-fire monitoring: Natural Areas Journal, v. 31, no. 1, p. 14–25. [Available at https://doi.org/10.3375/043.031.0103.]
R Core Team, 2022, R—A language and environment for statistical computing (version 4.0.4): Vienna, Austria, R Foundation for Statistical Computing software release. [Available at https://www.R-project.org/.]
Raymond, C.L., and Peterson, D.L., 2005, Fuel treatments alter the effects of wildfire in a mixed-evergreen forest, Oregon, USA: Canadian Journal of Forest Research, v. 35, no. 12, p. 2981–2995. [Available at https://doi.org/10.1139/x05-206.]
Ryan, K.C., Knapp, E.E., and Varner, J.M., 2013, Prescribed fire in North American forests and woodlands—History, current practice, and challenges: Frontiers in Ecology and the Environment, v. 11, no. s1, p. e15–e24. [Available at https://doi.org/10.1890/120329.]
Schwilk, D.W., Keeley, J.E., Knapp, E.E., McIver, J., Bailey, J.D., Fettig, C.J., Fiedler, C.E., Harrod, R.J., Moghaddas, J.J., Outcalt, K.W., Skinner, C.N., Stephens, S.L., Waldrop, T.A., Yaussy, D.A., and Youngblood, A., 2009, The national fire and fire surrogate study—Effects of fuel reduction methods on forest vegetation structure and fuels: Ecological Applications, v. 19, no. 2, p. 285–304. [Available at https://doi.org/10.1890/07-1747.1.]
Sohn, J.A., Hartig, F., Kohler, M., Huss, J., and Bauhus, J., 2016, Heavy and frequent thinning promotes drought adaptation in Pinus sylvestris forests: Ecological Applications, v. 26, no. 7, p. 2190–2205. [Available at https://doi.org/10.1002/eap.1373.]
Steel, Z.L., Goodwin, M.J., Meyer, M.D., Fricker, G.A., Zald, H.S.J., Hurteau, M.D., and North, M.P., 2021, Do forest fuel reduction treatments confer resistance to beetle infestation and drought mortality?: Ecosphere, v. 12, no. 1, 19 p. [Available at https://doi.org/10.1002/ecs2.3344.]
Stephens, S.L., McIver, J., Boerner, R.E.J., Fettig, C.J., Fontaine, J.B., Hartsough, B.R., Kennedy, P.L., and Schwilk, D.W., 2012, The effects of forest fuel-reduction treatments in the United States: Bioscience, v. 62, no. 6, p. 549–560. [Available at https://doi.org/10.1525/bio.2012.62.6.6.]
Stephens, S.L., and Moghaddas, J.J., 2005, Experimental fuel treatment impacts on forest structure, potential fire behavior, and predicted tree mortality in a California mixed conifer forest: Forest Ecology and Management, v. 215, nos. 1–3, p. 21–36. [Available at https://doi.org/10.1016/j.foreco.2005.03.070.]
Stephens, S.L., Moghaddas, J.J., Edminster, C., Fiedler, C.E., Haase, S., Harrington, M., Keeley, J.E., Knapp, E.E., McIver, J.D., Metlen, K., Skinner, C.N., and Youngblood, A., 2009, Fire treatment effects on vegetation structure, fuels, and potential fire severity in western U.S. forests: Ecological Applications, v. 19, no. 2, p. 305–320. [Available at https://doi.org/10.1890/07-1755.1.]
Stephenson, N.L., Das, A.J., Ampersee, N.J., Bulaon, B.M., and Yee, J.L., 2019, Which trees die during drought? The key role of insect host‐tree selection: Journal of Ecology, v. 107, no. 5, p. 2383–2401. [Available at https://doi.org/10.1111/1365-2745.13176.]
Taylor, A.H., 2000, Fire regimes and forest changes in mid and upper montane forests of the southern Cascades, Lassen Volcanic National Park, California, U.S.A.: Journal of Biogeography, v. 27, no. 1, p. 87–104. [Available at https://doi.org/10.1046/j.1365-2699.2000.00353.x.]
Vaillant, N.M., Fites-Kaufman, J., Reiner, A.L., Noonan-Wright, E.K., and Dailey, S.N., 2009, Effect of fuel treatments on fuels and potential fire behavior in California, USA, national forests: Fire Ecology, v. 5, no. 2, p. 14–29. [Available at https://doi.org/10.4996/fireecology.0502014.]
van Wagtendonk, J.W., and Moore, P.E., 2010, Fuel deposition rates of montane and subalpine conifers in the central Sierra Nevada, California, USA: Forest Ecology and Management, v. 259, no. 10, p. 2122–2132. [Available at https://doi.org/10.1016/j.foreco.2010.02.024.]
Vernon, M.J., Sherriff, R.L., van Mantgem, P., and Kane, J.M., 2018, Thinning, tree-growth, and resistance to multi-year drought in a mixed-conifer forest of northern California: Forest Ecology and Management, v. 422, p. 190–198. [Available at https://doi.org/10.1016/j.foreco.2018.03.043.]
Wenderott, Z., van Mantgem, P.J., Wright, M.C., Farris, C.A., and Sherriff, R.L., 2022, Long-term effects of prescribed fire on large tree growth in mixed conifer forests at Lassen Volcanic National Park, California: Forest Ecology and Management, v. 517, 10 p. [Available at https://doi.org/10.1016/j.foreco.2022.120260.]
Westlind, D.J., and Kerns, B.K., 2021, Repeated fall prescribed fire in previously thinned Pinus ponderosa increases growth and resistance to other disturbances: Forest Ecology and Management, v. 480, 10 p. [Available at https://doi.org/10.1016/j.foreco.2020.118645.]
Zald, H.S.J., Callahan, C.C., Hurteau, M.D., Goodwin, M.J., and North, M.P., 2022, Tree growth responses to extreme drought after mechanical thinning and prescribed fire in a Sierra Nevada mixed-conifer forest, USA: Forest Ecology and Management, v. 510, 13 p. [Available at https://doi.org/10.1016/j.foreco.2022.120107.]
Zeibig-Kichas, N.E., Ardis, C.W., Berrill, J.-P., and King, J.P., 2016, Bark thickness equations for mixed-conifer forest type in Klamath and Sierra Nevada mountains of California: International Journal of Forestry Research, v. 2016, 10 p. [Available at https://doi.org/10.1155/2016/1864039.]
Glossary
Basal Area
Cross-sectional area of a tree stem.
Basal Area Factor
Amount of basal area per hectare acre represented by each tree counted.
Basal Area Increment
Cross-sectional area of a single tree ring.
Diameter at Breast Height
Diameter of a tree stem at a height of 1.37 meters.
Stand Density Index
The number of trees in a stand relative to a tree of average basal area, providing an index of crowding and growth potenital.
Conversion Factors
International System of Units to U.S. customary units
For more information concerning the research in this report, contact the
Director, Western Ecological Research Center
U.S. Geological Survey
3020 State University Drive East
Sacramento, California 95819
https://www.usgs.gov/centers/werc
Publishing support provided by the U.S. Geological Survey
Science Publishing Network, Sacramento Publishing Service Center
Disclaimers
Any use of trade, firm, or product names is for descriptive purposes only and does not imply endorsement by the U.S. Government.
Although this information product, for the most part, is in the public domain, it also may contain copyrighted materials as noted in the text. Permission to reproduce copyrighted items must be secured from the copyright owner.
Suggested Citation
Wright, M.C., van Mantgem, P.J., and Farris, C., 2023, Forest structure and residual tree growth at the Northwest Gateway project area, Lassen Volcanic National Park, California: U.S. Geological Survey Open-File Report 2023–1021, 23 p., https://doi.org/10.3133/ofr20231021
ISSN: 2331-1258 (online)
Study Area
Publication type | Report |
---|---|
Publication Subtype | USGS Numbered Series |
Title | Forest structure and residual tree growth at the Northwest Gateway project area, Lassen Volcanic National Park, California |
Series title | Open-File Report |
Series number | 2023-1021 |
DOI | 10.3133/ofr20231021 |
Year Published | 2023 |
Language | English |
Publisher | U.S. Geological Survey |
Publisher location | Reston, VA |
Contributing office(s) | Western Ecological Research Center |
Description | viii, 23 p. |
Country | United States |
State | California |
Other Geospatial | Lassen Volcanic National Park |
Online Only (Y/N) | Y |
Google Analytic Metrics | Metrics page |