Assessment of Fecal Contamination Sources to Alley Creek, Queens County, New York, August 2020–June 2021
Links
- Document: Report (28.3 MB pdf) , HTML , XML
- Data Release: USGS data release - Assessment of fecal contamination sources to Alley Creek, Queens County, New York—Results from a sediment resuspension experiment, thermal imagery, and additional sample collection, 2020–2021
- Download citation as: RIS | Dublin Core
Acknowledgments
The authors would like to thank Kristin Ricigliano, Jorge Villacis, Edwige Lauture, Pinar Balci, and Abdulai Fofanah of the New York City Department of Environmental Protection for providing background data and documentation, assistance in the field, and feedback on this report. The authors would also like to acknowledge Daniel O'Rourke at CDM Smith for his assistance with project implementation.
Abstract
Alley Creek, a tributary to Little Neck Bay in Queens County, New York, has been designated by the New York State Department of Environmental Conservation as impaired (Class I) for fecal coliform because of pollution from combined sewer overflow, including stormwater runoff. The U.S. Geological Survey, in cooperation with the New York City Department of Environmental Protection, conducted a 1-year study from August 2020 to June 2021 using microbial source tracking (MST) methods to assess potential host sources of fecal contamination (for example, human, canine, and waterfowl) from the following: three outfall sites, TI–025, TI–008, and TI–024; an artesian well (Q277) adjacent to Alley Creek; and natural waters within the Alley Creek watershed and Little Neck Bay. In addition to analyzing for MST markers, field measurements such as water temperature and specific conductance, samples for total suspended solids, and fecal indicator bacteria (FIB; enterococci and fecal coliform) were collected. Pharmaceutical compounds were also collected for analysis, and the results of sampling were compared spatially and temporally to help support management decisions related to mitigation of fecal sources to Alley Creek. Factors that could affect concentrations, including tidal conditions, seasonality, and weather conditions, also were assessed. A sediment resuspension laboratory experiment was designed to replicate tidal activity in Alley Creek using sediment collected in the sewers and on the shoreline, as well as water collected from Oakland Lake. These sediment samples were assessed to understand the relation between sediment resuspension and FIB in the water column. The human MST markers used for this study, Bacteroides HF183/BacR287, and crAssphage CPQ_056 and CPQ_064, were detected in most samples (27 of 28) collected at the three outfall sites along Alley Creek, whereas the canine marker BacCan was less prevalent (20 of 28 samples) but exhibited a pattern of relative concentrations similar to the human markers. The waterfowl MST GFD marker was detected in 7 of 28 samples collected at the three outfall sites. Human MST markers were not detected at Oakland Lake (which drains through a combined sewer line to Alley Creek at TI–008), indicating minimal or nonexistent influence of sewage contamination in the lake. Groundwater samples collected from Q277 did not contain any MST markers, and concentrations of fecal coliform were less than 10 colony forming units per 100 milliliters. Although FIB did not correlate well with total suspended solids for individual sample sets, samples collected following precipitation and high-turbidity events were typically found to have higher concentrations of FIB than dry-weather samples. Results from the pharmaceutical compounds analysis provided additional evidence for determining known and suspected human sources when coupled with MST markers. Together, the MST, pharmaceutical, and FIB data generated by this study, along with supplementary data such as locations of point sources, locations of wildlife populations, and tidal exchange data, may provide reliable information on source identification and transport mechanisms of fecal contamination to Alley Creek.
Introduction
Alley Creek, a tributary to Little Neck Bay in Queens County, New York (fig. 1), is an urban waterway that has been designated by the New York State Department of Environmental Conservation (NYSDEC) as impaired (Class I) and is included on the 2018 303(d) List of Impaired Waterways (the Federal Clean Water Act, 33 U.S.C. 1313[d], section 303[d]) for fecal coliform related to urban stormwater runoff (including combined sewer overflow [CSO] contributions; NYSDEC, 2020). Salt marsh and parklands surround Alley Creek, with freshwater inputs from the shallow groundwater via natural springs, upgradient ponds connected by a network of streams, and deeper groundwater that discharges to surface waters via at least one artesian well. The Alley Creek and Little Neck Bay watershed is mostly sewered and is served by Tallman Island Wastewater Resource Reclamation Facility (WRRF) and Jamaica WRRF (both operated by New York City Department of Environmental Protection [NYCDEP]) in Queens County, and Belgrave Sewage Treatment Plant (operated by Belgrave Water Pollution Control District) in Nassau County. Tidal exchange through Alley Creek and Little Neck Bay can act as a source of fecal contamination to New York Harbor and to western Long Island Sound via the East River; therefore, a hydrodynamic model of the East River was developed in 2008 to help estimate fecal coliform concentrations associated with Alley Creek and surrounding waters (AECOM USA, 2014).
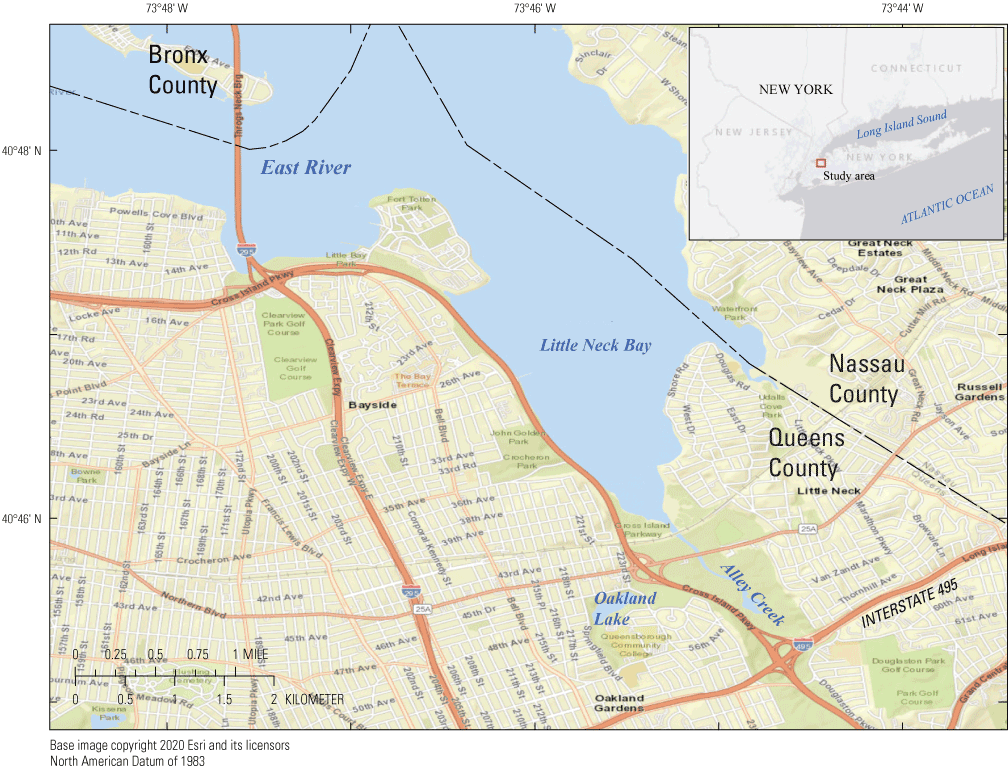
Alley Creek in Queens, New York, along with the East River, Little Neck Bay, and Oakland Lake.
In response to aging sewage infrastructure throughout New York City (NYC) that has resulted in leaks from and infiltration into sewer systems and waterways, NYC resource managers have developed and begun implementing management strategies to reduce sources of fecal contamination. For the Alley Creek watershed, the NYCDEP developed a long-term control plan (LTCP; AECOM USA, 2014) in coordination with the NYSDEC to identify appropriate CSO controls, and the New York City Department of Parks and Recreation developed a watershed management plan (Matic and others, 2015) to guide projects aimed at improving ecosystem health. Details on the Alley Creek watershed can be found in both the Alley Creek LTCP (AECOM USA, 2014) and the Alley Creek Watershed and Habitat Restoration Plan (Matic and others, 2015). In these plans, point sources (such as CSOs) of fecal contamination are identified, and nonpoint sources (such as waterfowl contributions and groundwater seepage with septic influence) are hypothesized to be contributing to Alley Creek and Little Neck Bay. To reduce the amount of sewage entering NYC waters, the NYCDEP has been working to repair, replace, and divert sewage by investigating sewer lines, upgrading treatment plants, separating combined sewer systems, and installing retention structures. However, events not related to infrastructure, such as the tidal cycle, weather, and boat traffic, can all induce sediment resuspension, which can result in higher concentrations of total suspended solids (TSS) and fecal indicator bacteria (FIB, including fecal coliform and enterococci). Bacteria associated with wastewater solids and bed-sediment resuspension have been documented in other NYC waterways (O’Mullan and others, 2019; Myers and Juhl, 2020). Although much progress has been made to reduce the number of dry- and wet-weather discharges to Alley Creek, FIB remains a persistent issue at several locations sampled by the NYCDEP (AECOM USA, 2014; NYCDEP, 2021).
Routine monitoring for FIB is conducted within the Alley Creek and Little Neck Bay watershed, and data are available for routine and source-specific monitoring, such as outfalls (AECOM USA, 2014; NYCDEP, 2021); however, host-specific analyses are required to better understand relative contributions of fecal contamination in stormwater, groundwater, and surface waters with respect to human (septic and sewage) and nonhuman contributions. In recent years, the NYCDEP has worked to mitigate some of the sources contributing to elevated FIB concentrations (for example, reducing CSO inputs with the construction of the 5,000,000-gallon [gal] Alley Creek CSO Retention Facility) and has conducted a single round of microbial source tracking (MST) assessments (AECOM USA, 2014); however, the previous MST assessment was inconclusive as to the extent to which the relative importance of host sources (human or wildlife) was affecting Alley Creek and Little Neck Bay.
To better understand host sources of bacteria in Alley Creek, the U.S. Geological Survey (USGS), in cooperation with the NYCDEP, monitored three outfall locations along Alley Creek, four sites in Little Neck Bay, and natural waters in the Alley Creek watershed that are influenced by fecal contamination from various sources. Between August 2020 and June 2021, water and bed-sediment samples were collected from Alley Creek, Oakland Lake, and a nearby artesian groundwater well and analyzed for FIB, bacterial and viral MST markers (Bacteroides, Helicobacter, and crAssphage), TSS, and pharmaceutical compounds. Samples from Little Neck Bay were also collected in May 2021 for crAssphage methods development.
Microbial source tracking can be used to classify the contributions of fecal contamination, particularly from point sources, because host-specific genetic markers have been identified in groups of bacteria found in the gut of most warm-blooded animals, such as humans, canines, and waterfowl (Kildare and others, 2007; Green and others, 2012; Green and others, 2014; Stachler and others, 2018). These MST techniques help reveal relative magnitude of fecal contributions relative to conditions and locations for a given marker, thus providing resource managers the information necessary to make decisions regarding extent and methods of remediation and practicality of reducing bacterial loads at a given site. One limitation of MST markers is that they are based on the genetic material of the bacteria or virus, which need not be viable in the sample. Thus, disinfected effluent from sewage treatment facilities has been shown to contain high concentrations of both human and canine markers (Tagliaferri and others, 2021b), with the presence of canine markers in sewage acting as a source in addition to canine waste within the watershed. Therefore, to provide another layer of evidence for human (sewage) contamination, a subset of samples was analyzed for a suite of 106 pharmaceutical compounds (constituents that are specific to human sources and would be expected to come from sewage only) and compared to MST results in surface water and groundwaters (table 1.1). Factors that could affect concentrations, including tidal conditions, seasonality, and weather conditions, also were assessed. Together, the MST, pharmaceutical, and FIB data generated by this study, along with supplementary data such as locations of point sources, locations of wildlife populations, and tidal exchange data, may provide reliable information on source identification and transport mechanisms of fecal contamination.
Methods
Sample collection occurred between August 2020 and June 2021. Monitoring sites were selected in consultation with the NYCDEP and NYSDEC, and four sites were selected as routine water-quality monitoring sites for this study: NYCDEP outfall sites TI–024, TI–008, and TI–25, and Oakland Lake (table 1; fig. 2). The routine sites were sampled during dry weather on four occasions, distributed seasonally throughout the year. Each of the sampling events consisted of two samples, collected during low- and high-tide conditions of Little Neck Bay, spaced about a week apart. Tide influenced two (TI–008 and TI–025) of the four routine water-quality monitoring sites daily (table 2). In addition, samples were collected at two of the routine sites, TI–024 and TI–025, twice during wet-weather conditions (table 2). Dry weather was defined as no rainfall greater than 0.1 inch (in.) in the outfall catchment area within a 48-hour period prior to sampling, per the NYCDEP Compliance Monitoring Unit protocol. This report also follows the NYCDEP protocol definition of wet weather as rainfall predicted, at least a day in advance and with 80-percent probability, to result in 0.2 in. or more rain. Groundwater from an artesian well Q277 near TI–024 that contributes freshwater to Alley Creek was also sampled. Two sets of samples (during low and high tides) were collected from Little Neck Bay to support method development of the viral crAssphage MST markers and provide open-water estuarine receptor samples to compare with source samples collected along Alley Creek (as was conducted in nearby Hempstead Harbor; Tagliaferri and others, 2021b). Bed sediment and sediment along a connecting combined sewer line were collected to assess resuspension contributions to fecal contamination. Laboratory analyses were conducted at NYCDEP and USGS laboratories (listed in the “Laboratory Analyses” section). Method-specific quality controls were run, and internal data reviews ensured high confidence in the data.
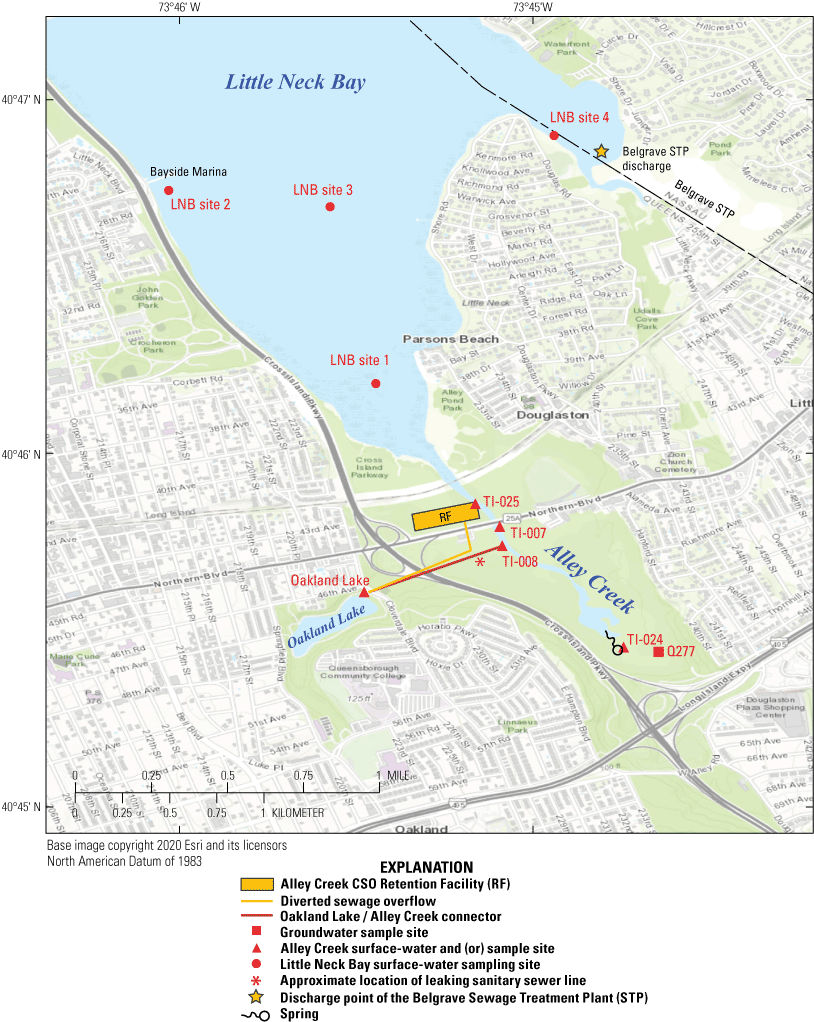
Study area with locations of the New York City Department of Environmental Protection surface-water and (or) bed-sediment sampling sites. The orange line represents diverted sewage lines designed to limit overflow from the combined sewer overflow (CSO) system to TI–008. The black/red line represents the pipe connecting Oakland Lake to TI–008. The asterisk (*) denotes the approximate location where sewage from an adjacent sanitary sewer line was leaking into a combined sewer line; the leak was repaired between April 27 and May 11, 2021. TI–007 is an emergency discharge outfall for a sewage pump station and does not flow, even during wet-weather conditions, and the sediment sample was collected on the opposite shoreline. For location, see inset map in figure 1.
Table 1.
Sites within the Alley Creek watershed at which water and bed-sediment samples were collected, Queens County, New York.[Number of samples collected represents the total from August 2020 to June 2021. Coordinates are referenced to the North American Datum of 1983. USGS Station ID, U.S. Geological Survey station identification number; NYCDEP, New York City Department of Environmental Protection; NY, New York; QW, water-quality; cm, centimeter; —, no data]
Table 2.
Schedule and conditions for samples collected within the Alley Creek watershed and Little Neck Bay, Queens County, New York.Site Selection
Information about sample sites is provided in table 1. Sites were selected, in consultation with the NYCDEP and NYSDEC, to include water and sediment sampling points adjacent to outfalls of specific concern related to the Tallman Island WRRF drainage area, along with freshwater sources tributary to Alley Creek. The outfalls are designated by the prefix “TI” (for Tallman Island) followed by an identifying number and are shown on figure 2. Outfall TI–025 (fig. 3A) is associated with the Alley Creek CSO Retention Facility, which became operational in 2011 and is designed to hold 5,000,000 gal of CSO that is then pumped to the Tallman Island WRRF for treatment (AECOM USA, 2014).
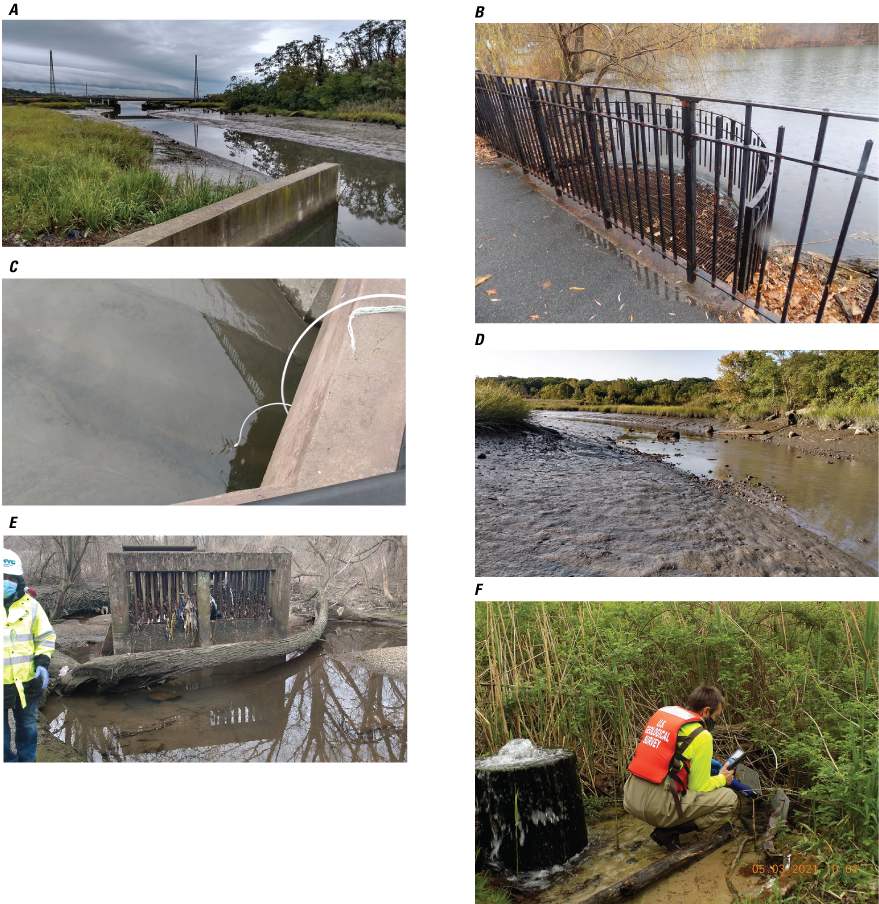
Sampling sites along Alley Creek, Queens County, New York: A, Sampling site TI–025 (looking north) with Alley Creek at low tide, from the concrete wall above the Alley Creek Combined Sewer Overflow Retention Facility discharge gates (September 30, 2020). B, The east side of Oakland Lake at a weir structure (December 14, 2020). C, Site TI–008 at end of the boardwalk overlooking Alley Creek with pump tubing deployed (March 16, 2021). D, Site TI–007, from the east bank of Alley Creek, looking south (September 22, 2020). E, Aboveground concrete combined sewer overflow structure (TI–024) in Alley Creek (March 16, 2021). F, Artesian groundwater well Q277 flowing from a cement pump base from former New York City water supply infrastructure (May 3, 2021). Photographs by Shawn C. Fisher, U.S. Geological Survey.
Oakland Lake (fig. 3B) flow to Alley Creek is approximately 2,500,000 gallons per day (gal/d) via outfall TI–008 (AECOM USA; 2014; fig. 3C). Oakland Lake is connected to the sewer system through a weir chamber to provide water-level control; the weir chamber is connected to a storm line, which is connected to a CSO line that then discharges from outfall TI–008 to Alley Creek (fig. 3C). With the construction of the Alley Creek CSO Retention Facility, CSO events are now rare at outfall TI–008 (AECOM USA; 2014). However, TI–008 had been receiving sewage from a parallel sanitary sewer leaking through a compromised joint (fig. 2) at approximately 400 feet (ft) upgradient of the outfall until it was repaired between April 27 and May 11, 2021. Both Oakland Lake and TI–008 were sampled as part of this study. About 500 ft downstream of TI–008 is TI–007, an emergency discharge outfall for a sewage pump station; TI–007 typically does not flow, even during wet-weather conditions, and the sediment sample for this study was collected on the opposite shoreline (fig. 3D).
Outfall TI–024 is an above-ground outfall that had been flowing continuously in recent years during both dry and wet weather (fig. 3E); but as of 2019, flow discharging from the outfall structure during dry weather had ceased. As of 2021, there is a hole in the top of the 7-ft-diameter sewer pipe that is exposed in the streambed immediately adjacent to the outfall from which water is continuously upwelling. Additional sampling was conducted at the hole location and is discussed later in this report.
Groundwater well Q277 (fig. 3F) is an artesian well with a total depth of 144 ft with flow that enters Alley Creek near TI–024 (USGS, 2021b). The well was originally part of a network of groundwater wells developed for public supply in Queens County and operated until the 1980s when the pumps were decommissioned and the structures removed. The flow reflects groundwater hydraulic pressure of the Magothy aquifer and discharges continuously with flow reaching Alley Creek near TI–024. Data from previous water-quality sampling conducted by the USGS in the 1990s and 2000s (USGS, 2021b) were reviewed for this study to determine the extent of urban land-use influence on the flowing groundwater.
Four sites in Little Neck Bay were selected to correspond with sampling locations used by the NYCDEP for either their Harbor Survey or the Sentinel Monitoring Program (SMP; table 1). LNB site 1 is at the mouth of Alley Creek and is near SMP station S1, and LNB site 2 is near SMP station S64 along the western shore of Little Neck Bay near Bayside Marina. LNB site 3 is near the Harbor Survey site LN1 in the middle of Little Neck Bay, and LNB site 4 is near SMP station S2 north of the mouth of a small creek and the discharge point for the Belgrave Sewage Treatment Plant (table 1; fig. 2).
Sample Collection and Processing
Procedures for collecting, processing, and cleaning samples for surface water, groundwater, and sediment were done according to the USGS National Field Manual for the Collection of Water-Quality Data (NFM; USGS, variously dated). Surface-water samples were either point or grab (dip), depending on accessibility (table 1). Field measurements of physicochemical constituents (water temperature, specific conductance, dissolved oxygen, pH, and turbidity) were collected at each site by using a YSI EXO multiparameter sonde (YSI, Inc.; Yellow Springs, Ohio). Water samples (point) at TI–025 and TI–008 were collected from an elevated perch directly above the outfall by using a peristaltic pump with sterilized Cole-Palmer Masterflex (Cole-Parmer: Vernon Hills, Illinois) pump head tubing connected to polytetrafluoroethylene (PTFE) intake tubing and were discharged into an 8-liter (L) PTFE churn splitter. Grab samples were collected at TI–024 and Oakland Lake by directly filling sample bottles roughly 0.5 ft below the water surface (TI–024) or from the flow before reaching the weir chamber (Oakland Lake). Cleaning of sampling equipment followed procedures outlined in the NFM (Wilde, 2004; Myers and others, 2007), except for sanitizing the PTFE churn, which was done in a bleach bath followed by thorough rinsing with sodium thiosulfate and deionized water. Field blank samples were collected to ensure cleaning and sterilizing procedures were adequate. Sample collection order of unfiltered water collected by spigot from the churn splitter was as follows: two high-density polyethylene 1-L bottles for MST, one 1-L glass wide-mouth jar for TSS, and two high-density polyethylene 500-milliliter (mL) bottles—one each for enterococci and fecal coliform. A 20-mL aliquot of sample water, for analysis of pharmaceutical compounds, retrieved directly from the churn splitter (TI–025 and TI–008) or from flow (TI–024 and Oakland Lake) via syringe, was passed through a 0.7-micrometer (µm) syringe filter into a 20-mL amber glass vial, placed on ice in the field, and frozen to a temperature of −20 degrees Celsius upon return to the office. Selected frozen dry-weather samples were later submitted for analysis of pharmaceutical compounds three times throughout the study. Criteria for submitting a sample for the pharmaceuticals analysis included detection of the human and (or) canine MST markers; all wet-weather and groundwater samples were submitted for analysis of pharmaceutical compounds; in all, 23 samples were submitted.
Bed-sediment samples were collected from the shoreline at three (TI–024, TI–008, and TI–025) of the four routine water-quality sampling sites plus an additional site (TI–007, table 1) during low tide from exposed shoreline by using a disposable sterile spatula to a depth of 2 centimeters (cm) from the surface in each area. Sediment was collected in 500-mL baked (at 450 degrees Celsius) amber glass jars filled with sediment to three-quarters full. Care was taken to only collect from points that did not appear disturbed by human or animal traffic. Sediment was partitioned for fecal coliform, enterococci, and MST-marker analyses.
In May 2021, samples in Little Neck Bay were collected during low (May 3) and high (May 10) tides by boat. Samples collected on May 10 also reflect wet-weather conditions because greater than (>) 0.1 in. of precipitation was recorded on May 9 (Weather Underground, 2021a, b, c). Grab samples were collected at each site by filling sample bottles directly at a depth of 0.5 ft below the water surface. Measurements of water temperature, specific conductance, and turbidity using a multiparameter sonde were also taken. Water samples were collected for fecal coliform, enterococci, TSS, and MST-marker analyses.
Thermal images were collected at TI–024 and the surrounding area by using an FLIR E8 handheld infrared camera (Teledyne FLIR Systems, Inc.; Wilsonville, Oregon) to determine if a difference in water temperature between areas of upwelling and surrounding surface waters was noticeable. Images were taken on May 3 and 10, 2021, corresponding to the depth-profile sample-collection events in the sewer-pipe hole at the streambed adjacent to the TI–024 outfall. Multiple temperature and specific conductance measurements were made as the multiparameter sonde was lowered incrementally into the hole to a maximum depth of 7.5 ft. Samples for TSS, fecal coliform, and enterococci were collected by using a peristaltic pump and sterile tubing at select depths corresponding to multiparameter measurements. Thermal images and temperature data, along with depth-profile data, are available in the USGS data release that accompanies this report (Fisher and others, 2021).
Laboratory Analyses
Samples collected throughout the study were analyzed at the USGS Ohio Water Microbiology Laboratory (OWML), the USGS National Water Quality Laboratory (NWQL), the NYCDEP Wards Island Process Control Laboratory (WIPCL), and the NYCDEP Newtown Creek Microbiology Laboratory (NCMBL). NYCDEP laboratories are accredited by the New York State Environmental Laboratory Accreditation Program (ELAP; https://www.wadsworth.org/regulatory/elap, Wadsworth, N.Y.) for TSS, fecal coliform, and enterococci in nonpotable water. The OWML analyzed water samples for MST markers and sediment samples for both MST markers and FIB, and the NWQL analyzed samples for pharmaceutical compounds.
Physicochemical Constituents
Samples for TSS and pharmaceutical compounds analysis were collected to supplement the MST and FIB data. Samples for TSS were collected in a 1-L clear glass jar, transported on ice to the WIPCL, and analyzed following method SM 2540 D–2011 (Standard Methods Committee, 2011). Data were reviewed and approved by a NYCDEP laboratory manager and the USGS. Results of TSS analysis are reported in milligrams per liter (mg/L).
Analysis of 106 pharmaceutical compounds (along with 41 internal surrogates for quality control, two pesticide compounds, and one industrial deicing compound; appendix 1) at the NWQL was conducted using direct-aqueous injection liquid chromatography-mass spectroscopy (NWQL laboratory schedule 2440) according to Furlong and others (2014; the full list of compounds included in the method, including reporting limits, is provided in table 1.1). Results for the compounds processed according to the method described herein are presented in nanograms per liter (ng/L) and available through the USGS National Water Information System (NWIS; USGS, 2021b). Because the samples submitted for analysis had been previously frozen at −20 °C (following guidance from the NWQL), most results in NWIS have a qualifier indicating a sample hold-time violation; however, these data were used for this study as a measure of relative sewage influence and not for precise measure of quantification. Only detections of pharmaceutical compounds were used as an indication of human sources in this study because pesticides and deicing agents have sources other than sewage (such as runoff or direct application).
Microbiology
Analyses for fecal coliform and enterococci at the NCMBL followed Standard Method 9222D–2006 (Standard Methods Committee, 2006) and the U.S. Environmental Protection Agency (EPA) Method 1600 (EPA, 2014), respectively. Samples were processed within 8 hours of collection and analyzed after a 24-hour incubation period. Data were reviewed and approved by a NYCDEP laboratory manager and the USGS. Results of these methods are presented in colony-forming units per 100 milliliters (CFU/100 mL).
Samples were analyzed for Bacteroides and Helicobacter MST markers at the OWML by protocols previously described in Fisher and others (2020) and are summarized here. Matrix-dependent volumes of each sample were filtered through a 0.4-μm polycarbonate filter (Whatman Inc.; Florham Park, New Jersey), and the filters were aseptically folded and placed into a 2-mL screw cap vial containing 0.3 grams (g) of sterile glass beads (Sigma-Aldrich Corp.; St. Louis, Missouri). Samples were frozen at −70 °C following filtration. Sample filters underwent deoxyribonucleic acid (DNA) extraction by use of the DNA–EZ extraction kit (GeneRite, LLC.; Monmouth Junction, N.J.), and extracts were subsequently stored at 4 °C until analysis by quantitative polymerase chain reaction (qPCR) within 7 days of extraction. Analysis by qPCR was done using either a StepOnePlus or a QuantStudio 3 Real-Time PCR System (Thermo Fisher Scientific; Waltham, Massachusetts) based on conditions for the following MST markers, as described in the publications cited:
-
• human-associated Bacteroides marker (HF183/BacR287) (Green and others, 2014)
-
• canine-associated Bacteroides marker (BacCan) (Kildare and others, 2007)
-
• waterfowl-associated Helicobacter marker (GFD) (Green and others, 2012)
-
• general marker of Bacteroides spp. (GenBac) (Siefring and others, 2008)
Samples were also analyzed at the OWML for crAssphage, an abundant type of Bacteroides-infecting phage of a human-specific virus that has shown promise for use in MST studies (Stachler and others, 2017). Because crAssphage markers originate from viruses, separate processing methods were required to prepare samples for analysis. One-liter water samples were pH-adjusted to 3.5±0.2 by using 1 molar molecular grade hydrochloric acid to support viral attachment during filtration. Sample water was then filtered in 250-mL aliquots through 0.45-µm mixed cellulose HAWG filters (MilliporeSigma; Billerica, Mass.). Remaining processing steps were conducted as described for Bacteroides and Helicobacter, and qPCR was done based on conditions as described in the following original reporting:
-
• human-associated crAssphage marker (CPQ_056) (Stachler and others, 2018)
-
• human-associated crAssphage marker (CPQ_064) (Stachler and others, 2018)
Results from the MST marker analyses are presented in copies of genes per 100 milliliters (copies/100 mL) and can be used to compare relative concentrations of each marker to gage the relative contributions of a specific host across the area and through time. Reporting limits for the MST markers varied based on differences in analytical characteristics for each marker and on factors related to water quality. Reporting limits were established by the OWML for each assay and are defined as the lowest concentration that is detected with 95-percent confidence. Data from the OWML were internally quality assured according to standard operating procedures (Francy and others, 2017; USGS, 2021a).
Sediment Resuspension Assessment
A laboratory experiment was designed to replicate tidal activity in Alley Creek using sediment collected in the sewers and on the shoreline, as well as water collected from Oakland Lake. These sediment samples were assessed to understand the relation between sediment resuspension and FIB in the water column. A set of samples was collected at low tide along the combined sewer pipe connecting Oakland Lake to TI–008 (fig. 2) by using a disposable sterile spatula at points upgradient and downgradient of the observed sewage leak. Sediment deposited along the walls of the combined sewer pipe was above water during low tide but had been deposited as result of tidal exchange moving sediment from Alley Creek via the TI–008 outfall. Two water samples (1 L each) from Oakland Lake, one upgradient and one downgradient of the observed leak in the sewer pipe, were also collected. The combined sewer sediment samples were collected and processed according to methods described in chapter A7 of the NFM (Myers and others, 2007). Samples were shipped on ice overnight to the OWML.
For the sediment resuspension experiment, varying amounts of sediment were added to 250-mL Erlenmeyer flasks containing either 150 mL of sterile phosphate buffer or Oakland Lake water. Flasks prepared were as follows: (1) four buffer flasks with 1.8, 5.0, 10.2, or 30.3 g of combined sewer sediment added, (2) one Oakland Lake flask containing 10.3 grams of combined sewer sediment, (3) one negative control flask containing only 150 mL of buffer, and (4) one matrix control flask containing only 150 mL of Oakland Lake water (appendix 2; table 2.1). All seven flasks were secured onto a Burrell Model 95 wrist-action shaker (Burrell Scientific, Pittsburgh, Pennsylvania) and allowed to rest for 60 minutes. Before shaking started, a 12-mL sample from each flask was pipetted from each of the seven flasks to represent a time step of 0 minutes. The set was shaken at approximately 50 oscillations per minute, and samples were collected from each flask that was amended with sediment after 5, 30, and 240 minutes of shaking. For the negative control and matrix control, samples were collected at 240 minutes. All samples were refrigerated immediately after collection. Within 1 hour of collection, samples were analyzed by EPA Method 1600 (EPA, 2009) and Standard Method 9222D (Standard Methods Committee, 2017) to determine enterococci and fecal coliform concentrations, respectively. Methods and results from the sediment resuspension assessment can also be found in Fisher and others (2021).
Quality Assurance
Quality-control samples were collected to ensure sampling procedures were consistent and did not result in outside contamination of the environmental samples. A review of the quality-control data confirmed that neither sampling procedures nor laboratory data qualifiers resulted in environmental data being disqualified from interpretation. Field blanks were collected by pumping deionized water (August 24, 2020) or sterile phosphate buffer solution (December 9, 2020, and June 8, 2021) through peristaltic pump tubing and into the PTFE churn splitter; thus, the field blanks represent TI–025 and TI–008 sample procedures. Replicate sample sets were collected sequentially, where replicate sets from sites TI–025 and TI–008 were sequential fills of the PTFE churn splitter (following deionized water and native water rinses) and replicate sets from sites TI–024 and Little Neck Bay (LNB site 1; table 1) were sequential grab samples. Variability among paired replicates with at least one detected value above the reporting limit was compared by using relative percent difference for physicochemical constituents and absolute value log-10 difference (AVLD) for microbiological constituents (Francy and others, 2011; Tagliaferri and others, 2021a). The number of quality-control samples varied based on analysis because samples for FIB and TSS were collected more regularly.
Three blank and three replicate samples were collected for FIB. Concentrations of fecal coliform in two of the three blank samples were above the reporting limit of 2 CFU/100 mL: 14 CFU/100 mL on August 24, 2020, and 4 CFU/100 mL on June 8, 2021. These values were at least 4 times lower than the lowest environmental sample collected; therefore, environmental results were not qualified. No detections of enterococci were found in the blanks. The AVLD of replicates coliform ranged from 0.01 to 0.14 for fecal coliform and from 0.03 to 0.26 for enterococci.
Two blank and five replicate samples were collected for TSS. Concentrations of TSS in both blank samples were below the reporting limit (less than [<] 2.5 mg/L). The relative difference among the four of five replicates with both values above the reporting limit ranged from 0.21 to 0.61 percent, with the highest values reflecting replicate values of 8.0 and 15 mg/L.
Two blank and four replicate samples were collected for the MST markers. Concentrations for all six MST markers were below reporting limits in both blank samples. The AVLD for the four replicates varied by marker: 0.00–0.08 (GenBac), 0.00–0.05 (HF183/BacR287), 0.10–0.19 (BacCan), 0.04–0.12 (CPQ_056), and 0.04–0.11 (CPQ_064); concentrations of GFD were below the reporting limit for all paired samples. Of the duplicate qPCR results run for each sample for internal laboratory quantification verification, 10 of the values did not match and were qualified; however, these data were not excluded from interpretation.
Pharmaceutical method results were evaluated for a range of concentrations and based on isotope dilution standard recovery, method reporting limits, and result qualifiers according to Furlong and others (2014). Isotope recovery for one compound (acetaminophen) in one sample was outside acceptable range (422 percent); however, the total pharmaceutical concentration for the corresponding sample is such that increasing the environmental detection of the acetaminophen concentration would result in less than a 4-percent difference in total pharmaceutical concentration. Relative percent differences for total pharmaceutical compound concentrations for replicate samples collected at TI–008 (313 and 563 ng/L; August 24, 2020) and TI–024 (1,211 and 1,189 ng/L; June 17, 2021) were 57 and 1.8 percent, respectively (table 3). The greater percent difference at TI–008 reflects the variability in the dynamic tidal system; substituting both replicate values for the environmental concentrations did not change the statistical significance when comparing HF183/BacR287 to total pharmaceutical concentrations. Therefore, no adjustments were made to the dataset used for this assessment.
Table 3.
Summary of pharmaceutical results by site for samples collected within the Alley Creek watershed and Little Neck Bay, Queens County, New York.[Full set of results available on the U.S. Geological Survey (USGS) National Water Information System website at https://waterdata.usgs.gov/nwis (USGS, 2021b) by entering the USGS station identifiers in table 1. See table 1 for site locations and table 2 for schedule and conditions for samples collected. ng/L, nanogram per liter; HF183/BacR287, human-associated Bacteroides marker; mL, milliliter; NA, not applicable; <, less than]
Data Availability and Analysis
Microbiological and physicochemical data from the field and laboratories are publicly available. Data from the routine water samples, bed-sediment samples, and Little Neck Bay samples can be accessed on the USGS National Water Information System website (USGS, 2021b) by entering the USGS station identifier (USGS station ID) listed in table 1 and selecting “Site Information” and then “Site Number” on the web page. Data from the sediment resuspension experiment and the TI–024 intensive sampling are available in the USGS data release that accompanies this report (Fisher and others, 2021).
Precipitation data from Weather Underground (stations KNYNEWYO1105, KNYNEWYO1408, and KNYNEWYO234; Weather Underground, 2021a, b, c) were used to determine whether rainfall occurred within 48 hours prior to sampling. While quality assurance on the data provided by Weather Underground stations could not be evaluated, precipitation data from several events within the study period were compared and were found to be in agreement on the basis of whether the total precipitation exceeded the criterion for wet-weather conditions of 0.1 in. 24 hours prior to the time of sample collection. Wet-weather samples were collected on September 30, 2020, May 10, 2021, and June 14, 2021; all other samples were collected during dry weather. For May 10, 2021, and June 14, 2021, rainfall totals exceeded 0.1 in. by the time of sample collection (Weather Underground, 2021a, b, c).
Additional analyses included summarizing pharmaceutical compound data and performing statistical analyses on FIB and MST marker data. Results from the pharmaceutical method are presented as number of detections and total concentration of pharmaceutical compounds for relative comparison to MST markers and FIB. A nonparametric analysis using Spearman Rank correlation (Helsel and others, 2020) was conducted using SigmaPlot 14 (Inpixon, Palo Alto, California) to determine correlations (ρ) between fecal coliform, enterococci, MST markers, and TSS across sites and with respect to the tidal cycle, and to compare HF183/BacR287 and total pharmaceuticals concentrations.
Results
Data presented in the following sections allowed for a better understanding of the sources of fecal contamination to Alley Creek. Monitoring during the study period at the three NYCDEP outfalls along Alley Creek and at Oakland Lake, Little Neck Bay, and the artesian groundwater well within the watershed provided baseline data for current and any future assessments. Data from the bed-sediment sampling and resuspension experiment helped explain some instances of elevated bacteria and relative contributions to Alley Creek. The intensive assessment at TI–024 resulted in data that helped define the extent of potential bacterial contamination and the influence of groundwater hydraulics on the aging sewer infrastructure.
Routine Water-Quality Monitoring
Results from samples collected along Alley Creek are presented by site and in the context of tidal stage and sampling conditions (dry versus wet weather) and differences in known and potential sources contributing to each outfall location. Fecal indicator bacteria concentrations were not consistently detected during wet-weather conditions or low tide and ranged from <2 to 10,700 CFU/100 mL for fecal coliform (fig. 4A) and from <2 to 13,100 CFU/100 mL for enterococci (fig. 4B). Concentrations of TSS were indicative of water clarity and ranged from <2.5 to 616 mg/L (fig. 4C) for this study. The general Bacteroides marker, GenBac, was detected in all surface-water samples and was strongly correlated with the human Bacteroides marker HF183/BacR287 (ρ=0.965, P<0.001, where P is significance value), across the entire dataset (fig. 5A). Human Bacteroides marker HF183/BacR287 was prevalent (27 of 28 samples) at the three outfall sites sampled along Alley Creek but was not found in any of the eight Oakland Lake or two Q277 samples. Both CrAssphage markers—CPQ_056 and CPQ_064—were detected in 38 of the 39 water samples (including Little Neck Bay) with HF183/BacR287 concentrations above the reporting limit, and good correlation of indicator performance between the methods was observed (ρ=0.875 and 0.855, respectively; P<0.001), which has also been shown in a different study (Stachler and others, 2018). Canine marker BacCan was also commonly detected, although less prevalent (20 of 28 samples) in samples from the three outfall sites and occasionally in Oakland Lake, and showed good correlation with HF183/BacR287 (ρ=0.821, P<0.001). Waterfowl marker GFD was detected in 8 of 28 water samples collected at the three outfall sites and Oakland Lake.
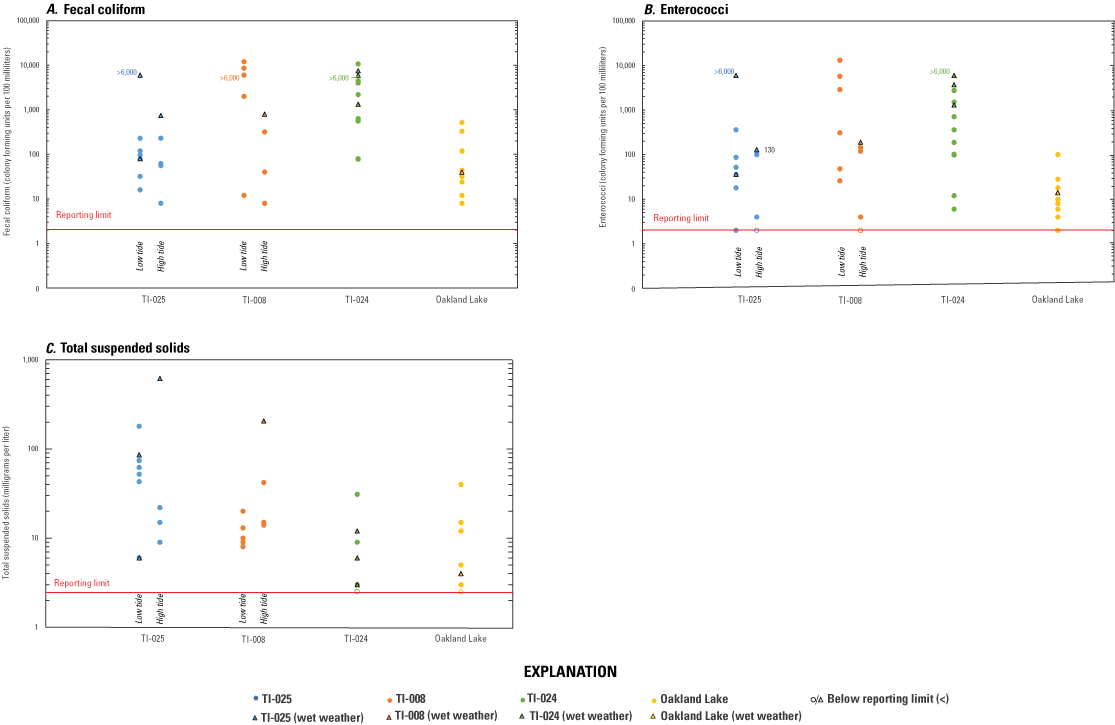
The results from water-quality and fecal indicator bacteria samples collected between August 24, 2020, and June 17, 2021, at New York City Department of Environmental Protection outfalls TI–025, TI–008, and TI–024, and at Oakland Lake, Queens County, New York. Concentrations of the following are presented with respect to tidal stage (low and high tide) and weather condition (dry and wet weather): A, fecal coliform in colony-forming units per 100 mL (CFU/100 mL); B, enterococci in CFU/100 mL; and C, total suspended solids in milligrams per liter. See table 1 for site locations and table 2 for schedule and conditions for samples collected.
At TI–025, concentrations of MST markers, concentrations of FIB, and both total number of detections and concentrations of pharmaceutical compounds were generally higher in wet-weather samples than in dry-weather samples. Concentrations of HF183/BacR287 in dry-weather samples ranged from 540 to 17,000 copies/100 mL (n=8; median 5,800 copies/100 mL) and were measured at 3,400,000 and 31,000,000 copies/100 mL for the wet-weather samples collected during low tide (fig. 5B), suggesting influence from sewage sources. The BacCan marker was detected in four of eight dry-weather samples at TI–025 (December 2020 and June 2021 samples only) and with roughly two orders of magnitude lower concentrations relative to the wet-weather samples of 130,000 and 870,000 copies/100 mL (fig. 5C). Further, BacCan concentrations were greater in samples collected at low tide relative to at high tide. The waterfowl-associated GFD marker was detected in the dry-weather samples from August (3,700 and 2,500 copies/100 mL) and December (1,500 and 3,800 copies/100 mL) and not in either wet-weather sample at TI–025. Concentrations of TSS ranged from 6 mg/L to 616 mg/L; the greatest concentration of TSS corresponded to a high concentration of fecal coliform (745 CFU/100 mL) and was detected during wet weather (figs. 4A, C). Fecal coliform concentrations ranged from 8 to 230 CFU/100 mL in dry-weather samples and from 80 to >6,000 CFU/100 mL in wet-weather samples (fig. 4A). Enterococci concentrations ranged from <2 to 360 CFU/100 mL in dry-weather samples and from 36 to >6,000 CFU/100 mL in wet-weather samples (fig. 4B). The number of pharmaceuticals detected in the two dry-weather, low-tide samples were 9 (August 24, 2020) and 3 (June 17, 2021), with total concentrations of 237 and 71.3 ng/L, respectively (table 3). One wet-weather sample (September 30, 2020; low tide), with 25 pharmaceutical compounds detected and a total concentration of 3,907 ng/L, was also collected. Interestingly, concentrations of pharmaceutical compounds on December 14, 2020 (designated as dry weather for this study) were 885 ng/L with 17 compounds detected, whereas concentrations and number of compounds detected for June 14, 2021 (designated as a wet-weather sample), were 56.8 ng/L and 2, respectively. The lower concentrations and number of detected compounds on June 14 is likely because the retention facility did not release during the earlier part of the storm during sample collection.
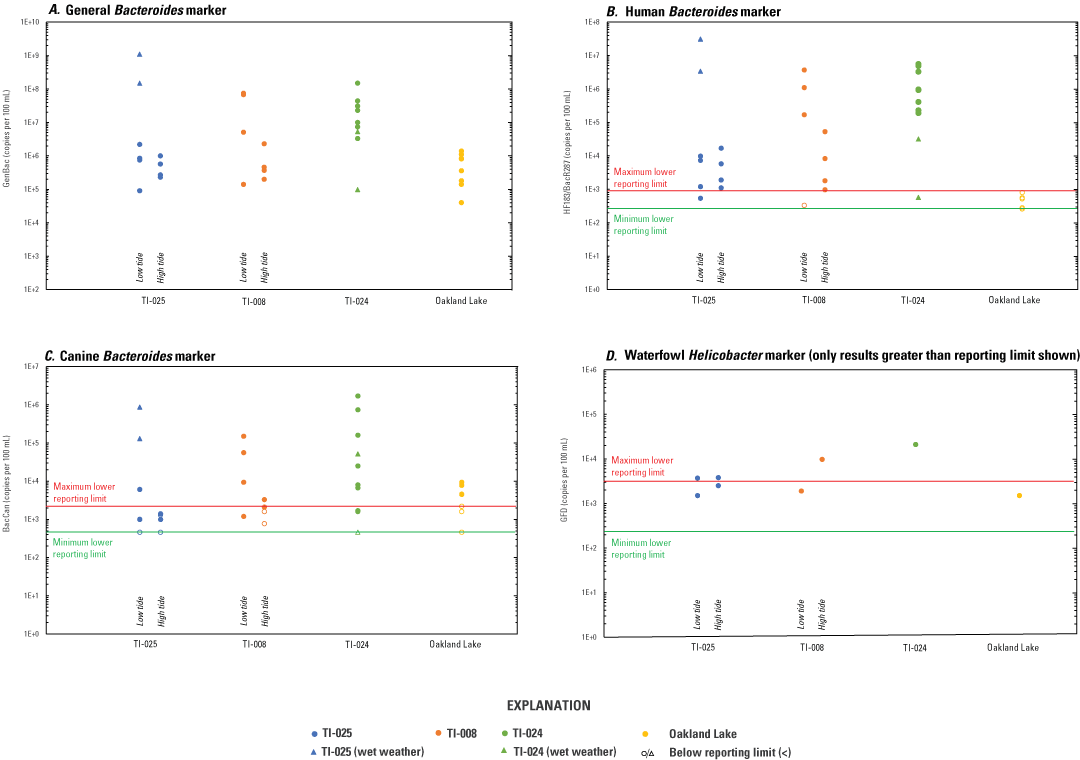
The results from microbial source tracking samples collected between August 24, 2020, and June 17, 2021, at New York City Department of Environmental Protection outfalls TI–025, TI–008, and TI–024, and at Oakland Lake, Queens County, New York. Concentrations of the following are presented with respect to tidal stage (low and high tide) and weather condition (dry and wet weather): A, General Bacteroides (GenBac) marker in copies per 100 milliliters (copies/100 mL); B, human Bacteroides (HF183/BacR287) markers in copies/100 mL; C, canine Bacteroides (BacCan) markers in copies/100 mL; and D, waterfowl Helicobacter (GFD) marker in copies/100 mL. In the y-axis scales, E means multiplied by 10 raised to the power of; 1E+2 is 1×102. For sample site location map, see figure 2.
At TI–008, concentrations of MST markers, concentrations of FIB, and both total number and concentrations of pharmaceutical compounds were generally higher in low-tide samples than in high-tide samples. Human marker HF183/BacR287 was detected in seven of the eight dry-weather samples (n=8, median 53,000 copies/100 mL; no wet-weather samples for MST markers were collected at this site). Of the six dry-weather samples collected between August 2020 and March 2021, concentrations of HF183/BacR287 were greater in low-tide samples (median 1,100,000 copies/100 mL) relative to high-tide samples (median 8,300 copies/100 mL), reflecting less dilution of the inputs from the leaking sanitary sewer parallel to the combined sewer between Oakland Lake and TI–008 (fig. 5B; fig. 2). Samples collected in June 2021 (HF183/BacR287 concentrations of 970 and <330 copies/100 mL) likely reflect repairs to the sanitary sewer conducted roughly 1 month prior to sample collection. Similar to what was observed at TI–025, the canine marker concentrations were also greater in samples collected at low tide than at high tide and likely reflect a combination of sewage and tidal dilution and would include pet waste inputs from Oakland Lake (fig. 5C). Only two of the eight samples had concentrations of the GFD marker above the reporting limit (1,900 and 9,700 copies/100 mL), both of which were collected in December and are possibly related to the fall migration of waterfowl (Matic and others, 2015). Concentrations of TSS ranged from 8 to 206 mg/L (fig. 4C). Fecal coliform and enterococci concentrations ranged from 8 to 12,000 CFU/100 mL (fig. 4A) and <2 to 13,100 CFU/100 mL (fig. 4B), respectively. On May 10, the highest observed concentrations of TSS (206 mg/L), enterococci (190 CFU/100 mL), and fecal coliform (800 CFU/100 mL) were from a sample collected during high tide following a rainfall event and possible CSO overflows the night before that resulted in sediment being resuspended throughout Alley Creek (fig. 4; samples for MST markers were not collected from Alley Creek outfalls on May 10). Four dry-weather samples—two during low tide (August 24, 2020, and June 17, 2021) and two during high tide (August 31 and December 14, 2020)—were analyzed for pharmaceutical compounds (table 3). The number of compounds detected in the three 2020 samples ranged from 8 to 16, whereas in June 2021, only 2 compounds were detected. Total concentrations of pharmaceutical compounds from 2020 samples ranged from 313 to 753 ng/L, whereas the concentration was 62.0 ng/L in the June sample (table 3), which is indicative of improved water quality emerging at TI–008; again, likely the result of stopping the sewage leak.
At TI–024, concentrations of MST markers and both total number and concentrations of pharmaceutical compounds were lower in wet-weather samples than in dry-weather samples, whereas concentrations of FIB were high. Concentrations of human MST markers were consistently high during dry-weather samples (HF183/BacR287 ranging from 190,000 to 4,900,000 copies/100 mL; median 950,000 copies/100 mL), which was similar to low-tide samples collected at TI–008 (fig. 5B). The two wet-weather samples appeared to be diluted with respect to human MST markers, with low marker concentrations for both September and June samples (570 and 32,000 copies/100 mL, respectively). Canine marker concentrations did not appear to be affected by precipitation, with the overall range from <460 to 1,700,000 copies/100 mL (median 16,500 copies/100 mL; fig. 5C). Of the 10 samples collected, the waterfowl marker GFD was only detected in one sample, from December 14, 2020, and was the highest concentration of the waterfowl marker detected in this study (21,000 copies/100 mL). Concentrations of TSS ranged from 2.5 to 31 mg/L and were not affected by wet weather for this study (fig. 4C). The ranges of fecal coliform and enterococci concentrations were greatest in the August 2020, December 2020, and June 2021 dry-weather samples, with maximum concentrations of 10,700 CFU/100 mL fecal coliform (August 24; fig. 4A) and 1,500 CFU/100 mL enterococci (August 31; fig. 4B). Concentrations of fecal coliform and enterococci in samples from March 2021 were both substantially lower, with concentrations of fecal coliform 7 times lower than the minimum concentration from the other three seasons. Results from the analysis of six dry-weather and two wet-weather samples for pharmaceutical compounds supports the finding based on high HF183/BacR287 marker concentrations that TI–024 is influenced by sewage (table 3). The number of compounds detected among the samples ranged from 6 to 12, and total concentrations ranged from 333 to 1,757 ng/L, inclusive of dry- and wet-weather samples.
At Oakland Lake, human MST markers were not detected in any of the eight samples (fig. 5B). The BacCan was detected in four of the samples, with concentrations ranging from 4,600 to 9,400 copies/100 mL (fig. 5C). Only one sample was positive for the waterfowl marker (1,500 copies/100 mL; December 9, 2020), and the detection coincided with one of the canine-marker detections and was possibly connected to the fall waterfowl migration (Matic and others, 2015). Fecal coliform and enterococci concentrations at Oakland Lake were typically low (especially relative to concentrations at TI–008), particularly for enterococci, which ranged from <2 to 100 CFU/100 mL (fig. 4A, B). Pharmaceuticals analysis from two Oakland Lake samples contained one (August 24, 2020) and two (December 9, 2020) compounds with total concentrations of 1.80 and 52.7 ng/L, respectively (table 3), which is consistent with little to no sewage influence.
Concentrations of all MST markers were below reporting limits in both groundwater samples collected at Q277, and very low concentrations of enterococci (6 CFU/100 mL) and fecal coliform (2 CFU/100 mL) were observed in one of the two sample sets collected. The total concentration of pharmaceutical compounds detected at Q277, from the two samples submitted, was the result of a single compound—carbamazapine—which was at a greater concentration than in any other sample for this study (USGS, 2021b); carbamazapine is regularly detected in groundwater influenced by urbanization and onsite wastewater disposal (Benotti and others, 2006; Bexfield and others, 2019).
Samples collected in Little Neck Bay represent open-water receptor sites as described in previous MST studies conducted in Nassau and Suffolk Counties (for example, Tagliaferri and others, 2021b). Human MST markers (HF183/BacR287, CPQ_056, and CPQ_064) were detected at all four sites (fig. 2) on May 3 and 10, 2021, and were at greatest concentration at LNB sites 1 (near the mouth of Alley Creek) and 4 (eastern part of the bay near Nassau County border; table 4). The BacCan marker was also detected in all samples, with the greatest concentrations again at LNB sites 1 and 4; at LNB site 1, concentrations were higher on May 10 (23,000 copies/100 mL), possibly because of precipitation the night before, than May 3 (4,200 copies/100 mL). The waterfowl marker was not detected in any of the Little Neck Bay samples, which was consistent with samples collected in Alley Creek and Oakland Lake in March and June 2021. Fecal coliform concentrations were greater on May 10 at three of the four sites, with the greatest concentration (600 CFU/100 mL) at LNB site 1. Enterococci concentrations were similar between dates, except at LNB site 4 on May 10, which was 73 CFU/100 mL, compared to 20 CFU/100 mL on May 3.
Table 4.
Summary of microbial source tracking data from Little Neck Bay, Queens County, New York.[See table 1 for site locations and table 2 for schedule and conditions for samples collected. MST, microbial source tracking; GenBac, general Bacteroides marker; mL, milliliter; HF183/BacR287, human-associated Bacteroides marker; BacCan, canine-associated Bacteroides marker; GFD, waterfowl-associated Helicobacter marker; LNB, Little Neck Bay]
Sediment and Resuspension
The four sediment samples, collected in September 2020 at the routine monitoring sites (table 2), provided a snapshot of FIB and MST marker concentrations that are bound in the bottom material and tidally influenced combined sewer lines of the Alley Creek watershed. Fecal coliform concentrations ranged from 1,400 to 15,000 colony-forming units per gram dry weight (CFU/g dw) (median 2,950 CFU/g dw), and enterococci concentrations ranged from 1,300 to 8,500 CFU/g dw (median 1,950 CFU/g dw; USGS, 2021b). The sediment collected at TI–025, TI–008, and TI–007 contained greater amounts of organic materials, representative of the tidal marsh and consistent with information reported in the Alley Creek LTCP (AECOM USA, 2014, p. 2–43), compared to the sandier sample collected at TI–024. The GenBac marker was present in all four samples with concentrations ranging from 290,000 to 7,000,000 copies/100 mL (median 1,700,000 copies of genes per gram dry weight [copies/g dw]). The HF183/BacR287 marker was not detected in the sediment sample at TI–007 and ranged from 4,000 to 34,000 copies/g dw in the other three outfall samples (median 14,900 copies/g gw). The BacCan marker was not detected in any of the samples, which was consistent with many other samples collected in Nassau and Suffolk Counties from a similar study (Tagliaferri and others, 2021b). The GFD marker was only detected in one sample (site TI–025; 49,000 copies/100 mL).
Sediment that had been deposited by tidal fluctuations was collected from the exposed area of the pipe above the water line inside the combined sewer connecting Oakland Lake to TI–008 and was used to demonstrate the potential for resuspension of sediment ladened with fecal contaminants from damaged infrastructure (the leaking sanitary sewer line) to contribute fecal bacteria to Alley Creek. Laboratory-produced sediment resuspension results are presented in table 2.1 and available in Fisher and others (2021). Enterococci and fecal coliform concentrations in the combined sewer sediment were 25,000 and 54,000 CFU/g dw, respectively. These concentrations were substantially greater than concentrations in shoreline sediment samples, including at TI–008 (8,500 CFU/g dw enterococci and 15,000 CFU/g dw fecal coliform). Prior to oscillation of the sample to simulate tidal disruption (time step=0 minutes), concentrations in the supernatant liquid of fecal coliform ranged from 60 to 170 CFU/100 mL and of enterococci ranged from 67 to 290 CFU/100 mL for the varying amounts of sediment added to the buffer and Oakland Lake water samples (table 2.1). Generally, concentrations of both FIB increased in the water matrix over time during oscillation, though concentrations of FIB did not increase as a function of the mass of sediment added to the slurry. After 240 minutes of oscillation, concentrations of fecal coliform ranged from 1,500 to 6,300 CFU/100 mL and of enterococci ranged from 540 to 2,800 CFU/100 mL for the varying amounts of sediment added to the buffer and Oakland Lake water samples (table 2.1).
Intensive Sampling at TI–024
The focused sampling at TI–024 revealed variability of FIB concentrations and specific conductance with depth within damaged infrastructure that is inundated by shallow groundwater and flowing continuously from the hole in the storm-sewer pipe and streambed adjacent to outfall TI–024 structure (fig. 6). Results from the samples collected on May 3 and 10, 2021, at TI–024 are available in Fisher and others (2021) and were useful for understanding the extent of wastewater that may become entrained in the groundwater upwelling. With the first depth profile, specific conductance values changed substantially with depth, ranging from 612 to 8,300 microsiemens per centimeter (μS/cm) at 25 °C from surface to bottom (fig. 6A); whereas specific conductance values collected during the second profile (conducted following a large precipitation event) were more uniform throughout, ranging from 304 to 438 μS/cm at 25 °C (fig. 6B), suggesting flushing of the stratified water in the pipe with a large amount of runoff. Similarly, fecal coliform and enterococci concentrations were greater at depth on May 3 (fig. 6A) but were elevated throughout the entire depth on May 10, 2021 (fig. 6B).
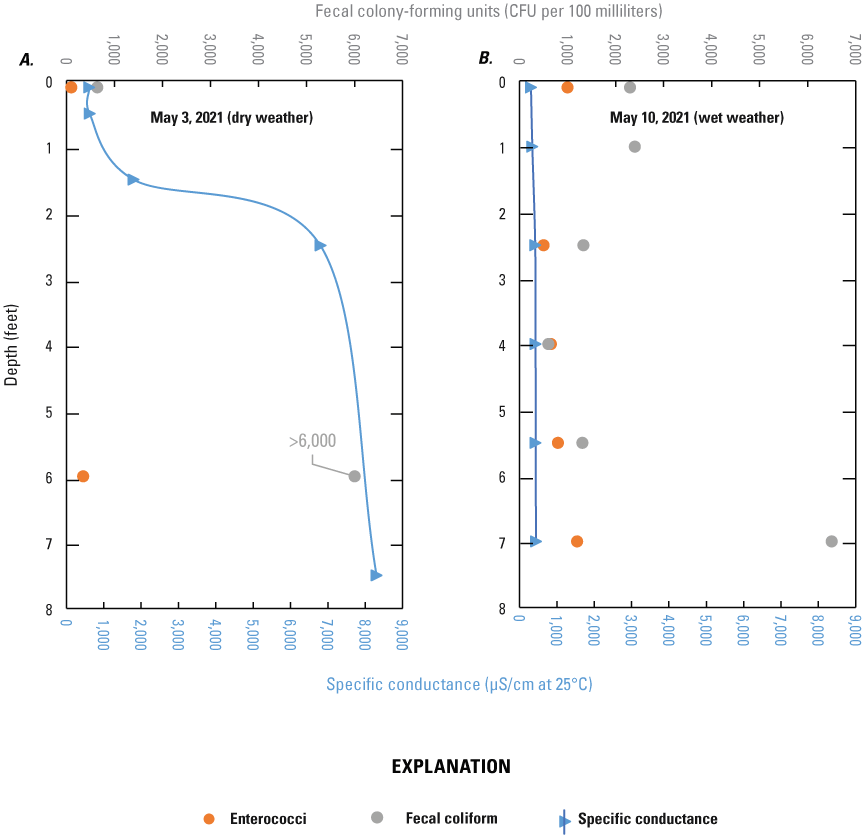
Depth-profile samples collected from a damaged storm-sewer line adjacent to outfall TI–024 on A, May 3, 2021, and B, May 10, 2021, Alley Creek, Queens County, New York. Results are presented in feet below water surface, with fecal indicator bacteria concentrations for fecal coliform and enterococci in colony-forming units per 100 milliliters (CFU/100mL) and specific conductance in microsiemens per centimeter at 25 degrees Celsius (μS/cm at 25 °C). Samples collected on May 3 were considered dry weather; samples collected on May 10 followed a recent precipitation (greater than 0.1 inch; wet weather) event. For sample site location map, see figure 2.
Thermal images were also collected around TI–024 by using an FLIR E8 handheld infrared camera to determine if a difference in water temperature was noticeable at and around the hole in the underground pipe leading to TI–024 (fig. 7; Fisher and others, 2021). Thermal gradients around the elevated outfall (fig. 7A), at the hole in the pipe (fig. 7B), and from areas of groundwater seepage (figs. 7C, D, and E) near TI–024 were assessed and compared to thermistor readings at the water surface using a YSI EXO sonde with a temperature probe (Fisher and others, 2021). No differences in surface temperatures were determined at the point of upwelling and at nearby and downstream points that would suggest significant thermal contributions from a different source (for example, a sewage leak).
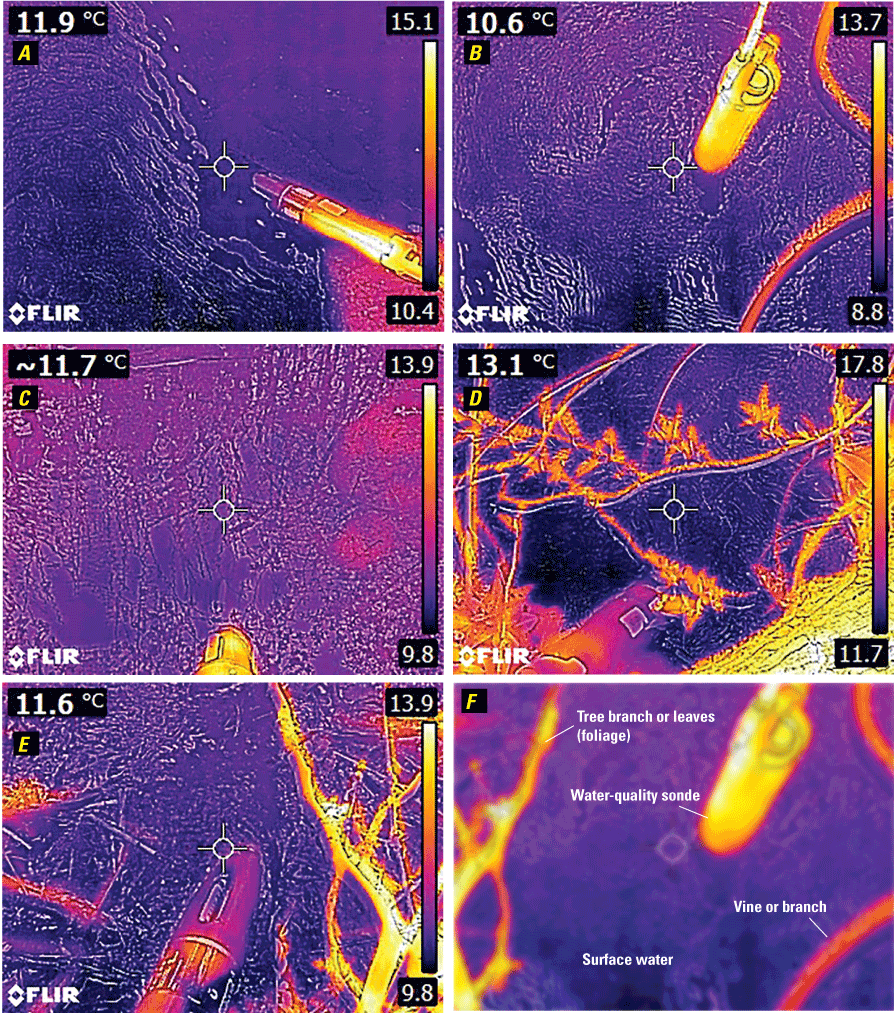
Thermal images taken using an FLIR E8 handheld infrared camera from sites at and around outfall TI–024, Alley Creek, Queens County, New York. The temperature reading in the top left corner of each image (in degrees Celsius [°C]) represents the average thermal reading in the center of the image (within the circle); a range of colors representing temperatures is along the right side of each image. A, Adjacent to TI–024 above-ground outfall structure. B, At the hole in the damaged storm-sewer pipe adjacent to TI–024 C, At a spring immediately upgradient from TI–024. D, At a freshwater pond near TI–024. E, Immediately downstream of flow from artesian well Q277. F, Composite image in which typical features of the thermal images are labeled. The multiparameter YSI EXO water-quality sonde used for temperature verification can be seen as the yellow object in A, B, and C, and in purple (bottom middle) in D and E. Tree branches can be seen in D and E. ~, approximate. For sample site location map, see figure 2.
Discussion
Interpretation of the microbiological and physicochemical data collected at the three outfalls along Alley Creek is presented based on temporal and spatial variability. Distinguishing by tidal stage and season provided a clear distinction in MST markers at TI–025 and TI–008, whereas wet-weather samples collected at TI–025 (during low tide) and at TI–024 differed in relative concentrations. Sediment contributions to the bacteria concentrations in the water column have been considered with respect to tidal stage and TSS results. Understanding differences in concentrations of MST markers, FIB and TSS under differing tidal and wet-weather conditions at each site and across sites, as well as using the pharmaceutical analytical method for additional information on source, may help inform management decisions regarding system repairs, upgrades, and maintenance.
Temporal Variability
Results from the two wet-weather samples at TI–025 and TI–024 showed different patterns of MST markers despite elevated FIB concentrations during both events. Precipitation preceding sample collection on September 30, 2020 (Weather Underground, 2021a, b, c), was sufficient to exceed the capacity of the 5,000,000-gal retention facility (NYCDEP, 2020), resulting in a combined sewer overflow at TI–025—this overflow is evidenced by the HF183/BacR287 marker concentrations detected at TI–025 that were three orders of magnitude higher in the September 30, 2020, wet-weather sample than in the preceding dry-weather samples. In contrast, dilution of the sewage signature discharging from the hole in the damaged storm sewer adjacent to TI–024 appears to have occurred and is reflected in the relatively lower concentrations of human MST markers in wet-weather samples as compared to dry-weather samples; however, concentrations of total pharmaceutical compounds and FIBs did not decrease proportionally and were within the same range as the dry-weather samples. Therefore, it is possible that contributions from stormwater infrastructure (for example, a storm drain or grit chamber) containing high concentrations of FIB resulted in the observed elevated FIB concentrations that were otherwise persistent in samples collected between August and December 2020.
There was a substantial difference in FIB and HF183/BacR287 concentrations during the low-tide sample collection following repair to the sanitary sewer running parallel to the combined sewer between Oakland Lake and TI–008. Concentrations of fecal coliform and HF183/BacR287 in the June 17, 2021, sample were 12 CFU/100 mL and <330 copies/100 mL, respectively, compared to low-tide concentrations of fecal coliform that ranged from 2,000 to 12,000 CFU/100 mL, and HF183/BacR287 that ranged from 170,000 to 3,700,000 copies/100 mL in samples prior to June 17, 2021. According to the NYCDEP (Jorge Villacis, NYCDEP, written commun., August 2021), repairs at TI–008 were conducted between April 27 and May 11, 2021. Whereas the precise date the leak stopped contributing to the TI–008 discharge is unknown, it was likely before May 3, 2021, based on low-tide concentrations of FIB at TI–008 on that date (12 CFU/100 mL fecal coliform and 46 CFU/100 mL enterococci, which were 26- and 7-times decreases, respectively, from the lowest concentrations collected for the previous three low-tide samples). Furthermore, the decrease in human markers and number and concentration of pharmaceuticals at TI–008 at low tide in such a short period of time between repairs and the June 2021 samples indicates the utility of combined analyses as a means of verifying successful implementation of corrective actions to sewage infrastructure through time.
Seasonal variability of canine and waterfowl MST markers was observed at the three outfall sites and Oakland Lake, while human markers were not observed to vary seasonally but did vary based on the tide and precipitation. Canine marker BacCan was detected in each of the December 2020 and March 2021 samples collected but infrequently in the August 2020 sample; this difference may be related to hotter summer months resulting in fewer dog walkers (Hinojosa and others, 2020) or fewer rain events causing runoff of parkland to the lake. Waterfowl markers were detected in at least one of the December 2020 samples from each site; the only other sample with a waterfowl marker concentration above the detection limit was TI–025 in August 2020. As was observed in an adjacent embayment during a 2018–19 study (Tagliaferri and others, 2021b) and in other MST studies (Weiskel and others, 1996), migratory waterfowl present in the watershed in spring and autumn (Matic and others, 2015) can directly contribute to bacterial contamination in receiving waters.
Spatial Variability
For much of the study period, there was a clear distinction between the three outfall sample sites with respect to concentrations of FIB and MST markers. Concentrations of FIB were consistently high at TI–024 and generally higher than at TI–025 under dry-weather conditions (figs. 4A, B). Dry-weather samples collected at TI–024 exhibited a range of concentrations of HF183/BacR287 similar to low-tide samples at TI–008, where a known source of sewage was influencing the water at the outfall. Groundwater from well Q277 was not found to be a source of bacteria or MST markers to Alley Creek, which was not surprising since Q277 is sourced from the deeper Magothy aquifer. Based on previous studies of shallow groundwater known to be affected by upgradient onsite wastewater disposal, fecal contamination is not likely because of the sandy substrate acting as a filter and limiting transport in the groundwater (Fisher and others, 2020; Tagliaferri and others, 2021b).
Correlation between either fecal coliform or enterococci and TSS concentrations was not observed at TI–024 and Oakland Lake and was mixed with respect to tide at sites TI–025 and TI–008 (table 2.2) in dry-weather samples. Correlation of both fecal coliform and enterococci with TSS was strong during high tide (ρ=0.880, P<0.05) when data from TI–008 were evaluated independently and when data from both TI–008 and TI–025 were combined; however, there was no correlation during low tide. Concentrations of TSS were 6 and 86 mg/L at TI–024 and TI–025, respectively, in the September 30, 2020, wet-weather samples, where both enterococci and fecal coliform were both >6,000 CFU/100 mL at each site. Conversely, TSS concentration in the May 10, 2021, wet-weather sample at TI–008 was 616 mg/L, and concentrations of enterococci and fecal coliform were lower than from September wet-weather samples. The overall lack of correlation between FIB and TSS can be attributed to factors including influences from sewage at TI–008 (particularly, dilution at high tide) and potential sampling interferences (such as bed sediment in the peristaltic pump sampling line during low tide).
The co-occurrence of the canine-associated BacCan and human-associated HF183/BacR287 MST markers was prevalent in this study, with 80, 71, and 60 percent of samples containing both markers at sites TI–024, TI–008, and TI–025, respectively. While studies have documented detection of both markers in sewage samples, often attributed to the cohabitation of humans and dogs (Kildare and others, 2007; Silkie and Nelson, 2009), differences in BacCan to HF183/BacR287 ratios throughout the study area and patterns at each site can be used to parse sources. Results from TI–025 and TI–024 show a similar pattern with respect to dry- and wet-weather conditions and, for TI–025, tidal stage (figs. 5B and C), which suggests coinciding sewage influence. At TI–008, the samples collected prior to June 2021 were influenced by both Oakland Lake and sewage and reflect both human and canine markers in most samples. In the June 17, 2021, (low tide) sample at TI–008, following repair of the sanitary sewer line, HF183/BacR287 was not detected above the reporting limit, but BacCan was detected, suggesting sources other than sewage (such as Oakland Lake). In samples collected at Oakland Lake in 2014 as part of the Alley Creek LTCP, one of five sample sets tested positive for human markers and no canine markers were detected (AECOM USA, 2014). In this study, only canine and waterfowl markers were detected (fig. 5), suggesting that dog or waterfowl waste regularly enters the lake through runoff from nearby sidewalks and roadways or other upstream sources, but there is no association with sewage that would also contain human markers.
Results from Little Neck Bay samples collected in May 2021 allowed for comparison of MST markers between source (that is, outfall sites along Alley Creek) and receptor (that is, Little Neck Bay). Interestingly, all three human MST markers—HF183/BacR287 and both CrAssphage—were detected in all samples along with similar patterns of BacCan, indicating widespread influence of sewage throughout the bay, although the source may also be from treated sewage and could explain the higher concentrations of HF183/BacR287 at LNB site 4 relative to the other three LNB sites because the Belgrave Sewage Treatment Plant discharges effluent nearby (fig. 2). However, this was not the case in an adjacent embayment to the northeast of the study area, Hempstead Harbor, sampled in 2018–19, where roughly 60 percent of samples from five open-water receptor sites detected HF183 above the method reporting limit (Tagliaferri and others, 2021b). The concentrations of HF183/BacR287 in Little Neck Bay were within the range found in Alley Creek, regardless of tidal stage, indicating consistent presence of markers likely being contributed from the East River and other wastewater treatment facilities within the Little Neck Bay watershed. If Alley Creek were the only source, it would be expected that concentrations of MST markers would be considerably lower because of dilution and marker decay (which one study of marine waters determined is roughly 6 days; Mattioli and others, 2017). Unfortunately, current analytical methods cannot readily discern whether Bacteroides or crAssphage viral indicators were introduced to the waterways by raw or treated sewage, or by surface runoff.
Decision Support and Future Work
Findings from this study may help guide future sampling and management strategies of outfalls along Alley Creek and provide additional context for persistently high concentrations of FIB that have been observed during regulatory compliance monitoring. Concentrations of FIB determined during this study and previous NYCDEP monitoring at TI–008 and TI–024 led to investigations of the sewer system and upstream sources. Sealing leaks, correcting illicit connections, and rerouting sewage away from CSO outfalls to retention tanks has been shown to improve water quality (AECOM USA, 2014). However, evidence of past and current bacterial contamination at TI–008 and TI–024 was found. Factors such as bed-sediment resuspension during tidal fluxes, wind or storm events, and the potential for sampling bias (particularly during low tide) was shown to increase detection of FIB in water samples from the resuspension experiment, despite TSS and FIB not correlating statistically at TI–008 and TI–025 at low tide or at TI–024 and Oakland Lake. There was also evidence of upgradient combined sewer line infiltration of sewage prior to upwelling along the way to outfall TI–024.
The sanitary sewer running parallel to the combined sewer that was leaking through a compromised joint was repaired between April 27 and May 11, 2021, thereby ceasing the constant infiltration of sewage into Alley Creek from TI–008. The clear difference in FIB and MST marker concentration in water samples collected at TI–008 before (six samples; fecal coliform concentrations ranged from 2,000 to 12,000 CFU/100 mL [low-tide samples] and from 8 to 321 CFU/100 mL [high-tide samples]) and after (two samples; fecal coliform concentrations were 12 and 40 CFU/100 mL) sewer line repair, as well as the order of magnitude difference between storm-sewer sediment and sediment at the TI–008 outfall, indicates the continued dosing of sediment within the line has likely been mitigated. Further consideration to the accumulation of sediment along the combined sewer line may be warranted given the concentrations of fecal coliform and enterococci in the sewer sediment just upgradient of the former leak (54,000 CFU/g dw and 25,000 CFU/g dw, respectively; Fisher and others, 2021). According to the Alley Creek LTCP, sediment within larger sewer lines must be removed as it accumulates; however, the sediment buildup within the combined sewer between Oakland Lake and TI–008 is the result of tidal flux moving and depositing bed sediment upgradient. Although these may be considered natural conditions, bacteria could potentially continue to incubate in the sediment (and thereby continue to pose a threat to downstream water quality) given the limited exposure to sunlight/UV radiation (Myers and Juhl, 2020). Outfall redesign to reduce sediment transport into the sewer line and (or) seasonal cleaning of sediment accumulating along the walls of the sewer at low tide may reduce source material that is resuspended in the line during tidal shifts and wet-weather events. Continued monitoring of TI–008 for FIB and TSS by the NYCDEP Harbor Survey Program (their site AC2), along with the addition of human MST markers and documentation of tidal stage and antecedent weather conditions, may indicate whether further inspections of the combined sewer line and cleanings are necessary. Additional bed-sediment samples and a more intensive resuspension experiment could be conducted to better quantify contributions of FIB to Alley Creek during events such as tidal exchanges, wakes, and storms that result in resuspension.
Within the drainage area of Alley Creek (including the nontidal reaches where TI–024 is located), the NYCDEP has been working for over a decade to track down illicit connections from buildings to storm-sewer pipes (AECOM USA, 2014) and has conducted dye testing, all of which has led to mitigation through repairs and disconnections of improper hookups in the drainage area. Within the past few years, the focus has been on the shoreline of Alley Creek and infrastructure integrity immediately surrounding TI–024. Although constant flow from the aboveground outfall TI–024 ceased during dry weather, upwelling can be observed adjacent to the outfall from a hole in the top of the storm-sewer pipe leading to it. It is possible that this flow is the result of hydraulic pressure causing groundwater to infiltrate into the damaged pipes at an upgradient location and then to emerge from the hole. Concentrations of FIB, HF183/BacR287, and pharmaceutical compounds from samples collected at TI–024 were consistently high, strongly suggesting that a persistent source of sewage is influencing the water upwelling at the outfall. This influence is further evidenced by the elevated concentrations of FIB throughout the depth profile observed during both sampling events, including after a storm that effectively flushed the pipe (thereby removing the density gradient). Since tidal influence is not regularly observed, sediment resuspension does not appear to be a major factor. Routine sample collection along a vertical gradient (that is, depth profiles) at the adjacent hole before and after each stage of future repairs upgradient (for example, cleaning inline grit chambers) could provide data needed to track progress and relative changes in FIB concentrations (and therefore, loads to Alley Creek) as investigations by the NYCDEP into probable causes continue. Following repairs, incorporating MST markers into the sample analysis along with FIB and TSS should improve monitoring of remedial work and for possible recurrence of sewage contamination.
Given the reliance on field-collected samples in determining compliance, care should be taken during sample collection, particularly during low tide in areas with shallow depth, to not introduce bias by disturbing bottom material or bed sediment. Routine documentation of water clarity, sampling depth, weather conditions, and tidal stage during the sample-collection process can provide necessary metadata for interpretation of data. Although not directly correlated at all sites and under all conditions during this study, collecting samples for TSS in addition to FIB may also be useful for understanding fluxes in solid particulates related to sediment and wastewater contributions.
The multiple lines of evidence approach used for this study (FIB analysis coupled with analyses for TSS, MST, and pharmaceutical compounds) proved useful for understanding the complex nature of fecal contamination inputs to Alley Creek. The bacterial and viral MST marker data, along with FIB data, were helpful in differentiating host sources (human, canine, and waterfowl) and watershed sources (outfalls, groundwater, and Oakland Lake) from receptor sites (Little Neck Bay). The CrAssphage analytical method used in this study showed a very good correlation between the two viral-MST human markers (CPQ_056 and CPQ_064) and the bacterial-MST human marker (HF183/BacR287) and that all were equally effective in detecting human influence. The viral CrAssphage marker may offer greater sensitivity and persist longer than Bacteroides and thus be more effective when human host sources are lower, or when samples become diluted (Stachler and others, 2018). Total concentrations of pharmaceutical compounds and HF183/BacR287 correlated marginally (ρ=0.585, P=0.005; n=21) and allowed a differentiation of samples with recent sewage inputs from ambient groundwater conditions.
Initially, additional sampling locations were sought along Alley Creek to assess alternative sites; however, thick, muddy bed sediment and an incised channel limited access by foot or boat and made sampling during low tide impractical. Additional samples collected along Alley Creek downgradient and upgradient from outfalls TI–024, TI–008, and TI–025 could serve to improve the dataset, especially at sites slated for remediation or repairs. Furthermore, verification by field crews that the model for discharge at TI–025 is accurate, or that lower precipitation amounts can cause overflow, may be warranted to better understand conditions observed on June 14, 2021. A field-deployed camera focused on the outfall at TI–025 collecting images at routine intervals and an in situ microbiological analyzer could also serve to confirm proper functioning at TI–025 and could also help evaluate remediation or wastewater system upgrades that are planned or carried out in the future.
Summary
Alley Creek, in Queens County, New York, has been designated by the New York State Department of Environmental Conservation as impaired for fecal coliforms related to runoff and combined sewer overflow contributions. From August 2020 to June 2021, using microbial source tracking (MST) methods, the U.S. Geological Survey conducted a study to assess potential host sources of fecal contamination—human, canine, and waterfowl—from three outfalls (TI–025, TI–008, and TI–024), natural waters at Oakland Lake, and groundwater from artesian well Q277 within the Alley Creek watershed and in Little Neck Bay. Results of the assessment revealed variable concentrations of fecal indicator bacteria (FIB; fecal coliforms and enterococci) that correlated with human Bacteroides marker HF183/BacR287 at TI–024 and at TI–008 and TI–025 when the latter two outfalls were evaluated collectively during high-tide conditions. Human microbial source tracking (MST) markers (including viral crAssphage markers CPQ_056 and CPQ_064) were detected in 27 of the 28 samples collected at the three outfall sites along Alley Creek during dry- and wet-weather conditions and regardless of tide, whereas canine Bacteroides (BacCan; 20 of 28 samples) and waterfowl-associated Helicobacter (GFD; 7 of 28 samples) markers were less prevalent.
Results from samples collected at Oakland Lake, which flows to Alley Creek through a combined sewer connection, indicated no sewage contamination in the lake. Groundwater samples collected adjacent to Alley Creek at well Q277 were below the reporting limits for all MST markers, and negligible concentrations of FIB and pharmaceutical compounds were observed. Neither Oakland Lake nor groundwater from Q277 was a significant source of FIB to Alley Creek for this study. During low tide, samples were influenced at TI–008 by a damaged sanitary sewer line parallel to the combined sewer connecting Oakland Lake to TI–008 and resulted in elevated FIB concentrations and human and canine MST markers. Repairs that occurred toward the end of the study resulted in a substantial decrease in FIB (roughly 100-fold decrease in fecal coliforms and enterococci compared to low-tide results prior to May 3, 2021) and MST marker (roughly 10,000-fold decrease in HF183/BacR287 compared to low-tide results prior to May 3, 2021) concentrations. An apparent seasonal variability in all December and March samples was observed in the BacCan and GFD markers at all sites where concentrations above the reporting limit were detected from the three outfall sites and Oakland Lake.
Samples collected at TI–024 and TI–025 following precipitation resulted in elevated concentrations of FIB, and samples collected during dry weather at TI–024 were also generally high for FIB but lower for MST markers compared with wet-weather samples. Bed-sediment and resuspension experiment data were useful for getting a baseline of FIB and MST markers and to help determine the potential effects of sediment when mixed in the water column at these sites. However, based on the data collected, correlation between FIB and TSS was only observed when TI–008 and TI–025 were evaluated collectively under high-tide conditions. Additional bed-sediment samples and a more intensive resuspension experiment would be needed to better quantify contributions of FIB to Alley Creek during events such as tidal exchanges, wakes, and storms that result in resuspension.
Results from the analysis of pharmaceutical compounds affirmed that human MST markers sourced from TI–008 (prior to June 2021), TI–024 (during both dry- and wet-weather conditions), and TI–025 (during wet-weather conditions) to Alley Creek were sewage related. This study provides information on sources of fecal contamination to Alley Creek and Little Neck Bay during dry- and wet-weather conditions and describes current (2021) status for future assessments. A baseline of data was also established related to the effects of tidal and other conditions leading to sediment resuspension and changes in FIB and MST marker concentrations following sewer infrastructure repairs. Options for future sampling and monitoring to limit bias and further expand the data are identified so that water quality may be improved and milestones (such as swimmable/fishable waters) outlined in the Alley Creek LTCP may be addressed.
References Cited
AECOM USA, 2014, Combined sewer overflow long term control plan for Alley Creek and Little Neck Bay: New York City Department of Environmental Protection, Capital Project no. WP–169, prepared by AECOM USA, 304 p. [Also available at https://www.dec.ny.gov/docs/water_pdf/csoalleycrk.pdf.]
Benotti, M.J., Fisher, S.C., and Terracciano, S.A., 2006, Occurrence of pharmaceuticals in shallow ground-water of Suffolk County, New York, 2002–2005: U.S. Geological Survey Open-File Report 2006–1297, 5 p., accessed December 27, 2021, at https://doi.org/10.3133/ofr20061297.
Bexfield, L.M., Toccalino, P.L., Belitz, K., Foreman, W.T., and Furlong, E.T., 2019, Hormones and pharmaceuticals in groundwater used as a source of drinking water across the United States: Environmental Science & Technology, v. 53, no. 6, p. 2950–2960, accessed December 7, 2021, at https://doi.org/10.1021/acs.est.8b05592.
Fisher, S.C., Kephart, C.M., and Cheung, N., 2021, Assessment of fecal contamination sources to Alley Creek, Queens County, New York—Results from a sediment resuspension experiment, thermal imagery, and additional sample collection, 2020–2021: U.S. Geological Survey data release, https://doi.org/10.5066/P9SLY4J8.
Fisher, S.C., McCarthy, B.A., Kephart, C.M., and Griffin, D.W., 2020, Assessment of water quality and fecal contamination sources at Hook Pond, East Hampton, New York: U.S. Geological Survey Scientific Investigations Report 2020–5071, 58 p., accessed August 12, 2021, at https://doi.org/10.3133/sir20205071.
Francy, D.S., Bushon, R.N., Cicale, J.R., Brady, A.M.G., Kephart, C.M., Stelzer, E.A., and Ecker, C.D., 2017, Quality assurance/quality control manual—Ohio Water Microbiology Laboratory: U.S. Geological Survey web page, accessed December 3, 2021, at https://oh.water.usgs.gov/micro_qaqc.htm.
Francy, D.S., Stelzer, E.A., Bushon, R.N., Brady, A.M.G., Mailot, B.E., Spencer, S.K., Borchardt, M.A., Elber, A.G., Riddell, K.R., and Gellner, T.M., 2011, Quantifying viruses and bacteria in wastewater—Results, interpretation methods, and quality control: U.S. Geological Survey Scientific Investigations Report 2011–5150, 44 p., accessed August 12, 2021, at https://doi.org/10.3133/sir20115150.
Furlong, E.T., Noriega, M.C., Kanagy, C.J., Kanagy, L.K., Coffey, L.J., and Burkhardt, M.R., 2014, Determination of human-use pharmaceuticals in filtered water by direct aqueous injection—High-performance liquid chromatography/tandem mass spectrometry: U.S. Geological Survey Techniques and Methods, book 5, chap. B10, 49 p., accessed August 12, 2021, at https://doi.org/10.3133/tm5B10.
Green, H.C., Dick, L.K., Gilpin, B., Samadpour, M., and Field, K.G., 2012, Genetic markers for rapid PCR-based identification of gull, Canada goose, duck, and chicken fecal contamination in water: Applied and Environmental Microbiology, v. 78, no. 2, p. 503–510, accessed August 12, 2021, at https://doi.org/10.1128/AEM.05734-11.
Green, H.C., Haugland, R.A., Varma, M., Millen, H.T., Borchardt, M.A., Field, K.G., Walters, W.A., Knight, R., Sivaganesan, M., Kelty, C.A., and Shanks, O.C., 2014, Improved HF183 quantitative real-time PCR assay for characterization of human fecal pollution in ambient surface water samples: Applied and Environmental Microbiology, v. 80, no. 10, p. 3086–3094, accessed August 12, 2021, at https://doi.org/10.1128/AEM.04137-13.
Helsel, D.R., Hirsch, R.M., Ryberg, K.R., Archfield, S.A., and Gilroy, E.J., 2020, Statistical methods in water resources: U.S. Geological Survey Techniques and Methods, book 4, chap. A3, 458 p., accessed December 7, 2021, at https://doi.org/10.3133/tm4A3. [Supersedes USGS Techniques of Water-Resources Investigations, book 4, chap. A3, version 1.1.]
Hinojosa, J., Green, J., Estrada, F., Herrera, J., Mata, T., Phan, D., Tanvir Pasha, A.B.M., Matta, A., Johnson, D., and Kapoor, V., 2020, Determining the primary sources of fecal pollution using microbial source tracking assays combined with land-use information in the Edwards Aquifer: Water Research, v. 184, article number 116211, 11 p., accessed August 12, 2021, at https://doi.org/10.1016/j.watres.2020.116211.
Kildare, B.J., Leutenegger, C.M., McSwain, B.S., Bambic, D.G., Rajal, V.B., and Wuertz, S., 2007, 16S rRNA-based assays for quantitative detection of universal, human-, cow-, and dog-specific fecal Bacteroidales—A Bayesian approach: Water Research, v. 41, no. 16, p. 3701–3715, accessed August 12, 2021, at https://doi.org/10.1016/j.watres.2007.06.037.
Matic, V., Larson, M., Swadek, R., and Bounds, K., 2015, Alley Creek watershed management and habitat restoration plan 2015: New York City Department of Parks and Recreation, Natural Resources Group, 210 p. [Also available at https://www.nycgovparks.org/greening/natural-resources-group/publication.]
Mattioli, M.C., Sassoubre, L.M., Russell, T.L., and Boehm, A.B., 2017, Decay of sewage-sourced microbial source tracking markers and fecal indicator bacteria in marine waters: Water Research, v. 108, p. 106–114, accessed August 12, 2021, at https://doi.org/10.1016/j.watres.2016.10.066.
Myers, D.N., Stoeckel, D.M., Bushon, R.N., Francy, D.S., and Brady, A.M.G., 2007, Fecal indicator bacteria (ver. 2.0, February 2007): U.S. Geological Survey Techniques of Water-Resources Investigations, book 9, chap. A7, section 7.1, accessed August 12, 2021, at https://doi.org/10.3133/twri09A7.1.
Myers, E.M., and Juhl, A.R., 2020, Particle association of Enterococcus sp. increases growth rates and simulated persistence in water columns of varying light attenuation and turbulent diffusivity: Water Research, v. 186, article number 116140, 15 p., accessed August 12, 2021, at https://doi.org/10.1016/j.watres.2020.116140.
New York City Department of Environmental Protection [NYCDEP], 2021, Harbor water-quality: New York City Department of Environmental Protection web page, accessed December 7, 2021, at https://www1.nyc.gov/site/dep/water/harbor-water-quality.page.
New York State Department of Environmental Conservation, [NYSDEC], 2020, Proposed final New York State 2018 section 303(d) list of impaired/TMDL waters: New York State Department of Environmental Conservation report, accessed December 7, 2021, at https://www.dec.ny.gov/docs/water_pdf/section303d2018.pdf.
O’Mullan, G.D., Juhl, A.R., Reichert, R., Schneider, E., and Martinez, N., 2019, Patterns of sediment-associated fecal indicator bacteria in an urban estuary—Benthic-pelagic coupling and implications for shoreline water quality: Science of the Total Environment, v. 656, p. 1168–1177, accessed December 7, 2021, at https://doi.org/10.1016/j.scitotenv.2018.11.405.
Siefring, S., Varma, M., Atikovic, E., Wymer, L., and Haugland, R.A., 2008, Improved real-time PCR assays for the detection of fecal indicator bacteria in surface waters with different instrument and reagent systems: Journal of Water and Health, v. 6, no. 2, p. 225–237, accessed August 12, 2021, at https://doi.org/10.2166/wh.2008.022.
Silkie, S.S., and Nelson, K.L., 2009, Concentrations of host-specific and generic fecal markers by quantitative PCR in raw sewage and fresh animal feces: Water Research, v. 43, no. 19, p. 4860–4871, accessed December 7, 2021, at https://doi.org/10.1016/j.watres.2009.08.017.
Stachler, E., Akyon, B., Aquino de Carvalho, N., Ference, C., and Bibby, K., 2018, Correlation of crAssphage qPCR markers with culturable and molecular indicators of human fecal pollution in an impacted urban watershed: Environmental Science & Technology, v. 52, no. 13, p. 7505–7512, accessed August 12, 2021, at https://doi.org/10.1021/acs.est.8b00638.
Stachler, E., Kelty, C., Sivaganesan, M., Li, X., Bibby, K., and Shanks, O.C., 2017, Quantitative crAssphage PCR assays for human fecal pollution measurement: Environmental Science & Technology, v. 51, no. 16, p. 9146–9154, accessed August 12, 2021, at https://doi.org/10.1021/acs.est.7b02703.
Standard Methods Committee of the American Public Health Association, American Water Works Association, and Water Environment Federation [Standard Methods Committee], 2006, 9222 Membrane filter technique for members of the coliform group, Part 9000—Microbiological examination, in Standard methods for the examination of water and wastewater (22d ed.): Washington, D.C., American Public Health Association.
Standard Methods Committee of the American Public Health Association, American Water Works Association, and Water Environment Federation [Standard Methods Committee], 2011, 2540 Solids, Part 2000—Physical and aggregate properties, in Standard methods for the examination of water and wastewater: Washington, D.C., American Public Health Association.
Standard Methods Committee of the American Public Health Association, American Water Works Association, and Water Environment Federation [Standard Methods Committee], 2017, 9222 Membrane filter technique for members of the coliform group, Part 9000—Microbiological examination, in Standard methods for the examination of water and wastewater (23d ed.): Washington, D.C., American Public Health Association.
Tagliaferri, T.N., Fisher, S.C., Kephart, C.M., Cheung, N., Reed, A.P., and Welk, R.J., 2021a, Overview and methodology for a study to identify fecal contamination sources using microbial source tracking in seven embayments on Long Island, New York: U.S. Geological Survey Scientific Investigations Report 2021–5033, 8 p., accessed December 7, 2021, at https://doi.org/10.3133/sir20215033.
Tagliaferri, T.N., Fisher, S.C., Kephart, C.M., Cheung, N., Reed, A.P., and Welk, R.J., 2021b, Using microbial source tracking to identify fecal contamination sources in an embayment in Hempstead Harbor on Long Island, New York: U.S. Geological Survey Scientific Investigations Report 2021–5042, 19 p., accessed December 7, 2021, at https://doi.org/10.3133/sir20215042.
U.S. Environmental Protection Agency [EPA], 2014, Method 1600—Enterococci in water by membrane filtration using membrane-enterococcus indoxyl-β-D-glucoside Agar (mEI): U.S. Environmental Protection Agency Office of Water, Report number EPA 821–R–14–011, accessed December 7, 2021, at https://www.epa.gov/sites/default/files/2018-06/documents/method_1600_sept-2014.pdf.
U.S. Geological Survey [USGS], 2021a, Ohio Water Microbiological Laboratory—Analytical methods: U.S. Geological Survey Ohio-Kentucky-Indiana Water Science Center web page, accessed December 27, 2021, at https://oh.water.usgs.gov/OWML/micro_methods_microbial_source_tracking_methods.htm.
U.S. Geological Survey [USGS], 2021b, USGS water data for the Nation: U.S. Geological Survey National Water Information System database, accessed December 27, 2021, at https://doi.org/10.5066/F7P55KJN. [Also available at https://waterdata.usgs.gov/nwis.]
U.S. Geological Survey [USGS], [variously dated], National field manual for the collection of water-quality data: U.S. Geological Survey Techniques of Water-Resources Investigations, book 9, chaps. A1–A9, [variously paged], accessed August 12, 2021, at https://www.usgs.gov/mission-areas/water-resources/science/national-field-manual-collection-water-quality-data-nfm.
Weather Underground, 2021a, 28th Ave, Bay Terrace—KNYNEWYO1105: Brookhaven, Ga., Weather Underground data, accessed August 12, 2021, at https://www.wunderground.com/dashboard/pws/KNYNEWYO1105.
Weather Underground, 2021b, Joseph’s Weather Station—KNYNEWYO1408: Brookhaven, Ga., Weather Underground data, accessed August 12, 2021, at https://www.wunderground.com/dashboard/pws/KNYNEWYO1408.
Weather Underground, 2021c, Oakland Gardens Station—KNYNEWYO234: Brookhaven, Ga., Weather Underground data, accessed August 12, 2021, at https://www.wunderground.com/dashboard/pws/KNYNEWYO234.
Weiskel, P.K., Howes, B.L., and Heufelder, G.R., 1996, Coliform contamination of a coastal embayment—Sources and transport pathways: Environmental Science & Technology, v. 30, no. 6, p. 1872–1881, accessed August 12, 2021, at https://doi.org/10.1021/es950466v.
Wilde, F.D., ed., 2004, Cleaning of equipment for water sampling: U.S. Geological Survey Techniques of Water-Resources Investigations, book 9, chap. A3, 68 p., accessed August 12, 2021, at https://doi.org/10.3133/twri09A3.
Appendix 1. List of Pharmaceutical Compounds Analyzed To Assess Fecal Contamination Sources to Alley Creek, Queens County, New York, August 2020–June 2021
Table 1.1.
Analytes for U.S. Geological Survey National Water Quality Laboratory schedule 2440 used to assess fecal contamination sources to Alley Creek, Queens County, New York, August 2020—June 2021.[Method and explanation of reporting limits available at Furlong and others (2014). Parameter code represents constituent identification number in the U.S. Geological Survey National Water Information System. Nonpharmaceutical compounds atrazine and piperonyl butoxide (pesticides) and methyl-1H-benzotriazole (deicing agent) were not counted towards summary of total concentrations or number of detected compounds. CASRN, Chemical Abstract Service registry number; ng/L, nanogram per liter; sur, surrogate; IS, internal standard; —, no data]
Reference Cited
Furlong, E.T., Noriega, M.C., Kanagy, C.J., Kanagy, L.K., Coffey, L.J., and Burkhardt, M.R., 2014, Determination of human-use pharmaceuticals in filtered water by direct aqueous injection—High-performance liquid chromatography/tandem mass spectrometry: U.S. Geological Survey Techniques and Methods, book 5, chap. B10, 49 p., accessed August 12, 2021, at https://doi.org/10.3133/tm5B10.
Appendix 2. Sediment Resuspension Data and Statistical Summary of Microbiological and Water-Chemistry Data, Alley Creek, Queens County, New York, August 2020–June 2021
Table 2.1.
Results from a sediment resuspension experiment using water and sediment collected at Alley Creek from the New York City Department of Environmental Protection combined sewer overflow outfall TI–008 through to Oakland Lake, Queens, New York, September 22, 2020.[From Fisher and others (2021). Sterile phosphate buffer. For sample site location map, see figure 2. CFU/100 mL, colony-forming units per 100 milliliters; NA, samples not included in resuspension experiment; E, estimated value; k, reported concentration estimated because colony counts were outside of the ideal range; <, value less than reporting limit for that sample (no target colony growth at any volume filtered); —, no qualifier; g, gram]
Table 2.2.
Determination of correlation between fecal indicator bacteria (fecal coliform and enterococci) and total suspended solids and human microbial source tracking marker HF183/BacR287, Alley Creek Study Area, Queens New York, August 2020–June 2021.[Sites with tidal influence were tested independently and together with each tide stage (low and high). Correlation was significant at P <0.050. For sample site location map, see fig. 2. n, number of values used for Spearman Rank correlation; ρ, Spearman correlation coefficient; P, significance value; TSS, total suspended solids; —, no data]
References Cited
Fisher, S.C., Kephart, C.M., and Cheung, N., 2021, Assessment of fecal contamination sources to Alley Creek, Queens County, New York—Results from a sediment resuspension experiment, thermal imagery, and additional sample collection, 2020–2021: U.S. Geological Survey data release, https://doi.org/10.5066/P9SLY4J8.
Helsel, D.R., Hirsch, R.M., Ryberg, K.R., Archfield, S.A., and Gilroy, E.J., 2020, Statistical methods in water resources: U.S. Geological Survey Techniques and Methods, book 4, chap. A3, 458 p., accessed December 7, 2021, at https://doi.org/10.3133/tm4A3. [Supersedes USGS Techniques of Water-Resources Investigations, book 4, chap. A3, version 1.1.]
Conversion Factors
International System of Units to U.S. customary units
Temperature in degrees Celsius (°C) may be converted to degrees Fahrenheit (°F) as follows: °F = (1.8 × °C) + 32.
Temperature in degrees Fahrenheit (°F) may be converted to degrees Celsius (°C) as follows: °C = (°F – 32) / 1.8.
Supplemental Information
Specific conductance is given in microsiemens per centimeter at 25 degrees Celsius (µS/cm at 25 °C).
Concentrations of chemical constituents in water are given in either milligrams per liter (mg/L), micrograms per liter (µg/L), or nanograms per liter (ng/L).
Concentrations of bacterial indicators are given in colony-forming units per 100 milliliters (CFU/100 mL) for water and in colony-forming units per gram dry weight (CFU/g dw) for bed sediment.
Abbreviations
AVLD
absolute value log-10 difference
BacCan
canine-associated Bacteroides marker
CFU
colony-forming units
CSO
combined sewer overflow
EPA
U.S. Environmental Protection Agency
FIB
fecal indicator bacteria
GenBac
general Bacteroides marker
GFD
waterfowl-associated Helicobacter marker
HF183/BacR287
human-associated Bacteroides marker
LTCP
long-term control plan
MST
microbial source tracking
NCMBL
Newtown Creek Microbiology Laboratory (New York City Department of Environmental Protection)
NFM
National Field Manual for the Collection of Water-Quality Data (U.S. Geological Survey)
NWIS
National Water Information System (U.S. Geological Survey)
NWQL
National Water Quality Laboratory (U.S. Geological Survey)
NYC
New York City
NYCDEP
New York City Department of Environmental Protection
NYSDEC
New York State Department of Environmental Conservation
OWML
Ohio Water Microbiology Laboratory (U.S. Geological Survey)
PTFE
polytetrafluoroethylene
qPCR
quantitative polymerase chain reaction
SMP
Sentinel Monitoring Program (New York City Department of Environmental Protection)
TSS
total suspended solids
USGS
U.S. Geological Survey
WIPCL
Wards Island Process Control Laboratory (New York City Department of Environmental Protection)
WRRF
Wastewater Resource Recovery Facility
For more information about this report, contact:
Director, New York Water Science Center
U.S. Geological Survey
425 Jordan Road
Troy, NY 12180–8349
(518) 285–5602
or visit our website at
Disclaimers
Any use of trade, firm, or product names is for descriptive purposes only and does not imply endorsement by the U.S. Government.
Although this information product, for the most part, is in the public domain, it also may contain copyrighted materials as noted in the text. Permission to reproduce copyrighted items must be secured from the copyright owner.
Suggested Citation
Fisher, S.C., Kephart, C.M., Cheung, N., and Tagliaferri, T.N., 2022, Assessment of fecal contamination sources to Alley Creek, Queens County, New York, August 2020–June 2021: U.S. Geological Survey Scientific Investigations Report 2022–5068, 35 p., https://doi.org/10.3133/sir20225068.
ISSN: 2328-0328 (online)
Study Area
Publication type | Report |
---|---|
Publication Subtype | USGS Numbered Series |
Title | Assessment of fecal contamination sources to Alley Creek, Queens County, New York, August 2020–June 2021 |
Series title | Scientific Investigations Report |
Series number | 2022-5068 |
DOI | 10.3133/sir20225068 |
Year Published | 2022 |
Language | English |
Publisher | U.S. Geological Survey |
Publisher location | Reston, VA |
Contributing office(s) | New York Water Science Center |
Description | Report: viii, 35 p.; Data Release |
Country | United States |
State | New York |
County | Queens County |
Other Geospatial | Alley Creek |
Online Only (Y/N) | Y |
Additional Online Files (Y/N) | N |
Google Analytic Metrics | Metrics page |