Cross Section N–N' Through the Valley and Ridge Province of the Southern Appalachian Basin, from Greene County, West-Central Alabama, to Bibb County, Central Alabama
Links
- Document: Pamphlet (2.14 MB pdf) , HTML , XML
- Sheet: Sheet (5.76 MB pdf)
- Related Works:
- Scientific Investigations Map 3425 - Geologic Cross Section A–A′ Through the Appalachian Basin from the Southern Margin of the Ontario Lowlands Province, Genesee County, Western New York, to the Valley and Ridge Province, Lycoming County, North-Central Pennsylvania
- Scientific Investigations Map 3343 - Geologic Cross Section I–I′ Through the Appalachian Basin from the Eastern Margin of the Illinois Basin, Jefferson County, Kentucky, to the Valley and Ridge Province, Scott County, Virginia
- Scientific Investigations Map 3172 - Geologic cross section C-C' through the Appalachian basin from Erie County, north-central Ohio, to the Valley and Ridge province, Bedford County, south-central Pennsylvania
- Scientific Investigations Map 3067 - Geologic Cross Section D-D' Through the Appalachian Basin from the Findlay Arch, Sandusky County, Ohio, to the Valley and Ridge Province, Hardy County, West Virginia
- Scientific Investigations Map 2985 - Geologic Cross Section E-E' through the Appalachian Basin from the Findlay Arch, Wood County, Ohio, to the Valley and Ridge Province, Pendleton County, West Virginia
- Download citation as: RIS | Dublin Core
Acknowledgments
The authors wish to thank William A. Thomas (deceased October 1, 2022) of the University of Kentucky and the Geological Survey of Alabama for his insightful comments, and contributions to cross section N–N', and for his interest, guidance, and time spent in scientific correspondence. We also wish to thank Ben Byerly and Brian S. Cook of the Geological Survey of Alabama and Timothy M. Chowns of the University of West Georgia for reviewing this report and for their insightful comments and contributions to cross section N–N'.
Introduction
Geologic cross section N–N′ is the sixth in a series of geologic cross sections constructed by the U.S. Geological Survey (USGS) to document and improve understanding of the geologic framework and petroleum systems of the Appalachian basin. Cross section N–N′ provides a regional view of the structural and stratigraphic framework of the Appalachian basin in the Valley and Ridge province in western and central Alabama; it spans approximately 69 miles (mi) (111 kilometers [km]) (see index maps 1–2 and figs. 1–2 on map sheet). The terms “cross section N–N′” and “study area” are used interchangeably in the report.
This geologic cross section is a companion to geologic cross sections E–E′, D–D′, C–C′, I–I′, and A–A′ (Ryder and others, 2008; Ryder and others, 2009; Ryder and others, 2012; Ryder and others, 2015, Trippi and others, 2019, respectively) that are located approximately 350 to 550 miles (563 to 885 km) to the northeast (see index map 1 on map sheet). Cross section N–N' complements earlier geologic cross sections through the Alabama part of the Appalachian basin by Eargle (1947), Thomas (1972, 1979, 1988, 1991, 2001, 2007, 2008, and 2018), Kidd and Neathery (1976), Hooks (1985), Bayona and others (2003), Bayona and Thomas (2003), Kopaska-Merkel and others (2005), Thomas and Bayona (2005), Surles (2007), Cook and Thomas (2010), Groshong and others (2010), Pashin (2010), Pashin and others (2011), Thomas and Pashin (2011), Pashin and others (2012), Robinson and others (2012), Cato (2014), and Pashin and others (2014). Although some of these other cross sections show more structural and stratigraphic detail, they are of more limited extent geographically and stratigraphically.
Cross section N–N′ contains information that is useful for evaluating energy resources in the Appalachian basin. Although the Appalachian basin petroleum systems identified by Swezey (2002) and Milici and others (2003) are not shown on the cross section, many of their key elements (such as source rocks, reservoir rocks, seals, and traps) can be inferred from lithologic units, unconformities, and geologic structures shown on the cross section. Other aspects of petroleum systems (such as the timing of petroleum generation and petroleum migration pathways) may be evaluated by burial history, thermal history, and fluid flow models based on what is shown on the cross section. In addition, cross section N–N′ may be used as a reconnaissance tool to identify plausible geologic structures and strata for the subsurface storage of liquid waste (for example, Colton, 1961; Lloyd and Reid, 1990; Clark and others, 2005) or for the sequestration of carbon dioxide (for example, Smith and others, 2002; Lucier and others, 2006).
Table 1.
Drill holes used to construct cross section N–N’.[Drill-hole locations are shown in figures 1 and 2. Source of all mud logs and drill-cutting and (or) core sample logs is the Geological Survey of Alabama. Latitude and longitude values are given in decimal degrees based on North American Datum of 1983. Abbreviations: Ala., Alabama; API, American Petroleum Institute; BKB, below kelly bushing; Co., County; No., number; ?, identity of system or stratigraphic unit is uncertain. *, total depth for drill hole 207 is feet below ground level]
Cross section N–N′ is oriented southwest to northeast, approximately parallel to the structural grain of the region, the Appalachian fold and thrust belt. The topographic profile for the cross section (on map sheet) was created from the USGS National Elevation Dataset (NED) with a one arc-second cell size. Corrections to the original topographic profile were based on topographic contour lines on the following USGS 7.5-minute topographic quadrangle maps (from southwest to northeast): Forkland, Mason Bend, Melton, Sawyerville, Ingram, Hogglesville, Heiberger, Pondville, Centreville West, West Blocton West, West Blocton East, and Halfmile Shoals, Alabama. This topographic profile is approximate and should not be used to determine accurate surface elevations. For example, we noticed errors near drill holes 203, 204, and 205, where the actual surface elevation (as shown on well logs) is more than 25 feet (ft) (7.6 meters [m]) above the topographic profile’s elevation. This discrepancy was probably because the NED elevation values are average values for numerous points in the one arc-second cells. In locations with highly variable topography, the actual elevation of a point location within the cell may differ from the average elevation of the cell. The data can be accessed from the USGS National Map website at https://www.usgs.gov/core-science-systems/national-geospatial-program/national-map.
Cross section N–N′ is based on geological and geophysical data from eight drill holes (table 1). Several bends in the cross section are required to accommodate the sparse number of drill holes that penetrate a thick section of Paleozoic sedimentary rocks.
The depths to the tops of each stratigraphic unit that was penetrated in the eight drill holes were converted from depth in feet below the kelly bushing (KB) to depth below ground level (GL) (except for drill hole 207, which only had a GL value), and then were plotted on the cross section with respect to mean sea level (MSL). Detailed depth information for the tops of the stratigraphic units in each drill hole is reported in appendix 1.
The correlation of stratigraphic intervals between drill holes was based on a variety of geophysical (wireline) and lithologic logs. Gamma-ray logs were the most common geophysical logs, whereas mud logs and sample description logs (for cuttings and [or] cores) produced by the Geological Survey of Alabama (GSA) were the most common lithologic logs (table 1, appendix 2, table 2.1). Gamma-ray logs used for correlations were digitized and (or) purchased as log ASCII standard files (LAS files), converted to graphic images, and then plotted next to their respective drill holes (see cross section N–N′). The lithology assigned to each stratigraphic interval was simplified to just a few rock types based on mud logs, GSA sample description logs, and previous publications (Butts, 1940; Wahl, 1966; Thomas, 1972, 1988; Kidd and Neathery, 1976; Batchelder, 1984; Sternbach, 1984; Hooks, 1985; Raymond and Copeland, 1987; Raymond and others, 1988; Szabo and others, 1988; Raymond, 1991a, b; Osborne and others, 1998, 2000; Osborne and Irvin, 2002; Kopaska-Merkel and others, 2005; Surles, 2007). We also used unpublished original Amoco well data for some formation top elevations in drill hole 202 (James L. Coleman, Coleman Geological Services, written communication, 1984).
Data from drill holes were supplemented by bedrock geology and topography. Some details of Alabama stratigraphy, and locations of anticlines, synclines, and faults were obtained from the geologic map of Alabama (Szabo and others, 1988) and other bedrock geologic maps and reports by Wahl (1966), Reed (1972), Szabo (1972), Thomas (1972, 1988, 1991), Davis and others (1975, 1980), Kidd and Neathery (1976), Szabo and others (1976), Causey (1978), Raymond and Copeland (1987), Higgins and others (1988), Raymond and others (1988), Osborne and others (1998, 2000), Osborne and Irvin (2002), Kopaska-Merkel and others (2005), Surles (2007), Groshong and others (2010), Thomas and Pashin (2011), and Steltenpohl and others (2013).
Coal beds in drill holes 206 and 208 were recognized from mud logs and identified based on the generalized section of the Pottsville Formation given by Szabo and others (1976). Using the geologic map from the same publication, we then used this information to infer the identity of coal beds in the northeastern half of cross section N–N′ as well as their geologic structure. Because coal bed horizons and formation contacts are inferred from surface outcrops rather than from well logs, the actual subsurface configuration may differ from that shown.
Although some correlations shown on cross section N–N′ are based on our own interpretations, many correlations are adopted or modified from sample description logs of the GSA and other logs from previous publications. Stratigraphic nomenclature follows existing terminology wherever possible. Useful references for stratigraphic correlations and (or) nomenclature include the following: Thomas (1972, 1991), Davis and others (1975), Reed and others (1975), Raymond and Copeland (1987), Higgins and others (1988), Raymond and others (1988), Szabo and others (1988), and Kopaska-Merkel and others (2005). A correlation chart for the stratigraphic units identified along cross section N–N′ is shown in figure 3 (on map sheet).
Regional unconformities shown in figure 3 include (1) the Ediacaran through Cambrian “Great Unconformity” (Karlstrom and Timmons, 2012; Shahkaramia and others, 2020), (2) the Lower to Middle Ordovician Knox unconformity (hereinafter called the “post-Knox unconformity”) (Harris and Repetski, 1982; Repetski, 1982; Mussman and others, 1988; Swezey, 2002; Dwyer and Repetski, 2012), (3) the Upper Ordovician to lower Silurian (Llandovery) Cherokee unconformity (Dennison and Head, 1975; Brett and others, 1990; Swezey, 2002), and (4) the Middle to Upper Devonian Acadian unconformity (Swezey, 2002). Five other regional unconformities are present in the study area, one from the middle to upper Silurian (Wenlock to Ludlow), one from the upper Silurian (Pridoli) to Lower Devonian (including the Lower Devonian Wallbridge unconformity), two smaller unconformities in the Upper Devonian to Lower Mississippian and the Lower Mississippian, and a fifth one from the Lower Pennsylvanian through the Jurassic (Raymond and others, 1988). Figure 3 also shows the North American sequences of Sloss (1988). Based on integrated regional paleontological and lithologic studies, Read and Repetski (2012) indicated that there may be multiple “intra-Knox” unconformities or highly thinned intervals, one of which separates the Chepultepec Dolomite from the overlying Longview Limestone; they also interpret unconformities at the top of the lower Cambrian (Series 2) Rome Formation and the top of the middle Cambrian (Series 2 to Miaolingian) Conasauga Formation.
Cross section N–N′ is located in the Appalachian fold-and-thrust belt (fig. 1) which is deformed by thin-skinned structures (Rodgers, 1963; Harris and Milici, 1977). Raymond and others (1988) subdivide the fold-and-thrust belt into three domains, a northwestern domain that is part of the Appalachian Plateau province, and central and southeastern domains that are within the Valley and Ridge province in Alabama (hereinafter referred to as “VRPA”) (fig. 1). Cross section N–N' is in the central and southeastern domains of Raymond and others (1988) and the VRPA (figs. 1 and 3).
The thin-skinned structures in the Appalachian fold-and-thrust belt are contractional in origin and characterized by listric or ramp-flat thrust faults (and associated folds) with basal detachment in lower to middle Cambrian strata (Thomas, 2001; Surles, 2007; Pashin and others, 2011). In addition, the thin-skinned structures are commonly associated with complicated duplex structures having expanded, ductile cores named “mushwads” by Thomas (2001). Alabama mushwads are controlled by basement-involved border faults of the Birmingham graben (Thomas, 2010) (see discussion of mushwads in the Structural Framework section of this report).
Strata of the VRPA, are juxtaposed against lesser deformed strata of the Black Warrior basin (and Appalachian Plateaus province) (northwestern domain of the fold-and-thrust belt) (Raymond and others, 1988; Pashin and others, 2011) (fig. 1).
Beneath the Phanerozoic rocks of Alabama lie Mesoproterozoic crystalline basement rocks of the Laurentian eastern granite-rhyolite province in northwestern Alabama (about 1.47 Ga [giga-annum]; Fisher and others, 2010) and the Grenville province in northeastern and central Alabama (1.1–1.0 Ga; Fisher and others, 2010), the peri-Gondwanan Uchee terrane (in southeastern Alabama), and the Gondwanan Suwannee terrane (in southern Alabama) (fig. 4) (Steltenpohl and others, 2013). Earlier work by Neathery and Copeland (1983) found K-Ar ages of 750 and 652 Ma (mega-annum) in basement rocks from wells in Cullman County, Alabama (F.W. Smith 26-6 No. 1), Morgan County, Alabama (Skidmore 36-1 No. 1), and Dade County, Georgia (Brown No. 1) (see index map 2 on map sheet). However, the authors also mention the possibility that the 750 Ma age could be anomalously low due to laboratory error, and the 652 Ma age might be low because of resetting by fault movement. This report follows the age range of 1.1–1.0 Ga for basement rocks in the Grenville province in Alabama per Fisher and others (2010).
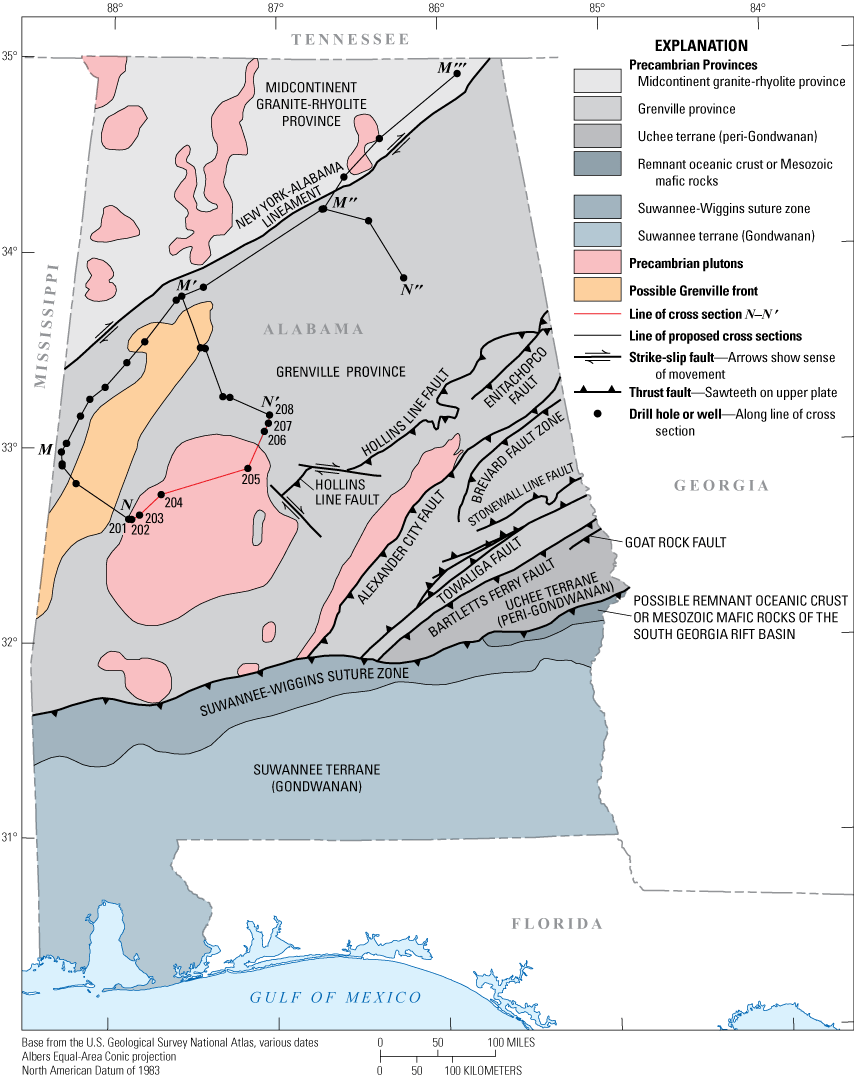
Map showing Precambrian provinces of Alabama based on magnetic and gravity data (Steltenpohl and others, 2013), and the locations of cross section N–N′ (red line with drill holes/wells) and proposed cross sections (black lines with drill holes/wells).
Crystalline basement rocks underlying the Paleozoic sedimentary cover rocks on cross section N–N’ are deformed by extensional faults described by Thomas (2001, 2007, 2010, 2018), Bayona and others (2003), Bayona and Thomas (2003), Thomas and Bayona (2005), Bailey (2007), Surles (2007), Pashin (2010), Thomas and Pashin (2011), Robinson and others (2012), and Cato (2014). These basement-involved border faults of the Birmingham graben commonly controlled the location of the thin-skinned structures, particularly the mushwads.
Structural Framework
Basement Rocks and Associated Studies
Basement rocks along cross section N–N' consist of igneous and metamorphic rocks of the southernmost part of the Grenville province (Steltenpohl and others, 2013) (fig. 4). Underlying drill holes 201–205 is a large circular feature (Domain 6 of Steltenpohl and others, 2013) with a magnetic-high anomaly and coincident gravity-low interpreted to be a possible shallow crustal bulbous pluton of unknown age (fig. 4). Drill holes 206–208 are underlain by an area of indistinct magnetic and gravity character (Domain 4 of Steltenpohl and others, 2013) with northeast-trending magnetic-highs and gravity-lows that surrounds the northern half of Domain 6 (fig. 4). Domain 4 constitutes the northernmost part of the Grenville province in Alabama, which contacts the adjacent midcontinent granite-rhyolite province at the New York-Alabama lineament (Steltenpohl and others, 2013) (fig. 4). Domain 4 also includes a N15°E-trending magnetic low (Domain 4C) about 17 miles (27 km) northwest of drill hole 201 that Steltenpohl and others (2013) interpret to be the southernmost part of the true Grenville front, which they interpret as being severed and displaced from its northern part by right-lateral movement along the New York-Alabama lineament (fig. 4). Rocks of the Grenville province in Alabama range between 1.1 and 1.0 billion years old (Fisher and others, 2010).
Typical of many foreland basins, the crystalline basement of the Appalachians to the northeast of Alabama dips gently southeastward from an interior craton to the external margin of a fold-and-thrust belt (for example, see Ryder and others, 2008, 2009, 2012, and 2015; and Trippi and others, 2019). But cratonic basement rocks in western Alabama and adjoining Mississippi dip to the southwest and underlie the Ouachita thrust belt (fig. 5). Large-magnitude, northwest-striking, down-to-the-southwest basement-involved normal faults parallel the Ouachita thrust belt and the Alabama-Oklahoma transform fault (Surles, 2007).
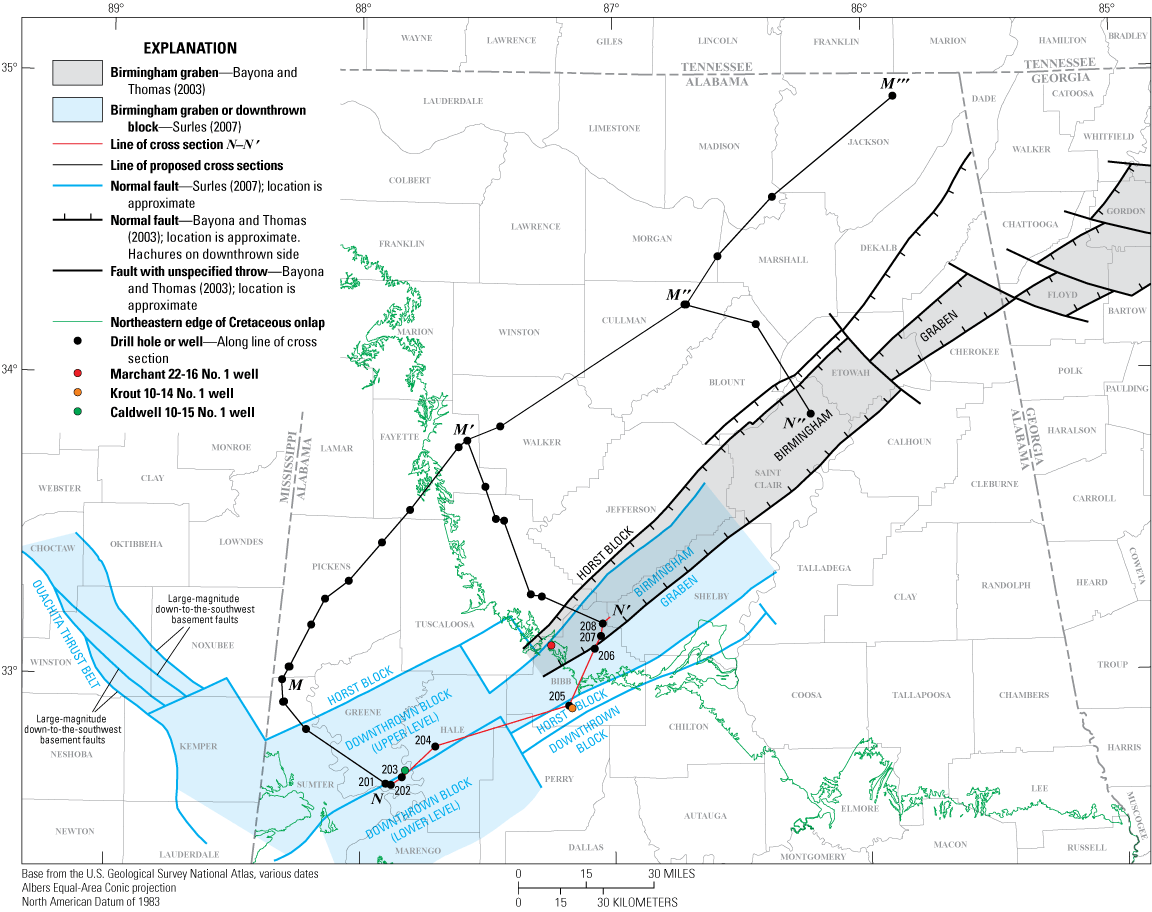
Map showing the Birmingham graben in Georgia and Alabama, a larger downthrown block southwest of the Birmingham graben in Alabama, and downthrown blocks in the Ouachita thrust belt in western Alabama and Mississippi. All of these are features in Precambrian basement rocks based on Bayona and Thomas (2003) and Surles (2007). The Birmingham graben (northeast of Cretaceous onlap sediments) is from Bayona and Thomas (2003). Surles (2007) shows a wider Birmingham graben that appears to open into a larger downthrown block to its southeast and downthrown blocks in the Ouachita thrust belt to the west (see fig. 6). The locations of cross section N–N′ (with drill holes 201–208) and proposed cross sections are also shown for reference.
The Birmingham graben (figs. 5 and 6) is a lower Cambrian rift system trending northeastward from west-central Alabama to northwestern Georgia (Thomas, 1991; Bayona and Thomas, 2003; Surles, 2007). Bayona and Thomas (2003) show the Birmingham graben ranging from 8 to 20 miles (12.8 to 32 km) wide and about 120 miles (193 km) long from northwestern Georgia to central Alabama where it disappears below Cretaceous onlap sediments (fig. 5). Surles (2007) shows part of the Birmingham graben ranging from 10 to 25 miles (16 to 40 km) wide trending northeast from Hale County through Bibb, Jefferson, Shelby, and St. Clair Counties, Alabama (figs. 5 and 6) (Surles [2007] doesn’t show the full extent of the graben on his map). Cross section N–N′, runs from Greene County, Alabama, northeastward through Hale and Perry Counties to Bibb County in the VRPA partially within the Birmingham graben (figs. 5 and 6). Although no wells on cross section N–N′ penetrate the Bessemer mushwad strata of the Conasauga Formation (fig. 6), drill hole 202 penetrates the Conasauga Formation in Greene County, and as discussed later, the nearby Marchant 22-16 No. 1 penetrates the contorted Conasauga Formation within the Bessemer mushwad (figs. 6 and 7).
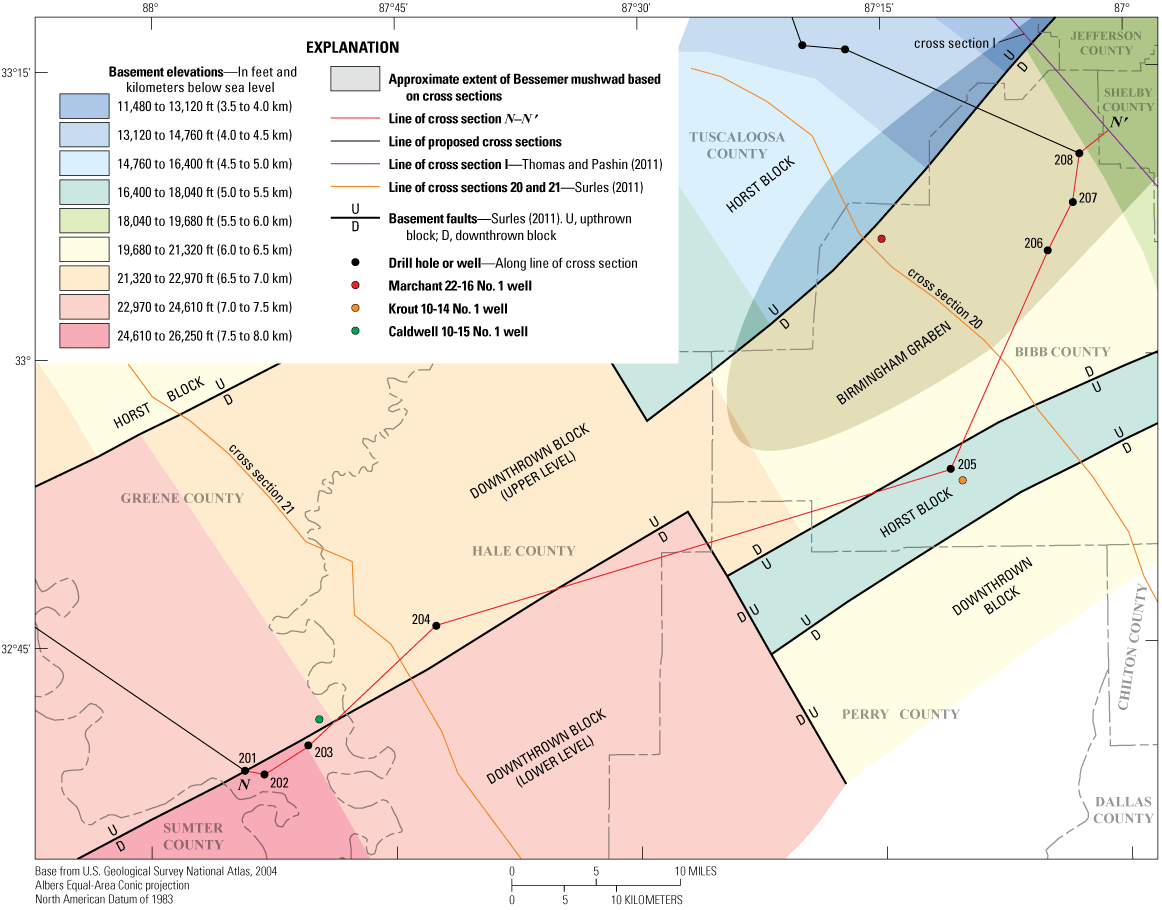
Map showing basement elevations in the study area, including the Birmingham graben and the downthrown block southwest of the Birmingham graben (based on Surles, 2007). Basement faults (based on Surles, 2007), locations of cross section N–N′ and proposed cross sections, cross sections 20 and 21 from Surles (2007), cross section I from Thomas and Pashin (2011), and the approximate extent of the Bessemer mushwad are also shown. The Bessemer mushwad extent is based on cross sections 20 and 21 from Surles (2007) and cross section I from Thomas and Pashin (2011). D, downthrown block; ft, feet; km, kilometer; U, upthrown block.
The basement rocks in the study area were tectonically divided into several blocks that define the Birmingham graben, adjacent horst blocks, and a larger downthrown block to the southwest of the Birmingham graben (figs. 5 and 6). Based on well data and seismic reflection profiles, depth to the top of basement along cross section N–N' increases southwestward from about 19,400 ft (5,913 m) below mean sea level (BMSL) at the northeastern end of the cross section where it intersects cross section I of Thomas and Pashin (2011) to 24,900 ft (7,590 m) BMSL near the southwestern end of the cross section (Surles, 2007) (fig. 6). Drill holes 206, 207, and 208 are located within the Birmingham graben area with projected approximate depths to basement of 19,988, 19,822, and 19,706 ft (6,092, 6,042, and 6,006 m) BMSL, respectively (fig. 6). Southwest of these drill holes, the top of basement in the Birmingham graben descends to a depth of about 21,571 ft (6,575 m) BMSL (fig. 6). Drill hole 205 is located above a horst block southeast of the Birmingham graben with a projected depth to basement of only 16,811 ft (5,124 m) BMSL (fig. 6). Depths to basement along the cross section on the horst block southeast of the Birmingham graben range from 16,716 to 17,053 ft (5,095 to 5,198 m) BMSL (from northeast to southwest) (fig. 6).
The Birmingham graben opens to the southwest into a much wider downthrown block for which the Surles (2007) map shows no horst block to the southeast (fig. 6). It is unclear whether there is a horst block to the southeast of the downthrown block outside the extent of the map, or if this in fact, is a half graben (fig. 6). The downthrown block has two levels, an upper northwestern level ranging from 21,571 to 24,610 ft (6,575 to 7,501 m) BMSL, and a lower southeastern level that ranges from 22,970 to 26,250 ft (7,001 to 8,001 m) BMSL (fig. 6). Drill hole 204 lies above the upper level of the downthrown block where basement depth is 22,538 ft (6,870 m) BMSL. Drill holes 203 and 202 lie above the lower level of the downthrown block where basement depths are 24,614 and 24,835 ft (7,502 and 7,570 m) BMSL, respectively. Drill hole 201, the most southwestern drill hole on the cross section, lies above the upper level of the downthrown block where basement depth is 23,233 ft (7,081 m) BMSL (fig. 6). The depth to basement in the adjoining horst block to the northwest of the Birmingham graben and the upper level of the downthrown block ranges from 9,840 to 22,970 ft (2,999 to 7,001 m) (from northeast to southwest) (fig. 6; highest and lowest depths to basement are not shown in fig. 6 but are shown in fig. 5 in Surles [2007]). To the northwest of the upper level of the downthrown block northeast of the Ouachita thrust belt (southwest of the Black Warrior basin), basement depths range from 22,970 to 24,610 ft (7,001 to 7,501 m).
The normal (extensional) basement faults bounding the Birmingham graben in Alabama and Georgia may have been reactivated with reverse movement during the Middle Ordovician (Whiterockian) (Bayona and Thomas, 2003; Pashin and others, 2011; Pashin and others, 2012). Bayona and Thomas (2003) propose that contraction and lithospheric flexure during the Middle Ordovician Blountian phase of the Taconic orogeny resulted in inversion of the graben as a subaerially exposed upthrown block. They cite as evidence for this inversion (1) anomalously thin Knox Group carbonates in the graben area, (2) concurrent thick Knox Group carbonates to the northwest and southeast of the graben, and (3) restriction of upper Middle Ordovician clastic deposits to the southeast of the graben.
In the study area for cross section N–N', only drill hole 202 penetrated a complete stratigraphic interval of Knox Group carbonates. Upper Knox Group carbonates were also identified in drill holes 201, 204, 205, and 208; drill holes 203, 206, and 207 did not encounter Knox Group carbonates. It is possible that inversion of the Birmingham graben also occurred in Bibb County; however, we have no evidence of thinning of the Knox Group because of sparsity of well data in the area.
Potential syndepositional, sedimentary, Neoproterozoic to middle Cambrian (Stage 3, approximately 514 Ma) conglomeratic or brecciated strata may be present adjacent to or near the basement-involved normal fault between the Birmingham graben and the horst block to its southeast below drill hole 205. However, the presence of these strata remains speculative.
Thin-Skinned Structures and Mushwads
The southern Appalachian fold-and-thrust belt in Alabama exhibits many typical thin-skinned structures (bedding-plane detachment zones, footwall ramps, ramp anticlines, and imbricate thrust faults) found in thrust belts worldwide. In addition, a structural feature named a “mushwad” (Malleable, Unctuous SHale, Weak layer Accretion in a Ductile duplex) by Thomas (2001) has been recognized in multiple locations in the Appalachian fold-and-thrust belt of Alabama and Georgia. Mushwads form when a stratigraphically thick layer of weak strata (typically shale) forms a ductile duplex above a basal detachment and below an overriding thrust sheet of “stiff” competent strata (sandstones and [or] carbonates) (Thomas and Pashin, 2011).
The Bessemer mushwad of southeastern Jefferson and Shelby Counties, Alabama, continues to the southwest below the Cretaceous onlap in southeastern Tuscaloosa County, northwestern Bibb County, and possibly northeastern Hale County (Surles, 2007; Thomas and Pashin, 2011) (fig. 6). Below the Bessemer mushwad, there is a basal decollement in shales of the Rome Formation and (or) the lower shaly part of the Conasauga Formation above the Birmingham graben (Thomas and Pashin, 2011) (fig. 7). Within the study area, the upper part of the Conasauga Formation is composed mostly of dolomite. During thrusting in the Pennsylvanian and Permian, the shaly strata were highly contorted and tectonically thickened, forming the Bessemer mushwad below overriding “stiff” competent carbonates of the upper Conasauga Formation through the Knox Group and higher strata in the Jones Valley thrust sheet (Thomas and Pashin, 2011) (fig. 7). At the apex of the Birmingham anticlinorium above the thickest part of the Bessemer mushwad, the contorted shaly strata attained thicknesses of several thousand feet (Thomas and Pashin, 2011) (fig. 7).
In the Marchant 22-16 No. 1 well of northwestern Bibb County (location shown in figs. 1, 2, 5, and 6), near cross section 20 of Surles (2007) (fig. 7; location of cross section 20 is shown in figs. 1, 2, and 6), shale and argillaceous carbonates of the Conasauga Formation exceed 10,600 ft (3,231 m) (Thomas and Pashin, 2011). The Bessemer mushwad disappears in southwestern Bibb County or northeastern Hale County as shown on cross section 21 of Surles (2007) (fig. 7; cross section 21 location shown in figs. 1, 2, and 6; the approximate extent of the mushwad is shown in fig. 6). Note that the location on cross section N–N' where the mushwad thins and terminates is not the southwesternmost point of the Bessemer mushwad. The mushwad termination location shown on N–N' (at mile 56) is about five miles (8 km) south of drill hole 206 (at mile 61) in central Bibb County (fig. 6). The Bessemer mushwad is approximately 40 miles (64 km) long (from northeast to southwest) and 20 miles (32 km) wide (northwest to southeast); only the southwestern part of the Bessemer mushwad is shown in fig. 6.
A few miles northwest of the Bessemer mushwad, the Blue Creek fault rises upsection to where it becomes the northwesternmost thrust fault of the VRPA (figs. 7 and 8). Northwest of the Blue Creek fault, the relatively undisturbed Black Warrior basin is cut by numerous northwest-trending normal faults (some involving basement rocks), generally with minor offsets (Groshong and others, 2010).
The southwestern end of cross section N–N' below drill holes 201 through 203, intersects southeast-to-northwest trending cross sections by Bailey (2007), Pashin and others (2011) (fig. 8), and Robinson and others (2012) that portray a series of thrusts exhibiting ramps, flats, and anticlines. Surles (2007) in his cross section 22 (plate 2) portrays a different interpretation with multiple listric thrusts that gradually steepen toward the surface without forming ramps, flats, or anticlines (fig. 8). On cross section N–N', we show a single listric thrust fault between drill holes 202 and 203 that is the northeastern lateral edge of the Bibb-Scott thrust sheet as shown on plate 1 of Surles (2007). The authors do not endorse Surles’ (2007) interpretation over the alternative interpretations by Bailey (2007), Pashin and others (2011), and Robinson and others (2012). Additional well and seismic data could help to better understand the configuration of thrust faults in this area. Figure 8 shows a comparison between cross section 22 of Surles (2007) and the cross section of Pashin and others (2011).
Also, at the southwest end of N–N′ we show a possible mushwad northeast of the Bibb-Scott thrust fault. This mushwad, with an associated roof thrust, is proposed to account for the abnormally thick Conasauga Formation between wells 201 and 204. Surles (2007) shows a mushwad with a similar configuration enclosed by the northeastern Larkin lateral thrust and the southwestern Bibb-Scott lateral thrust in his along-strike cross section C (plate 2 of Surles, 2007). Our inclusion of this mushwad is presented here only as a possible structural feature and should not be taken as demonstrable interpretation at this time.
The abnormally thick Conasauga Formation in the hanging wall of the Bibb-Scott fault is an unresolved problem. Additional well and seismic data could help to better understand the configuration of thrust faults in this area.
In the upper-central section of cross section N–N', there are two stacked thrust sheets that transport Rome Formation and Copper Ridge and Chepultepec Dolomites over the Pottsville Formation. The GSA sample description log for drill hole 205 calls the lower fault the “Helena fault” and the upper fault the “Rock Creek School fault.” These faults were originally named by Butts (1940) and later recognized by Raymond (1991b). But Thomas and Bayona (2005) and Surles (2007) call the lower and upper faults the “Helena frontal splay fault” and the “Helena fault,” respectively (fig. 7). This discrepancy is explained by Surles (2007) as follows:
“In previous reports (Butts, 1940; Raymond, 1991[b]), the name “Helena” was applied to the thrust fault that bounds the southeast side of the Cahaba synclinorium southwest to the Coastal Plain onlap. The name “Rock Spring School” (sic) was given to the thrust fault and thrust sheet located between the “Helena” fault on the northwest and the Talladega thrust fault on the southeast. In contrast, Thomas and Bayona (2005) noted that the presently named frontal splay of the Helena thrust fault is truncated to the northeast by the presently named Helena thrust fault between cross sections 17 and 18 of their study (also between cross sections 19 and 20 of this study), and the presently named Helena fault is continuous with the mapped Helena fault to the northeast (Szabo and others, 1988). The naming convention of Thomas and Bayona (2005) is used in this study, as it most accurately reflects the available data.
Northeast of the Coastal Plain onlap, the frontal splay of the Helena thrust fault was mapped in outcrop by Butts (1940) as the main Helena thrust fault, and the trace of the thrust fault was confirmed by Surles (unpublished map). Southwest of the Coastal Plain onlap, the frontal splay of the Helena thrust sheet is known through one seismic reflection profile (cross section 20) and one well, well 4583 (Appendix A [of Surles, 2007]), which drilled into the frontal splay of the Helena thrust sheet below the main Helena thrust sheet at a depth of -3,887 ft (-1,185 m).”
So, as described by Surles (2007), the “main” Helena fault of Butts (1940) and Raymond (1991b) is equivalent to the Helena frontal splay fault of Thomas and Bayona (2005) and Surles (2007), and the Rock Creek School fault of Butts (1940) and Raymond (1991b) is equivalent to the Helena fault of Thomas and Bayona (2005) and Surles (2007). We have included two fault labels for each of these two faults on cross section N–N' to prevent any possible confusion for the reader. Hereinafter, in this report, we will follow the naming convention of Butts (1940) and Raymond (1991b) for these two faults and their adjoining thrust sheets.
All of these contractional features resulted from the Late Mississippian to Permian continental collision (Alleghanian orogeny) between eastern North America (Laurentia) and Africa (Gondwana) (Pashin and others, 2011; Pashin and others, 2012). Much of southern Alabama is actually peri-Gondwanan (the Uchee terrane in fig. 4) and Gondwanan (all strata south of the Suwannee-Wiggins suture zone in fig. 4) that remained attached to the North American craton after the rifting of Pangea and the formation of the Atlantic Ocean in the Triassic and Jurassic periods (Steltenpohl and others, 2013).
Stratigraphic Framework and Geologic History
Definition of Terms and References
Although some correlations shown on cross section N–N′ are based on our own interpretations, many correlations are adopted or modified from sample description logs of the GSA and other logs from previous publications. Stratigraphic nomenclature follows existing terminology wherever possible. Useful references for stratigraphic correlations and (or) nomenclature include the following: Thomas (1972, 1991), Davis and others (1975), Reed and others (1975), Raymond and Copeland (1987), Higgins and others (1988), Raymond and others (1988), Szabo and others (1988), and Kopaska-Merkel and others (2005). A correlation chart for the stratigraphic units identified along cross section N–N′ is shown in figure 3.
In general, the stratigraphy on cross section N–N' (fig. 3) follows Butts (1940), Batchelder (1984), Sternbach (1984), Hooks (1985), Raymond and others (1988), Raymond (1991b), Osborne and others (1998), and Osborne and Irvin (2002). The southwestern half of cross section N–N' (the left half) includes drill holes 201 through 204 (in Greene and Hale Counties), whereas the northeastern half of cross section N–N' (the right half) includes drill holes 205 through 208 (in Bibb County). The southwestern half of cross section N–N' follows the stratigraphy of the southwestern end of the western VRPA as described by Raymond and others (1988); the northeastern half of N–N' follows the stratigraphy of the southwestern end of the eastern VRPA as described by Raymond and others (1988). Cambrian and Ordovician formation thicknesses on N–N′ were based on Butts (1940), Mack (1980), Batchelder (1984), Sternbach (1984), Hooks (1985), Osborne and others (1998), and Osborne and Irvin (2002). Please note that the Paleozoic strata of the southwestern half of cross section N–N' are covered by Cretaceous onlap strata. Unless otherwise noted, stratigraphic information in the following sections is based on Raymond and others (1988). Note that no new fossils were collected or identified for this study. All fossil occurrences are based on decades of paleontological research as recorded in the literature (for example, Raymond and others, 1988). Depths to the top of the stratigraphic units discussed in the following sections are listed in table 1.1 of appendix 1 at the end of this report.
Lower to Middle Cambrian Siliciclastic and Carbonate Strata
The lower to middle Cambrian (Terreneuvian to Miaolingian) siliciclastic and carbonate strata in Alabama are characterized by dolomite, limestone, mudstone, shale, siltstone, sandstone, and conglomerate (fig. 3, N–N′ cross section). These siliciclastic and carbonate strata consist of the following units (in ascending order): the lower Cambrian Cochran, Nichols, Wilson Ridge, and Weisner Formations of the Chilhowee Group; the lower Cambrian Shady Dolomite; the lower Cambrian Rome Formation; and the lower to middle Cambrian Conasauga Formation. With the exception of parts of the Rome and Conasauga Formations, all of these formations are too deep to have been encountered in drill holes along cross section N–N′. Descriptions are based on outcrops and drill holes further northeast in the VRPA.
Chilhowee Group
The lower Cambrian (Terreneuvian?) Chilhowee Group (fig. 3) lies unconformably above Mesoproterozoic basement rocks in the Birmingham graben and the downthrown block to its southwest but is most likely absent on adjacent horst blocks. Kidd and Neathery (1976) indicate that the Chilhowee Group thins dramatically and pinches out between northwestern Georgia and the Black Warrior basin in north-central Alabama. In northeastern Alabama, the Chilhowee Group includes four formations (in ascending order): the Cochran, Nichols, Wilson Ridge, and Weisner Formations. It is unclear whether these four formations as described by Mack (1980) in northeastern Alabama extend as far south as our study area. For this reason, on cross section N–N′ and figure 3 we show the Chilhowee Group as a single undivided unit with a total thickness of about 2,500 ft (762 m), based on the sum of the thicknesses of the Cochran, Nichols, Wilson Ridge, and Weisner Formations (233, 410, 1,394, and 492 ft [71, 125, 425 and 150 m], respectively, from Mack [1980]) in the Birmingham graben and the upper level of the downthrown block to its southwest (below drill holes 204, 206, 207 and 208). In the lower level of the downthrown block (below drill holes 202 and 203, and between drill holes 204 and 205), we show Chilhowee Group fill from the basement rocks up to the overlying Shady Dolomite with thicknesses ranging from 2,500 to 4,400 ft (762 to 1,341 m).
The lower Cambrian (Terreneuvian?) metamorphosed (lower greenschist facies) clastic sediments of the Kahatchee Mountain Group, the lowest stratigraphic unit in the Talladega slate belt in Shelby, Chilton, Coosa, Talladega, and Clay Counties, Alabama (fig. 9), are considered the lateral equivalent of the Chilhowee Group, with thicknesses greater than 2,000 m (6,562 ft) (Tull and others, 1988; Johnson and Tull, 2002). The Talladega slate belt is a thrust sheet that was translated more than 62 mi (100 km) on the Talladega-Cartersville fault from a location southeast of the study area to its present location (Johnson and Tull, 2002). Rocks of the Talladega slate belt contain lower greenschist facies metamorphic mineral assemblages representing possible maximum temperatures between 300 and 400 degrees Celsius (572 to 752 degrees Fahrenheit) (Johnson and Tull, 2002).
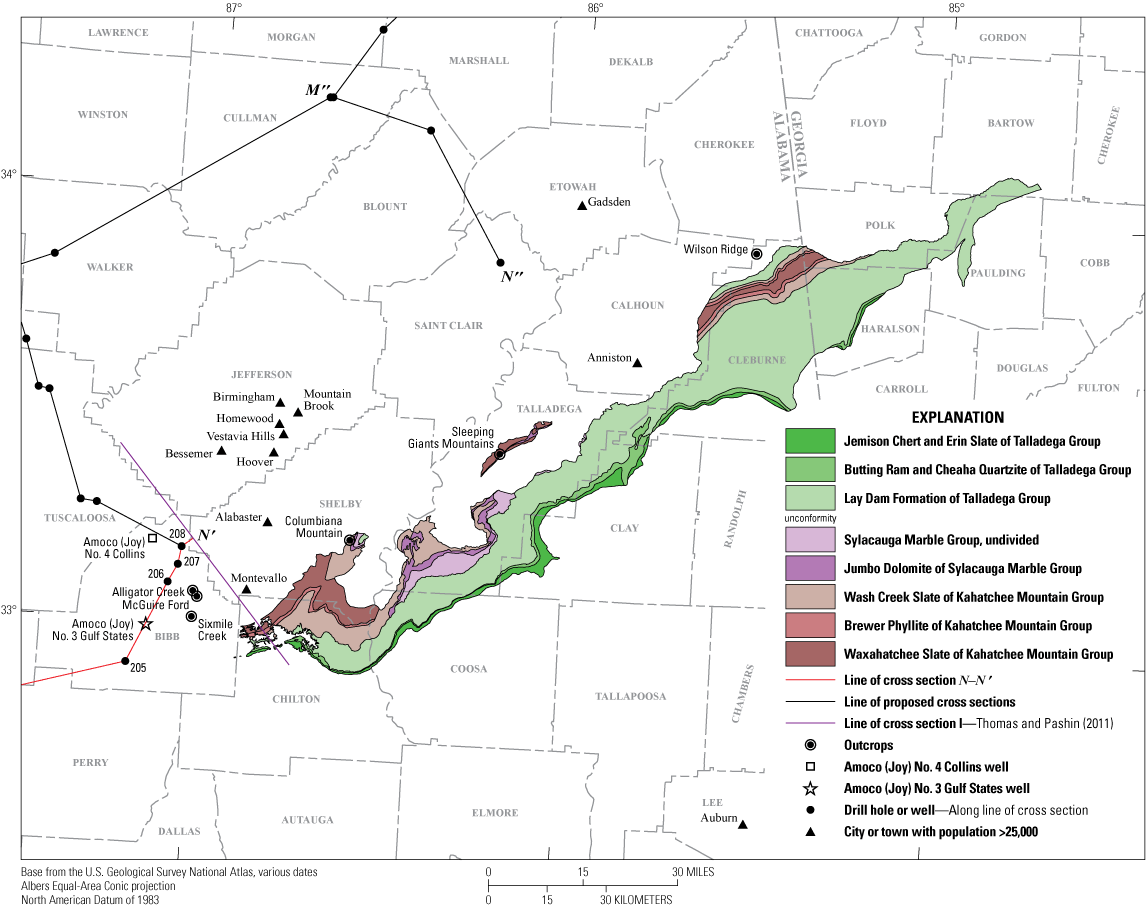
Map of the Talladega slate belt formations and outcrop locations for Chilhowee Group formations described in the report. The map is based on figure 2 from Tull and others (2010). Cross section I of Thomas and Pashin (2011) runs from northwest to southeast from Jefferson to Chilton Counties, Alabama. The surface geologic formations and groups that are not identified with the Talladega Group (as mapped by Tull and others [2010]) are not shown but can be identified in Szabo and others (1988). County boundaries are shown with dashed lines.
Cochran Formation of Chilhowee Group
The lowermost unit of the Chilhowee Group, the lower Cambrian (Terreneuvian?) Cochran Formation lies unconformably above Mesoproterozoic basement rocks of the Grenville province (1.1 to 1.0 Ga; Steltenpohl and others, 2013). The Cochran Formation consists of poorly sorted arkosic sandstone and conglomerate, with interbedded greenish-gray siltstone and mudstone. It crops out in the eastern VRPA in northeastern Alabama. Mack (1980) measured an incomplete section of the Cochran Formation at the Wilson Ridge outcrop in Cleburne County, Alabama, about 6 miles (10 km) east of Piedmont, Alabama (about 100 miles [160 km] northeast of the northeastern end of cross section N–N′; fig. 9) that was 71 m (233 ft) thick. The Cochran Formation was deposited in a fluvial to shallow-marine environment.
Nichols Formation of Chilhowee Group
The lower Cambrian (Terreneuvian?) Nichols Formation of the Chilhowee Group lies conformably above the Cochran Formation of the Chilhowee Group. It is a greenish-gray to black mudstone that is massively bedded to laminated, and micaceous, with minor interbedded siltstone and very fine grained sandstone. Mack (1980) measured a complete section of the Nichols Formation at the Wilson Ridge outcrop (fig. 9) (previously described in the Cochran Formation of Chilhowee Group paragraph) that was 125 m (410 ft) thick. The Nichols Formation crops out in the eastern VRPA in northeastern Alabama. It was deposited in a fluvial to shallow-marine environment.
Wilson Ridge Formation of Chilhowee Group
The next highest unit of the Chilhowee Group is the lower Cambrian (Terreneuvian?) Wilson Ridge Formation, which lies conformably above the Nichols Formation and consists of interbedded quartzose and feldspathic sandstone, and silty mudstone. It crops out in the eastern VRPA in northeastern Alabama. Mack (1980) measured complete sections of the Wilson Ridge Formation at two locations (1) the Sleeping Giants Mountains near Renfroe, Alabama (about 51 miles [82 km] northeast of the northeastern end of cross section N–N′; fig. 9), where it is 425 m (1,394 ft) thick; and (2) the Wilson Ridge outcrop (previously described in the Cochran Formation of Chilhowee Group paragraph) where it was 184 m (604 ft) thick. The Wilson Ridge Formation was deposited in a fluvial to shallow-marine environment.
Weisner Formation of Chilhowee Group
The highest unit of the Chilhowee Group is the lower Cambrian (Terreneuvian?) Weisner Formation which lies conformably above the Wilson Ridge Formation and is composed of interbedded quartzose sandstone and conglomerate, containing minor greenish-gray mudstone. It crops out in the eastern VRPA in northeastern Alabama. Mack (1980) measured a complete section of Weisner Formation with a thickness of 150 m (492 ft) in the Sleeping Giants Mountains outcrop (previously described in the Wilson Ridge Formation of Chilhowee Group paragraph; fig. 9). The Weisner Formation was deposited as an overall transgressive shallow-water-marine sandstone facies (Mack 1980).
Shady Dolomite
The lower Cambrian (Terreneuvian? to Series 2?) Shady Dolomite (fig. 3) lies conformably above the Chilhowee Group. It occurs in the Birmingham graben and the downthrown block to its southwest but is absent on the adjacent horst blocks to the northwest. As with the underlying Chilhowee Group, Kidd and Neathery (1976) indicate that the Shady Dolomite thins and pinches out between northwestern Georgia and the No. 1 F.W. Smith well (drill hole 112) in Cullman County, Alabama (fig. 10). It is composed of limestone and dolomite, with the limestone being bluish to yellowish gray, thick bedded, and partially medium crystalline, and the dolomite being light to dark gray, finely to coarsely crystalline, argillaceous to sandy, siliceous, and massively bedded to laminated. It ranges from 0 ft thick in Cherokee, Etowah, St. Clair, Shelby, and Bibb Counties, Alabama, to over 1,000 ft (305 m) thick in Clay, Cleburne, Talladega, Coosa, and Chilton Counties, Alabama (fig. 10) and it crops out in the eastern VRPA in northeastern Alabama, in central Shelby County, Alabama (Butts, 1940). Outcrop exposures are generally of poor quality exhibiting residual lithologies like sandy clay, limonite, chert, and finely crystalline quartz veins. The Shady Dolomite represents the earliest part of a regionally extensive carbonate bank facies.
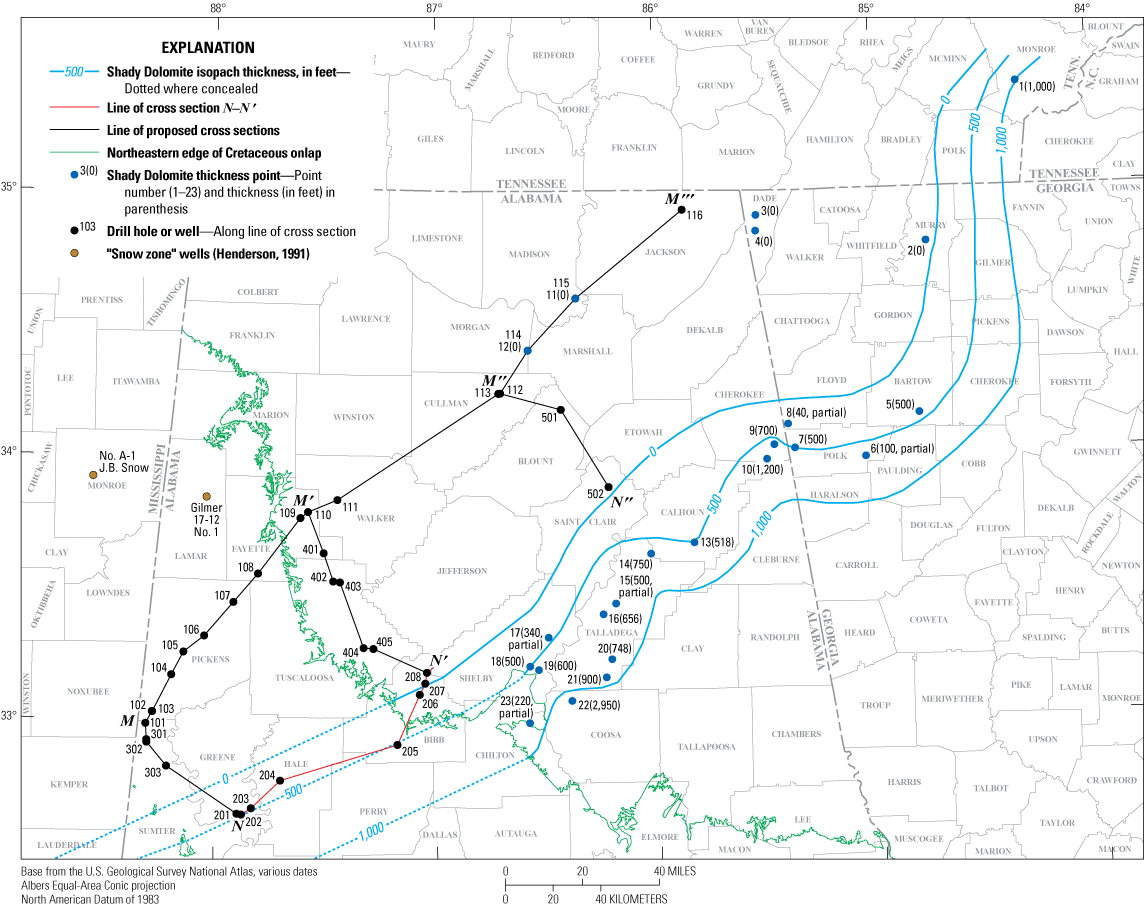
Isopach map of the Shady Dolomite with isopach lines representing thicknesses of 0, 500, and 1,000 feet (0, 152, and 305 meters) in central and eastern Alabama, Georgia, and southern Tennessee. Extensions of the isopachs to the southwest indicate that the Shady Dolomite may be present in Bibb, Hale, Perry, and Greene Counties, Alabama, but the locations of these isopachs beneath the Cretaceous onlap are unknown, thus making the location and thickness of the Shady Dolomite uncertain. Sources of the point thicknesses of the Shady Dolomite are as follows: 1-Tellico Plains, Rodgers (1953); 2-Campground Mountain, Murray County, Ga., Cressler (1974); 3-No. 1 Brown well, Neathery and Copeland (1983); 4-No. 1 Cureton well, Neathery and Copeland (1983); 5-Cartersville, Cressler and others (1979); 6-Crawford Lake, Cressler (1970); 7-Etna, Crawford and Kath (2016); 8-Spring Creek, Cressler (1970); 9-Indian Mountain, Bearce and others (1977); 10-unnamed location in Cherokee County, Ala., Causey (1965); 11-No. 1 Skidmore well, Neathery and Copeland (1983); 12-No. 1 Smith well, Neathery and Copeland (1983); 13-Anniston, McCalley (1897); 14-Estaboga, Osborne (1998); 15-Sleeping Giants Mountains, Bearce and others (2008); 16-Eastern Coosa Valley, Bearce (1978); 17-No. 1 Alabama Property well, Raymond (1991b); 18-Columbiana Mountain, Butts (1940); 19-Beeswax Creek, Adams and others (1926); 20-Tallasseehatchee Creek, Guthrie (1989); 21-Sylacauga, Johnson and Tull (2002); 22-Marble Valley, Prouty (1916); 23-Jumbo, Pendexter (1982). Points 1–23 are present-day locations, not palinspastic locations. The term “partial” means only a partial formation thickness was measured at that location.
The lower Cambrian (Terreneuvian? to Series 2?) Jumbo Dolomite of the Sylacauga Marble Group, which lies conformably above the Kahatchee Mountain Group in the Talladega slate belt in Shelby, Talladega, and Coosa Counties, Alabama (fig. 9) is considered to be equivalent to the Shady Dolomite (Tull and others, 1988; Johnson and Tull, 2002). The Jumbo Dolomite has an average thickness of about 300 m (984 ft), but it can range as high as 600 m (1,969 ft) thick (Johnson and Tull, 2002).
Figure 10 shows the Shady Dolomite (and [or] Jumbo Dolomite) increasing in thickness southeastward from 0 ft in northwestern Bibb, Shelby, St. Clair, Etowah, and Cherokee Counties to greater than 1,000 ft (305 m) in Chilton, Coosa, Talladega, Clay, and Cleburne Counties northeast of the study area. We used thicknesses from 23-point locations in Alabama, Georgia, and Tennessee from 18 publications to locate the isopach lines on the figure 10 map (see fig. 10 caption for the list of point locations and source publications). Southwestward extensions of isopach lines in fig. 10 suggest that the Shady Dolomite may be present in parts of Bibb, Perry, Hale, and Greene Counties, but the precise locations of these isopach lines beneath the Cretaceous onlap is unknown (fig. 10). The Shady Dolomite has not been identified in drill holes shown on cross section N–N'. On cross section N–N′, we show the thickness of the Shady Dolomite ranging from 0 ft (about one mile [1.6 km] northeast of drill hole 206) to about 500 ft (152 m) at locations southwest of drill hole 205 based on the proximity of the 500-ft isopach to drill holes 201 through 205 in fig. 10.
Rome Formation
The lower Cambrian (Series 2) Rome Formation (figs. 2 and 3) lies conformably above the Shady Dolomite in the Birmingham graben and the downthrown block to its southwest. No drill holes reached the Rome Formation in place above the Chilhowee Group formations and Shady Dolomite, but a partial section was encountered in the Rock Creek School thrust sheet at 1,361 ft below kelley bushing (BKB) in drill hole 205. Thomas and others (2001) determined an age for the Rome Formation of between 520 and 510 Ma (Series 2) based on Strontium isotope ratios from anhydrite in the No. 1 John Goodson 9-7 well (drill hole 205 on cross section N–N′) and the No. 1 Alabama Property well in Shelby County, Alabama (point 17 in fig. 10). Kidd and Neathery (1976) indicate that the Rome Formation thins between northwestern Georgia and the Black Warrior basin in north-central Alabama, where it lies unconformably above Precambrian crystalline basement rocks; further to the northwest they show it pinching out entirely in the Interior Highland Rim province of Giles County in southern Tennessee. It consists of red to grayish-green shale that is laminated to thin bedded; interbedded with red, yellow, and brown siltstone and sandstone; light-gray calcareous sandstone; and minor amounts of limestone, dolomite, and anhydrite. The Rome Formation can be found in the VRPA, eastern Appalachian Plateau province, and Black Warrior basin in Alabama. The thickness of the Rome Formation ranges from 290 ft (88 m) in the Black Warrior basin and Appalachian Plateau provinces to a possible maximum thickness of 4,000 ft (1,219 m) in the VRPA.
On cross section N–N′, we show the Rome Formation (not including the mushwad part of the formation) with a thickness of about 1,050 ft (320 m) in the Birmingham graben and the downthrown block to its southwest, and the same thickness in the hanging wall above the Rock Creek School fault based on Osborne and others (1998).
The GSA sample description log for drill hole 205 describes 2,490 ft (759 m) of Rome Formation strata between depths of 150 and 2,640 ft (46 and 805 m) in drill hole 205, but the upper part of this unit is probably Conasauga Formation. Therefore, we placed an arbitrary contact between the Rome and Conasauga Formations at a depth of 1,590 ft (485 m), assigning the lower 1,050 ft (320 m) to the Rome Formation and the upper 1,440 ft (439 m) to the Conasauga Formation.
The Rome Formation was probably deposited in marine-shelf environments, and along with the underlying Chilhowee Group and Shady Dolomite, filled the Birmingham graben and the downthrown block to its southwest during the early Cambrian. Later, during the Alleghanian orogeny a basal decollement formed in the Rome and (or) Conasauga Formations and was involved in the deformation of the ductile shales that formed the contorted mass of shale that is the Bessemer mushwad in the northeastern half of the study area (Surles, 2007; Thomas and Pashin, 2011). At the northeastern end of cross section N–N′, we show the Bessemer mushwad between depths of 10,000 and 16,900 ft (3,048 and 5,151 m) based on cross section I of Thomas and Pashin (2011) where it intersects cross section N–N′. The Bessemer mushwad thins southwestward and is absent on cross section 20 of Surles (2007), which intersects cross section N–N′ at approximately mile 54 (fig. 6); we show the mushwad completely closing at mile 55.
The lower Cambrian (Series 2?) Fayetteville Phyllite of the Sylacauga Marble Group, which lies conformably above the Jumbo Dolomite in the Talladega slate belt in Shelby, Chilton, Coosa, Talladega, and Clay Counties, Alabama (fig. 9), is considered to be the lateral equivalent of the Rome Formation (Tull and others, 1988; Johnson and Tull, 2002), with a thickness of 80 to 290 m (262 to 951 ft) (Johnson and Tull, 2002).
Conasauga Formation
The middle to upper Cambrian (Series 2? to Furongian) Conasauga Formation (figs. 2 and 3) is generally thought to lie conformably above the Rome Formation, but as mentioned previously, Read and Repetski (2012) interpret an unconformity at the top of the Rome Formation. The Conasauga Formation consists of limestone, dolomite, and shale in varying amounts. In the western VRPA, Conasauga Formation limestone is bluish gray, fine grained, thin bedded, and partially argillaceous. In the eastern VRPA, the lower part of the formation is composed of shales and mudstones with limestone and rare siltstone interbeds. The shales and mudstones are dark greenish gray, dusky yellow, or pale olive, and the limestone interbeds are medium to dark gray, thin to medium bedded, micritic, argillaceous, and locally oolitic or oncolitic. The upper part of the formation at the northeastern end of the eastern VRPA (northeast of the study area) is composed of dolostone that is light to dark gray and medium to thick bedded that is laterally equivalent to the Brierfield, Ketona, and Bibb Dolomites at the southwestern end of the eastern VRPA. The upper part of the Conasauga may contain minor amounts of white, light-bluish-gray, or light-brownish-gray chert, that may be cryptocrystalline or oolitic. Locally, the Conasauga can have very fossiliferous intervals. The Conasauga Formation crops out in the VRPA and ranges from 500 ft (152 m) thick in Talladega County, Alabama, to about 2,600 ft (792 m) thick elsewhere. Thomas and Bayona (2005) estimated that the Conasauga Formation may have been up to 6,000 ft (1,829 m) thick in the Birmingham graben before orogenic uplift, erosion, and thrust faulting (Surles, 2007).
Over most of cross section N–N′, we show the Conasauga Formation with a thickness of about 1,500 ft (457 m) based on Butts (1940), but southwest of the Bibb-Scott thrust fault we show the Conasauga Formation with a thickness of greater than 2,020 ft (616 m) based on a partial, faulted section from the GSA sample description log for drill hole 202. This abnormally thick section of the Conasauga Formation shown in the hanging wall of the Bibb-Scott thrust fault is an unresolved problem. Perhaps some of the 2,020 ft (616 m) of the Conasauga Formation in drill hole 202 could be the lower part of the overlying Ketona Dolomite. If there was 1,500 ft (457 m) of Ketona Dolomite southwest of the fault as we show northeast of the fault (the 360 ft [110 m] of strata assigned to the Ketona Dolomite by the GSA sample description log, plus the upper 1,140 ft [347 m] of the Conasauga Formation), that would leave only 880 ft (268 m) of Conasauga in drill hole 202. On cross section N–N′, we show a dashed line and query (?) at the possible location of the contact of the Conasauga Formation and Ketona Dolomite with a 1,500 ft (457 m) thick Ketona Dolomite section as described above. Additional well and seismic data could help to better understand the configuration of thrust faults in this area.
As mentioned previously, we assigned the upper 1,440 ft of strata in the Rock Creek School thrust sheet in drill hole 205 to the Conasauga Formation above an arbitrarily placed contact with the underlying Rome Formation.
The Conasauga Formation was deposited in a marine-shelf environment. In the northeastern half of the study area, a basal decollement formed during the Alleghanian orogeny in the Rome and (or) Conasauga Formations and was involved in the formation of the Bessemer mushwad (Surles, 2007; Thomas and Pashin, 2011). We show the Bessemer mushwad at the northeastern end of cross section N–N′ below the Jones Valley thrust fault and above the basal decollement where it transitions into the upward ramping Blue Creek thrust fault. At the northeastern end of cross section N–N′ where it intersects cross section I of Thomas and Pashin (2011), the Bessemer mushwad is present between depths of about 10,000 and 16,900 ft (3,048 and 5,151 m). To the southwest, the mushwad terminates before mile 54 of cross section N–N′ and cross section 20 of Surles (2007). Another mushwad associated with this decollement may be present at depth at the southwestern end of cross section N–N′ as described earlier in the section “Thin-Skinned Structures and Mushwads.” Our inclusion of this mushwad is presented here only as a possible structural feature and should not be taken as demonstrable interpretation at this time.
The middle to upper Cambrian (Series 2? to Furongian) Shelvin Rock Church Formation of the Sylacauga Marble Group (lower greenschist facies), which lies conformably above the Fayetteville Phyllite in the Talladega slate belt east and north of the study area in Shelby, Chilton, Coosa, Talladega, and Clay Counties, Alabama, (fig. 9) is considered to be the lateral equivalent of the Conasauga Formation, and ranges from 350 to 1,000 m (1,148 to 3,280 ft) thick (Tull and others, 1988; Johnson and Tull, 2002).
Upper Cambrian to Lower Ordovician Carbonate Strata
The upper Cambrian (Furongian) and Lower Ordovician carbonate rocks in the VRPA make up the southern part of a passive continental margin that developed along the east coast of Laurentia (Thomas, 1972; Raymond and others, 1988). The lower part of this carbonate bank facies consists of (in ascending order) the upper Cambrian Brierfield, Ketona, and Bibb Dolomites. The upper part of the carbonate bank consists of formations of the Knox Group including (in ascending order) the upper Cambrian Copper Ridge Dolomite, the Lower Ordovician Chepultepec Dolomite, and the Lower Ordovician Longview and Newala Limestones.
Brierfield, Ketona, and Bibb Dolomites
At the southwestern end of the western VRPA, the Conasauga Formation (figs. 2 and 3) is generally thought to be conformably overlain by the upper Cambrian (Dresbachian to Franconian) Ketona Dolomite, which is laterally equivalent to (in ascending order) the Brierfield, Ketona, and Bibb Dolomites of the southwestern end of the eastern VRPA, but, as mentioned previously, Read and Repetski (2012) interpret an unconformity at the top of the Conasauga Formation. The Brierfield, Ketona, and Bibb Dolomites are in turn laterally equivalent to the upper Cambrian (Dresbachian to Franconian) part of the Conasauga Formation at the northeastern end of the eastern VRPA. Sternbach and Friedman (1984) suggest that the Brierfield, Ketona, and Bibb Dolomites are merely laterally equivalent facies of the upper Conasauga Formation rather than separate formations.
Brierfield Dolomite
The upper Cambrian (Dresbachian) Brierfield Dolomite (figs. 2 and 3) lies conformably above the Conasauga Formation and is composed of bluish-gray dolomite that is coarsely crystalline, thick bedded, and partially siliceous. Butts (1940) described distinctive weathering products of the Brierfield Dolomite including cavernous crusts or fine laceworks of ridges that he used to identify the unit, even when fresh outcrops were unavailable. Upon further dissolution of the dolomite, small to large boulders of chert were left. The weathering of the boulders and crusts produces a residual white chalky powder. The Brierfield Dolomite crops out in the Cahaba River valley (fig. 1) in Shelby County, where it is 1,250 ft (381 m) thick at Sixmile Creek (about 7 miles [11 km] south-by-southeast from drill hole 206) (figs. 1 and 9). Osborne and others (1998) show a thickness of greater than 1,000 ft (305 m) northwest of the Spring Valley fault about 2.5 miles (4.0 km) southwest of Calera, Alabama, but only about 300 ft (91 m) southeast of the fault. On cross section N–N′ (southwest of drill hole 204), we show the Brierfield Dolomite being absent based on the GSA sample description log for drill hole 202. Northeast of drill hole 204, we show the Brierfield Dolomite present as a lateral equivalent of the lower part of the overlying Ketona Dolomite, gradually increasing to a thickness of about 1,250 ft (381 m) northeast of drill hole 205 based on Butts (1940). We also show about 150 ft (46 m) of the Brierfield Dolomite as a single formation at the surface in the Helena thrust sheet at mile 57 based on Szabo and others (1988) (fig. 2).
Ketona Dolomite
The upper Cambrian (Dresbachian to Franconian) Ketona Dolomite (figs. 2 and 3) lies conformably above the Brierfield Dolomite and the Conasauga Formation in the eastern and western parts of the VRPA, respectively. It is composed of light-gray dolomite that is coarsely crystalline, thick bedded, and very pure, with no chert. Reported thicknesses range from about 120 ft (37 m) northwest of the Spring Valley fault about two miles (3.2 km) northeast of Montevallo, Alabama (Osborne and Irvin, 2002), to about 1,000 ft (305 m) in the Shoal Creek and Beaverdam Creek valleys between 5 and 11 miles (8 and 18 km) north of Montevallo, Alabama, and 3 miles (4.8 km) west of Alabaster, Alabama (Butts, 1940) (fig. 9). Osborne and others (1998) show greater than 4,500 ft (1,372 m) of “structurally thickened” Ketona Dolomite in their B–B′ cross section in the Shoal Creek valley about 5 miles (8 km) north of Montevallo, Alabama (fig. 9).
On cross section N–N′ (southwest of the Bibb-Scott thrust fault), we show the Ketona Dolomite with a thickness of 360 ft (110 m) based on the GSA sample description log for drill hole 202. Northeast of the Bibb-Scott thrust fault we show about 1,500 ft (457 m) of Ketona Dolomite. As previously mentioned, it is possible that the upper 1,140 ft (347 m) of the 2,020 ft (616 m) thick Conasauga Formation in drill hole 202 (based on the GSA sample description log) could be Ketona Dolomite. If so, then there would be 1,500 ft (457 m) of Ketona Dolomite and only 880 ft (268 m) of the Conasauga Formation in drill hole 202.
Northeast of drill hole 204, we show the lower and upper parts of the Ketona Dolomite grading laterally into the underlying Brierfield and overlying Bibb Dolomites, respectively. Northeast of drill hole 205, we show the Ketona Dolomite with a thickness of about 1,000 ft (305 m) based on Butts (1940). Based on Szabo and others (1988), we also show about 300 ft (91 m) of the Ketona Dolomite at the surface in the Helena thrust sheet at mile 57 on cross section N–N' (fig. 2).
Bibb Dolomite
The upper Cambrian (Franconian) Bibb Dolomite (figs. 2 and 3) lies conformably above the Ketona Dolomite and consists of dark-gray dolomite that is coarsely crystalline, thick bedded, and siliceous, with chert and irregular cavities. Butts (1940) described chert weathering products of the Bibb Dolomite that were identical to those found in the Brierfield Dolomite and claimed that the Bibb and Brierfield Dolomites would be indistinguishable from each other without the intervening chert-free Ketona Dolomite. Reported thicknesses range from 275 ft (84 m) at the Sixmile Creek outcrop (the same location previously described in the Brierfield Dolomite section of this report; fig. 9) to 500 ft (152 m) near Brierfield, Alabama (about 5 miles [8 km] south-by-southwest of Montevallo) (Butts, 1940). On cross section N–N′ (southwest of mile 36), the Bibb Dolomite is absent based on the GSA sample description log for drill hole 202. Northeast of mile 36, the Bibb Dolomite appears as a lateral equivalent of the upper part of the underlying Ketona Dolomite, gradually thickening from 0 to about 500 ft (0 to 152 m) near drill hole 205, then thinning to about 400 ft (122 m) to the northeast. Unlike the Brierfield and Ketona Dolomites, the Bibb Dolomite is not present at the surface in the Helena thrust sheet at mile 57 on cross section N–N' (Szabo and others, 1988) (fig. 2), even though it crops out about 5 miles (8 km) to the east of this location. We interpret that the Bibb Dolomite was replaced by the laterally equivalent upper Ketona Dolomite in this part of the Helena thrust sheet.
Knox Group
The upper Cambrian (Franconian to Trempealeauan) to Lower Ordovician (Ibexian [Canadian]) Knox Group lies conformably above the Ketona and Bibb Dolomites in the western and eastern parts of the VRPA, respectively. In ascending order, the Knox Group includes the following formations: the Copper Ridge Dolomite, the Chepultepec Dolomite, the Longview Limestone, and the Newala Limestone. These four formations are mappable in much of the VRPA, but it is difficult to identify them in the western VRPA, thus, the term “Knox Group undivided” is often employed there. As previously described, these Knox Group formations along with the underlying Brierfield, Ketona, and Bibb Dolomites comprise the “stiff” competent rocks that were thrust over the underlying contorted, ductile, Cambrian shales of the Bessemer mushwad. Drill hole 202 is the only well on cross section N–N′ that penetrates each of the formations comprising the Knox Group strata, and total Knox Group thickness in drill hole 202 is 5,160 ft (1,573 m).
Copper Ridge Dolomite of Knox Group
The lowest formation in the Knox Group, the upper Cambrian (Franconian to Trempealeauan) Copper Ridge Dolomite (figs. 2 and 3) lies conformably above the Ketona Dolomite in the western VRPA, the Bibb Dolomite at the southwestern end of the eastern VRPA, and the Conasauga Formation at the northeastern end of the eastern VRPA (not shown on cross section N–N'). It is composed of gray dolomite that is thick bedded, medium to coarsely crystalline, and siliceous, with white to yellowish-gray chert. Butts (1940) described distinctive weathering products of the Copper Ridge Dolomite including dense tough chunks and boulders of chert that break down into angular masses and fine angular fragments of chert. Two forms of cryptozoans, a few specimens of cephalopods (Shelbyoceras), and gastropods (Scaevogyra) have been reported in the Copper Ridge Dolomite (Butts, 1940). Reported thicknesses of the Copper Ridge Dolomite range from 954 ft (291 m) in the Amoco (Joy) No. 4 Collins well about 3 miles (4.8 km) northwest of drill hole 208 (Hooks, 1985), and 1,227 ft (374 m) in the Amoco (Joy) No. 3 Gulf States well at mile 54 of cross section N–N′ (Hooks, 1985) to 1,750 ft (533 m) at an outcrop next to Alligator Creek, Alabama, about 4 miles (6.4 km) southeast of drill hole 206 (Butts, 1940; Batchelder, 1984) (fig. 9). On cross section N–N′ (southwest of drill hole 204), we show the Copper Ridge Dolomite with a thickness of 2,080 ft (634 m) based on a top depth of 10,580 ft (3,225 m) from original Amoco drilling records for drill hole 202 (James L. Coleman, Coleman Geological Services, written communication, 1984) and a bottom depth of 12,660 ft (3,859 m) from the GSA sample description log for drill hole 204. Between drill holes 204 and 205, we show the Copper Ridge Dolomite thinning northeastward to a thickness of about 1,750 ft (533 m) (based on Butts, 1940); northeast of drill hole 205 we maintain the 1,750 ft (533 m) thickness. We chose to use the 1,750 ft (533 m) thickness from Butts (1940) instead of the 958 ft (292 m) thickness from Hooks (1985) because Butts’ thickness is based on an outcrop, whereas Hooks’ thickness is based on a core. Also, Batchelder (1984) gives nearly the same thickness as Butts (1940) (1,770 ft [539 m]) at the Alligator Creek outcrop.
In the Helena thrust sheet, we show 720 ft (219 m) of Copper Ridge Dolomite below an arbitrarily placed contact between the Copper Ridge and Chepultepec Dolomites in drill hole 205. The GSA sample description log for drill hole 205 describes 1,480 ft (451 m) of undivided Copper Ridge and Chepultepec Dolomite. We assigned the upper 760 ft (232 m) of this unit to the Chepultepec Dolomite.
The upper Cambrian Gooch Branch Chert of the Sylacauga Marble Group, which lies conformably above the Shelvin Rock Church Formation in the Talladega slate belt in Shelby, Chilton, Coosa, Talladega, and Clay Counties, Alabama (fig. 9), is considered to be the lateral equivalent of the Copper Ridge Dolomite (Tull and others, 1988; Johnson and Tull, 2002). The Gooch Branch Chert ranges from 820 to 1,969 ft (250 to 600 m) thick (Johnson and Tull, 2002).
Chepultepec Dolomite of Knox Group
The next highest formation in the Knox Group is the Lower Ordovician (Ibexian [Canadian]) Chepultepec Dolomite (figs. 2 and 3), which lies conformably above the Copper Ridge Dolomite of the Knox Group. Raymond and others (1988) describe two members in the Chepultepec Dolomite: (1) a lower member composed of gray limestone that is compact and thick bedded, with gastropods and dolomite interbeds, and (2) an upper member composed of bluish-gray dolomite that is medium to coarsely crystalline, thin to thick bedded, with cavernous and fossiliferous chert. Butts (1940) described distinctive weathering products of the Chepultepec Dolomite that he used to differentiate the formation from the Copper Ridge Dolomite, including (1) mealy-textured chert exhibiting irregular “worm-eaten” cavities, (2) low-chert density and resistance resulting in very few large boulders, and (3) chalky-textured final breakdown products. The Chepultepec Dolomite includes a considerable collection of fauna, mainly gastropods, but also cephalopods, one trilobite species, one archaeocyathid species, and one radiolarian species, which are generally found in the chert (Butts, 1940). Reported thicknesses of the Chepultepec Dolomite range from 1,100 ft (335 m) at the Alligator Creek outcrop (previously described in the Copper Ridge Dolomite section of this report; fig. 9) (Butts, 1940) to 1,520 ft (463 m) also at the Alligator Creek outcrop (Batchelder, 1984), but the GSA sample description log for drill hole 202 describes a measured total thickness of 1,330 ft (2,140 m) divided between an upper dolomite member (650 ft [198 m]), and a lower limestone member (680 ft [207 m]). On cross section N–N' (southwest of drill hole 204), we show the Chepultepec Dolomite with a thickness of 1,970 ft (600 m) based on top and bottom depths of 8,610 ft (2,624 m) and 10,580 ft (3,225 m) from original Amoco drilling records for drill hole 202 (James L. Coleman, Coleman Geological Services, written communication, 1984). Between drill holes 204 and 205, we show the Chepultepec Dolomite thinning northeastward to a thickness of 1,440 ft (436 m) based on a top depth of 9,340 ft (2,847 m) for “Chepultepec and Copper Ridge Dolomites undifferentiated” in the GSA sample description log for drill hole 205 and an arbitrary bottom depth of the Chepultepec Dolomite that we placed at 10,780 ft (3,286 m). Northeastward from drill hole 205, we show the Chepultepec Dolomite thickening very gradually towards drill hole 208, where at depths between 7,170 and 8,665 ft (2,185 and 2,641 m), the mud log passes through 1,495 ft (456 m) of predominantly chert and dolomite with very little limestone and clastics, which we interpret as Chepultepec Dolomite with no lower limestone member. We suggest that the lower limestone member of the Chepultepec Dolomite pinches out by facies change below the upper dolomite member between drill holes 204 and 205 and is absent northeast of drill hole 205. As mentioned previously, in the Helena thrust sheet, we show 760 ft (232 m) of Chepultepec Dolomite above an arbitrarily placed contact between the Copper Ridge and Chepultepec Dolomites in drill hole 205.
The Lower Ordovician Gantts Quarry Formation of the Sylacauga Marble Group, which lies conformably above the Gooch Branch Chert in the Talladega slate belt in Shelby, Chilton, Coosa, Talladega, and Clay Counties, Alabama (fig. 9), is considered to be the lateral equivalent of the Chepultepec Dolomite and Longview and Newala Limestones (Tull and others, 1988; Johnson and Tull, 2002). The Gantts Quarry Formation is about 1,640 ft (500 m) thick (Johnson and Tull (2002).
Longview Limestone of Knox Group
The third highest formation in the Knox Group is the Lower Ordovician (Ibexian [Canadian]) Longview Limestone (figs. 2 and 3). It is composed of light-gray limestone and dolomite that is cherty and sandy, thick bedded, and finely to medium crystalline. The chert is brittle, compact, and fragile, and weathers to small fragments. The Longview Limestone contains scattered Lecanospira gastropod fossils. Its average thickness in the VRPA is about 500 ft (152 m) (Raymond and others, 1988). On cross section N–N', we show “Longview and Newala Limestones, undivided” with thicknesses of 1,110 ft (338 m) in drill hole 202 based on a formation top at 7,500 ft (2,286 m) below kelly bushing (BKB) from the GSA sample description log and a formation bottom at 8,610 ft (2,624 m) BKB from original Amoco drilling records (James L. Coleman, Coleman Geological Services, written communication, 1984) and 120 ft (37 m) in drill hole 205 (based on the GSA sample description log). The GSA sample description log for drill hole 205 shows the Middle to Upper Ordovician Chickamauga Limestone resting unconformably on top of the Chepultepec Dolomite with the Longview and Newala Limestones absent. The omission of the Longview and Newala Limestones in drill hole 205 appears to indicate that the two formations were completely removed at the regional post-Knox unconformity (see discussion in the Post-Knox Unconformity section of this report). On cross section N–N', we chose to show the Longview and Newala Limestones as pinching out below the post-Knox unconformity between drill holes 204 and 205, but it is possible that they may be present to the northeast of drill hole 205 in the lower part of a limestone unit shown in the mud log of drill hole 208 between 6,520 and 7,170 ft (2,003 and 2,185 m) that we interpret as the Chickamauga Limestone. For simplicity, we have labelled this limestone unit as Chickamauga Limestone, but we note on the cross section that this unit may also include Newala and Longview Limestones. We show the Longview Limestone (fig. 2) as a single formation with an estimated thickness of about 100 ft (30 m) in the Helena thrust sheet at the surface between miles 53 and 55 based on the geologic map of Alabama (Szabo and others, 1988).
As described previously in the Chepultepec Dolomite section of this report, the Lower Ordovician Gantts Quarry Formation of the Sylacauga Marble Group in the Talladega slate belt (fig. 9) is considered to be the lateral equivalent of the Chepultepec Dolomite and Longview and Newala Limestones (Johnson and Tull, 2002; Tull and others, 1988).
Newala Limestone of Knox Group
The fourth and highest formation in the Knox Group is the Lower Ordovician (Ibexian [Canadian]) Newala Limestone (figs. 2 and 3). It is composed of dark-, light-, and bluish-gray limestone that is compact, noncrystalline to textureless, thick bedded, and micritic or peloidal, with some finely to medium crystalline dolomite. It ranges from 200 to 1,000 ft (61 to 305 m) thick in the VRPA (see the Longview Limestone section of this report for the discussion of the “Longview and Newala Limestones, undivided” that we show on cross section N–N'). The top of the Newala Limestone is truncated by the regional post-Knox unconformity that is recognized throughout much of the Appalachian basin. In the eastern VRPA, the unconformity generally overlies the Odenville Limestone Member of the upper part of the Newala Limestone (Raymond, 1991a) (not shown in fig. 3 or cross section N–N'). On cross section N–N', we also show the Newala Limestone as a single formation above the Longview Limestone in the Helena thrust sheet between miles 53 and 55 based on Szabo and others (1988). As previously described for the Longview Limestone, the Newala Limestone is depicted on cross section N–N' as pinching out below the post-Knox unconformity between drill holes 204 and 205, and absent northeast of drill hole 205, but it is possible that the two limestones may be present to the northeast of drill hole 205 as the lower part of the limestone unit in drill hole 208 that we interpret as the Chickamauga Limestone.
As previously described in the Chepultepec Dolomite section of this report, the Lower Ordovician Gantts Quarry Formation of the Sylacauga Marble Group in the Talladega slate belt (fig. 9) is considered to be the lateral equivalent of the Chepultepec Dolomite and Longview and Newala Limestones (Tull and others, 1988; Johnson and Tull, 2002).
Post-Knox Unconformity
As previously described, the regionally extensive post-Knox unconformity is shown above the Newala Limestone in figure 3 and extends as deep as the Chepultepec Dolomite in the northeastern half of cross section N–N' below drill holes 205 through 208. The unconformity spans from the end of the Early Ordovician (Ibexian [Canadian]) through the Middle Ordovician (Whiterockian and Chazyan), and possibly as late as the Late Ordovician (Blackriveran) at the southwestern end of the VRPA (Raymond and others, 1988; Raymond, 1991a) (fig. 3). The amount of erosion was greatest at the southwestern end of the western VRPA with truncation of up to hundreds of feet of the Newala Limestone, Longview Limestone, and Chepultepec Dolomite (Raymond, 1991a). Paleokarst depressions in the Newala, Longview, and Chepultepec are locally filled with the overlying Attalla Chert Conglomerate Member at the base of the Chickamauga Limestone (Raymond, 1991a). Dwyer and Repetski (2012) describe extensive secondary porosity development (including solution pipes), solution-collapse breccias, and erosional truncation of dolomites below the post-Knox unconformity. Fritz and others (2012) describe “paleokarst collapse breccias occurring 3,000 ft (914 m) below the top of the Knox,” suggesting that some breccias may have formed along other unconformities within the Knox Group.
Unfortunately, the post-Knox unconformity is not easily recognized in the subsurface where the Attalla Chert Conglomerate Member of the Chickamauga Limestone is missing, thin, or without a significant thickness of erosional residuum that is detectable on wireline logs. The apparent absence of the Attalla Chert Conglomerate Member in the drill holes of N–N′ may indicate that the period of subaerial erosion was too short to create a detectable unconformity using conventional wireline log tools. Well logs examined for this study displayed no clearly identifiable interval or erosional surface, and the GSA sample description logs for drill holes 202, 204, and 205 showed no obvious lithologic markers to indicate the presence of an unconformity.
The post-Knox unconformity has been explained by some authors as the result of the collision of a volcanic arc with the east coast of Laurentia (Mussman, 1982; Read and Repetski, 2012), but Hatcher and Repetski (2007) propose that it may reflect a global sea-level drop that took place during the Middle Ordovician (Whiterockian). As previously mentioned in the Structural Framework section of this report, Bayona and Thomas (2003) describe inversion of the Birmingham graben boundary faults resulting in subaerial exposure and erosion of Knox Group carbonates during the Middle Ordovician Taconic orogeny.
A regional unconformity overlies the Gantts Quarry Formation of the Sylacauga Marble Group, and erodes as deep as the Kahatchee Mountain Group in the Talladega slate belt east and north of the study area in Shelby, Chilton, Coosa, Talladega, and Clay Counties, Alabama (fig. 9). It is considered to be the lateral equivalent of the post-Knox unconformity (Tull and others, 1988; Johnson and Tull, 2002).
Middle to Upper Ordovician Siliciclastic and Carbonate Strata
The Middle to lower Upper Ordovician siliciclastic and carbonate formations (the Chickamauga and Lenoir Limestones, Athens Shale, and Sequatchie Formation) record the transition from passive margin shelf carbonates of the Brierfield through Newala formations to argillaceous carbonates and clastics of the foreland basin on the east side of Laurentia during the Taconic orogeny of the Middle to Late Ordovician (Bayona and Thomas, 2003). The source of the clastics was highlands of the Taconic orogen to the northeast in eastern Tennessee. The Little Oak Limestone, Colvin Mountain Sandstone, and Greensport Formation overlie the Athens Shale at the northeastern end of the eastern VRPA closer to the Taconic orogen, but these formations are not found in the study area at the southwestern end of the VRPA. Please note that Raymond and others (1988) considered the Porterfieldian through Shermanian stages to be Middle Ordovician, whereas Harland and others (1990) and Cohen and others (2013) considered these stages to be Upper Ordovician. This report recognizes these stages as Upper Ordovician following the practice of the Harland and others (1990) and Cohen and others (2013).
Chickamauga Limestone
In the western VRPA, the Middle to Upper Ordovician (Chazyan to Shermanian) Chickamauga Limestone (figs. 2 and 3) lies unconformably on top of the Knox Group which was truncated by the post-Knox unconformity as previously described (Raymond and others, 1988; Raymond, 1991a). The Chickamauga Limestone is composed of medium- to dark-gray limestone that is thick to thin bedded, sometimes argillaceous or silty, micritic to medium grained, with locally abundant fossil horizons and thin dolomite beds near the top and bottom of the unit. It crops out in the western VRPA, and its thickness ranges from 260 to 1,100 ft (79 to 335 m). On cross section N–N′, we show the Chickamauga Limestone with measured thicknesses (from GSA sample description logs and [or] gamma-ray logs) of 443, 476, 557, and 1,040 ft (135, 145, 170, and 317 m) in drill holes 201, 202, 204, and 205, respectively. The GSA sample description log for drill hole 205 shows the Chickamauga Limestone lying directly above the Chepultepec Dolomite, without Longview or Newala Limestones intervening. For drill hole 208, we interpret the limestone shown on the mud log at depths between 6,520 and 7,170 ft (1,987 and 2,585 m) as the Chickamauga Limestone, but it is possible that the lower part includes Newala and Longview Limestones because they are difficult to differentiate. At the northeastern end of the cross section, we show the Chickamauga Limestone with an estimated thickness of about 600 ft (183 m). Locally, at the base of the Chickamauga Limestone, the Attalla Chert Conglomerate Member fills paleokarst depressions in the Knox Group as previously described (Raymond and others, 1988; Raymond, 1991a). The Attalla member is a conglomerate composed of subangular to rounded pebbles, cobbles, and boulders of chert and occasionally dolomite or quartzite, within a matrix of chert, quartz sand, and clay. It sometimes grades into a medium- to coarse-grained sandstone and may feature greenish-gray and red shale lenses or beds at its base. The thickness of the Attalla Chert Conglomerate Member ranges from 0 to 110 ft (0 to 34 m) in the western VRPA.
Henderson (1991) discussed the “Snow zone,” a zone of high porosity in the Middle to Upper Ordovician Stones River Group (laterally equivalent to the Chickamauga Limestone) that was associated with production of a small amount of oil and gas from the Magnolia Petroleum No. A-1 J.B. Snow well in Monroe County, Mississippi (fig. 10). The Anderman-Smith No. 4 Gilmer well (currently named Gilmer 17-12 No. 1) in Lamar County, Alabama, also produced a small amount of oil and gas from “Snow zone” strata (Henderson, 1991). Henderson (1991) interprets the “Snow zone” as a zone of brecciation created as the result of a widespread regression and karstification event in the early Middle Ordovician. Similar brecciated dolomite zones may be recognizable in density porosity logs from the Chickamauga Limestone in drill holes 202, 204, and 205, but lacking detailed correlations, we cannot be certain they are equivalent to the “Snow zone” of northeastern Mississippi. Fritz and others (2012) state that a “lack of markers makes it difficult to distinguish the upper Knox dolomites from the Snow dolomite” [lower Stones River Group in the Black Warrior basin, which is equivalent to the Chickamauga Limestone].
In drill hole 205 at a depth of 8,767 ft (2,672 m), a gamma-ray spike exceeding 100 API (American Petroleum Institute) units may indicate the presence of the T-4 bentonite, which is identified by the GSA sample description log. A similar gamma-ray spike is found in drill hole 208 at a depth of 6,978 ft (2,127 m). The T-4 bentonite (also known as the Millbrig or Mud Cave bentonite) has been used to correlate Upper Ordovician strata across much of North America (Huff and Kolata, 1990; Haynes, 1994; Bergstrom and others, 2004). Using graptolite zones, the Millbrig bentonite has been placed in the earliest Chatfieldian (Rocklandian) Stage (Huff and Kolata, 1990; Haynes, 1994; Bergstrom and others, 2004). Unfortunately, the mud log for drill hole 208 does not describe bentonitic clays within the limestone unit.
Lenoir Limestone
In the eastern VRPA, the Middle Ordovician (Whiterockian to Chazyan) Lenoir Limestone (figs. 2 and 3), a lateral equivalent of the Chickamauga Limestone, lies unconformably on top of the Knox Group, which was truncated by the post-Knox unconformity as previously described (Raymond and others, 1988; Raymond, 1991a). It is composed of dark-gray limestone that is very argillaceous, medium to thick bedded, evenly bedded, finely crystalline, and fossiliferous, with thin irregular clay partings and fine-grained impurities that remain as anastomosing bands after weathering. Locally in Alabama, at the base of the Lenoir Limestone is the Middle Ordovician (Whiterockian) Mosheim Limestone Member (not shown on cross section N–N'), which is a medium- to dark-gray lime mudstone that is thick bedded to massive with abundant fenestrae. Its thickness ranges from 0 to more than 200 ft (61 m) in the eastern VRPA. Below the Mosheim Member the post-Knox unconformity spans only about 10 million years (Whiterockian) (Raymond, 1991a). On cross section N–N', we show the Lenoir Limestone above the Newala Limestone in the Helena thrust sheet at the surface between miles 54 and 55 of the cross section based on its presence on the geologic map of Alabama (Szabo and others, 1988) (fig. 2). We interpret that the Helena thrust sheet transported the Lenoir Limestone and several other formations into the study area from the southeast in the eastern VRPA. The stratigraphic position of the Lenoir Limestone is shown in figure 3.
Athens Shale
The Middle to Upper Ordovician (Chazyan to Porterfieldian) Athens Shale overlies the Lenoir Limestone (figs. 2 and 3). It is a black, graptolitic, fissile shale that contains dark-gray to black limestone interbeds in its lower part. The Athens Shale ranges from 0 to 300 ft (0 to 91 m) thick in outcrop and is found mainly at the southwestern end of the eastern VRPA. Based on the geologic map of Alabama (Szabo and others, 1988) we show the Athens Shale above the Lenoir Limestone in the Helena thrust sheet at the surface between miles 54 and 55 of cross section N–N′ (fig. 2). We interpret that the Helena thrust sheet transported the Athens Shale and several other formations such as the underlying Lenoir Limestone into the study area from the southeast in the eastern VRPA. The stratigraphic position of the Athens Shale is shown in figure 3.
Sequatchie Formation
At the southwestern end of the western VRPA (our study area location), the Upper Ordovician (Edenian) Sequatchie Formation (fig. 3) conformably overlies the Chickamauga Limestone. It is composed of reddish-gray, green, and yellow mudstone that is calcareous and laminated with limestone that is medium to coarsely crystalline and fossiliferous. In places, it is glauconitic, arenaceous, and silty, and may include yellowish-gray and greenish-brown shale that is thinly bedded and calcareous, or may include argillaceous limestone. Thickness ranges from 0 to 200 ft (61 m) in the western VRPA. GSA sample description logs for drill holes 202 and 204 describe shales above the Chickamauga Limestone that are 560 and 390 ft (171 and 119 m) thick, respectively, that may be of Late Ordovician age, but GSA sample description logs do not identify these strata as the Sequatchie Formation. The GSA sample description log for drill hole 205 shows no Ordovician strata above the Chickamauga Limestone. We do not show the Sequatchie Formation on cross section N–N', even though it is known to exist in the western VRPA. It is possible that the lower part of the overlying Silurian Red Mountain Formation in drill hole 205 may include the Sequatchie Formation, but the GSA did not identify it as such. We have followed Pashin and others (2011) who consider these strata to be Silurian, and do not include the Sequatchie Formation in our cross section.
Silurian to Middle Mississippian Siliciclastic and Carbonate Strata
The Silurian to Middle Mississippian siliciclastic and carbonate formations (Red Mountain Formation, Frog Mountain Sandstone [possibly including Devonian chert], Chattanooga Shale, Maury Shale, Fort Payne Chert, and Tuscumbia Limestone) (figs. 2 and 3) record (1) clastic sedimentation related to the late Taconic orogeny in the Silurian, (2) clastic sedimentation of the Acadian orogeny in the Devonian and Lower Mississippian, and (3) post-Acadian carbonate deposition in the Lower to Middle Mississippian (Osagean and Meramecian). The source of the clastic sediments shifted from eastern Tennessee during the Silurian part of the Taconic orogeny to the Acadian highlands southeast of the study area during the Devonian and Lower Mississippian. As described by Pashin and others (2011), a thick (greater than 1,500 ft [457 m]) clastic wedge consisting of Silurian and Devonian strata, including black shales, accumulated within the Greene-Hale synclinorium, then thought to be a potentially important shale gas resource. The southeastward-thickening clastic wedge likely extends into northern Perry and southern Bibb Counties as evidenced by thick Silurian and Devonian strata in the Krout 10-14 No. 1 well (Ben Byerly, Geological Survey of Alabama, written communication, 2022) (fig. 1). As the southeastern limb of the synclinorium has not been delineated, we will use the name Greene-Hale-Perry-Bibb depositional margin from this point forward (fig. 1). Five of the eight wells used to make cross section N–N' (drill holes 202–205 and 208) were included on cross section B–B′ of plate 1 in Pashin and others (2011).
The Silurian(?) to Lower Mississippian(?) Talladega Group, which lies unconformably above the Gantts Quarry Formation of the Sylacauga Marble Group in the Talladega slate belt east and north of the study area in Shelby, Chilton, Coosa, Talladega, and Clay Counties, Alabama (fig. 9), is considered to be the lateral equivalent of Silurian to Lower Mississippian formations in the Appalachian fold and thrust belt (Tull and others, 1988; Johnson and Tull, 2002). It is greater than 2,500 m (8,202 ft) thick and includes the lower Lay Dam Formation (metamorphosed shale, sandstone, and conglomerate), the middle Butting Ram and Cheaha Quartzite (metamorphosed sandstone and conglomerate), and the upper Jemison Chert and Erin Slate (metamorphosed chert, black slate, and siliceous argillite) (fig. 9).
Red Mountain Formation
At the southwestern end of the VRPA, the regional Cherokee unconformity at the Ordovician-Silurian boundary (Swezey, 2002) above the Sequatchie Formation is overlain by the Silurian (Llandovery, Wenlock, and Pridoli [with a Ludlow hiatus]) Red Mountain Formation (Ferrill, 1984; Berdan and others, 1986; Chowns and Rindsberg, 2015) (fig. 3). The Red Mountain Formation contains six members that include four iron-ore beds that were important to the steel industry of the late 19th to middle 20th century in Bessemer and Birmingham, Alabama (Chowns and Rindsberg, 2015). The formation consists of interbedded sandstones, shales, and limestones, some containing large amounts of hematite (Chowns and Rindsberg, 2015). Note that the two upper members of the Red Mountain Formation (the Rocky Row and Sparks Gap Members) were originally mapped as the lower part of the overlying Devonian Frog Mountain Sandstone but were reassigned to the Red Mountain Formation based on paleontology (Ferrill, 1984; Berdan and others, 1986). The Sparks Gap Member is mainly limestone and thickens to about 98.4 ft (30 m) at the southwestern end of the VRPA.
The GSA describes “Silurian undifferentiated” strata in their sample description logs for drill holes 202 and 204. The GSA sample description log for drill hole 202 shows the top of the “Silurian undifferentiated” strata at a depth of 6,330 ft (1,929 m), whereas Pashin and others (2011) show it at 6,577 ft (2,005 m) (see cross section B–B′ on plate 1 of Pashin and others, 2011). On cross section N–N′ in drill hole 202, we follow Pashin and others (2011) and place the top of the Red Mountain Formation at 6,577 ft (2,005 m). In drill hole 204, the GSA sample description log shows the top of “Silurian undifferentiated” strata at 10,359 ft (3,157 m), whereas Pashin and others (2011) show it at 10,300 ft (3,139 m). On cross section N–N′ in drill hole 204, we follow the GSA sample description log and place the top of the Red Mountain Formation at 10,359 ft (3,157 m).
The GSA sample description log identifies Silurian strata in drill hole 205 as the “Red Mountain Formation” with its top at 8,070 ft (2,460 m) (with intentional use of quotation marks), which probably indicates that the limestone-dominated lithology is considered unusual for the Red Mountain Formation, or possibly indicates that the unit may include the underlying Sequatchie Formation and the overlying Frog Mountain Sandstone. Pashin and others (2011) show the Red Mountain Formation as limestone and dolomite in drill hole 205 with its top at about 8,050 ft (2,454 m) (see cross section B–B′ on plate 1 of Pashin and others, 2011). On cross section N–N′ in drill hole 205, we follow the GSA sample description log and place the top of the Red Mountain Formation at 8,070 ft (2,460 m).
In drill hole 208, Pashin and others (2011) show the Red Mountain Formation as carbonate strata above and below a middle shaley unit. On cross section N–N′ in drill hole 208, we follow Pashin and others (2011) with the 202 ft (62 m) of Red Mountain Formation between 6,318 and 6,520 ft (1,926 to 1,987 m). Tim Chowns (University of West Georgia, written communication, 2022) measured 312.3 ft (95 m) of Red Mountain Formation in a roadside outcrop at Tannehill State Park, Alabama, about 5 miles (8 km) northwest of the northeastern end of cross section N–N′.
The Red Mountain Formation was deposited in shallow-marine shelf and shoreface environments (Chowns and Rindsberg, 2015). According to Raymond and others (1988), thickening of the unit to the northeast appears to indicate that the source of the clastic sediments was the same Taconic highlands that sourced the Ordovician clastics. However, thickening of Silurian sediments in the Greene-Hale-Perry-Bibb depositional margin should not be taken as an indication of a sediment source to the southwest. On cross section N–N′ in drill holes 202 and 204, we show the Red Mountain Formation with thicknesses of 447 and 614 ft (138 and 189 m), respectively. Formation tops for drill holes 202 and 204 are based on Pashin and others (2011) and GSA sample description logs, respectively. Formation bottoms for both drill holes are based on gamma-ray logs. Pashin and others (2011) depict the Red Mountain Formation as having thicknesses of about 450 and 700 ft (137 and 213 m) in drill holes 202 and 204, respectively, in the Greene-Hale-Perry-Bibb depositional margin. For drill hole 205 to the northeast, we identify 230 ft (70 m) of the Red Mountain Formation based on the GSA sample description log while Pashin and others (2011) show only about 130 ft. Pashin and others (2011) show the presence of up to six radioactive shale units in drill holes 202 through 204 (labeled and shown as green horizontal lines to the right of gamma-ray curves for these drill holes on cross section N–N'). Tim Chowns (University of West Georgia, written communication, 2022), Ben Byerly (Geological Survey of Alabama, written communication, 2022), and the authors of this report doubt that there are black shales in the Red Mountain Formation in this part of Alabama. The intervals of the Red Mountain Formation as observed on outcrop in Alabama and Georgia were probably not deposited in environments conducive for anoxic black shale deposition. Perhaps the contact between the Red Mountain Formation and the overlying Frog Mountain Sandstone should be placed below the lowest black shales. However, another possibility is that the depositional environment of the Red Mountain Formation was more anoxic along the Greene-Hale-Perry-Bibb depositional margin than in locations to the north and west where these black shales are not found in the formation. More information about potential oil and gas in black shales of the Greene-Hale-Perry-Bibb depositional margin is contained in the last paragraph in the Frog Mountain Sandstone section of this report.
Frog Mountain Sandstone (Alone and Included in Undivided Devonian Formations)
The Lower to Middle Devonian (Ulsterian to Erian) Frog Mountain Sandstone (fig. 3) was deposited on an unnamed upper Silurian (Cayugan) unconformity above the Silurian and Ordovician rocks previously described in the VRPA. It is composed of gray to brown weathered sandstone that is fine to coarse grained, argillaceous, and medium bedded; gray subarkosic sandstone that is medium to coarse grained, silica-cemented, medium bedded, and cherty, with rare fossiliferous pods; and gray chert (Berdan and others, 1986). Note that the lower part of the Frog Mountain Formation as originally defined was reassigned to the Red Mountain Formation based on paleontology (Ferrill, 1984; Berdan and others, 1986). The Frog Mountain Formation is present in the VRPA and its thickness ranges from 0 to 213 ft (0 to 65 m) (Raymond and others, 1988). Tim Chowns (University of West Georgia, written communication, 2022) measured 12.9 ft (3.9 m) of Armuchee Chert in a roadside outcrop at Tannehill State Park, Alabama, about 5 miles (8 km) northwest of the northeastern end of cross section N–N′. In drill hole 205, the GSA sample description log identifies 60 ft (18 m) of argillaceous cherty limestone between 8,010 and 8,070 ft (2,442 and 2,460 m) as the Frog Mountain Sandstone. From drill hole 201 to mile 44 on cross section N–N′ and in figure 3, we show the Frog Mountain Sandstone and the Chattanooga Shale together in a unit called “undivided Devonian formations” ranging from a maximum of 1,535 ft (468 m) in drill hole 202 to a minimum of 200 ft (61 m) at mile 44. Northeast of mile 44 on cross section N–N′, we show the Frog Mountain Sandstone and the Chattanooga Shale as individual units separated by an unconformity. Unlike the Ordovician and Silurian sediments previously discussed, the clastics of the Frog Mountain Sandstone probably came from a source southeast of the study area, which may indicate a shift in the location of the orogenic highlands southward during the Acadian orogeny.
Pashin and others (2011) show the Chattanooga Shale and Frog Mountain Sandstone in drill hole 208 with thicknesses of about 10 and 20 ft (3.0 and 6.1 m), respectively (see cross section B–B' on plate 1 of Pashin and others, 2011). In drill hole 205, the Chattanooga Shale and Frog Mountain Sandstone are about 40 and 60 ft (12.2 and 18.3 m) thick, respectively (see cross section B–B' on plate 1 of Pashin and others, 2011). Southwest of drill hole 205, Pashin and others (2011) show these two formations greatly increasing in thickness as a single undivided unit of Devonian strata in the Greene-Hale-Perry-Bibb depositional margin ranging between about 1,150 and 1,550 ft (351 and 472 m) thick in drill holes 204 and 202, respectively (on cross section N–N′, we show them as 1,159 and 1,535 ft, based on gamma-ray logs). It is not clear on the Pashin and others (2011) cross section which parts of this undivided unit are equivalent to the Chattanooga Shale and the Frog Mountain Sandstone. In drill hole 204, the lower one-third of the unit, which is mostly gray shale and limestone, may be equivalent to the Frog Mountain Sandstone, the middle black-shale-rich one-third may be equivalent to the Chattanooga Shale, and the upper gray-shale-rich one-third may be an unnamed overlying formation that is not present in drill hole 205 (see cross section B–B' on plate 1 of Pashin and others, 2011). In drill holes 202 and 203, the lower one-third contains black shale as well as gray shale and limestone. An alternative interpretation is that black shale units lying immediately below the Fort Payne Chert in drill holes 202, 203, and 204 (20, 28, and about 10 ft [6.1, 8.5, and 3.0 m] thick, respectively) are Chattanooga Shale, and undivided Devonian strata that lie below are partially to wholly equivalent to the Frog Mountain Sandstone. Up to 24 radioactive shale units are present in Devonian strata in drill holes 202 through 204. On cross section N–N', we show these radioactive shales as green horizontal lines to the right of the gamma-ray curves for these drill holes.
Black shales in the Silurian and Devonian strata of the Greene-Hale-Perry-Bibb depositional margin have been of interest as potential natural gas exploration targets (Pashin and others, 2011). Multiple gas shows were detected in the Bayne-Etheridge 36-9 No. 1 well (drill hole 203 on cross section N–N') in undivided Silurian and Devonian formations at depths between 8,400 ft (2,560 m) and the top of the Chickamauga Limestone at 9,345 ft (2,848 m) (Pashin and others, 2011). In addition to drill hole 203, completions in this interval were attempted in the nearby Caldwell 19-15 No. 1 well (drilled horizontally in Greene County) and the Krout 10-14 No. 1 well in Bibb County (Ben Byerly, Geological Survey of Alabama, written communication, 2022) (fig. 1). Approximate isopach thicknesses of undivided Silurian-Devonian strata in the depositional margin are shown in figure 1 (based on Ben Byerly, Geological Survey of Alabama, written communication, 2022). The depth of burial of the sediments within the depositional margin seems favorable for maturation of kerogen and shale gas generation, but uneconomic production results between 2006 and 2011 discouraged continued exploration (Ben Byerly, Geological Survey of Alabama, written communication, 2022).
In drill hole 208, we identify 30 ft (9 m) of strata from 6,288 to 6,318 ft (1,917 to 1,926 m) as “undivided Devonian formations” following Pashin and others (2011). The Frog Mountain Sandstone is shown as very thin to negligible in geologic maps of the Woodstock and McCalla, Alabama, quadrangles to the southeast and northwest of drill hole 208 (Irvin and Osborne, 2000, and Osborne and others, 2006, respectively). In drill hole 205, we picked formation tops of the Chattanooga Shale and Frog Mountain Sandstone from the gamma-ray log at 7,940 and 7,980 ft (2,420 and 2,432 m), respectively. For more information about this topic, see the discussions in the Red Mountain Formation and Chattanooga Shale sections of this report.
Chattanooga Shale (Alone and Included in Undivided Devonian Formations)
The Middle to Upper Devonian (Chautauquan) Acadian unconformity of Swezey (2002) separates the Frog Mountain Sandstone from the overlying Upper Devonian (Chautauquan) Chattanooga Shale (fig. 3) in the VRPA. The Chattanooga Shale is composed of brownish- to grayish-black shale that is silty and organic rich, with light- to dark-gray, fine-grained sandstone containing pyrite and phosphatic inclusions. Gamma-ray response is typically greater than 150 API (Ben Byerly, Geological Survey of Alabama, written communication, 2022). Its thickness ranges from 0 to 82 ft (0 to 25 m) in Alabama (Raymond and others, 1988). Tim Chowns (University of West Georgia, written communication, 2022) measured 22.6 ft (6.9 m) of Chattanooga Shale in a roadside outcrop at Tannehill State Park, Alabama, about 5 miles (8 km) northwest of the northeastern end of cross section N–N′. As mentioned previously, from drill hole 201 to mile 44 on cross section N–N′ and in figure 3, we show the Frog Mountain Sandstone and the Chattanooga Shale together in a unit called “undivided Devonian formations” ranging from a maximum of 1,535 ft (468 m) in drill hole 202 to a minimum of 200 ft (61 m) at mile 44. Northeast of mile 44 on cross section N–N′ we show the Frog Mountain Sandstone and the Chattanooga Shale as two units separated by an unconformity. The GSA sample description log for drill hole 205 identifies 70 ft (21 m) of shale-dominated rocks at depths from 7,940 to 8,010 ft (2,420 to 2,442 m) as the Chattanooga Shale, however, wireline logs for drill hole 205 indicate the hot gamma-ray signature of the Chattanooga Shale between 7,940 and 7,980 ft (2,420 to 2,432 m).
For drill hole 208, we identify 30 ft (9 m) of strata between depths of 6,288 and 6,318 ft (1,917 and 1,926 m) as “undivided Devonian formations” per Pashin and others (2011) (see cross section B–B′ on plate 1 of Pashin and others, 2011). The top of the undivided Devonian unit in drill hole 208 was placed at a gamma-ray spike that is greater than 150 API at 6,288 ft (1,917 m) that was interpreted by Pashin and others (2011) to be the Chattanooga Shale. We follow this interpretation of Pashin and others (2011) identifying the top of the Chattanooga Shale. Argillaceous cherty limestones in the interval below the Chattanooga Shale are common in the Black Warrior basin; chert content (and porosity) in this interval increases sharply westward into Mississippi where the interval is referred to as “tripolitic chert” (Ben Byerly, Geological Survey of Alabama, written communication, 2022). The Chattanooga Shale is shown as very thin to negligible in geologic maps of the Woodstock and McCalla, Alabama, quadrangles to the southeast and northwest of drill hole 208 (Irvin and Osborne, 2000, and Osborne and others, 2006, respectively).
Wireline logs from the Krout 10-14 No. 1 well (1 mile [1.6 km] southeast of drill hole 205) (fig. 1) indicate the shallowest radioactive Chattanooga Shale between 6,574 and 6,605 ft (2,004 and 2,013 m) (Ben Byerly, Geological Survey of Alabama, written communication, 2022). Slickensides within this interval are identified on the mud log suggesting the possibility of a detachment surface (Ben Byerly, Geological Survey of Alabama, written communication, 2022). Although the potential repetition of the Frog Mountain Sandstone and Chattanooga Shale intervals in imbricate thrust sheets and structural thickening cannot be precluded, the similar thickness of the undivided Devonian formations in the Krout 10-14 No. 1 well and drill holes 201 through 204 is apparent (Ben Byerly, Geological Survey of Alabama, written communication, 2022). The Silurian-Devonian Greene-Hale-Perry-Bibb depositional margin parallels the grain of the Appalachian fold and thrust belt with thickening to the south and southeast (fig. 1) (Ben Byerly, Geological Survey of Alabama, written communication, 2022).
The Chattanooga Shale was deposited in a euxinic basin environment in a cratonic extension of the Acadian foreland basin (Pashin and others, 2011). See the discussions in the Red Mountain Formation and Frog Mountain Sandstone sections of this report for more information about the stratigraphy of the Red Mountain Formation, Frog Mountain Sandstone, and Chattanooga Shale, and the potential for oil and gas in black shales in the Greene-Hale-Perry-Bibb depositional margin.
Maury Shale
The Lower Mississippian (middle Kinderhookian) Maury Shale was deposited on an unnamed Lower Mississippian (lower Kinderhookian) unconformity above the Chattanooga Shale (fig. 3) at the southwestern end of the western VRPA (our study area location). The Maury Shale is composed of greenish-gray to grayish-red thinly laminated shale containing phosphate nodules. It is only 0 to 7 ft (0 to 2 m) thick. The Maury Shale was not recognized in the GSA sample description logs for drill holes 202, 204, and 205, therefore, we do not include it on cross section N–N' or in the figure 3 correlation chart.
Fort Payne Chert and Tuscumbia Limestone
Please note that Raymond and others (1988) show the Mississippian subdivided into a lower and upper series, whereas Cohen and others (2013) recognize a lower, middle, and upper series with the Kinderhookian and lower Osagean in the Lower Mississippian, the upper Osagean, Meramecian, and lower Chesterian in the Middle Mississippian, and the middle to upper Chesterian in the Upper Mississippian. This report follows the practice of the Cohen and others (2013) showing the Lower, Middle, and Upper Mississippian series.
The Lower to Middle Mississippian (Osagean) Fort Payne Chert (figs. 2 and 3) was deposited on an unnamed Lower Mississippian (upper Kinderhookian) unconformity above the Maury Shale at the southwestern end of the western VRPA (our study area location) (fig. 3). The Fort Payne Chert is composed of dark- to light-gray limestone that is finely crystalline to microcrystalline dolomitic and siliceous, with irregularly bedded to nodular smoky chert, dark shale, and light-gray limestone that is coarse, bioclastic, and lenticular. It ranges from 0 to 207 ft (0 to 63 m) thick in the VRPA. The Fort Payne Chert was deposited in a shallow-marine shelf environment during an orogenic lull between the Acadian and Alleghanian orogenies.
The overlying Middle Mississippian (lower Meramecian) Tuscumbia Limestone (figs. 2 and 3) lies conformably above the Fort Payne Chert and is composed of light-gray limestone that is bioclastic, micritic, or oolitic, in individual beds that are generally thicker than 1 ft (0.3 m), sometimes up to 10 ft (3 m) thick and massively crossbedded, very coarsely bioclastic (crinoidal), and sometimes containing light-gray and white chert nodules or concentrically banded “concretionary” chert nodules. It ranges from 0 to 250 ft (0 to 76 m) thick in the VRPA. Like the Fort Payne Chert, the Tuscumbia Limestone was also deposited in a shallow-marine shelf environment during an orogenic lull between the Acadian and Alleghanian orogenies.
The Fort Payne Chert and Tuscumbia Limestone are often shown together as one unit with the name “Fort Payne Chert and Tuscumbia Limestone, undivided.” Well logs in drill hole 202 reveal the top of the Fort Payne Chert and Tuscumbia Limestone undivided at 4,849 ft (1,478 m) and the base at 5,042 ft (1,537 m). The GSA sample description log for drill hole 202 incorrectly does not recognize the Fort Payne or Tuscumbia, showing the Mississippian Floyd Shale lying directly above a unit identified as “Devonian undifferentiated” at 4,740 ft (1,445 m). On cross section N–N′, we show the “Fort Payne Chert and Tuscumbia Limestone, undivided” unit varying from a minimum thickness of 307 ft (94 m) in drill hole 201 to a maximum thickness of 193 ft (59 m) in drill hole 202. In the gamma-ray log for drill hole 208, we identify 183 ft (56 m) of strata between depths 6,105 and 6,288 ft (1,861 and 1,917 m) as “Fort Payne Chert and Tuscumbia Limestone, undivided.” As mentioned previously, a large gamma-ray spike at 6,288 ft (1,917 m) records the presence of the underlying Chattanooga Shale as interpreted by Pashin and others (2011).
Upper Middle Mississippian to Middle Pennsylvanian Siliciclastic Strata
The upper Middle Mississippian to Middle Pennsylvanian siliciclastic formations (Floyd Shale, Parkwood Formation, and Pottsville Formation, and their lateral equivalents) record clastic sedimentation of the Alleghanian orogeny. For these Alleghanian clastics, two sources of sediments have been proposed, one to the southwest near the Ouachita thrust belt and one to the northeast near the previous Taconic highlands (Thomas, 1972; Raymond and others, 1988; Pashin and others, 2011).
Floyd Shale and Lateral Equivalents
At the southwestern end of the VRPA, the upper Middle to Upper Mississippian (upper Meramecian to middle Chesterian) Floyd Shale (figs. 2 and 3) lies conformably above the Tuscumbia Limestone. It is composed of dark-gray clay shale with siderite nodules interbedded with gray claystone, sandstone, limestone, and chert. It ranges from 0 to about 2,000 ft (607 m) thick. On cross section N–N′, we show the Floyd Shale varying from a maximum complete thickness of 943 ft (287 m) in drill hole 201 to a minimum complete thickness of 485 ft (148 m) in drill hole 208. The Floyd Shale thickens to the southwest, which is the apparent source of sediments, perhaps orogenic highlands associated with the Ouachita thrust belt (Raymond and others, 1988; Surles, 2007). It has been interpreted as northeastward prograding delta front, prodelta, and marine or barrier-bar sediments (Thomas, 1972; Raymond and others, 1988). However, Pashin and others (2011) (see their fig. 5) show an alternative interpretation for the Floyd Shale source area. They suggest two sources for the Floyd Shale and equivalent formation clastics that include (1) from the southwest in Mississippi and (2) from the northeast in Alabama. The Floyd Shale marks the end of the shallow-shelf environment that existed when the Fort Payne Chert and Tuscumbia Limestone were deposited. The Floyd Shale and its black shale facies, the Neal shale (not shown on cross section N–N′), are of interest for their potential as organic-rich shale sources for natural gas production (Pashin and others, 2011).
The Floyd Shale is present throughout the study area, but several Upper Mississippian formations that are laterally equivalent to the Floyd Shale exist to the northeast such as (1) the Middle to Upper Mississippian (Meramecian to Chesterian) Pride Mountain Formation, (2) the Upper Mississippian (Chesterian) Hartselle Sandstone, (3) the Middle to Upper Mississippian (Meramecian to Chesterian) Monteagle Limestone, and (4) the Upper Mississippian (Chesterian) Bangor Limestone (Thomas, 1972; Raymond and others, 1988; Pashin and others, 2011). We do not show any of the lateral equivalents of the Floyd Shale on cross section N–N' because they are not present at the southwestern end of the VRPA.
Parkwood Formation and Pennington Formation
At the southwestern end of the VRPA, the Upper Mississippian to Lower Pennsylvanian (Chesterian to lower Morrowan) Parkwood Formation (figs. 2 and 3) lies conformably on top of the Floyd Shale (Raymond and others, 1988). It is composed of medium- to dark-gray silty clay shale and mudstone interbedded with light- to medium-gray, very fine to fine grained, argillaceous, micaceous, crossbedded, and ripple-marked sandstone, containing siderite nodules, cherty limestone beds, red and grayish-green mudstone, and clayey coal. Thickness ranges from 0 to greater than 2,500 ft (762 m). On cross section N–N′, we show the Parkwood Formation varying from a minimum complete thickness of 510 ft (155 m) in drill hole 208 to a maximum complete thickness of 950 ft (290 m) in drill hole 204. As with the Floyd Shale, the Parkwood Formation thickens to the southwest, which is its apparent sediment source, possibly orogenic highlands associated with the Ouachita thrust belt (Thomas, 1972; Raymond and others, 1988; Surles, 2007). An alternate interpretation suggests that sandstone units in the Parkwood Formation (for example, the Carter sandstone in Alabama) were derived from the northwest (Bearden and Mancini, 1985). Also, Kugler and Pashin (1992) discuss alternate interpretations for the source of the Carter sandstone and other sandstones of the Parkwood Formation. The Parkwood Formation has been interpreted as being deposited in shallow marine bay and distributary front environments (Thomas, 1972).
At the northeastern end of the western VRPA, the Parkwood Formation grades into the laterally equivalent Upper Mississippian (Chesterian) Pennington Formation. We do not show the Pennington Formation on cross section N–N' because it is absent at the southwestern end of the VRPA.
Pottsville Formation
The youngest Paleozoic formation in the study area is the Lower to Middle Pennsylvanian (Morrowan to Atokan; Wanless, 1975) Pottsville Formation (figs. 2 and 3) that conformably overlies the Parkwood and Pennington Formations. The Pottsville Formation is composed of interbedded sandstone, conglomerate, siltstone, shale, and coal. The upper section has the most coal beds; clastic sediments between coal beds exhibit cyclic coarsening upward sequences that start with basal, bioturbated shales containing marine fossils overlain by siltstones and fine-grained sandstones. Total thickness ranges from 0 to more than 9,000 ft (2,743 m). On cross section N–N′, we show the Pottsville Formation varying from maximum partial thickness of 7,605 ft (2,318 m) in drill hole 206 to a minimum partial thickness of about 665 ft (203 m) in drill hole 201 (note that no complete sections of the Pottsville Formation are present along the cross section). At the southwestern end of the VRPA, the source of Pottsville Formation sediments was to the southwest as was the case with the underlying Parkwood Formation. In the Cahaba and Coosa coal fields (fig. 1, index map 2), the Pottsville Formation includes several thick-bedded, conglomeratic, quartzose sandstone members (for example, the basal Shades Sandstone, middle Pine Sandstone, and upper Chestnut Sandstone Members). The Shades Sandstone Member is a thick-bedded, coarse-grained, quartzose sandstone that is conglomeratic at its base. It is present at the base of the Pottsville Formation averaging about 200 ft (61 m) in the Coosa coal field and ranging from 190 to 500 ft (58 to 152 m) in the Cahaba coal field (fig. 1, index map 2). The Pine Sandstone Member grades upward to a flaggy fine-grained sandstone. It lies 200 to 1,000 ft (61 to 305 m) above the basal Shades Sandstone Member. The Pine Sandstone Member increases in thickness from 250 ft (76 m) in the northern Cahaba coal field (index map 1) to 400 ft (122 m) in the southern Cahaba coal field (fig. 1, index map 2), and 200 to 500 ft (61 to 152 m) in the Coosa coal field (index map 2). The Chestnut Sandstone Member lies above the Gould coal group in the Cahaba coal field about 500 to 800 ft (152 to 244 m) above the Pine Sandstone Member. It thins from 200 to 100 ft (61 and 30 m) from south to north in the Cahaba coal field. These quartz arenite sandstones in the Pottsville Formation have been interpreted as barrier island deposits overlain by coal-bearing delta plain deposits in the upper formation (Thomas, 1972). On cross section N–N', we identified the Shades Sandstone Member at the base of the Pottsville Formation by its low radioactivity on gamma-ray well logs. It ranges from 155 ft (37 m) in drill hole 201 to 260 ft (79 m) in drill hole 208. The Pine Sandstone Member may also be represented by intervals of low radioactivity from 250 to 900 ft (91 to 274 m) above the Shades Sandstone Member in the gamma-ray logs of drill holes 201–205 and 208.
As previously described, in the northeastern half of cross section N–N', we determined depths to coal beds in the Pottsville Formation in drill holes 206 and 208 from mud logs, using the generalized section of the Pottsville Formation from the Mineral Resources Map of Bibb County, Alabama (Szabo and others,1976) for identification. We used the geologic map from the same publication to locate and identify coal beds at the surface and to infer the location of coal beds, formation boundaries, and fold structures at depth. Because these coal bed horizons and formation contacts were drawn based on the shape of overlying coal horizons at the surface and not on actual depths determined from well logs or other forms of empirical observation, the actual configuration of subsurface coal beds and formations in this area may be different than the configuration shown on the northeastern half of cross section N–N'.
The anticline and syncline configurations of coal bed horizons at the surface near drill holes 206 through 207 are our interpretations based on the surface locations of the coal beds along cross section N–N' and the depths to those same coal beds in drill holes 206 and 208. The geologic map from the Mineral Resources Map of Bibb County (Szabo and others, 1976) was also used to determine the locations of the axes of the syncline northeast of drill hole 205 (at mile 54 on cross section N–N′) and the anticline southwest of drill hole 206 (at mile 60). Subsurface coal bed horizons and formation contacts in the northeastern half of the cross section were drawn subparallel to the surface coal-bed horizons above. Northeast of drill hole 207, the formation contacts below the Pottsville Formation were drawn rising to the northeast where they meet drill hole 208 (based on the gamma-ray, mud, and GSA sample description logs for drill hole 208 and cross section B–B′ from plate 1 of Pashin and others [2011]) and cross section I of Thomas and Pashin (2011) (fig. 7) at the northeastern end of cross section N–N′.
Several dozen coal beds in the Cahaba coal field (fig. 1, index map 2) have been described by Culbertson (1964), Shotts (1971), Southern Railway (1972), and Ward (1984). The Gould and Nunnally coal groups, and the Harkness, Big Bone, Woodstock, Gholson, and Lower and Upper Thompson coal beds shown on cross section N–N' were considered important by Southern Railway (1972). Furthermore, Culbertson (1964) described the Woodstock, Gholson, Thompson, and Helena beds as high-quality coals with low sulfur and ash (mineral matter) content. The Nunnally, Harkness, and Montevallo coals were also low in sulfur, but had moderate to high ash content (Culbertson, 1964). Ward (1984) described the Thompson, Coke, and Woodstock coal beds as consistent producers of coking quality coal. Szabo and other (1976) recognize the following 41 coal beds in northeastern Bibb County in the Cahaba coal field (in ascending order): the Earhart, Lower Gould, Upper Gould, Nunnally No. 1, Nunnally No. 2, Nunnally No. 3, Nunnally No. 4, Lower Harkness, Upper Harkness, Martin, Lower Wadsworth, Upper Wadsworth, Clean, Big Bone, Beechtree, Jones, Alice, Atkins, Coke, Sixteen Inch, Woodstock, Gholson, Slatey, Moyle, Little Pittsburgh, Quarry, Smithshop, Lower Thompson, Upper Thompson, Helena, Lower Yeshic, Upper Yeshic, Lower Montevallo, Upper Montevallo, Air Shaft, and Straven No. 1 coal beds, and five anonymous coal beds in the Five-Seam coal group between the Nunnally No. 4 and Lower Harkness coal beds. We show 18 of these coal beds on cross section N–N'. Coal production from the Cahaba coal field varied between 1978 and 1993 (Pashin and others, 1995) with the maximum production of 776,000 short tons in 1980 and a minimum of 18,000 short tons in 1987.
The Pottsville Formation produced 49,623,609 thousand cubic feet (Mcf) (1,405,184 thousand cubic meters [Mcm]) of coalbed gas in the Gurnee coalbed gas field of Bibb and Shelby Counties, Alabama, between April 1990 and December 1991 (Geological Survey of Alabama, 2024). Drill holes 206 and 207 fall within the boundaries of the Gurnee coalbed gas field, but only drill hole 207 was considered part of the field. Drill hole 206 only produced 291 Mcf (8.24 Mcm) of gas in 1990, while drill hole 207 produced 74,088 Mcf (2,098 Mcm) of coalbed gas between March 2009 and June 2022 (mostly between April 2014 and June of 2022) (Geological Survey of Alabama, 2024).
Cretaceous and Quaternary Siliciclastic and Carbonate Strata
A regional unconformity that ranges from the Lower Pennsylvanian (Morrowan-Atokan) to the Holocene truncates the top of the Pottsville Formation and multiple underlying formations in the study area. Whereas numerous formations ranging in age from Triassic to Holocene were deposited on top of the unconformity in Alabama, in the study area, only units in the Cretaceous were deposited and preserved above the unconformity and dip to the southwest at about 35 ft (11 m) per mile (1.6 km) (less than one degree) (Wahl, 1966; Raymond and others, 1988). In ascending order, they are: (1) undivided Lower Cretaceous formations; (2) the Coker Formation of the Tuscaloosa Group; (3) the Gordo Formation of the Tuscaloosa Group; (4) the Eutaw Formation; and (5) the Mooreville Chalk of the Selma Group. In general, Cretaceous formations thicken to the southwest where they may attain a total thickness of up to 24,000 ft (7,315 m) near the Gulf of Mexico. Multiple unconformities eroded the tops of these southwestward dipping units before deposition of the next higher unit. A final early Pleistocene unconformity truncated the surface of underlying strata before Pleistocene alluvial terrace deposits and Holocene alluvial deposits were deposited at the surface.
Undivided Lower Cretaceous Formations
Undivided Lower Cretaceous formations (fig. 3) overlie the top of truncated Parkwood and Pottsville strata in the southwestern half of the study area (cross section N–N′). These formations are composed of pink and yellow coarse-grained sand and gravel with gray shale and red to ochre clay. GSA sample description logs for drill holes 202 and 204 record the presence of “undifferentiated” Lower Cretaceous formations, but the unit was not recognized in the sample description log of drill hole 205. On cross section N–N′, we show the undivided Lower Cretaceous formations varying from measured thicknesses of 680 ft (207 m) in drill hole 202 and 210 ft (64 m) in drill hole 204, then pinching out between drill holes 204 and 205.
Tuscaloosa Group
The Upper Cretaceous Tuscaloosa Group (Cenomanian to Coniacian) lies unconformably above truncated strata of undivided Lower Cretaceous formations, the Pottsville Formation, and Cambrian and Ordovician strata of the Helena and Rock Creek School thrust sheets in the southwestern half of cross section N–N'. In the Coastal Plain outcrop belt of Alabama, the Tuscaloosa Group ranges from less than 300 ft (91 m) thick in eastern Alabama to 900 ft (274 m) thick in western Alabama, and includes two formations, the Coker Formation, and the overlying Gordo Formation (Raymond and others, 1988).
Coker Formation of Tuscaloosa Group
The Upper Cretaceous (upper Cenomanian to lower Turonian) Coker Formation (figs. 2 and 3), the lower unit of the Tuscaloosa Group, lies unconformably above undivided Lower Cretaceous formations, the Pottsville Formation, and Cambrian strata of the Helena and Rock Hill School thrust sheets in the southwestern half of cross section N–N'. The formation is composed of light-colored sand that is very fine to medium grained, micaceous, and crossbedded, with varicolored micaceous clay, and a few thin gravel beds containing chert and quartz pebbles. In western Alabama, the basal Eoline Member of the Coker Formation (not shown on cross section N–N') is composed of very fine to medium grained sand, silt, and dark-gray carbonaceous and lignitic clay. Thickness of the Coker Formation ranges from 230 to over 500 ft (152 m). GSA sample description logs recognized 840 and 870 ft (256 and 265 m) of Coker Formation in drill holes 202 and 204, respectively. On cross section N–N′, we show the Coker Formation with measured thicknesses of 840 ft (256 m) in drill hole 202 and 870 ft (265 m) in drill hole 204, then rising to the northeast where it is exposed at the surface between miles 38 and 52. According to the geologic map of Alabama (Szabo and others, 1988) (fig. 2), the Coker Formation is completely removed by erosion to the northeast of mile 52, except for two small outliers between miles 55 and 58 above the Helena thrust sheet, and two other outliers northwest of cross section N–N′ between drill holes 206 and 208.
Gordo Formation of Tuscaloosa Group
The Upper Cretaceous (Turonian to Coniacian) Gordo Formation (figs. 2 and 3), the upper unit of the Tuscaloosa Group, lies unconformably above the Coker Formation in the southwestern half of cross section N–N'. The formation is composed of continental sediments. It includes crossbedded sand in massive beds that contain gravel and mottled gray, red, and purple clay that is lenticular, and sometimes carbonaceous; the lower part of the formation is predominantly gravelly sand with chert and quartz pebbles. Thickness ranges from 115 to 300 ft (35 to 91 m). On cross section N–N′, we show the Gordo Formation with a measured thickness of 280 ft (85 m) in drill hole 202, then rising in elevation to the northeast where it is exposed at the surface between miles 33 and 44. The Gordo Formation is completely removed by erosion to the northeast of mile 44.
McShan Formation
The Upper Cretaceous (Coniacian to Santonian) McShan Formation is an interval of marine sand and clay that rests unconformably on the continental sediments of the Gordo Formation of the Tuscaloosa Group (Monroe and others, 1946). It is mapped at the surface in Hale and Greene Counties within the study area (Monroe and others, 1946) and regionally in the subsurface just northwest of the study area (Eargle, 1946) but is difficult to identify on wireline logs beneath the overlying Eutaw Formation. It is often included with the Eutaw Formation as “Eutaw and McShan Formations, undivided” or “lower, unnamed member of the Eutaw Formation.” It was not recognized by Raymond and others (1988) and is not shown on cross section N–N′ or figure 3.
Eutaw Formation
The Upper Cretaceous (Santonian to Campanian) Eutaw Formation (figs. 2 and 3) lies unconformably above the Gordo Formation of the Tuscaloosa Group in the southwestern half of cross section N–N'. The formation is composed of light-greenish-gray sand that is fine to medium grained, well sorted, micaceous, crossbedded, fossiliferous, and glauconitic, with interbedded greenish-gray silty, micaceous clay, and medium-dark-gray carbonaceous clay. Thickness in the outcrop belt ranges from 100 ft (30 m) in western Alabama to 400 ft (122 m) in eastern Alabama. The upper Tombigbee Sand Member of the Eutaw Formation (not shown on cross section N–N' or fig. 3) is a light-gray sand that is massive to weakly bedded, very glauconitic, somewhat clayey, abundantly burrowed, containing mollusk shells, and layers of calcareous sandstone and sandy chalk; it is present in west-central Alabama. On cross section N–N′, we show the Eutaw Formation with a measured thickness of 430 ft (131 m) in drill hole 202, then rising in elevation to the northeast where it is exposed at the surface between miles 10 and 37. The Eutaw Formation is completely removed by erosion to the northeast of mile 37.
Selma Group
The Upper Cretaceous (Campanian to Maastrichtian) Selma Group lies unconformably above truncated strata of the Eutaw Formation in the southwestern half of cross section N–N'. In the Coastal Plain outcrop belt of central and western Alabama, the Selma Group includes four formations (in ascending order): the Mooreville Chalk, the Demopolis Chalk, the Ripley Formation, and the Prairie Bluff Chalk. The Mooreville Chalk is the only one of these formations that is present in the study area and is the only one discussed in this report.
Mooreville Chalk of Selma Group
The highest Cretaceous formation in the study area, the Upper Cretaceous (Campanian) Mooreville Chalk (figs. 2 and 3), is the lowest unit of the Selma Group and it lies unconformably above the Eutaw Formation in the southwestern part of cross section N–N'. The Mooreville Chalk is composed of yellowish-gray to dark-bluish-gray compact chalk and fossiliferous, glauconitic chalky marl. The lowest section of the Mooreville Chalk is a compact calcareous sand that is only a few feet thick, contains quartz pebbles, phosphatic steinkerns, and pellets. The upper Arcola Limestone Member (about 10 ft [3 m] thick at the top of the Mooreville Chalk; not shown on cross section N–N') has two to four light-gray limestone beds that are impure, dense, brittle, and fossiliferous, that are interbedded with softer marl. The thickness of the Mooreville Chalk ranges from 280 ft (85 m) in western Alabama to 600 ft (183 m) in Montgomery County, Alabama (index map 2). On cross section N–N′, we show the Mooreville Chalk with a measured thickness of 260 ft (79 m) in drill hole 202, then rising in elevation to the northeast where it is exposed at the surface between miles 7 and 11 and is completely removed by erosion to the northeast of mile 11.
Quaternary Deposits
The Quaternary deposits in the study area lie unconformably above truncated strata of Cretaceous and Paleozoic formations across the entire length of cross section N–N'. The Quaternary deposits include (in ascending order): Pleistocene terrace deposits, and Holocene alluvial deposits.
Pleistocene Terrace Deposits
Pleistocene terrace deposits (figs. 2 and 3) lie horizontally above older units that were planed off by an earlier Pleistocene unconformity in the study area. These terraces represent floodplain deposits of ancient streams that were abandoned when base level dropped and the streams eroded deeper channels into the deposits. They are composed of poorly sorted gravel, crossbedded sand, silt, and clay. These deposits may contain organic matter, peat, and shell debris. On cross section N–N', Pleistocene terrace deposits have a measured thickness of 80 ft (24 m) in drill hole 202 and are found at the surface between miles 0 and 5, and between miles 11 and 14. Wahl (1966) gives a maximum thickness of 70 ft (21 m) for terrace deposits in Greene County, Alabama (index map 2).
Holocene Alluvial Deposits
Holocene alluvial deposits (figs. 2 and 3) are found in the floodplains of modern streams in the study area lying unconformably above older units that were planed off by a final early Pleistocene unconformity. These deposits are lithologically similar to the Pleistocene terrace deposits as previously described, but generally contain more organic material (Raymond and others, 1988). Holocene alluvial deposits are found at the surface near numerous creeks and rivers along cross section N–N'.
References Cited
Bailey, R.M., 2007, Seismic interpretation and structural restoration of a seismic profile through the southern Appalachian thrust belt under Gulf Coastal Plain sediments: Tuscaloosa, Ala., University of Alabama, M.S. thesis, 71 p., accessed March 29, 2024, at https://ir.ua.edu/handle/123456789/8360.
Bayona, G., and Thomas, W.A., 2003, Distinguishing fault reactivation from flexural deformation in the distal stratigraphy of the peripheral Blountian foreland basin, southern Appalachians, USA: Basin Research, v. 15, no. 4, p. 503–526, accessed March 29, 2024, at https://doi.org/10.1046/j.1365-2117.2003.00217.x.
Bayona, G., Thomas, W.A., and Van der Voo, R., 2003, Kinematics of thrust sheets within transverse zones—A structural and paleomagnetic investigation in the Appalachian thrust belt of Georgia and Alabama: Journal of Structural Geology, v. 25, no. 8, p. 1193–1212, accessed March 29, 2024, at https://doi.org/10.1016/S0191-8141(02)00162-1.
Bearce, D.N., 1978, Structure of the eastern Coosa Valley, Alabama: American Journal of Science, v. 278, no. 4, p. 463, accessed March 29, 2024, at https://doi.org/10.2475/ajs.278.4.461.
Bearce, D.N., Irvin, G.D., Osborne, W.E., and Ward, W.E., 2008, Geologic map of the Sleeping Giants 7.5-minute quadrangle, St. Clair and Talladega Counties, Alabama: Geological Survey of Alabama Quadrangle Series Map 27, 2 pls., scale 1:24,000. [Also available at https://www.ogb.state.al.us/gsa/geologic/mapping.]
Bearce, D.N., Thomas, W.A., and Drahovzal, J.A., 1977, Bedrock geology of parts of Cherokee, Calhoun and Cleburne Counties, Alabama: Alabama Geological Society, 15th Annual Field Trip Guidebook, 1 pl., scale 1:63,360. [Also available at https://archives.datapages.com/data/alabama/22/roadlog.htm?q=%2BauthorStrip%3Abearce.]
Bearden, B.L., and Mancini, E.A., 1985, Petroleum geology of Carter sandstone (Upper Mississippian), Black Warrior basin, Alabama: American Association of Petroleum Geologists Bulletin, v. 69, no. 3, p. 361–377, accessed March 29, 2024, at https://pubs.geoscienceworld.org/aapgbull/article-abstract/69/3/361/37931/petroleum-geology-of-carter-sandstone-upper.
Berdan, J.M., Boucot, A.J., and Ferrill, B.A., 1986, The first fossiliferous Pridolian beds from the southern Appalachians in northern Alabama, and the age of the uppermost Red Mountain Formation: Journal of Paleontology, v. 60, no. 1, p. 180–185. [Also available at https://www.jstor.org/stable/1305106.]
Bergstrom, S.M., Huff, W.D., Saltzman, M.R., Kolata, D.R., and Leslie, S.A., 2004, The greatest volcanic ash falls in the Phanerozoic—Trans-Atlantic relations of the Ordovician Millbrig and Kinnekulle K-bentonites: The Sedimentary Record, v. 2, no. 4, p. 4–8, accessed March 29, 2024, at https://doi.org/10.2110/sedred.2004.4.4.
Brett, C.E., Goodman, W.M., and LoDuca, S.T., 1990, Sequences, cycles, and basin dynamics in the Silurian of the Appalachian foreland basin: Sedimentary Geology, v. 69, nos. 3–4, p. 191–244, accessed March 29, 2024, at https://doi.org/10.1016/0037-0738(90)90051-T.
Butts, C., 1940, Montevallo-Columbiana folio, Alabama: U.S. Geological Survey Atlas, Folio 226, 19 p., 9 pls., 7 sheets, scale 1:62,500, accessed March 29, 2024, at https://doi.org/10.3133/gf226.
Cato, C.L., 2014, Seismic interpretation and structural analysis of the Alleghanian fold-thrust belt in central Alabama: Tuscaloosa, Ala., University of Alabama, M.S. thesis, 71 p., 1 pl., accessed March 29, 2024, at https://ir.ua.edu/handle/123456789/2236.
Causey, L.V., 1978, Availability of ground water in Bibb County, Alabama: Geological Survey of Alabama Map 144, pl. 1, scale 1:126,720, accessed March 29, 2024, at https://ngmdb.usgs.gov/Prodesc/proddesc_67827.htm.
Causey, L.V., 1965, Geology and ground-water resources of Cherokee County, Alabama—A reconnaissance: Geological Survey of Alabama Bulletin 79, 65 p., accessed March 29, 2024, at https://www.gsa.state.al.us/Home/DownloadPubDocument/?path=Bulletins&fileName=B079.pdf.
Chowns, T.M., and Rindsberg, A.K., 2015, Stratigraphy and depositional environments in the Silurian Red Mountain Formation of the southern Appalachian basin, USA, in Holmes, A.E., ed., Diverse excursions in the southeast—Paleozoic to present: Geological Society of America Field Guide 39, p. 95–143, accessed March 29, 2024, at https://doi.org/10.1130/2015.0039(04).
Clark, J.E., Bonura, D.K., Miller, C., and Fischer, F.T., 2005, Demonstration of presence and size of a CO2-rich fluid phase after HCl injection in carbonate rock, chap. 33 of Tsang, C.-F., and Apps, J.A., eds., Underground injection science and technology: Developments in Water Science, v. 52, p. 451–458, accessed March 29, 2024, at https://doi.org/10.1016/S0167-5648(05)52033-1.
Cohen, K.M., Finney, S.C., Gibbard, P.L., and Fan, J.-X., 2013, The ICS international chronostratigraphic chart (ver. 2022/02, updated 2022): Episodes, v. 36, p. 199–204, accessed March 29, 2024, at https://www.stratigraphy.org/ICSchart/ChronostratChart2022-02.pdf.
Colton, G.W., 1961, Geologic summary of the Appalachian basin, with reference to the subsurface disposal of radioactive waste solutions: U.S. Geological Survey Trace Elements Investigations 791, 120 p., 16 pls. in pocket, accessed March 29, 2024, at https://doi.org/10.3133/tei791. [Prepared on behalf of the U.S. Atomic Energy Commission.]
Cook, B.S., and Thomas, W.A., 2010, Ductile duplexes as potential natural gas plays—An example from the Appalachian thrust belt in Georgia, USA, in Goffey, G.P., Craig, J., Needham, T., and Scott, R., eds., London, Geological Society Special Publications 2010, v. 348, p. 57–70, accessed March 29, 2024, at https://doi.org/10.1144/SP348.4.
Crawford, T.J., and Kath, R.L., 2016, Geology of Indian Mountain, Rock Run and Borden Springs area, Georgia and Alabama—A New Paradigm: Alabama Geological Society Guidebook, v. 56, p. 4, accessed March 29, 2024, at https://www.researchgate.net/publication/349721911_GEOLOGY_OF_INDIAN_MOUNTAIN_ROCK_RUN_AND_BORDEN_SPRINGS_AREA_GEORGIA_AND_ALABAMA_A_NEW_PARADIGM.
Cressler, C.W., 1970, Geology and ground-water resources of Floyd and Polk Counties, Georgia: Georgia Geological Survey Information Circular 39, p. 6, accessed March 29, 2024, at https://epd.georgia.gov/outreach/publications/georgia-geologic-survey-information-circulars.
Cressler, C.W., 1974, Geology and ground-water resources of Gordon, Whitefield, and Murray Counties, Georgia: Georgia Geological Survey Information Circular 47, 56 p., accessed March 29, 2024, at https://epd.georgia.gov/outreach/publications/georgia-geologic-survey-information-circulars.
Cressler, C.W., Blanchard, H.E., Jr., and Hester, W.G., 1979, Geohydrology of Bartow, Cherokee and Forsyth Counties, Georgia: Georgia Geological Survey Information Circular 50, p. 12, accessed March 29, 2024, at https://epd.georgia.gov/outreach/publications/georgia-geologic-survey-information-circulars.
Culbertson, W.C., 1964, Geology and coal resources of the coal-bearing rocks of Alabama: U.S. Geological Survey Bulletin 1182–B, 79 p., 4 pls. [Also available at https://doi.org/10.3133/b1182B.]
Davis, M.E., Sanford, T.H., Jr., and Ellard, J.S., 1980, Water availability and geology of Sumter County, Alabama: Geological Survey of Alabama Special Map 158, pl. 1, scale 1:125,000, accessed March 29, 2024, at https://alabamamaps.ua.edu/historicalmaps/counties/sumter/sumter.html.
Davis, M.E., Sanford, T.H., Jr., and Jefferson, P.O., 1975, Water availability and geology of Hale County, Alabama: U.S. Geological Survey Open-File Report 75–452, 90 p., accessed March 29, 2024, at https://doi.org/10.3133/ofr75452.
Dennison, J.M., and Head, J.W., 1975, Sea level variations interpreted from the Appalachian basin Silurian and Devonian: American Journal of Science, v. 275, no. 10, p. 1089–1120, accessed March 29, 2024, at https://doi.org/10.2475/ajs.275.10.1089.
Dwyer, G.S., and Repetski, J.E., 2012, The Middle Ordovician Knox unconformity in the Black Warrior Basin, in Derby, J., Fritz, R., Longacre, S., Morgan, W., and Sternbach, C., eds., The great American carbonate bank—The geology and economic resources of the Cambrian-Ordovician Sauk megasequence of Laurentia: American Association of Petroleum Geologists Memoir, v. 98, p. 345–356, accessed March 29, 2024, at https://doi.org/10.1306/13331498M983499.
Eargle, D.H., 1946, Correlation of the pre-Selma Upper Cretaceous formations between Tuscaloosa County, Alabama, and Neshoba County, Mississippi: U.S. Geological Survey Oil and Gas Investigations Chart, 1 sheet, scale 1:760,000, accessed March 29, 2024, at https://ngmdb.usgs.gov/Prodesc/proddesc_5178.htm.
Eargle, D.H., 1947, Profiles showing geology along highways in the vicinity of Tuscaloosa, Alabama: U.S. Geological Survey Oil and Gas Investigations Preliminary Chart 31, 1 sheet, scale 1:253,440, accessed March 29, 2024, at https://pubs.er.usgs.gov/publication/oc31.
Fisher, C.M., Loewy, S.L., Miller, C.F., Berquist, P., Van Schmus, W.R., Hatcher, R.D., Jr., Wooden, J.L., and Fullagar, P.D., 2010, Whole-rock Pb and Sm-Nd isotopic constraints on the growth of southeastern Laurentia during Grenvillian orogenesis: Geological Society of America Bulletin 122, nos. 9–10, p. 1646–1659, accessed March 29, 2024, at https://doi.org/10.1130/B30116.1.
Fritz, R.D., Medlock, P., Kuykendal, M., and Wilson, J.L., 2012, The great American carbonate bank—Knox Group in the Black Warrior basin, in Derby, J., Fritz, R., Longacre, S., Morgan, W., and Sternbach, C., eds., The great American carbonate bank—The geology and economic resources of the Cambrian-Ordovician Sauk megasequence of Laurentia: American Association of Petroleum Geologists Memoir, v. 98, p. 301–344, accessed March 29, 2024, at https://doi.org/10.1306/13331497M980077.
Geological Survey of Alabama, 2006, Digital geologic map of Alabama (1st ed.): Geological Survey of Alabama Special Map Series 220A, scale 1:250,000, accessed March 29, 2024, at https://www.gsa.state.al.us/gsa/geologic/algeology/algmaps. [Vector digital data for faults and polygons available at https://www.gsa.state.al.us/gsa/geologic/geospatial.]
Geological Survey of Alabama, 2024, State of Alabama oil and gas wells database—Well search: Geological Survey of Alabama, State of Alabama Oil and Gas Board, accessed February 22, 2024, at https://ogb.state.al.us/ogb/fields and https://ogb.state.al.us/ogb/wells.
Groshong, R.H., Jr., Hawkins, W.B., Jr., Pashin, J.C., and Harry, D.L., 2010, Extensional structures of the Alabama promontory and Black Warrior foreland basin—Styles and relationship to the Appalachian fold-thrust belt, in Tollo, R.P., Bartholomew, M.J., Hibbard, J.P., and Karabinos, P.M., eds., From Rodinia to Pangea—The lithotectonic record of the Appalachian region: Geological Society of America Memoir 206, p. 579–605, accessed March 29, 2024, at https://doi.org/10.1130/MEM206.
Harris, L.D., and Milici, R.C., 1977, Characteristics of thin-skinned style of deformation in the southern Appalachians, and potential hydrocarbon traps: U.S. Geological Survey Professional Paper 1018, 40 p., 9 pls. in pocket, accessed March 29, 2024, at http://pubs.er.usgs.gov/publication/pp1018.
Hatcher, R.D., Jr., and Repetski, J.E., 2007, The post-Knox unconformity—Product of global, not regional processes [abs.]: Geological Society of America Abstracts with Programs, v. 39, no. 2, p. 8, accessed March 29, 2024, at https://gsa.confex.com/gsa/2007SE/webprogram/Paper118749.html.
Haynes, J.T., 1994, The Ordovician Deicke and Millbrig K-bentonite beds of the Cincinnati arch and the southern Valley and Ridge province: Geological Society of America Special Paper 290, 80 p., accessed March 29, 2024, at https://doi.org/10.1130/SPE290-p1.
Higgins, M.W., Atkins, R.L., Crawford, T.J., Crawford, R.F., III, Brooks, R., and Cooke, R.B., 1988, The structure, stratigraphy, tectonostratigraphy, and evolution of the southernmost Appalachian orogen: U.S. Geological Survey Professional Paper 1475, 173 p., 2 pls., accessed March 29, 2024, at https://doi.org/10.3133/pp1475.
Huff, W.D., and Kolata, D.R., 1990, Correlation of the Ordovician Deicke and Millbrig K-bentonites between the Mississippi Valley and the Southern Appalachians: American Association of Petroleum Geologists Bulletin, v. 74, no. 11, p. 1736–1747, accessed March 29, 2024, at https://doi.org/10.1306/0C9B2583-1710-11D7-8645000102C1865D.
Irvin, G.D., and Osborne, W.E., 2000, Geologic map of the Woodstock, 7.5-minute quadrangle, Bibb and Tuscaloosa Counties, Alabama: Geological Survey of Alabama Quadrangle Series Map 19, 2 pls., scale 1:24,000, digital GIS data. [Also available at https://www.ogb.state.al.us/gsa/geologic/mapping.]
Johnson, L.W., and Tull, J.F., 2002, Sylacauga Marble Group—Distal fragment of the southern Appalachian Cambrian-Ordovician carbonate platform: Southeastern Geology, v. 41, no. 2, p. 75–102, accessed March 29, 2024, at https://www.researchgate.net/publication/288705381_Sylacauga_Marble_Group_Distal_fragment_of_the_southern_Appalachian_Cambrian-Ordovician_carbonate_pl atform.
Kidd, J.T., and Neathery, T.L., 1976, Correlation between Cambrian rocks of the southern Appalachian geosyncline and the interior low plateaus: Geology, v. 4, no. 12, p. 767–769, accessed March 29, 2024, at https://doi.org/10.1130/0091-7613(1976)4<767:CBCROT>2.0.CO;2.
Kopaska-Merkel, D.C., Dean, L.S., and Moore, J.D., 2005, Hydrogeology and vulnerability to contamination of major aquifers in Alabama—Area 4: Geological Survey of Alabama Circular 199D, 95 p., 11 pls., accessed March 29, 2024, at https://www.gsa.state.al.us/Home/DownloadPubDocument/?path=Circulars&fileName=C199D.pdf.
Karlstrom, K.E., and Timmons, J.M., 2012, Many unconformities make one 'Great Unconformity', in Timmons, J.M., and Karlstrom, K.E., eds., Grand Canyon geology—Two billion years of earth's history: Geological Society of America Special Paper 489, p. 73–79, accessed March 29, 2024, at https://doi.org/10.1130/2012.2489(04).
Kugler, R.L., and Pashin, J.C., 1992, Reservoir heterogeneity in Carter Sandstone, North Blowhorn Creek oil unit and vicinity, Black Warrior basin: Geological Survey of Alabama, report no. DOE/BC/14448–9, 106 p., accessed March 29, 2024, at https://www.osti.gov/servlets/purl/5289910.
Lloyd, O.B., Jr., and Reid, M.S., 1990, Evaluation and liquid waste-storage potential based on porosity distribution in the Paleozoic rocks in central and southern parts of the Appalachian basin: U.S. Geological Survey Professional Paper 1468, 81 p., 3 pls. in pocket, accessed March 29, 2024, at https://doi.org/10.3133/pp1468.
Lucier, A., Zoback, M., Gupta, N., and Ramakrishnan, T.S., 2006, Geomechanical aspects of CO2 sequestration in a deep saline reservoir in the Ohio River Valley region: Environmental Geology, v. 13, no. 2, p. 85–103, accessed March 29, 2024, at http://eg.geoscienceworld.org/content/13/2/85.full.pdf+html.
Mack, G.H., 1980, Stratigraphy and depositional environments of the Chilhowee Group (Cambrian) in Georgia and Alabama: American Journal of Science, v. 280, no. 6, p. 497–517, accessed March 29, 2024, at https://doi.org/10.2475/ajs.280.6.497.
Menning, M., Alekseev, A.S., Chuvashov, B.I., Davydov, V.I., Devuyst, F.-X., Forke, H.C., Grunt, T.A., Hance, L., Heckel, P.H., Izokh, N.G., Jin, Y.-G., Jones, P.J., Kotlyar, G.V., Kozur, H.W., Nemyrovska, T.I., Schneider, J.W., Wang, X.-D., Weddige, K., Weyer, D., and Work, D.M., 2006, Global time scale and regional stratigraphic reference scales of central and west Europe, east Europe, Tethys, south China, and North America as used in the Devonian-Carboniferous-Permian correlation chart 2003 (DCP 2003): Palaeogeography, Palaeoclimatology, Palaeoecology, v. 240, nos. 1–2, p. 318–372, accessed March 29, 2024, at https://doi.org/10.1016/j.palaeo.2006.03.058.
Milici, R.C., Ryder, R.T., Swezey, C.S., Charpentier, R.R., Cook, T.A., Crovelli, R.A., Klett, T.R., Pollastro, R.M., and Schenk, C.J., 2003, Assessment of undiscovered oil and gas resources of the Appalachian basin province, 2002: U.S. Geological Survey Fact Sheet 009–03, 2 p., accessed March 29, 2024, at https://doi.org/10.3133/fs00903.
Monroe, W.H., Conant, L.C., and Eargle, D.H., 1946, Pre-Selma Upper Cretaceous stratigraphy of western Alabama: Bulletin of the American Association of Petroleum Geologists, v. 30, no. 2, p. 187–212, accessed March 29, 2024, at https://doi.org/10.1306/3D9337EE-16B1-11D7-8645000102C1865D.
Mussman, W.J., 1982, The Middle Ordovician Knox unconformity, Virginia Appalachians—Transition from passive to convergent margin: Blacksburg, Va., Virginia Polytechnic Institute and State University, M.S. thesis, 160 p., accessed March 29, 2024, at https://vtechworks.lib.vt.edu/bitstream/handle/10919/87130/LD5655.V855_1982.M877.pdf.
Mussman, W.J., Montanez, I.P., and Read, J.F., 1988, Ordovician Knox paleokarst unconformity, Appalachians, in James, N.P., and Choquette, P.W., eds., Paleokarst: New York, Springer-Verlag, p. 211–228, accessed March 29, 2024, at https://link.springer.com/chapter/10.1007/978-1-4612-3748-8_11.
Osborne, W.E., 1998, Geologic map of the Eastaboga, Alabama 7.5-minute quadrangle: Geological Survey of Alabama Quadrangle Series Map 11, pl. 2, scale 1:24,000, accessed March 29, 2024, at https://ngmdb.usgs.gov/Prodesc/proddesc_67813.htm.
Osborne, W.E., Brewer, M.C., and Ward, W.E., 2006, Geologic map of the McCalla 7.5-minute quadrangle, Jefferson and Tuscaloosa Counties, Alabama: Geological Survey of Alabama Quadrangle Series Map 43, 2 pls., scale 1:24,000, digital GIS data, accessed March 29, 2024, at https://www.ogb.state.al.us/gsa/geologic/mapping.
Osborne, W.E., and Irvin, G.D., 2002, Geologic map of the Montevallo 7.5-minute quadrangle, Chilton and Shelby Counties, Alabama: Geological Survey of Alabama Quadrangle Series Map 21, 2 pls., scale 1:24,000, accessed March 29, 2024, at https://www.ogb.state.al.us/gsa/geologic/mapping.
Osborne, W.E., Thomas, W.A., Astini, R.A., and Irvin, G.D., 2000, Stratigraphy of the Conasauga Formation and equivalent units, Appalachian thrust belt in Alabama, in Osborne, W.E., Thomas, W.A., and Astini, R.A., eds., The Conasauga Formation and equivalent units in the Appalachian thrust belt in Alabama—A guidebook for the 37th annual field trip of the Geological Society of Alabama: Tuscaloosa, Ala., Alabama Geological Society, v. 37, 17 p., accessed March 29, 2024, at https://archives.datapages.com/data/alabama/52/01.htm.
Osborne, W.E., Ward, W.E., and Irvin, G.D., 1998, Geologic map of the Alabaster 7.5-minute quadrangle, Shelby County, Alabama: Geological Survey of Alabama Quadrangle Series Map 16, 2 pls., scale 1:24,000, accessed March 29, 2024, at https://www.ogb.state.al.us/gsa/geologic/mapping.
Pashin, J.C., 2010, Devonian shale plays of the Black Warrior basin and Appalachian thrust belt in Alabama: American Association of Petroleum Geologists Datapages, Search and Discovery Article 80095, 22 p., accessed March 29, 2024, at https://www.searchanddiscovery.com/documents/2010/80095pashin/ndx_pashin.pdf.
Pashin, J.C., Carroll, R.E., Barnett, R.L., and Beg, M.A., 1995, Geology and coal resources of the Cahaba coal field: Geological Survey of Alabama Bulletin 163, 49 p., 2 pls., accessed March 29, 2024, at https://www.gsa.state.al.us/ogb/publications/B163/.
Pashin, J.C., Kopaska-Merkel, D.C., Arnold, A.C., and McIntyre, M.R., 2011, Geological foundation for production of natural gas from diverse shale formations: Geological Survey of Alabama Open-File Report 1110, 156 p., 3 pls. [Research Partnership to Secure Energy for America Final Report 07122.17.01, New Orleans, La., Annual Conference and Exposition.]
Pashin, J.C., Kopaska-Merkel, D.C., Arnold, A.C., McIntyre, M.R., and Thomas, W.A., 2012, Gigantic, gaseous mushwads in Cambrian shale—Conasauga Formation, southern Appalachians, USA: International Journal of Coal Geology, v. 103, p. 70–91, accessed March 29, 2024, at https://doi.org/10.1016/j.coal.2012.05.010.
Pashin, J.C., McIntyre-Redden, M.R., Mann, S.D., Kopaska-Merkel, D.C., Varonka, M., and Orem, W., 2014, Relationships between water and gas chemistry in mature coalbed methane reservoirs of the Black Warrior basin: International Journal of Coal Geology, v. 125, p. 92–105, accessed March 29, 2024, at https://doi.org/10.1016/j.coal.2013.10.002.
Pendexter, W.S., 1982, Stratigraphic relationships of the carbonate sequence in the Talladega slate belt, Chilton and Coosa Counties, Alabama, in Bearce, D.N., Black, W.W., Kish, S.A., and Tull, J.F., eds., Geological Society of America Special Paper 191, p. 63–65, accessed March 29, 2024, at https://doi.org/10.1130/SPE191.
Raymond, D.E., 1991a, More wells will expand knowledge of Knox Group, Black Warrior basin: Oil and Gas Journal, v. 89, no. 20, p. 58–62, accessed March 29, 2024, at https://www.ogj.com/home/article/17238227/more-wells-will-expand-knowledge-of-knox-group-black-warrior-basin.
Raymond, D.E., 1991b, New subsurface information on Paleozoic stratigraphy of the Alabama fold and thrust belt and the Black Warrior basin: Geological Survey of Alabama Bulletin 143, 185 p., accessed March 29, 2024, at https://www.gsa.state.al.us/ogb/publications/B143/.
Raymond, D.E., and Copeland, C.W., 1987, Selected columnar sections for the Coastal Plain, Appalachian Plateaus, Interior Low Plateaus, and Valley and Ridge provinces in Alabama: Geological Survey of Alabama Atlas Series 20, 43 p., accessed March 29, 2024, at https://www.gsa.state.al.us/Home/DownloadPubDocument/?path=Atlas_Series&fileName=AS020.pdf.
Raymond, D.E., Osborne, W.E., Copeland, C.W., and Neathery, T.L., 1988, Alabama stratigraphy: Geological Survey of Alabama Circular 140, 97 p., 1 pl., accessed March 29, 2024, at https://www.gsa.state.al.us/Home/DownloadPubDocument/?path=Circulars&fileName=C140.pdf and https://www.gsa.state.al.us/ppm/?image=C140p1.jp2.
Read, J.F., and Repetski, J.E., 2012, Cambrian-lower Middle Ordovician passive carbonate margin, southern Appalachians, in Derby, J.R., Fritz, R.D., Longacre, S.A., Morgan, W.A., and Sternbach, C.A., eds., The great American carbonate bank—The geology and economic resources of the Cambrian-Ordovician Sauk megasequence of Laurentia: American Association of Petroleum Geologists Memoir, v. 98, p. 357–382, accessed March 29, 2024, at https://doi.org/10.1306/13331499M980271.
Reed, P.C., 1972, Geology of Perry County, Alabama: Geological Survey of Alabama Special Map 118, 1 sheet, scale 1:126,000, accessed March 29, 2024, at https://www.gsa.state.al.us/ogb/publications/SM118/.
Reed, P.C., Willmon, J.R., and Jefferson, P.O., 1975, Water availability in Perry County, Alabama: U.S. Geological Survey Open-File Report 75–482, 24 p., accessed March 29, 2024, at https://pubs.er.usgs.gov/publication/ofr75482.
Robinson, D.M., Bailey, R.M., and Goodliffe, A.M., 2012, Structure of the Alleghanian thrust belt under the Gulf Coastal Plain of Alabama: Gulf Coast Association of Geological Societies Journal, v. 1, p. 44–54, accessed March 29, 2024, at http://www.gcags.org/Journal/2012.gcags.journal/GCAGS.Journal.2012.vol1.p44-54.Robinson.pdf.
Rodgers, J., 1953, Geologic map of east Tennessee with explanatory text [reprinted in 1993]: Tennessee Division of Geology Bulletin 58, pt. II, p. 43, accessed March 29, 2024, at https://www.tn.gov/content/dam/tn/environment/geology/documents/geology_bulletin-58_2text.pdf.
Rodgers, J., 1963, Mechanics of Appalachian foreland folding in Pennsylvania and West Virginia: American Association of Petroleum Geologists Bulletin, v. 47, no. 8, p. 1527–1536, accessed March 29, 2024, at http://archives.datapages.com/data/bulletns/1961-64/images/pg/00470008/1500/15270.pdf.
Ryder, R.T., Crangle, R.D., Jr., Trippi, M.H., Swezey, C.S., Lentz, E.E., Rowan, E.L., and Hope, R.S., 2009, Geologic cross section D‒Dʹ through the Appalachian basin from the Findlay arch, Sandusky County, Ohio, to the Valley and Ridge province, Hardy County, West Virginia: U.S. Geological Survey Scientific Investigations Map 3067, 2 sheets, 52-p. text, accessed March 29, 2024, at https://doi.org/10.3133/sim3067.
Ryder, R.T., Swezey, C.S., Crangle, R.D., Jr., and Trippi, M.H., 2008, Geologic cross section E–Eʹ through the Appalachian basin from the Findlay arch, Wood County, Ohio, to the Valley and Ridge province, Pendleton County, West Virginia: U.S. Geological Survey Scientific Investigations Map 2985, 2 sheets, 48-p. text, accessed March 29, 2024, at https://doi.org/10.3133/sim2985.
Ryder, R.T., Trippi, M.H., and Swezey, C.S., 2015, Geologic cross section I–I′ through the Appalachian basin from the eastern margin of the Illinois basin, Jefferson County, Kentucky, to the Valley and Ridge province, Scott County, Virginia: U.S. Geological Survey Scientific Investigations Map 3343, 2 sheets and pamphlet A, 41 p.; pamphlet B, 102 p., accessed March 29, 2024, at https://doi.org/10.3133/sim3343.
Ryder, R.T., Trippi, M.H., Swezey, C.S., Crangle, R.D., Jr., Hope, R.S., Rowan, E.L., and Lentz, E.E., 2012, Geologic cross section C–C' through the Appalachian basin from Erie County, north-central Ohio, to the Valley and Ridge province, Bedford County, south-central Pennsylvania: U.S. Geological Survey Scientific Investigations Map 3172, 2 sheets, 70-p. pamphlet, accessed March 29, 2024, at https://doi.org/10.3133/sim3172.
Shahkaramia, S., Buatoisa, L.A., Mánganoa, M.G., Hagadorn, J.W., and Almond, J., 2020, The Ediacaran–Cambrian boundary—Evaluating stratigraphic completeness and the Great Unconformity: Precambrian Research, v. 345, 26 p., accessed March 29, 2024, at https://doi.org/10.1016/j.precamres.2020.105721.
Sloss, L.L., 1988, Tectonic evolution of the craton in Phanerozoic time, in Sloss, L.L., ed., Sedimentary cover—North American craton, U.S., v. D‒2 of The geology of North America: Boulder, Colo., Geological Society of America, p. 25‒51, accessed March 29, 2024, at https://doi.org/10.1130/DNAG-GNA-D2.
Smith, L.A., Gupta, N., Sass, B.M., Bubenik, T.A., Byrer, C., and Bergman, P., 2002, Engineering and economic assessment of carbon dioxide sequestration in saline formations: Journal of Energy and Environmental Research, v. 2, no. 1, p. 5‒22, accessed March 29, 2024, at https://www.netl.doe.gov/publications/proceedings/01/carbon_seq/4a3.pdf.
Steltenpohl, M.G., Horton, J.W., Jr., Hatcher, R.D., Jr., Zietz, I., Daniels, D.L., and Higgins, M.W., 2013, Upper crustal structure of Alabama from regional magnetic and gravity data—Using geology to interpret geophysics, and vice versa: Geosphere, v. 9, no. 4, p. 1044–1064, accessed March 29, 2024, at https://doi.org/10.1130/GES00703.1.
Sternbach, L.R., and Friedman, G.M., 1984, Depositions of ooid shoals marginal to the late Cambrian proto-Atlantic (Iapetus) ocean in New York and Alabama—Influence on the interior shelf, in Harris, P.M., ed., Carbonate sand shoals: Society of Economic Paleontologists and Mineralogists Core Workshop No. 5, San Antonio, Tex., May 19–20, 1984, Tulsa, Okla., Society of Economic Paleontologists and Mineralogists, accessed March 29, 2024, at https://doi.org/10.2110/cor.84.05.0002.
Surles, D.M., 2007, Interactions between structures in the Appalachian and Ouachita foreland beneath the Gulf Coastal Plain: Lexington, Ky., University of Kentucky, Ph.D. dissertation 554, 156 p., 6 pls., accessed March 29, 2024, at https://uknowledge.uky.edu/gradschool_diss/554.
Swezey, C.S., 2002, Regional stratigraphy and petroleum systems of the Appalachian basin, North America: U.S. Geological Survey Scientific Investigations Map I–2768, 1 sheet, accessed March 29, 2024, at https://doi.org/10.3133/i2768.
Szabo, M.W., 1972, Mineral resources map of Perry County, Alabama: Geological Survey of Alabama Special Map 115, 1 sheet, scale 1:126,720, accessed March 29, 2024, at https://www.gsa.state.al.us/ogb/publications/SM115/.
Szabo, M.W., Daniel, T.W., Jr., and Chaffin, H.S., Jr., 1976, Mineral resources map of Bibb County, Alabama: Geological Survey of Alabama Special Map 119, 1 sheet, scale 1:125,000, accessed March 29, 2024, at https://www.gsa.state.al.us/ogb/publications/SM119/.
Szabo, M.W., Osborne, E.W., and Copeland, C.W., 1988. Geologic map of Alabama: Geological Survey of Alabama Special Map 220, northwest sheet, scale 1:250,000, accessed March 29, 2024, at https://www.gsa.state.al.us/ogb/publications/SM220_1/.
Thomas, W.A., 2008, A mushwad of Floyd Shale in the Appalachian thrust belt in Alabama—Combining the structure of the Conasauga gas play with the stratigraphy of the Floyd gas play: American Association of Petroleum Geologists Datapages, Search and Discovery Article 10150, 28 p. [Also available at https://www.searchanddiscovery.com/pdfz/documents/2008/08236thomas/ndx_thomas.pdf.html.]
Thomas, W.A., 2018, Evolution of the concept and structure of a MUSHWAD: Journal of Structural Geology, v. 125, p. 311–318, accessed March 29, 2024, at https://doi.org/10.1016/j.jsg.2018.05.001.
Thomas, W.A., 2010, Interactions between the southern Appalachian–Ouachita orogenic belt and basement faults in the orogenic footwall and foreland, in Tollo, R.P., Bartholomew, M.J., Hibbard, J.P., and Karabinos, P.M., eds., From Rodinia to Pangea—The lithotectonic record of the Appalachian region: Geological Society of America Memoir 206, p. 897–916, accessed March 29, 2024, at https://doi.org/10.1130/2010.1206(34).
Thomas, W.A., 1972, Mississippian stratigraphy of Alabama: Geological Survey of Alabama Monograph 12, 121 p., accessed March 29, 2024, at https://www.gsa.state.al.us/Home/DownloadPubDocument/?path=Monographs&fileName=M012.pdf.
Thomas, W.A., 1979, Mississippian stratigraphy of Alabama, chap. I in The Mississippian and Pennsylvanian (Carboniferous) Systems in the United States: U.S. Geological Survey Professional Paper 1110–A–L, p. I1–I22, accessed March 29, 2024, at https://pubs.usgs.gov/pp/1110a-l/report.pdf.
Thomas, W.A., 2001, Mushwad—Ductile duplex in the Appalachian thrust belt in Alabama: American Association of Petroleum Geologists Bulletin 85, no. 10, p. 1847–1869, accessed March 29, 2024, at https://pubs.geoscienceworld.org/aapgbull/article/85/10/1847/39851/Mushwad-Ductile-Duplex-in-the-Appalachian-Thrust.
Thomas, W.A., 2007, Role of the Birmingham basement fault in thin-skinned thrusting of the Birmingham anticlinorium, Appalachian thrust belt in Alabama: American Journal of Science, v. 307, no. 1, p. 46–62, accessed March 29, 2024, at https://doi.org/10.2475/01.2007.03.
Thomas, W.A., 1991, The Appalachian‐Ouachita rifted margin of southeastern North America: Geological Society of America Bulletin 103, no. 3, p. 415–431, accessed March 29, 2024, at https://doi.org/10.1130/0016-7606(1991)103<0415:TAORMO>2.3.CO;2.
Thomas, W.A., 1988, The Black Warrior basin, in Sloss, L.L., ed., Sedimentary cover—North American craton, U.S., v. D–2 of The geology of North America: Boulder, Colo., Geological Society of America, p. 471–492, accessed March 29, 2024, at https://doi.org/10.1130/DNAG-GNA-D2.471.
Thomas, W.A., Astini, R.A., and Denison, R.E., 2001, Strontium isotopes, age, and tectonic setting of Cambrian salinas along the rift and transform margins of the Argentine Precordillera and southern Laurentia: The Journal of Geology, v. 109, no. 2, p. 231–246, accessed March 29, 2024, at https://doi.org/10.1086/319241.
Thomas, W.A., and Pashin, J.C., 2011, Of mushwads and Mayhem; disharmonically deformed shale in the southern Appalachian thrust belt—A guidebook for the 48th annual field trip of the Alabama Geological Society: Tuscaloosa, Ala., Geological Survey of Alabama, 126 p., accessed March 29, 2024, at https://www.alageolsoc.org/uploads/3/4/4/4/34440427/field_trip_guidebook_list_full_list.pdf.
Trippi, M.H., Kinney, S.A., Gunther, G., Ryder, R.T., and Ruppert, L.F., 2014, Digital data in support of studies and assessments of coal and petroleum resources in the Appalachian basin, chap. I.1 of Ruppert, L.F., and Ryder, R.T., eds., Coal and petroleum resources in the Appalachian basin—Distribution, geologic framework, and geochemical character: U.S. Geological Survey Professional Paper 1708, 11 p., database files, accessed March 29, 2024, at https://doi.org/10.3133/pp1708.
Trippi, M.H., Ryder, R.T., and Enomoto, C.B., 2019, Geologic cross section A–A′ through the Appalachian basin from the southern margin of the Ontario lowlands province, Genesee County, western New York, to the Valley and Ridge province, Lycoming County, north-central Pennsylvania: U.S. Geological Survey Scientific Investigations Map 3425, 2 sheets, 74-p. pamphlet, accessed March 29, 2024, at https://pubs.er.usgs.gov/publication/sim3425.
Tull, J.F., Allison, D.T., Whiting, S.E., and John, N.L., 2010, Southern Appalachian Laurentian margin initial drift-facies sequences—Implications for margin evolution, in Tollo, R.P., Bartholomew, M.J., Hibbard, J.P., and Karabinos, P.M., eds., From Rodinia to Pangea—The lithotectonic record of the Appalachian region: Geological Society of America Memoir 206, p. 935–956, accessed March 29, 2024, at https://doi.org/10.1130/2010.1206(36).
Tull, J.F., Harris, A.G., Repetski, J.E., McKinney, F.K., Garrett, C.B., and Bearce, D.N., 1988, New paleontologic evidence constraining the age and paleotectonic setting of the Talladega slate belt, southern Appalachians: Geological Society of America Bulletin 100, no. 8, p. 1291–1299, accessed March 29, 2024, at https://doi.org/10.1130/0016-7606(1988)100<1291:NPECTA>2.3.CO;2.
Wahl, K.D., 1966, Geology and ground-water resources of Greene County, Alabama: Geological Survey of Alabama Bulletin 86, 93 p., accessed March 29, 2024, at https://www.gsa.state.al.us/Home/DownloadPubDocument/?path=Bulletins&fileName=B086.pdf.
Wanless, H.R., 1975, Appalachian region, chap. C in McKee, E.D., Crosby, E.J., Bachman, G.O., Bell, K.G., Dixon, G.H., Frezon, S.E., Glick, E.E., Irwin, W.P., Mallory, W.W., Mapel, W.J., Maughan, E.K., Pritchard, G.E., Shideler, G.L., Stewart, G.F., Wanless, H.R., and Wilson, R.F., Paleotectonic investigations of the Pennsylvanian System in the United States, part 1—Introduction and regional analyses of the Pennsylvanian System: U.S. Geological Survey Professional Paper 853–1, p. 17–62, 14 pls., accessed March 29, 2024, at https://doi.org/10.3133/pp8531.
Ward, W.E., II, 1984, Reserve base of bituminous coal and lignite in Alabama: Geological Survey of Alabama Circular 118, 102 p., accessed March 29, 2024, at https://gsa.state.al.us/ogb/publications/C118/.
Appendix 1. Stratigraphic Units and Depths of Stratigraphic Units for Drill Holes 201–208 in Cross Section N–N'
Table 1.1.
Stratigraphic units and depths of stratigraphic units for drill holes 201–208 in cross section N–N'.[Blank cells indicate no formation was encountered in drill hole. DF, drill floor; ft, feet; GL, ground level; KB, kelly bushing; n.a., not applicable; No., number; SL, sea level. API numbers, well numbers and names, permanent datum values, KB elevations, DF elevations, GL elevations, formation names, and system, series, and (or) stage names come from well logs and (or) the Geological Survey of Alabama website (https://www.gsa.state.al.us/); formation top and thickness values are interpretations by the authors of this report]
Drill hole number | 201 | 202 | 203 | 204 | 205 | 206 | 207 | 208 |
---|---|---|---|---|---|---|---|---|
API no. (from State) | 01-063-20041 | 01-063-20003 | 01-063-20031 | 01-065-20001 | 01-007-20001 | 01-007-20004 | 01-007-20220 | 01-007-20002 |
Well number and name | No. 1 Tate 9-4 | No. 1 Ethel M. Koch 10-6 | No. 1 Bayne-Etheridge 36-1 | No. 1 Burke 29-7 | No. 1 John Goodson 9-7 | No. 1 University of Alabama 4-7 | No. 1 Gulf States Paper Company 10-12-60 | No. 1 Gulf States Paper Company 27-15 |
Permanent datum | GL | GL | GL | GL | GL | GL | GL | GL |
KB elevation (ft) | 157 | 175 | 126 | 259 | 229 | 497 | n.a. | 440 |
DF elevation (ft) | 156 | 174 | 125 | 258 | 228 | 496 | n.a. | 439 |
GL elevation (ft) | 141 | 147 | 106 | 241 | 208 | 484 | 491 | 429 |
Formation 1 | Terrace deposits | Terrace deposits | Terrace deposits | Eutaw Formation | Alluvial deposits | Pottsville Formation (above Shades Sandstone Member) | Pottsville Formation (above Shades Sandstone Member) | Pottsville Formation (above Shades Sandstone Member) |
System, Series, and (or) Stage | Pleistocene | Pleistocene | Pleistocene | Upper Cretaceous (Campanian) | Holocene | Lower to Middle Pennsylvanian (Morrowan to Atokan) | Lower to Middle Pennsylvanian (Morrowan to Atokan) | Lower to Middle Pennsylvanian (Morrowan to Atokan) |
Formation top (relative to KB) | -16 | -28 | -20 | -18 | -21 | -13 | n.a. | -11 |
Formation top (relative to GL) | 0 | 0 | 0 | 0 | 0 | 0 | 0 | 0 |
Formation top (relative to SL) | 141 | 147 | 106 | 241 | 208 | 484 | 491 | 429 |
Thickness (ft) | 74 | 52 | 40 | 372 | 29 | 7,362 | 5,000 | 4,839 |
Formation 2 | Mooreville Chalk of Selma Group | Mooreville Chalk of Selma Group | Mooreville Chalk of Selma Group | Gordo Formation of Tuscaloosa Group | Coker Formation of Tuscaloosa Group | Shades Sandstone Member of Pottsville Formation | Shades Sandstone Member of Pottsville Formation | |
System, Series, and (or) Stage | Upper Cretaceous (Campanian) | Upper Cretaceous (Campanian) | Upper Cretaceous (Campanian) | Upper Cretaceous (Coniacian) | Upper Cretaceous (Turonian) | Lower Pennsylvanian (Morrowan) | Lower Pennsylvanian (Morrowan) | |
Formation top (relative to KB) | -90 | -80 | -60 | -390 | -50 | -7,375 | -4,850 | |
Formation top (relative to GL) | -74 | -52 | -40 | -372 | -29 | -7,362 | -4,839 | |
Formation top (relative to SL) | 67 | 95 | 66 | -131 | 179 | -6,878 | -4,410 | |
Thickness (ft) | 320 | 260 | 120 | 240 | 100 | 230 | 260 | |
Formation 3 | Eutaw Formation | Eutaw Formation | Eutaw Formation | Coker Formation of Tuscaloosa Group | Conasauga Formation (in Rock Creek School thrust sheet of Butts [1940]) | Parkwood Formation | Parkwood Formation | |
System, Series, and (or) Stage | Upper Cretaceous (Campanian) | Upper Cretaceous (Campanian) | Upper Cretaceous (Campanian) | Upper Cretaceous (Turonian) | middle Cambrian | Lower Pennsylvanian (Morrowan) | Lower Pennsylvanian (Morrowan) | |
Formation top (relative to KB) | -410 | -340 | -180 | -630 | -150 | -7,605 | -5,110 | |
Formation top (relative to GL) | -394 | -312 | -160 | -612 | -129 | -7,592 | -5,099 | |
Formation top (relative to SL) | -253 | -165 | -54 | -371 | 79 | -7,108 | -4,670 | |
Thickness (ft) | 430 | 430 | 430 | 870 | 1,440 | 700 | 510 | |
Formation 4 | Gordo Formation of Tuscaloosa Group | Gordo Formation of Tuscaloosa Group | Gordo Formation of Tuscaloosa Group | Undivided Lower Cretaceous formations | Rome Formation (in Rock Creek School thrust sheet of Butts [1940]) | Floyd Shale | Floyd Shale | |
System, Series, and (or) Stage | Upper Cretaceous (Coniacian) | Upper Cretaceous (Coniacian) | Upper Cretaceous (Coniacian) | Lower Cretaceous | lower Cambrian | Upper Mississippian (Chesterian) | Upper Mississippian (Chesterian) | |
Formation top (relative to KB) | -840 | -770 | -610 | -1,500 | -1,590 | -8,305 | -5,620 | |
Formation top (relative to GL) | -824 | -742 | -590 | -1,482 | -1,569 | -8,292 | -5,609 | |
Formation top (relative to SL) | -683 | -595 | -484 | -1,241 | -1,361 | -7,808 | -5,180 | |
Thickness (ft) | 280 | 280 | 240 | 210 | 1,050 | 195 | 485 | |
Formation 5 | Coker Formation of Tuscaloosa Group | Coker Formation of Tuscaloosa Group | Coker Formation of Tuscaloosa Group | Pottsville Formation (above Shades Sandstone Member) | Chepultepec Dolomite of Knox Group (in Helena thrust sheet of Butts [1940]) | Fort Payne Chert and Tuscumbia Limestone, undivided | ||
System, Series, and (or) Stage | Upper Cretaceous (Turonian) | Upper Cretaceous (Turonian) | Upper Cretaceous (Turonian) | Lower to Middle Pennsylvanian (Morrowan to Atokan) | Lower Ordovician (Ibexian [Canadian]) | Middle Mississippian (Meramecian) | ||
Formation top (relative to KB) | -1,120 | -1,050 | -850 | -1,710 | -2,640 | -6,105 | ||
Formation top (relative to GL) | -1,104 | -1,022 | -830 | -1,692 | -2,619 | -6,094 | ||
Formation top (relative to SL) | -963 | -875 | -724 | -1,451 | -2,411 | -5,665 | ||
Thickness (ft) | 862 | 840 | 830 | 5,485 | 760 | 183 | ||
Formation 6 | Undivided Lower Cretaceous formations | Undivided Lower Cretaceous formations | Undivided Lower Cretaceous formations | Shades Sandstone Member of Pottsville Formation | Copper Ridge Dolomite of Knox Group, undivided (in Helena thrust sheet of Butts [1940]) | Undivided Devonian formations | ||
System, Series, and (or) Stage | Lower Cretaceous | Lower Cretaceous | Lower Cretaceous | Lower Pennsylvanian (Morrowan) | upper Cambrian (Trempealeauan) | Upper Devonian (Senecan) | ||
Formation top (relative to KB) | -1,982 | -1,890 | -1,680 | -7,195 | -3,400 | -6,288 | ||
Formation top (relative to GL) | -1,966 | -1,862 | -1,660 | -7,177 | -3,379 | -6,277 | ||
Formation top (relative to SL) | -1,825 | -1,715 | -1,554 | -6,936 | -3,171 | -5,848 | ||
Thickness (ft) | 653 | 680 | 670 | 245 | 720 | 30 | ||
Formation 7 | Pottsville Formation (above Shades Sandstone Member) | Pottsville Formation (above Shades Sandstone Member) | Pottsville Formation (above Shades Sandstone Member) | Parkwood Formation | Pottsville Formation (above Shades Sandstone Member) | Red Mountain Formation | ||
System, Series, and (or) Stage | Lower to Middle Pennsylvanian (Morrowan to Atokan) | Lower to Middle Pennsylvanian (Morrowan to Atokan) | Lower to Middle Pennsylvanian (Morrowan to Atokan) | Lower Pennsylvanian (Morrowan) | Lower to Middle Pennsylvanian (Morrowan to Atokan) | Silurian (Pridoli) | ||
Formation top (relative to KB) | -2,635 | -2,570 | -2,350 | -7,440 | -4,120 | -6,318 | ||
Formation top (relative to GL) | -2,619 | -2,542 | -2,330 | -7,422 | -4,099 | -6,307 | ||
Formation top (relative to SL) | -2,478 | -2,395 | -2,224 | -7,181 | -3,891 | -5,878 | ||
Thickness (ft) | 500 | 630 | 3,340 | 950 | 2,210 | 202 | ||
Formation 8 | Shades Sandstone Member of Pottsville Formation | Shades Sandstone Member of Pottsville Formation | Shades Sandstone Member of Pottsville Formation | Floyd Shale | Shades Sandstone Member of Pottsville Formation | Chickamauga Limestone | ||
System, Series, and (or) Stage | Lower Pennsylvanian (Morrowan) | Lower Pennsylvanian (Morrowan) | Lower Pennsylvanian (Morrowan) | Upper Mississippian (Chesterian) | Lower Pennsylvanian (Morrowan) | Upper Ordovician (Shermanian) | ||
Formation top (relative to KB) | -3,135 | -3,200 | -5,690 | -8,390 | -6,330 | -6,520 | ||
Formation top (relative to GL) | -3,119 | -3,172 | -5,670 | -8,372 | -6,309 | -6,509 | ||
Formation top (relative to SL) | -2,978 | -3,025 | -5,564 | -8,131 | -6,101 | -6,080 | ||
Thickness (ft) | 155 | 250 | 220 | 707 | 240 | 650 | ||
Formation 9 | Parkwood Formation | Parkwood Formation | Parkwood Formation | Fort Payne Chert and Tuscumbia Limestone, undivided | Parkwood Formation | Chepultepec Dolomite of Knox Group | ||
System, Series, and (or) Stage | Lower Pennsylvanian (Morrowan) | Lower Pennsylvanian (Morrowan) | Lower Pennsylvanian (Morrowan) | Middle Mississippian (Meramecian) | Lower Pennsylvanian (Morrowan) | Lower Ordovician (Ibexian [Canadian]) | ||
Formation top (relative to KB) | -3,290 | -3,450 | -5,910 | -9,097 | -6,570 | -7,170 | ||
Formation top (relative to GL) | -3,274 | -3,422 | -5,890 | -9,079 | -6,549 | -7,159 | ||
Formation top (relative to SL) | -3,133 | -3,275 | -5,784 | -8,838 | -6,341 | -6,730 | ||
Thickness (ft) | 795 | 840 | 860 | 103 | 690 | 1,495 | ||
Formation 10 | Floyd Shale | Floyd Shale | Floyd Shale | Undivided Devonian formations | Floyd Shale | |||
System, Series, and (or) Stage | Upper Mississippian (Chesterian) | Upper Mississippian (Chesterian) | Upper Mississippian (Chesterian) | Upper Devonian (Senecan) | Upper Mississippian (Chesterian) | |||
Formation top (relative to KB) | -4,085 | -4,290 | -6,770 | -9,200 | -7,260 | |||
Formation top (relative to GL) | -4,069 | -4,262 | -6,750 | -9,182 | -7,239 | |||
Formation top (relative to SL) | -3,928 | -4,115 | -6,644 | -8,941 | -7,031 | |||
Thickness (ft) | 943 | 559 | 645 | 1,159 | 515 | |||
Formation 11 | Fort Payne Chert and Tuscumbia Limestone, undivided | Fort Payne Chert and Tuscumbia Limestone, undivided | Fort Payne Chert and Tuscumbia Limestone, undivided | Red Mountain Formation | Fort Payne Chert and Tuscumbia Limestone, undivided | |||
System, Series, and (or) Stage | Middle Mississippian (Meramecian) | Middle Mississippian (Meramecian) | Middle Mississippian (Meramecian) | Silurian (Pridoli) | Middle Mississippian (Meramecian) | |||
Formation top (relative to KB) | -5,028 | -4,849 | -7,415 | -10,359 | -7,775 | |||
Formation top (relative to GL) | -5,012 | -4,821 | -7,395 | -10,341 | -7,754 | |||
Formation top (relative to SL) | -4,871 | -4,674 | -7,289 | -10,100 | -7,546 | |||
Thickness (ft) | 307 | 193 | 174 | 614 | 165 | |||
Formation 12 | Undivided Devonian formations | Undivided Devonian formations | Undivided Devonian formations | Chickamauga Limestone | Undivided Devonian formations | |||
System, Series, and (or) Stage | Upper Devonian (Senecan) | Upper Devonian (Senecan) | Upper Devonian (Senecan) | Upper Ordovician (Shermanian) | Upper Devonian (Senecan) | |||
Formation top (relative to KB) | -5,335 | -5,042 | -7,589 | -10,973 | -7,940 | |||
Formation top (relative to GL) | -5,319 | -5,014 | -7,569 | -10,955 | -7,919 | |||
Formation top (relative to SL) | -5,178 | -4,867 | -7,463 | -10,714 | -7,711 | |||
Thickness (ft) | 1,272 | 1,535 | 1,251 | 557 | 130 | |||
Formation 13 | Red Mountain Formation | Red Mountain Formation | Red Mountain Formation | Longview and Newala Limestones of Knox Group, undivided | Red Mountain Formation | |||
System, Series, and (or) Stage | Silurian (Pridoli) | Silurian (Pridoli) | Silurian (Pridoli) | Lower Ordovician (Ibexian [Canadian]) | Silurian (Pridoli) | |||
Formation top (relative to KB) | -6,607 | -6,577 | -8,840 | -11,530 | -8,070 | |||
Formation top (relative to GL) | -6,591 | -6,549 | -8,820 | -11,512 | -8,049 | |||
Formation top (relative to SL) | -6,450 | -6,402 | -8,714 | -11,271 | -7,841 | |||
Thickness (ft) | 490 | 447 | 505 | 120 | 230 | |||
Formation 14 | Chickamauga Limestone | Chickamauga Limestone | Chickamauga Limestone | Chepultepec Dolomite of Knox Group | Chickamauga Limestone | |||
System, Series, and (or) Stage | Upper Ordovician (Shermanian) | Upper Ordovician (Shermanian) | Upper Ordovician (Shermanian) | Lower Ordovician (Ibexian [Canadian]) | Upper Ordovician (Shermanian) | |||
Formation top (relative to KB) | -7,097 | -7,024 | -9,345 | -11,650 | -8,300 | |||
Formation top (relative to GL) | -7,081 | -6,996 | -9,325 | -11,632 | -8,279 | |||
Formation top (relative to SL) | -6,940 | -6,849 | -9,219 | -11,391 | -8,071 | |||
Thickness (ft) | 443 | 476 | 201 | 375 | 1,040 | |||
Formation 15 | Longview and Newala Limestones of Knox Group, undivided | Longview and Newala Limestones of Knox Group, undivided | Chepultepec Dolomite of Knox Group | |||||
System, Series, and (or) Stage | Lower Ordovician (Ibexian [Canadian]) | Lower Ordovician (Ibexian [Canadian]) | Lower Ordovician (Ibexian [Canadian]) | |||||
Formation top (relative to KB) | -7,540 | -7,500 | -9,340 | |||||
Formation top (relative to GL) | -7,524 | -7,472 | -9,319 | |||||
Formation top (relative to SL) | -7,383 | -7,325 | -9,111 | |||||
Thickness (ft) | 1,094 | 1,110 | 1,440 | |||||
Formation 16 | Chepultepec Dolomite of Knox Group | Chepultepec Dolomite of Knox Group | Copper Ridge Dolomite of Knox Group | |||||
System, Series, and (or) Stage | Lower Ordovician (Ibexian [Canadian]) | Lower Ordovician (Ibexian [Canadian]) | upper Cambrian (Trempealeauan) | |||||
Formation top (relative to KB) | -8,634 | -8,610 | -10,780 | |||||
Formation top (relative to GL) | -8,618 | -8,582 | -10,759 | |||||
Formation top (relative to SL) | -8,477 | -8,435 | -10,551 | |||||
Thickness (ft) | 1,996 | 1,970 | 417 | |||||
Formation 17 | Copper Ridge Dolomite of Knox Group | Copper Ridge Dolomite of Knox Group | ||||||
System, Series, and (or) Stage | upper Cambrian (Trempealeauan) | upper Cambrian (Trempealeauan) | ||||||
Formation top (relative to KB) | -10,630 | -10,580 | ||||||
Formation top (relative to GL) | -10,614 | -10,552 | ||||||
Formation top (relative to SL) | -10,473 | -10,405 | ||||||
Thickness (ft) | 96 | 2,080 | ||||||
Formation 18 | Ketona Dolomite | |||||||
System, Series, and (or) Stage | upper Cambrian (Franconian) | |||||||
Formation top (relative to KB) | -12,660 | |||||||
Formation top (relative to GL) | -12,632 | |||||||
Formation top (relative to SL) | -12,485 | |||||||
Thickness (ft) | 360 | |||||||
Formation 19 | Conasauga Formation | |||||||
System, Series, and (or) Stage | middle Cambrian | |||||||
Formation top (relative to KB) | -13,020 | |||||||
Formation top (relative to GL) | -12,992 | |||||||
Formation top (relative to SL) | -12,845 | |||||||
Thickness (ft) | 2,020 | |||||||
Formation 20 | Ketona Dolomite (below thrust fault) | |||||||
System, Series, and (or) Stage | upper Cambrian (Franconian) | |||||||
Formation top (relative to KB) | -15,040 | |||||||
Formation top (relative to GL) | -15,012 | |||||||
Formation top (relative to SL) | -14,865 | |||||||
Thickness (ft) | 280 | |||||||
Formation 21 | Conasauga Formation | |||||||
System, Series, and (or) Stage | middle Cambrian | |||||||
Formation top (relative to KB) | -15,320 | |||||||
Formation top (relative to GL) | -15,292 | |||||||
Formation top (relative to SL) | -15,145 | |||||||
Thickness (ft) | 182 | |||||||
Bottom depth (relative to KB) | -10,726 | -15,502 | -9,546 | -12,025 | -11,197 | -8,500 | n.a. | -8,665 |
Bottom depth (relative to GL) | -10,710 | -15,474 | -9,526 | -12,007 | -11,176 | -8,487 | -5,000 | -8,654 |
Bottom depth (relative to SL) | -10,569 | -15,327 | -9,420 | -11,766 | -10,968 | -8,003 | -4,509 | -8,225 |
Appendix 2. Scale, Units, and Depths for Gamma-Ray Logging Runs
Table 2.1.
Scale, units, and depths for gamma-ray logging runs.[API, American Petroleum Institute; ft, feet; GL, ground level; KB, kelly bushing; TD, total depth. Data comes from well logs]
Conversion Factors
Temperature in degrees Celsius (°C) may be converted to degrees Fahrenheit (°F) as follows: °F = (1.8 × °C) + 32.
Temperature in degrees Fahrenheit (°F) may be converted to degrees Celsius (°C) as follows: °C = (°F – 32) / 1.8.
Datums
Horizontal coordinate information is referenced to the North American Datum of 1983.
Elevation, as used in this report, refers to distance above or below mean sea level, or distance above or below kelly bushing.
Abbreviations
Ala.
Alabama
API
American Petroleum Institute
BKB
below kelly bushing
BMSL
below mean sea level
Co.
County
ft
foot
Ga
giga-annum, billion years before present
GL
ground level
GSA
Geological Survey of Alabama
K-Ar
potassium-argon
KB
kelly bushing
km
kilometer
LAS
log ASCII standard
m
meter
Ma
mega-annum, million years before present
Mcf
thousand cubic feet
Mcm
thousand cubic meters
mi
mile
MSL
mean sea level
mushwad
malleable, unctuous shale, weak layer accretion in a ductile duplex
NAD 83
North American Datum of 1983
NED
National Elevation Dataset
No.
number
USGS
U.S. Geological Survey
VRPA
Valley and Ridge province in Alabama
?
uncertain or questionable
Disclaimers
Any use of trade, firm, or product names is for descriptive purposes only and does not imply endorsement by the U.S. Government.
Although this information product, for the most part, is in the public domain, it also may contain copyrighted materials as noted in the text. Permission to reproduce copyrighted items must be secured from the copyright owner.
Suggested Citation
Trippi, M.H., Coleman, J.L., and Ryder, R.T., 2024, Cross section N–N' through the Valley and Ridge province of the southern Appalachian basin, from Greene County, west-central Alabama, to Bibb County, central Alabama: U.S. Geological Survey Scientific Investigations Map 3524, 1 sheet, 51-p. pamphlet, https://doi.org/10.3133/sim3524.
ISSN: 2329-132X (online)
Study Area
Publication type | Report |
---|---|
Publication Subtype | USGS Numbered Series |
Title | Cross section N–N' through the Valley and Ridge province of the southern Appalachian basin, from Greene County, west-central Alabama, to Bibb County, central Alabama |
Series title | Scientific Investigations Map |
Series number | 3524 |
DOI | 10.3133/sim3524 |
Year Published | 2024 |
Language | English |
Publisher | U.S. Geological Survey |
Publisher location | Reston, VA |
Contributing office(s) | Geology, Energy & Minerals Science Center |
Description | Report: viii, 51 p.; 1 Sheet: 71.83 x 37.31 inches |
Country | United States |
State | Alabama |
Online Only (Y/N) | Y |
Additional Online Files (Y/N) | Y |
Google Analytic Metrics | Metrics page |