Hydrogeologic Framework of Southwestern Louisiana
Links
- Document: Report (11.4 MB pdf) , HTML , XML
- Data Release: USGS data release—Altitudes and thicknesses of hydrogeologic units of southwestern Louisiana
- Download citation as: RIS | Dublin Core
Acknowledgments
The author gratefully acknowledges the cooperation of the Public Works and Water Resources Division, Louisiana Department of Transportation and Development. Additional thanks are given to the Louisiana Department of Natural Resources and the U.S. Department of the Interior, Bureau of Ocean Safety and Environmental Enforcement, for maintaining and providing access to their well information databases.
Abstract
A hydrogeologic framework was constructed for the Coastal Lowlands aquifer system in southwestern Louisiana. Data from previous hydrogeologic and geologic studies were synthesized and expanded using 2,242 geophysical logs to map 4 hydrogeologic units: the Chicot aquifer system, Evangeline aquifer, Jasper aquifer system, and Catahoula aquifer. Raster surfaces were created for the base and thickness of each unit to provide a generalized framework that can be used for regional groundwater studies and as a foundation for additional or local refinement.
Introduction
Groundwater is a valuable resource for southwestern Louisiana (fig. 1) and supports a range of industrial and agricultural activities in addition to providing municipal and rural domestic supply. In 2015, about 927.3 million gallons of water per day (Mgal/d) were withdrawn in total from the four primary aquifers or aquifer systems in southwestern Louisiana—the Chicot aquifer system, the Evangeline aquifer, the Jasper aquifer system, and the Catahoula aquifer (fig. 2A) (Collier, 2018). The Chicot aquifer system, which is the shallowest and most extensively pumped of the four hydrogeologic units, accounted for about 91.6 percent (849.9 Mgal/d) of the total withdrawals. The Evangeline aquifer, Jasper aquifer system, and Catahoula aquifer accounted for about 3.1 percent (28.6 Mgal/d), 5.2 percent (48.0 Mgal/d), and 0.1 percent (0.93 Mgal/d), respectively.
Although substantial quantities of groundwater are present in the aquifers that underlie the study area (Nyman, 1989), overpumping can lead to declining water levels and saltwater encroachment. The Chicot aquifer system has experienced declining water levels that have altered flow directions (Lovelace and others, 2004), particularly as result of seasonal withdrawals for rice irrigation, which accounted for more than 48 percent of the total withdrawals from the aquifer system in 2015 (Collier, 2018). Saltwater occurs in the Chicot aquifer system in southwestern Louisiana beneath coastal parishes, in localized areas near the cities of Lake Charles, Iowa, and Abbeville, and in some areas within the Mississippi alluvial valley (Nyman, 1984) (fig. 1).
Increased development may create additional demand that can present challenges for the stewardship of groundwater resources. Information on the geometry and extents of aquifers and confining units are basic components for analyses of groundwater flow, availability, and quality. Previous studies have described hydrogeologic units in southwestern Louisiana, but in various scales of resolution and areal extent or with a focus on a single aquifer (Whitfield, 1975; Nyman, 1984; Milner and Fisher, 2009). Many areas lack available maps or cross sections, and there are inconsistencies between different sources of information.
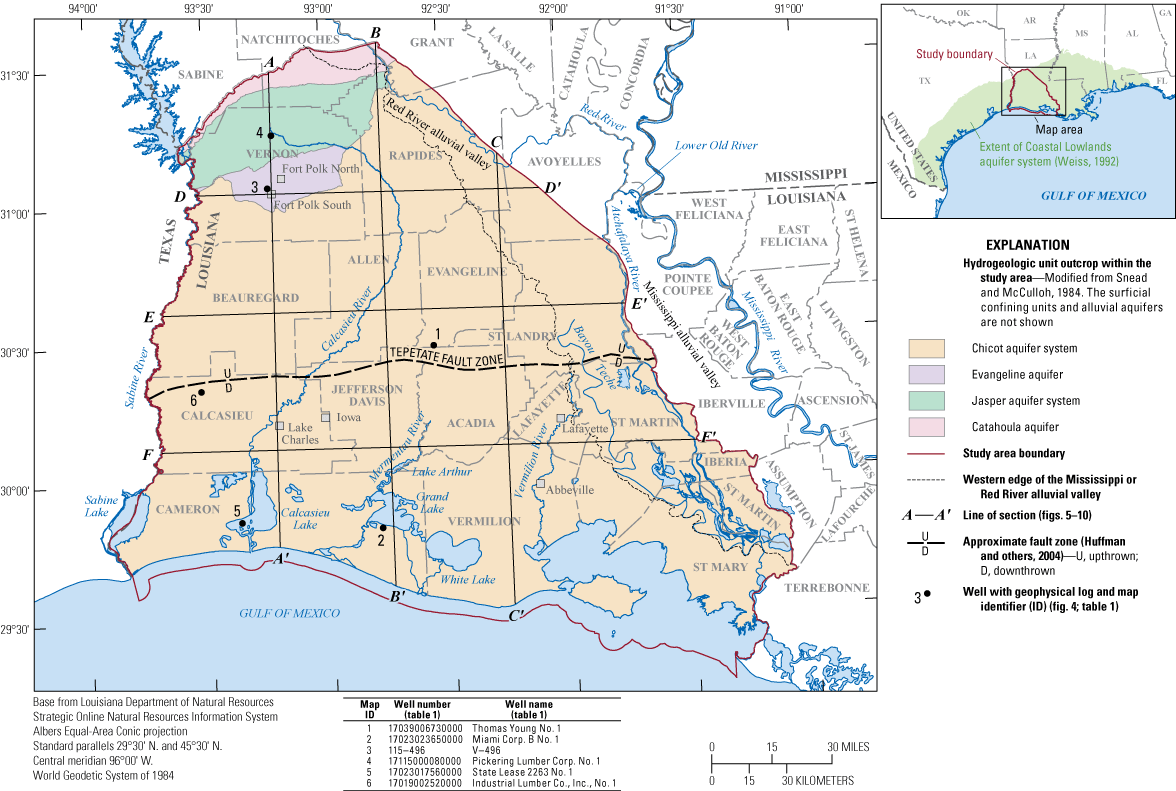
Location of the study area, with streams, cities, cross-section traces, and the locations of wells with example geophysical logs in southwestern Louisiana.
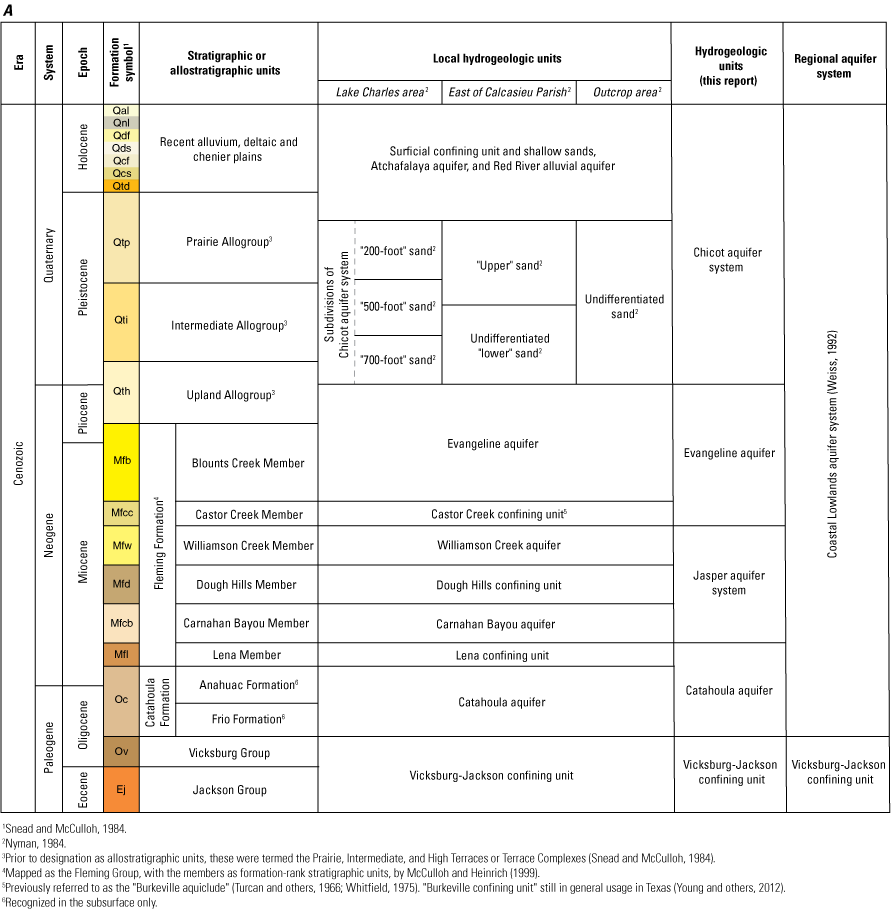
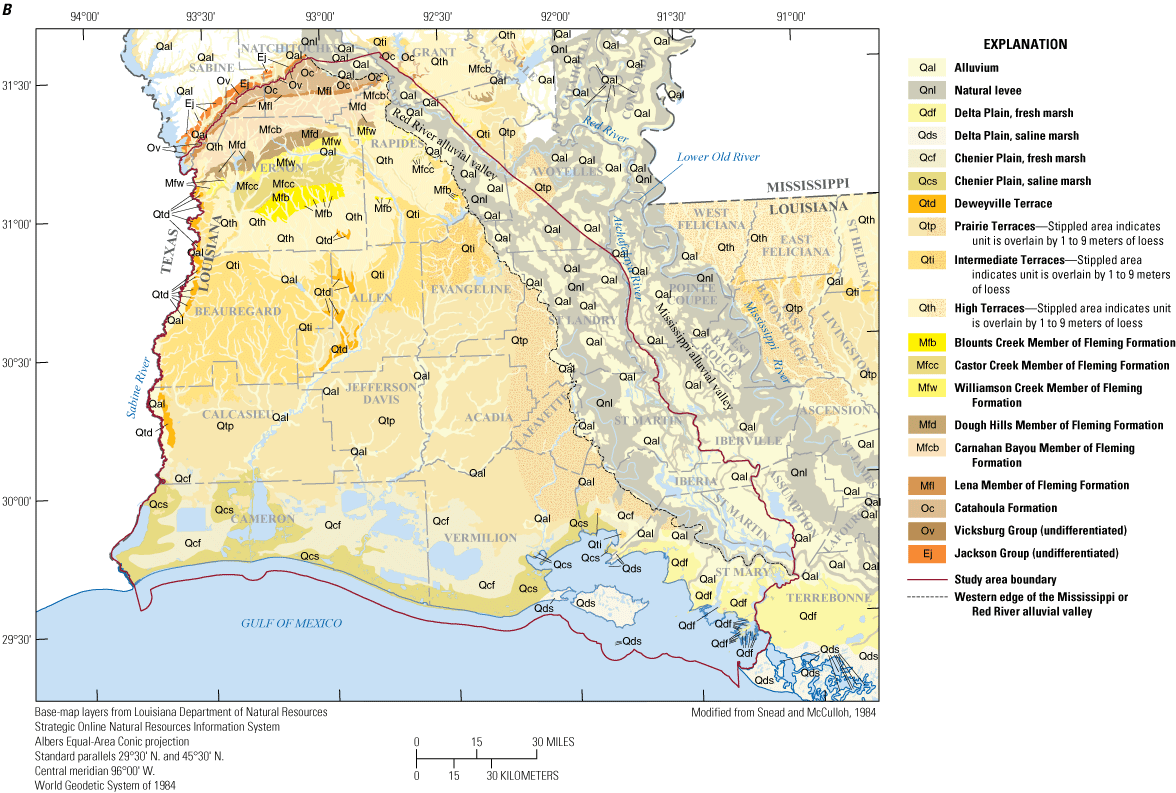
A, Chart showing stratigraphic and hydrogeologic units of southwestern Louisiana (modified from Johnston and others, 2000), and B, map showing stratigraphic units at land surface and other landforms, southwestern Louisiana (modified from geology reference layer from the Louisiana Department of Natural Resources [Louisiana DNR] Strategic Online Natural Resources Information System [SONRIS] [Louisiana DNR, 2020]). Original geologic map from Snead and McCulloh (1984).
Purpose and Scope
The U.S. Geological Survey (USGS), in cooperation with the Louisiana Department of Transportation and Development, has mapped the structure of four generalized hydrogeologic units in southwestern Louisiana: the Chicot aquifer system, Evangeline aquifer, Jasper aquifer system, and the Catahoula aquifer (fig. 2A). The study area is approximately bounded by the northern extent of the Coastal Lowlands aquifer system to the north, the Gulf of Mexico to the south, the Texas-Louisiana border to the west, and the Atchafalaya River or Red River to the east (fig. 1). An accompanying data release (Lindaman, 2023) provides a table of point data and derived raster surfaces for the base altitude and thickness of each unit. For tracking future updates, the data presented in this report and the accompanying data release are considered version 1.0. These data are intended for the study of groundwater resources at a regional scale.
Geologic Setting
The hydrogeologic units described in this study are part of the Coastal Lowlands aquifer system (fig. 2A), which is composed of Oligocene-age and younger sediments underlying about 160,000 square miles of the coastal plain of Texas, Louisiana, Mississippi, Alabama, western Florida, and nearby offshore areas of the Gulf of Mexico (Weiss, 1992) (fig. 1). The aquifer system forms a wedge of heterogenous unconsolidated to semiconsolidated sediments that were deposited in a range of shifting nonmarine, transitional, and marine environments. The predominant lithologies are sand, silt, and clay, with subordinate amounts of gravel, marl, and lignite (Williamson and Grubb, 2001).
In southwestern Louisiana, deposition occurred primarily through two large extrabasinal river systems—the Mississippi and Red Rivers, or their ancestral equivalents (Galloway, 2005) (fig. 1). These river systems delivered material eroded from the continental interior of North America and constructed networks of fluvial-deltaic systems that built progressively outward into the Gulf of Mexico. The coastal plain of the northern Gulf of Mexico has been low-lying and relatively flat through the entire Cenozoic Era, and the low relief allows for pronounced changes in response to variations in sea level, sediment supply, and land subsidence. Transgressive events, or periods of relative sea-level rise, punctuated longer periods of deltaic outbuilding. During these events, marine and transitional environments temporarily shifted inland, and relatively thin tongues of marine clay were deposited, some of which are regionally extensive and contain abundant nannofossils and foraminifera that facilitate correlation and provide age control. These marine clays are useful for sequence-stratigraphic analysis and have been used as “depositional episode” boundaries to construct a stratigraphic framework for the offshore and nearshore areas of the northern Gulf of Mexico (Galloway and others, 2000).
The marine facies of the Eocene Jackson Group and Oligocene Vicksburg Group extend to outcrop, but subsequent transgressive marine clays pinch out in the subsurface. The late Oligocene- through Miocene-age deposits that overlie the Jackson and Vicksburg Groups are nonmarine in character at their outcrop (fig. 2B) and have been mapped as the Catahoula and Fleming Formations in southwestern Louisiana. The Fleming Formation (or Fleming Group [McCulloh and Heinrich, 1999]) includes six members (or formations) that are differentiated by their bulk lithology and stratigraphic position in outcrop and the shallow subsurface (Fisk, 1940; Rogers and Calandro, 1965; Newcome and Sloss, 1966, McCulloh and Heinrich, 1999; Prakken and Griffith, 200043) (fig. 2A). The six members are lithologically heterogenous but alternate between relatively finer or coarser grained bulk compositions that are thought to reflect deposition in either brackish-water or fluvial environments, or more specifically in lower or upper coastal plain environments, respectively (Hinds, 1999). The relatively coarser grained stratigraphic units are the Carnahan Bayou, Williamson Creek, and Blounts Creek Members, and the relatively finer grained units are the Lena, Dough Hills, and Castor Creek Members.
The late Pliocene- through Pleistocene-age sediments that overlie the Fleming Formation reflect the influence of intensified glacial-interglacial cyclicity and the associated increase in the sediment supply rate during this period. During glacial periods, sea level fell, and the change in base level led to channel incision. As sea level rose during interglacial periods, these channels were filled with glacial outwash, forming fining-upward sequences of gravel and sand capped by silts and clays (Fisk, 1940). Repeated cycles of this process led to the formation of a thick amalgamated sequence of braided stream deposits across southwestern Louisiana that were subsequently covered by backswamp, levee, and crevasse splay deposits of the meandering Red and Mississippi Rivers (Jones and others, 1954; Saucier, 1994; Milner and Fisher, 2009). At land surface, the Pliocene-Pleistocene deposits exhibit varying degrees of preservation of their original fluvial-deltaic depositional structures (Saucier, 1994; Snead and others, 2019; Heinrich and others, 2020) and are broadly expressed across the coastal plain of the Gulf of Mexico as three primary coast-parallel “terrace” landforms that step up from the Holocene floodplain or coastal plain (fig. 2B). These terrace features are the exposed remnants of older coastal plains that have been uplifted relative to nearshore areas where deltaic deposition and greater subsidence occurs (Snead and others, 2019). In Louisiana, the three coast-parallel terraces have most recently been conceptualized as allostratigraphic units and termed the Upland, Intermediate, and Prairie Allogroups (fig. 2A) (McCulloh and others, 2003), and were previously referred to informally as the High, Intermediate, and Prairie Terraces (Snead and McCulloh, 1984) (fig. 2B).
Structural Features
The primary structural features within the study area are faults and salt domes (fig. 3). Many of the faults are high-angle listric growth faults that trend east-west and are downthrown to the south. Offset typically increases with depth, and individual beds can thicken abruptly across the fault plane. Some faults form surficial scarps that indicate recent movement (McCulloh and Heinrich, 2012). Faulting may displace the boundaries of hydrogeologic units and can act as both conduits or leaky barriers to lateral and vertical fluid migration.
Salt domes are common throughout the southern half of the study area (fig. 3) and rise to variable depths in the subsurface. They are formed by the plastic flow of deeply buried salt deposits in response to sediment loading and they grow as sediments are deposited around them (Williamson and Grubb, 2001). Their growth is associated with both uplift and subsidence of surrounding sediments. Uplift and drag occur along and above the domes, where sediment beds drape across and pinch out over their flanks or arch over their tops. Subsidence and down-warping occur around the rims of the structures (Hamlin, 2006). The diameters of the tops of salt domes are typically between 1 and 3 miles but associated radial faulting can continue for miles away from the domes (Jones and others, 1954). The surrounding strata can be substantially deformed, but the most substantial deformation is limited to their immediate vicinity.
Salt domes are mostly halite salt, and their crests are capped by relatively insoluble minerals such as anhydrite, gypsum, and calcite left behind by dissolution of the salt stock by groundwater (Hamlin, 2006). Dissolution of salt domes may contribute to the concentrated brine water that occurs throughout the deeper parts of the aquifer system and in shallower sediments near the domes (Williamson and Grubb, 2001). Within the study area, the Lockport dome (fig. 3) has been associated with the presence of saltwater in the Chicot aquifer system near the city of Lake Charles, Louisiana (Suding and Wicks, 2013). Other notable salt domes within the study area are the “five islands” structures, consisting of the Avery Island, Belle Isle, Cote Blanche Island, Jefferson Island, and Weeks Island domes (fig. 3), where the caprock rises above land surface by as much as 150 feet (ft) (Milner and Fisher, 2009).
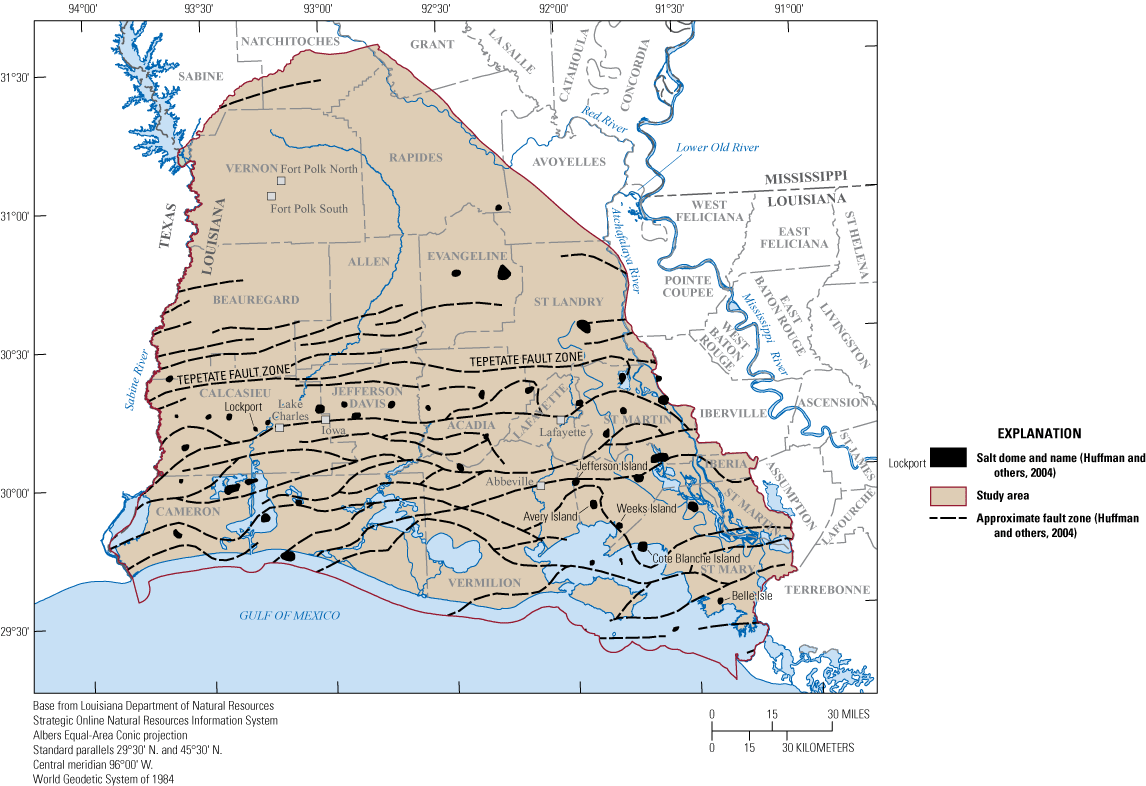
Approximate locations of faults and salt domes in southwestern Louisiana.
Hydrogeology
Under predevelopment conditions, groundwater in southwestern Louisiana flowed from topographically higher recharge areas such as the outcrop bands in central Louisiana (fig. 2B) towards major river valleys and discharge areas near the coast (Williamson and Grubb, 2001). Under postdevelopment conditions, part of this flow has been redirected towards areas with intensive pumping (Nyman and others, 1990; Martin and Whiteman, 1999; Williamson and Grubb, 2001; Lovelace and others, 2004; Vahdat-Aboueshagh and Tsai, 2021).
The Coastal Lowlands aquifer system is predominantly composed of sands and clays arranged in complex assemblages. Where deposition occurred in nonmarine environments, the sands that form the aquifers are generally interconnected at a large scale but interbedded with clays that control local patterns of lateral and vertical flow (Weiss, 1992). The thickness of beds can change abruptly, with sands ranging from thin beds to stacked channel deposits that are hundreds of feet thick. Each nonmarine sequence grades into a thicker, time-equivalent sequence of intermeshed deltaic, shorezone, and shallow marine deposits. In comparison to the nonmarine sequence, the sands in these facies are generally finer grained and confined by more continuous layers of clay.
The top of the Coastal Lowlands aquifer system is considered land surface (Weiss, 1992), but in southwestern Louisiana the groundwater system is generally confined by a layer of clay with interspersed sands, referred to as the surficial confining unit (fig. 2A). The surficial confining unit thickens towards the Gulf of Mexico and ranges from a thin veneer to more than 520 ft thick, with an average thickness of about 135 ft (Sargent and others, 2004). Where sufficiently thick, it reduces infiltration of precipitation and separates surface-water bodies from underlying aquifers (Jones and others, 1954; Nyman and others, 1990). Although considered a confining unit in aggregate, its discontinuous sand lenses can supply water to small-diameter wells (Sargent and others, 2004).
The base of the Coastal Lowlands aquifer system in upland areas is the top of the Vicksburg-Jackson confining unit (fig. 2A), a regionally extensive clay that is typically several hundreds of feet thick (Weiss, 1992). An additional constraint on deep groundwater flow is the occurrence of overpressure (or “geopressure”). Overpressure is defined as formation pressure that exceeds what would be expected at a given depth based on the normal hydrostatic pressure gradient; for the Gulf of Mexico, the normal hydrostatic pressure gradient is 0.465 pound per square inch per foot ([lb/in2]/ft) (Burke and others, 2013). Overpressure is thought to be caused by an increased resistance to fluid flow by the compaction of clays, or the isolation of sand beds by fault displacement (Weiss, 1992). The increased resistance to flow impedes fluid expulsion, which increases fluid pressure. Because overpressure occurs where fluid flow is impeded, the top of the zone of overpressure has been used to define the base of the Coastal Lowlands aquifer system where it is shallower than the Vicksburg-Jackson confining unit (Weiss, 1992; Martin and Whiteman, 1999; Williamson and Grubb, 2001).
Hydrogeologic Units
Quantification of groundwater flow is affected by the geometries, extents, and hydraulic properties of hydrogeologic units. However, several characteristics of the Coastal Lowlands aquifer system complicate its subdivision into a conventional sequence of aquifers and confining units. These characteristics were described in detail by Weiss (1992) and include (1) facies changes in all directions, (2) discontinuity of individual sand and clay beds, (3) heterogeneous but repetitious sedimentation, (4) indefinite limits to locally defined stratigraphic and hydrogeologic units, and (5) an absence of unique geophysical log patterns or paleontological markers to aid correlation through nonmarine and transitional facies. Additional challenges are introduced by the uncertain locations and offsets across faults and salt domes, unknown subcrop locations, and the irregular coverage or quality of available geophysical logs. Because of these difficulties, different studies have subdivided the Coastal Lowlands aquifer system in ways that can vary considerably in their details or overall method. Through time, a generalized framework has been established in southwestern Louisiana that uses formations or groupings of formations to define broad hydrogeologic units—the Chicot aquifer system, Evangeline aquifer, Jasper aquifer system, and Catahoula aquifer (fig. 2A).
Previous Studies of the Chicot Aquifer System
The Chicot aquifer system was formally named by Jones and others (1954) to describe the thick sand and gravel deposits of Pleistocene age in southwestern Louisiana. Subsequent studies of various scopes have expanded on their work. Harder (1960) subdivided the Chicot aquifer system into three sands—the “200-foot,” “500-foot,” and “700-foot” sands near Lake Charles in Calcasieu Parish. East of Calcasieu Parish, Whitman and Kilburn (1963) described “lower” and “upper” sand units (fig. 2A). Harder and others (1967) recognized an “upper” sand unit and the threefold subdivision established in Calcasieu Parish and correlated these sands across several cross sections. Nyman (1984) consolidated information from these earlier studies and showed the interconnections of the sands of the Chicot aquifer system in a series of cross sections and maps, and later developed a groundwater flow model (Nyman and others, 1990). The Chicot aquifer system and surficial confining unit were also characterized across southwestern Louisiana by the Louisiana Geological Survey (LGS) (Milner and Fisher, 2009) in response to saltwater encroachment concerns.
A study commissioned by the Texas Water Development Board (TWDB) to refine the hydrogeologic framework of the Texas Gulf Coast also included part of southwestern Louisiana near the Louisiana-Texas State line (Young and others, 2012). In that study, the Chicot aquifer of Texas was divided into three chronostratigraphic units that correspond with the three main terraces (or allostratigraphic units, see fig. 2A) of the coastal plain of the northern Gulf of Mexico. Attempts have been made to correlate the terrace surfaces into the subsurface in Louisiana (Jones and others, 1954; Akers and Holck, 1957; McFarlan and LeRoy, 1988), but their subsurface expression is unclear (Harder, 1960; Nyman, 1984; Saucier, 1994; Milner and Fisher, 2009).
Most recently, a lithofacies model for the Chicot aquifer system was constructed to a depth of about 2,625 ft using an extensive geophysical and drillers’ log dataset (Vahdat-Aboueshagh and Tsai, 2021). Existing geologic correlations were used to determine dip directions and angles, and then an indicator interpolation algorithm was used to construct a lithofacies model of sand and clay layers.
Previous Studies of the Evangeline Aquifer, Jasper Aquifer System, and Catahoula Aquifer
The Evangeline aquifer was named by Jones and others (1954) to describe the discontinuous sands that underlie the Chicot aquifer system in southwestern Louisiana. The aquifer was not mapped in their 1954 report, but the sands that form the aquifer were assigned a Pliocene age and were described as sufficiently connected to function as a singular aquifer unit at a larger scale. Later work by Turcan and others (1966) showed the Evangeline aquifer in two cross sections that spanned the Louisiana-Texas State line and showed two additional hydrogeologic units—the “Burkeville aquiclude” (referred to as the Castor Creek confining unit in southwestern Louisiana in later publications) and the Jasper aquifer system (fig. 2A). These units were described as consisting of stratigraphic units or groupings of stratigraphic units, including the members of the Fleming Formation described at the surface in the Miocene outcrop belt in southwestern Louisiana. The Evangeline aquifer was described as equivalent to the Blounts Creek Member, the “Burkeville aquiclude” equivalent to the Castor Creek Member, and the Jasper aquifer system equivalent to the Williamson Creek, Dough Hills, and Carnahan Bayou Members (fig. 2A). Around the same time, these stratigraphic units and the Catahoula Formation were correlated into the subsurface and operatively treated as hydrogeologic units as part of county-level water resource bulletins in Rapides and Vernon Parishes in southwestern Louisiana (Rogers and Calandro, 1965; Newcome and Sloss, 1966).
The hydrogeologic nomenclature suggested by Turcan and others (1966) was generally adopted and maintained in subsequent studies in Texas (Baker, 1979; Carr and others, 1985; Strom and others, 2003a, 2003b, 2003c; Young and others, 2012) and Louisiana. In Louisiana, Whitfield (1975) correlated the Fleming Formation members through several cross sections and included derived contour maps for the tops of the Evangeline aquifer and Jasper aquifer system. Smoot (1989) constructed generalized cross sections for the State of Louisiana that extended the hydrogeologic units of southwestern Louisiana across the Mississippi alluvial valley into southeastern Louisiana, where they were designated the Chicot, Evangeline, Jasper, and Catahoula “equivalent” aquifer systems. Later work by Prakken and Griffith (2000) analyzed geophysical logs near Fort Polk in Vernon Parish (fig. 1) and annotated them with the contacts of the aquifers and confining units named after the members of the Fleming Formation.
An alternative method to subdivide the Coastal Lowlands aquifer system was used by Weiss (1992) as part of the USGS Regional Aquifer-System Analysis (RASA) Program. Given the large extent of the program’s study area, reconciling the multitude of local hydrogeologic and stratigraphic units was impractical. Instead, the aquifer system was subdivided based on other criteria, including vertical variations in hydraulic head near major pumping centers, confining units of limited areal extent, and extrapolations of layer boundaries by using percentages of the total aquifer system thickness. The RASA hydrogeologic framework ultimately consisted of five permeable “zones” and three confining units. The top of the zone of overpressure in the subsurface was taken as a barrier to flow, and the model layers were progressively truncated southward against the top of the zone.
More recently, the TWDB developed a hydrogeologic framework to support groundwater availability modeling that included a portion of southwestern Louisiana near the Texas-Louisiana border (Young and others, 2012). Their study characterized the Chicot, Evangeline, and Jasper aquifers of Texas using chronostratigraphic concepts, with their boundaries defined by lines of correlation drawn between updip stratigraphic units and downdip marine clays.
Methodology
The hydrogeologic framework developed in this study maintains the established subdivisions of the Coastal Lowlands aquifer system consisting of the Chicot aquifer system, Evangeline aquifer, Jasper aquifer system, and Catahoula aquifer. Information was compiled from relevant geologic and hydrogeologic publications, then synthesized and augmented with additional well log data obtained from publicly available sources. Some assumptions and simplifications were necessitated by the scale of the study area and to reconcile different sources of information. These are described in subsequent sections of this report describing the selection of boundaries.
The hydrogeologic units were mapped by using a total of 2,242 geophysical well logs, including gamma ray, spontaneous potential, single point resistance, and various types of resistivity logs. Resistivity log types included long-short normal and induction resistivity. Log images were loaded into the Neuralog software suite (Neuralog, 2020), and a grid of cross sections was created to facilitate correlation. To better preserve regional trends, hydrogeologic unit boundaries were not interpreted for well logs near salt domes where substantial local deformation or offset of strata was apparent.
The hydrogeologic unit boundary interpretations were exported from Neuralog and brought into ArcPro (Esri, 2022) for interpolation. Raster surfaces were generated by using a natural neighbor technique (Esri, 2022), which was found to efficiently produce reasonable surfaces even though the spatial distribution of data points was sparse, linear, or clustered in certain locations. Natural neighbor interpolation does not extrapolate beyond the convex hull of the input data points; to extend the surfaces to their inferred outcrop or subcrop limits or the geographic boundaries of the study area, a total of 301 synthetic data points were used. Of this number, 176 data points were distributed across outcrop or estimated subcrop margins to extend the interpolated surfaces toward their northern extents. The remaining 125 data points were used to extend the surfaces to the geographic boundaries of the study area.
The rasters were created to match the orientation and 1-square-kilometer cell size of the National Hydrogeologic Grid (Clark and others, 2018). Because of the relative importance of the Chicot aquifer system with respect to water use, more logs were used to characterize its base surface compared to the other units. Consequently, picks for only the base of the Chicot aquifer system were made for some logs even though picks for the deeper surfaces may have been possible. In general, picks were made for the other hydrogeologic units in the northern part of the study area where they contain more freshwater1 (Smoot, 1988).
Freshwater contains a chloride concentration of 250 milligrams per liter or less.
Following the method of Weiss (1992), the base of the Coastal Lowlands aquifer system was defined by the top of the Vicksburg-Jackson confining unit, or by the top of the zone of overpressure where it occurs above the top of the Vicksburg-Jackson confining unit. Interpretations of hydrogeologic unit surfaces were made as far south as either their intersection with the top of the zone of overpressure or the approximate shoreline of the Gulf of Mexico. The first occurrence of the 0.60 (lb/in2)/ft pressure gradient, as derived from the isopressure gradient contour map of Burke and others (2013), was used to define the top of the zone of overpressure. The contours were converted into a raster surface in ArcGIS using the “Topo to Raster” geoprocessing tool. The raster value was subtracted from a digital elevation model (DEM) to convert to an approximate altitude relative to the North American Vertical Datum of 1988 (NAVD 88). The resulting raster was then used to clip the hydrogeologic unit surfaces where the unit base altitudes fell below the raster surface.
Sources of Data
Logs collected during oil and gas drilling were obtained from the Louisiana Department of Natural Resources (Louisiana DNR) Strategic Online Natural Resources Information System (SONRIS) (Louisiana DNR, 2020). Additional logs and paleontological reports for the offshore area were obtained from the Bureau of Safety and Environmental Enforcement (2019) online data center. Water well logs were scanned from paper copies stored in the log libraries of the USGS and Louisiana DNR offices in Baton Rouge, Louisiana.
Land surface altitudes were derived from the USGS National Elevation Dataset 1/3-arc-second DEM (USGS, 2013) and the National Oceanic and Atmospheric Administration Coastal National Elevation Database 3-meter resolution topobathymetric DEM for the Gulf of Mexico (National Oceanic and Atmospheric Administration, National Ocean Service, Office for Coastal Management, 2014). The use of a topobathymetric DEM was required for offshore areas and coastal waterbodies. The two DEMs were mosaiced, with preference assigned to the Coastal National Elevation Database DEM where they overlapped. DEM values were rounded to the nearest foot.
The measured depth shown on well logs is typically relative to a point on the drilling rig above land surface, and the depth scale should be corrected for this height. The height of this point above land surface or a fixed point on the wellhead is usually recorded on the well log header. In many cases, the height was reported relative to the casing head (or “bradenhead”) rather than land surface without additional clarification. The casing head is a metal adapter that connects the first casing string to the wellhead and was assumed to be at land surface. In cases where altitude information was not recorded or is illegible, the log was assumed to have been measured from 18 ft above land surface for oil and gas wells and 1 ft above land surface for water wells. These values are the average for the dataset rounded to the nearest integer. Logs measured from offshore rigs were recorded from a point above “mean water level” or “mean sea level,” and these values were taken directly from the well header.
Selection of Boundaries for the Chicot Aquifer System
The Chicot aquifer system has been described in several studies, and the information presented in this report was synthesized from their interpretations (Jones and others, 1954; Harder and others, 1967; Whitfield, 1975; Nyman, 1984; Milner and Fisher, 2009). The top of the Chicot aquifer system is defined here as land surface, and consequently its total thickness includes the overlying surficial confining unit. Characterizing the surficial confining unit requires the analysis of drillers’ logs because most oil and gas geophysical logs terminate before land surface is reached.
The Chicot aquifer system is differentiated from the underlying Evangeline aquifer primarily by its higher content of sand and gravel (Jones and others, 1954; Harder, 1960; Whitfield, 1975; Baker, 1979; Milner and Fisher, 2009) (see fig. 4A for example geophysical log; well information in table 1). This lithologic difference often results in higher resistivities on geophysical logs for the sands of the Chicot aquifer system (Whitfield, 1975). Across the study area, the sands of the Chicot aquifer system are also generally thicker and more amalgamated and exhibit greater lateral continuity than those of the Evangeline aquifer (Jones and others, 1954).
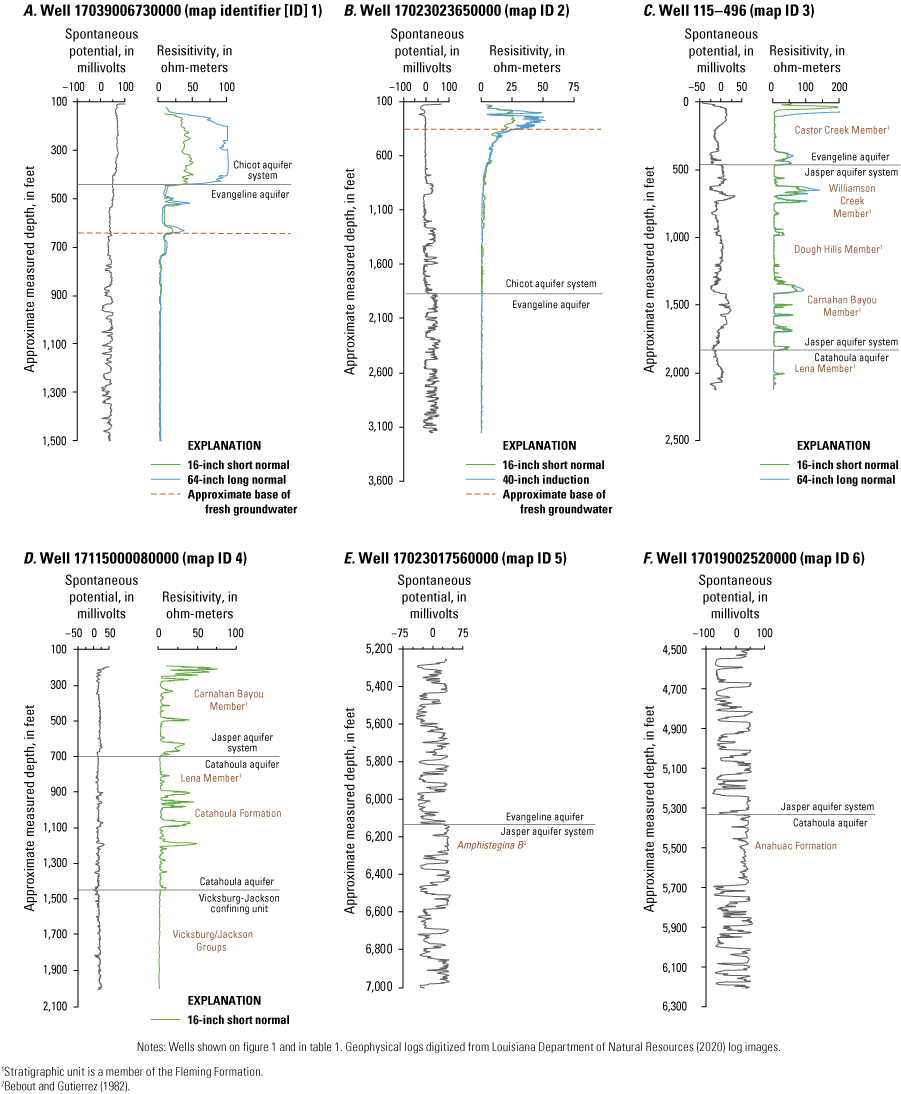
Examples of geophysical logs showing hydrogeologic unit boundaries in southwestern Louisiana.
Table 1.
Information for selected wells with example geophysical logs in southwestern Louisiana.Map identifier (fig. 1) |
Well number | Well name | Log-measured depth (feet) |
Parish |
---|---|---|---|---|
1 | 17039006730000 | Thomas Young No. 1 | 9,003 | Evangeline |
2 | 17023023650000 | Miami Corp. B No. 1 | 18,000 | Cameron |
3 | 115-496 | V-496 | 2,125 | Vernon |
4 | 17115000080000 | Pickering Lumber Corp. No. 1 | 8,524 | Vernon |
5 | 17023017560000 | State Lease 2263 No. 1 | 13,586 | Cameron |
6 | 17019002520000 | Industrial Lumber Co., Inc., No. 1 | 8,180 | Calcasieu |
Towards the south in the coastal parishes, the lower part of the Chicot aquifer system grades into finer textured sediments that are increasingly intercalated by silts and clays (Jones and others, 1954; Nyman, 1984; Milner and Fisher, 2009) (fig. 4B). In this area, the Chicot aquifer system is not easily differentiated from the underlying Evangeline aquifer on lithologic criteria alone. Interpretations of the base of the Chicot aquifer system vary substantially among reports in this region, and more recent reports that used chronostratigraphic styles of correlation (Milner and Fisher, 2009; Young and others, 2012) generally pick the base deeper than earlier publications. Determination of the base of the Chicot aquifer system near the coast for this report was guided by using publications that included cross sections through this area (Nyman, 1984; Milner and Fisher, 2009). The east-west cross section through the coastal area shown in McFarlan and LeRoy (1988), which shows six Pleistocene time-stratigraphic units, was also used for reference. This horizon was correlated updip until it merged with the base of the thick, amalgamated sands in the middle part of the study area (fig. 4A), and then it was correlated northward towards the outcrop area.
Different boundaries have been used to demarcate the northern outcrop limit of the Chicot aquifer system. Nyman (1984) showed a generalized recharge area, but structural contours or data points were not shown. Milner and Fisher (2009) associated the recharge area and northern extent of the Chicot aquifer system with the surface extent of the Intermediate Allogroup (fig. 2A). For this report, the northern extent of the Chicot aquifer system was fixed to the northern limit of the “High Terraces” as depicted in the LGS 1984 Geologic Map of Louisiana (Snead and McCulloh, 1984) (fig. 2B).
Within the limits of the Mississippi alluvial valley (fig. 2B), the Chicot aquifer system is overlain and progressively truncated to the north by the more recently deposited alluvium that composes the Atchafalaya aquifer (Nyman, 1984). Similarly, the Chicot aquifer system effectively merges with the Red River alluvial aquifer in the northeastern corner of the study area within the Red River alluvial valley (fig. 2B). The Atchafalaya aquifer and Red River alluvial aquifer are compositionally similar and hydraulically connected to the Chicot aquifer system (Harder and others, 1967; Nyman, 1984) and were not differentiated in this report.
Selection of Boundaries for the Evangeline Aquifer, Jasper Aquifer System, and Catahoula Aquifer
Published hydrogeologic information on the Evangeline aquifer, Jasper aquifer system, and Catahoula aquifer is limited. The contacts between these hydrogeologic units have conventionally been interpreted by identifying the relatively finer grained members of the Fleming Formation, which are conceptualized as intervening confining units (fig. 2A) (Turcan and others, 1966; Whitfield, 1975; Smoot, 1989; Prakken and Griffith, 2000). Near the outcrop area, the Castor Creek, Dough Hills, and Lena Members (confining units) are generally composed of silt and clay, and the sands that occur within them tend to be fine grained and discontinuous. In comparison, the Blounts Creek, Williamson Creek, and Carnahan Bayou Members, and the underlying Catahoula Formation, generally contain higher percentages of sand (fig. 4C and 4D).
A complication of using the members of the Fleming Formation to delineate hydrogeologic units through the entire study area is that they are only recognizable in the subsurface near their outcrop areas, and their contacts tend to be indistinct. Towards the south, the members of the Fleming Formation are increasingly difficult to differentiate because of facies changes. Approximately south of Vernon and Rapides Parishes (fig. 2B), they lose their lithologic distinction (Holland and others, 1952; Varvaro, 1957; McCulloh and Heinrich, 1999). An additional complication is the lack of borehole data near their outcrop areas that are sufficiently deep (or shallow) enough to map these units in three-dimensional space (McCulloh and Heinrich, 1999; Prakken and Griffith, 2000).
Given these constraints, representing the members of the Fleming Formation that were previously conceptualized as intervening confining units as discrete layers is difficult, and a simplification was made to include them with the larger overlying or underlying hydrogeologic units. The sediments associated with the relatively thin Lena Member (Lena confining unit) were included with the Catahoula aquifer, and the sediments of the Castor Creek Member (Castor Creek confining unit) were included with the Evangeline aquifer. In this manner, the base of the Evangeline aquifer in updip areas is defined in this report by the approximate base of the Castor Creek Member, and the base of the Jasper aquifer system is defined by the approximate base of the Carnahan Bayou Member (fig. 2A).
Publications with cross sections, contour maps, or annotated well log images were used to help identify these stratigraphic units (Rogers and Calandro, 1965; Whitfield, 1975; McCulloh and Heinrich, 1999; Prakken and Griffith, 2000). The outcrop bands shown in the LGS 1984 Geologic Map of Louisiana (Snead and McCulloh, 1984) were used to estimate the northern extents of each hydrogeologic unit. The northern extents of the Evangeline aquifer, Jasper aquifer system, and Catahoula aquifer were fixed to the northern extents of the Castor Creek Member, the Carnahan Bayou Member, and the Catahoula Formation, respectively. In many places, the outcrops of these stratigraphic units are buried beneath recent alluvium or subcrop into overlying units. Where this occurs, their extents were estimated by manually extrapolating the trend of their outcrop bands.
Downdip from the outcrop areas, the deep subsurface is typically differentiated based on paleontological zonation and the boundaries provided by transgressive marine shales that occur within the repetitive sequence of interfingering aggradational or progradational coastal sediments (Rainwater, 1964; Bebout and Gutierrez, 1982, 1983; Galloway and others, 2000; Young and others, 2012). Hydrogeologic studies in Texas have used the more extensive marine shales to help delineate downdip hydrogeologic layer boundaries (Knox and others, 2006; Young and others, 2012). A similar approach is used in this report to better constrain the overall geometry of the aquifer system. The use of prominent transgressive shales to define hydrogeologic unit boundaries has several benefits. Sand bodies are less likely to be vertically connected across transgressive shale facies (Young and others, 2012), and major marine transgressions are associated with large-scale changes in sediment dispersal and accumulation patterns (Galloway, 2005). From a practical standpoint, the shales provide multiple points of correlation and can be traced across relatively large areas. The shales typically contain paleontological markers that provide age control and further aid correlation. The base of each hydrogeologic unit underlying the Chicot aquifer system was fixed to the top of a marine shale in the deep subsurface, and these horizons were then correlated northward and merged with the approximate lithologic boundaries of the members of the Fleming Formation.
The base of the Evangeline aquifer, and the top of the Jasper aquifer system, was placed at the top of a marine shale associated with the paleomarker Amphistegina B (fig. 4E). The shale tongue associated with Amphistegina B, like other transgressive marine shales overlying the Anahuac Formation, does not extend as far inland as the Anahuac Formation, and consequently its association with the members of the Fleming Formation is uncertain. However, some limited paleontological evidence and age constraints are available that indicate that the Amphistegina B shale tongue may approximate the downdip stratigraphic position of the Castor Creek Member. Vertebrate fossils have been recovered from sites within the outcrop belt of the Castor Creek Member in Fort Polk, Louisiana (Schiebout, 1994; Schiebout and others, 1996), and subsequent work has collected palynological samples and paleomagnetic information (Schiebout and others, 2004). Paleomagnetic information has placed the Fort Polk sites between 12.6 and 13.8 mega-annum, which supports the inference of Hinds (1999) that the Castor Creek Member may in part be the updip expression of the middle Miocene transgressive event associated with the Amphistegina B paleomarker (fig. 5 in Williams and Schiebout, 2003). The Castor Creek Member has also been reported to contain the brackish-water molluscan species Potamides matsoni and associated fauna (Fisk, 1940; Welch, 1942; Rogers and Calandro, 1965). This paleontological marker has not been encountered in more recent studies of the outcrop area (Schiebout, 1994; McCulloh and Heinrich, 1999); however, its zone of occurrence has been traced in the subsurface, and the basal part of this zone coincides with the approximate top of the Amphistegina B fauna zone (Skinner, 1972; Brunhild, 1984). Additionally, Ewing (2016) indicated that projection of the marine shale associated with the Amphistegina B paleomarker approximately corresponds to the outcrop of the Castor Creek Member.
The shaley zone associated with the Amphistegina B paleomarker was identified by using data from paleontological reports from offshore wells (Bureau of Safety and Environmental Enforcement, 2019), LGS regional geologic cross sections (Bebout and Gutierrez, 1982, 1983), and the interpretations from Young and others (2012). Regional time-stratigraphic contour maps based on other paleontological markers were also used as reference (Huffman and others, 2004).
The top of the Catahoula aquifer in downdip areas, and the base of the Jasper aquifer system, was placed at the top of the Anahuac Formation (fig. 2A), a regionally extensive transgressive marine shale that pinches out in the subsurface and is thought to be the subsurface equivalent of the Catahoula Formation (Fisk, 1940; Ellisor, 1944; Holland and others, 1952; Varvaro, 1957; Galloway, 1977; Johnston and others, 2000) (fig. 2A). The Anahuac Formation is identified on well logs as a wedge of fine-grained sediments (fig. 4F) that thickens rapidly towards the south from its pinchout. Its middle zone is associated with the paleomarker Heterostegina sp. (Fisk, 1940; Ellisor, 1944; Holland and others, 1952; Varvaro, 1957; Bebout and Gutierrez, 1982, 1983; Galloway and others, 2000; Young and others, 2012). Several publications that included geologic cross sections, well log data, or contours were used to help identify the Anahuac Formation (Holland and others, 1952; Varvaro, 1957, Bebout and Gutierrez, 1982, 1983; Huffman and others, 2004; Young and others, 2012).
The base of the Catahoula aquifer was placed at the top of the Vicksburg-Jackson confining unit (fig. 2A), a continuous fine-grained interval (fig. 4D) that represents the only transgressive marine facies in the study area that extends to outcrop. Data from the USGS RASA study (Wilson and Hosman, 1988) and LGS regional geologic cross sections (Bebout and Gutierrez, 1982, 1983) were used to help identify the top of the Vicksburg-Jackson confining unit.
Limitations
The hydrogeologic units of this report are large subdivisions of the total sequence and encompass substantial heterogeneity. The changeable character of the sediments makes the selection of hydrogeologic unit boundaries at least partially arbitrary, but efforts were made to maintain the established subdivisions and to associate their boundaries with identifiable features in the subsurface and at the surface. Uncertainty is introduced through the limitations of log correlation through large parts of the sequence, and correlation between logs often relied on extrapolation of approximate dip angles or projection towards inferred outcrop limits. The mapped units provide a generalized structural framework for regional analyses but will be of limited use where a characterization of individual sand aquifers or clay confining units is needed.
Characteristics of Hydrogeologic Units
The configurations of the mapped hydrogeologic units are shown in generalized regional cross sections across the study area (fig. 1). Cross sections A–Aʹ, B–Bʹ, and C–Cʹ (figs. 5–7) are aligned closer to the orientation of regional dip, and sections D–Dʹ, E–Eʹ, and F–Fʹ (figs. 8–10) are aligned closer to regional strike. All the units gradually dip and thicken towards the south-southeast, but the easterly trend is less pronounced for the Chicot aquifer system. The Chicot aquifer system is the only unit that is completely above the top of the zone of overpressure within the study area as defined by the 0.60 (lb/in2)/ft pressure-gradient surface. A part of the Evangeline aquifer is within the zone of overpressure in the southeastern corner of the study area, and the Jasper aquifer system and the Catahoula aquifer are truncated by this zone farther inland.
The base of fresh groundwater shallows towards the Gulf of Mexico and the Mississippi alluvial valley (figs. 8–10) and is deepest in northern Beauregard and southern Vernon and Rapides Parishes (Smoot, 1989). Shallowing of the base of fresh groundwater can occur abruptly and is likely associated with faulting. The Tepetate fault zone, although its location is only approximately known (fig. 3), appears to demarcate an area to the south where fresh groundwater is largely restricted to the Chicot aquifer system (figs. 5–7).
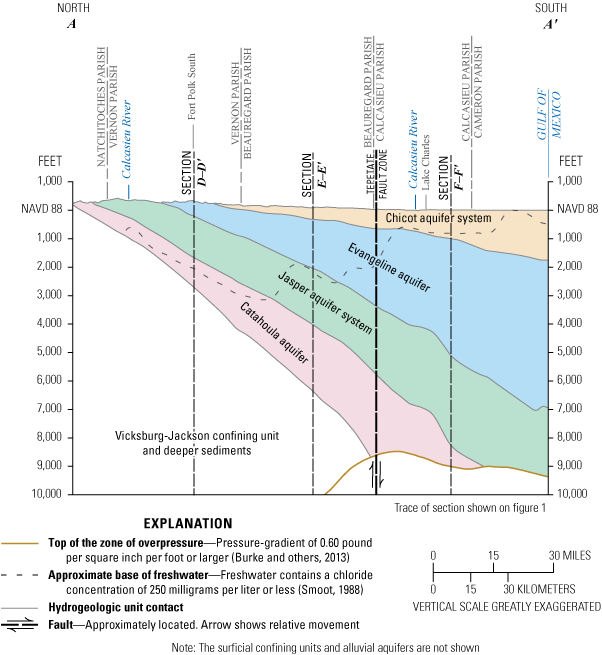
Idealized north-to-south cross section A–Aʹ through southwestern Louisiana, showing hydrogeologic units. Trace of section shown on figure 1.
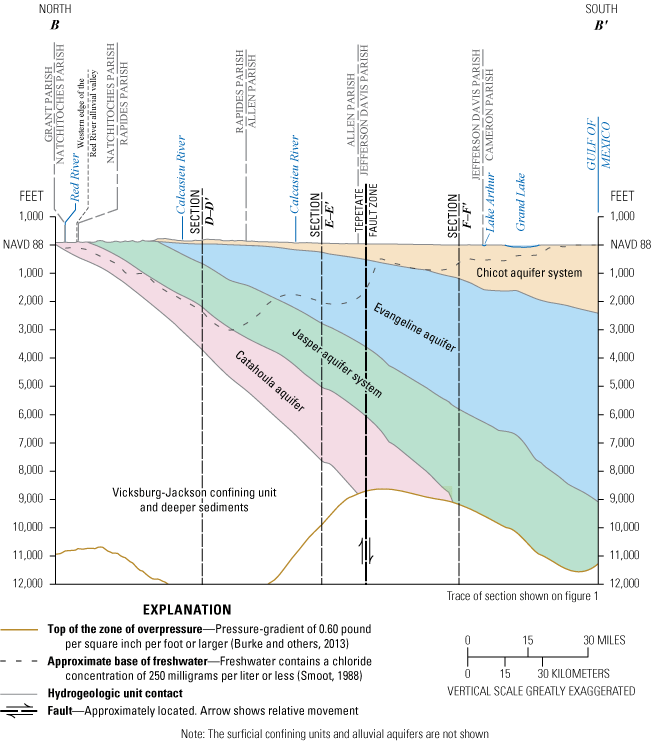
Idealized north-to-south cross section B–Bʹ through southwestern Louisiana, showing hydrogeologic units. Trace of section shown on figure 1.
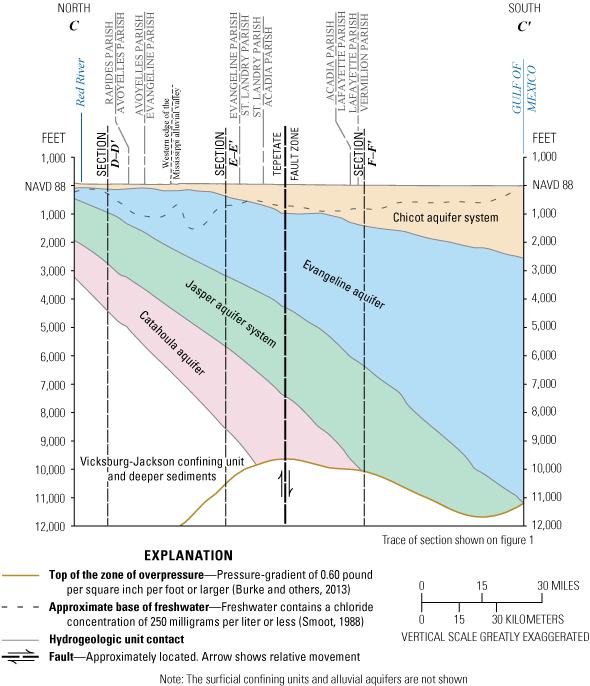
Idealized north-to-south cross section C–Cʹ through southwestern Louisiana, showing hydrogeologic units. Trace of section shown on figure 1.
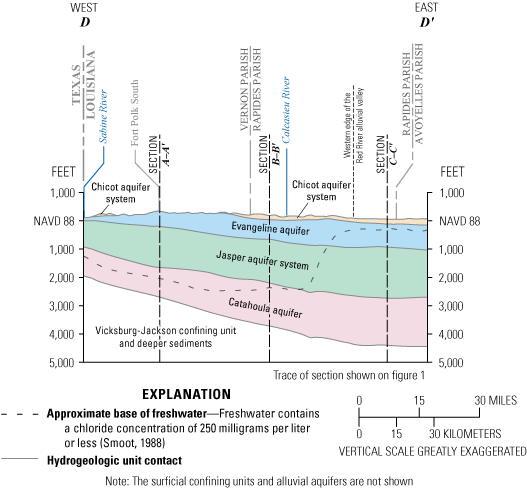
Idealized west-to-east cross section D–Dʹ through southwestern Louisiana, showing hydrogeologic units. Trace of section shown on figure 1.
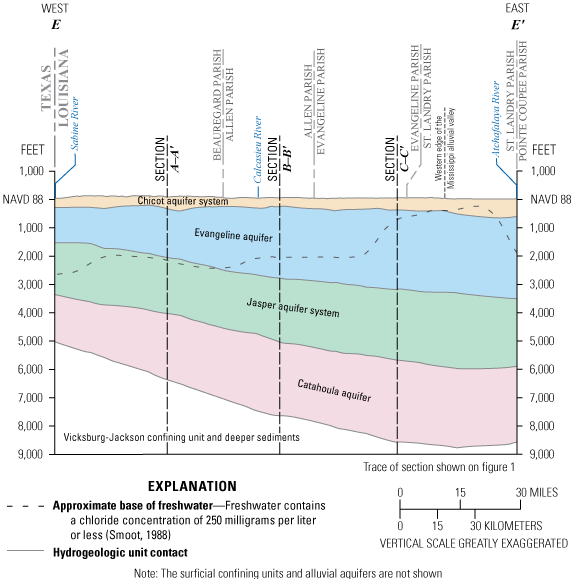
Idealized west-to-east cross section E–Eʹ through southwestern Louisiana, showing hydrogeologic units. Trace of section shown on figure 1.
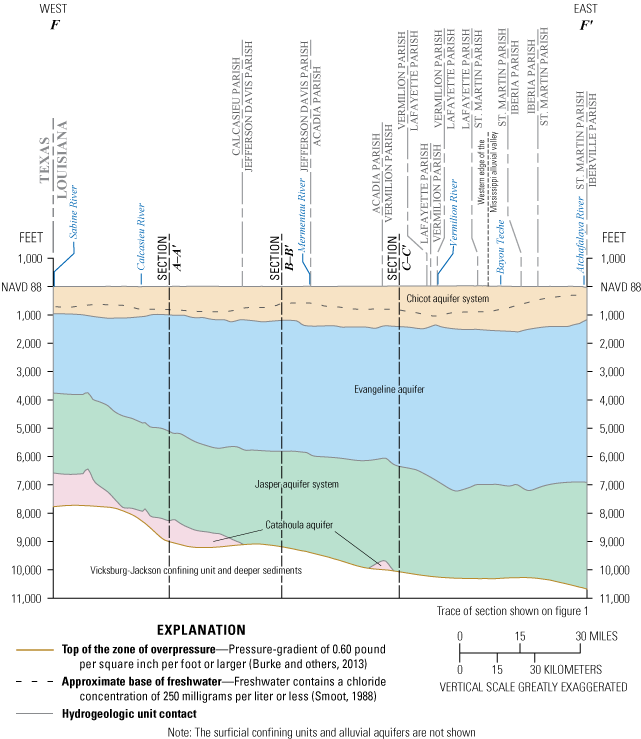
Idealized west-to-east cross section F–Fʹ through southwestern Louisiana, showing hydrogeologic units. Trace of section shown on figure 1.
Chicot Aquifer System
The altitude of the base of the Chicot aquifer system within the study area ranges from 326 ft above NAVD 88 along its northern limits to 3,908 ft below NAVD 88 offshore from St. Mary Parish (fig. 11), where it reaches a thickness of 3,896 ft (fig. 12). Within northern Allen and Evangeline Parishes and Rapides Parish, the dip of the base of the aquifer system flattens, but its thickness becomes more irregular, owing to the irregular surface that the deposits were laid down upon and subsequent erosion (Newcome and Sloss, 1966). The aquifer system contains freshwater in at least a portion of its total thickness through most of the study area (figs. 5–10).
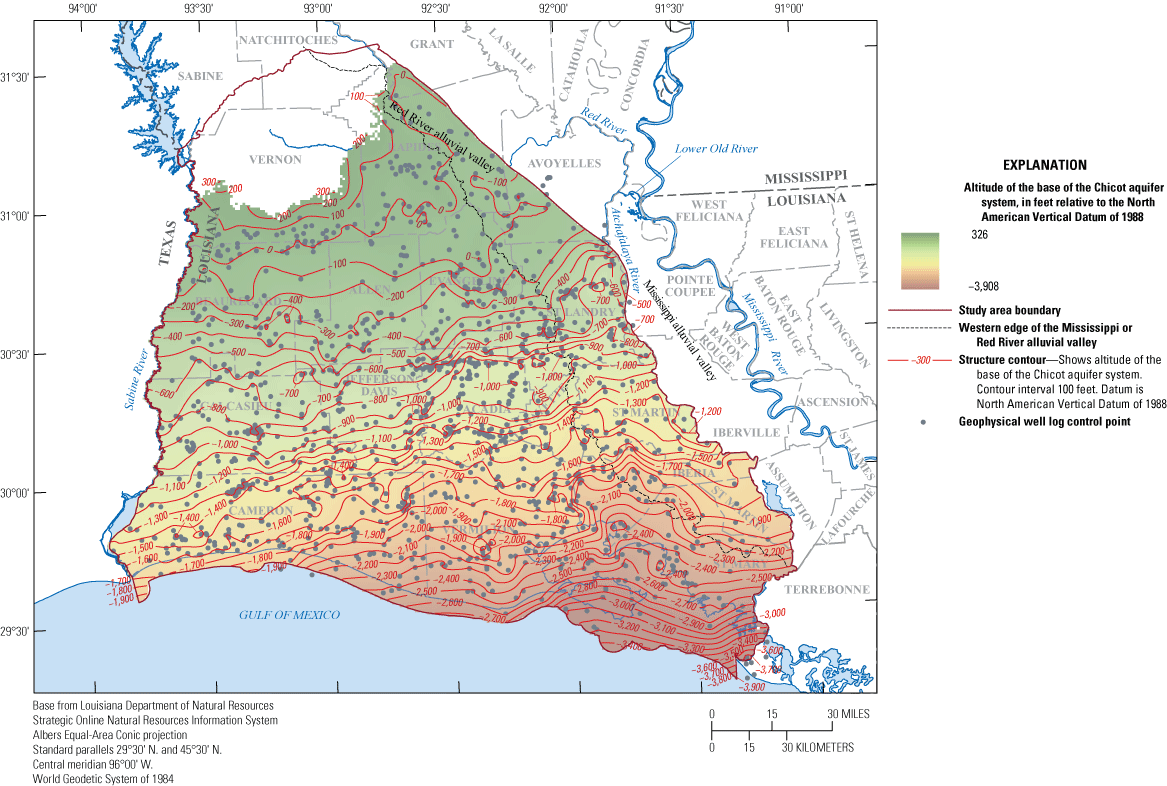
Altitude of the base of the Chicot aquifer system in southwestern Louisiana.
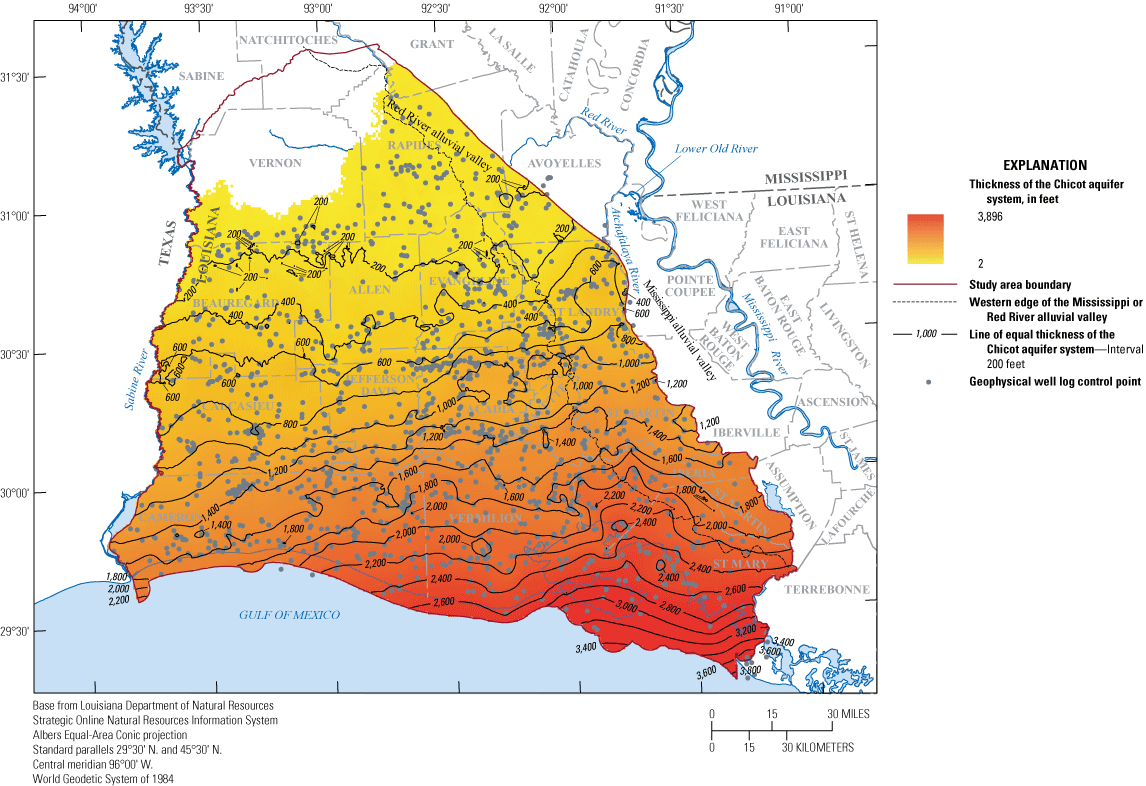
Thickness of the Chicot aquifer system in southwestern Louisiana.
Evangeline Aquifer
The base of the Evangeline aquifer ranges from a maximum of about 284 ft above NAVD 88 near its outcrop area to about 12,308 ft below NAVD 88 in St. Mary Parish before it starts intersecting the top of the zone of overpressure (fig. 13). The Evangeline aquifer crops out in Vernon Parish and subcrops out beneath the Chicot aquifer system or Red River alluvial aquifer elsewhere. These relationships are illustrated in cross section A–Aʹ (fig. 5), where part of the aquifer comes to the surface, and in B–Bʹ (fig. 6), where it is progressively truncated by the overlying Chicot aquifer system.
The Evangeline aquifer is mapped in this report as a thicker unit than in previous studies because of the inclusion of the Castor Creek Member of the Fleming Formation. As shown in this report and others, the Evangeline aquifer is an exceedingly thick subdivision of the total aquifer system and reaches a maximum thickness of about 9,966 ft before it starts intersecting the zone of overpressure in the southeastern corner of the study area (fig. 14). Freshwater is generally absent in the aquifer south of Beauregard, Allen, Evangeline, and St. Landry Parishes (figs. 5–7, 9–10), and consequently a large portion of its total volume within the study area contains saltwater.
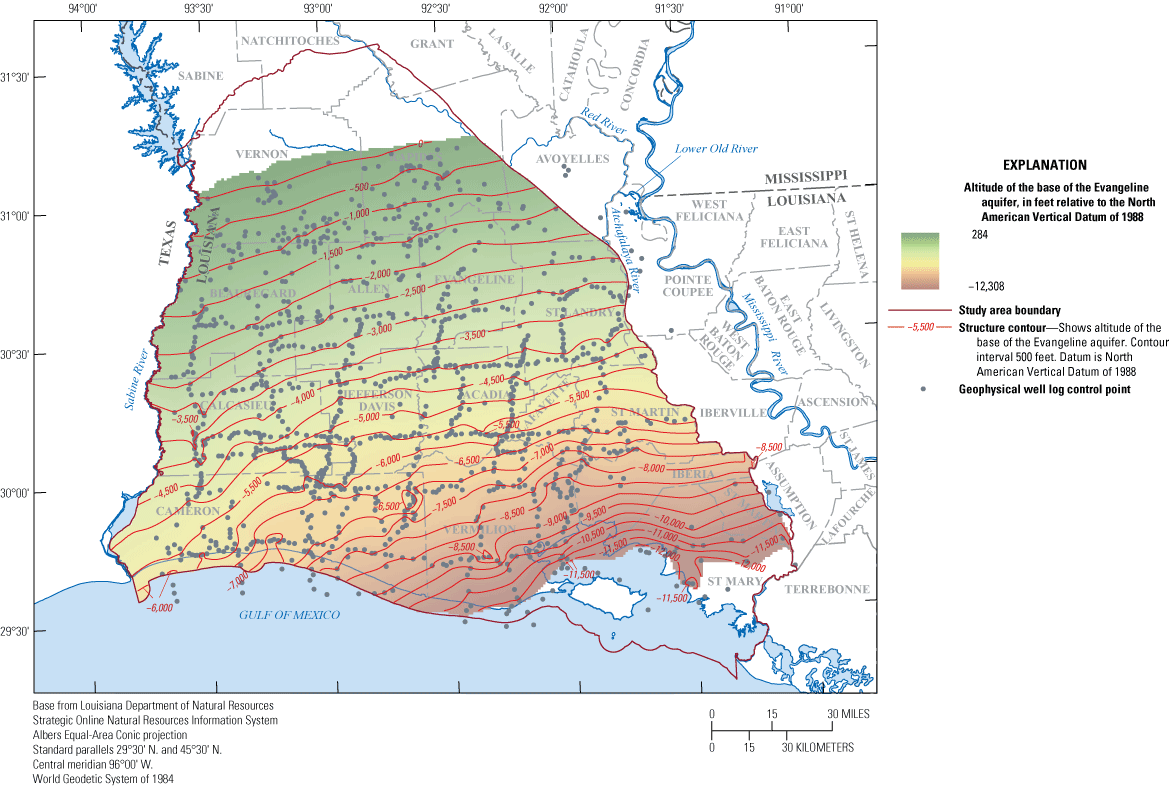
Altitude of the base of the Evangeline aquifer in southwestern Louisiana.
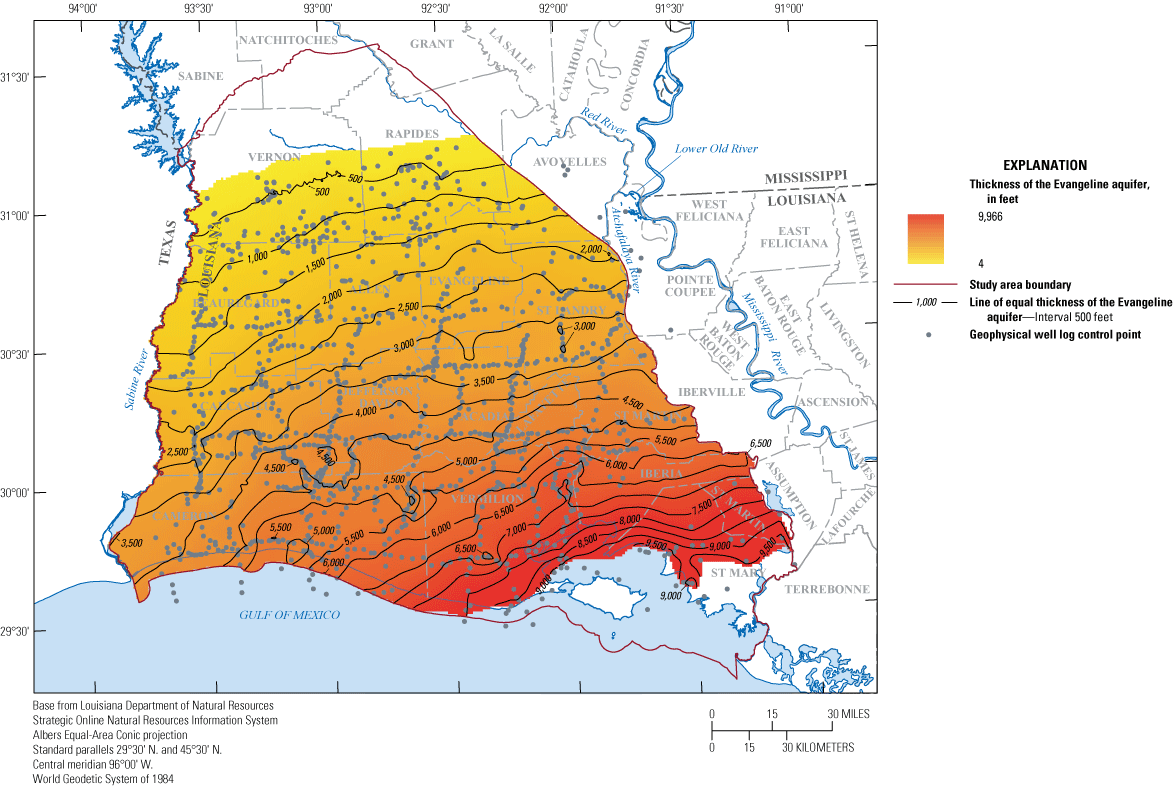
Thickness of the Evangeline aquifer in southwestern Louisiana.
Jasper Aquifer System
The base of the Jasper aquifer system ranges from about 359 ft above NAVD 88 to a maximum of 10,259 ft below NAVD 88 before it starts to intersect the top of the zone of overpressure (fig. 15). Its thickness increases from its outcrop or subcrop area in the north to about 4,286 ft in the southeast before intersecting the zone of overpressure inland from the Gulf of Mexico (fig. 16). The base of the Jasper aquifer system is mapped shallower in this report near the southern border of Rapides and Vernon Parishes in comparison to previous studies that included information on the Jasper aquifer system or the Carnahan Bayou Member, which forms the lower part of the Jasper aquifer system (Rogers and Calandro, 1965; Newcome and Sloss, 1966;Whitfield, 1975). The difference is attributable to the use of the top of the Anahuac Formation as the downdip boundary for the base of the Jasper aquifer system in this report and indicates that the Anahuac Formation may be in part equivalent to the Carnahan Bayou or Dough Hills Members rather than just the Catahoula Formation. The discrepancy decreases towards the outcrop area because of the direct tie to the outcrop limit of the Carnahan Bayou Member. The Jasper aquifer system contains freshwater throughout the northern part of the study area in Vernon and Rapides Parishes (figs. 5–6) but contains saltwater beneath the Mississippi alluvial valley (figs. 7–8).
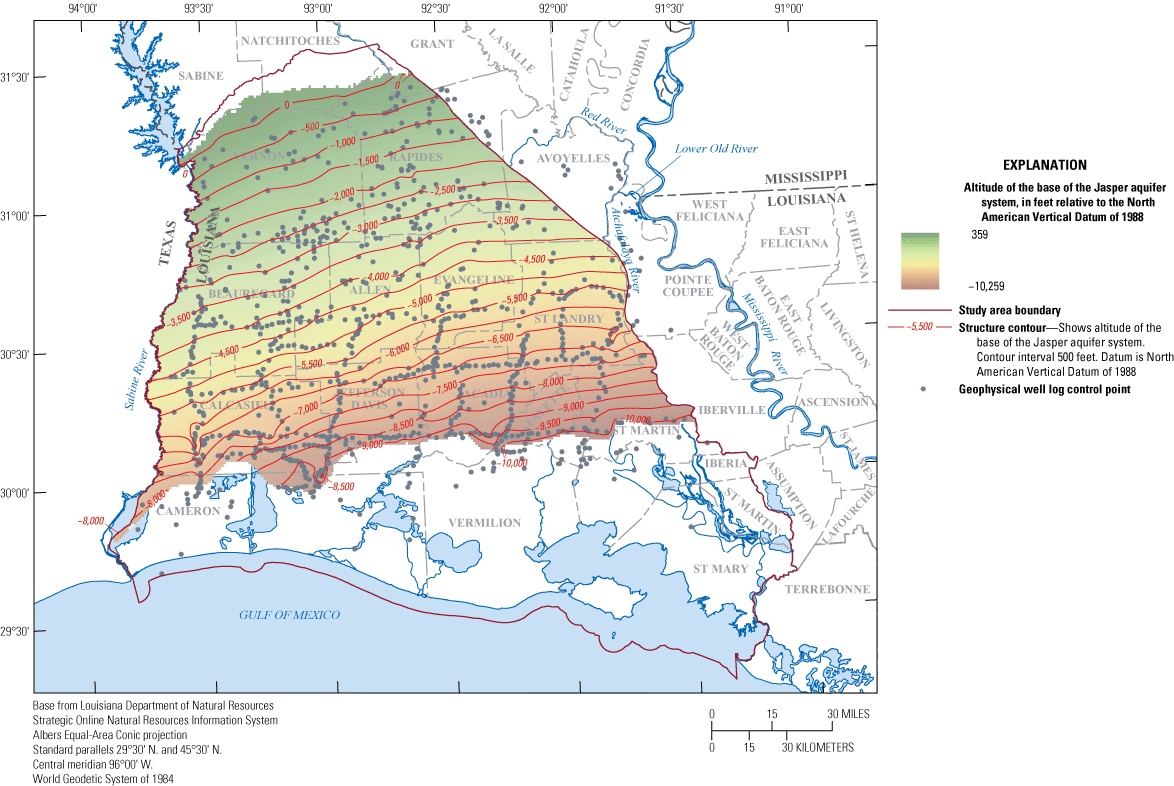
Altitude of the base of the Jasper aquifer system in southwestern Louisiana.
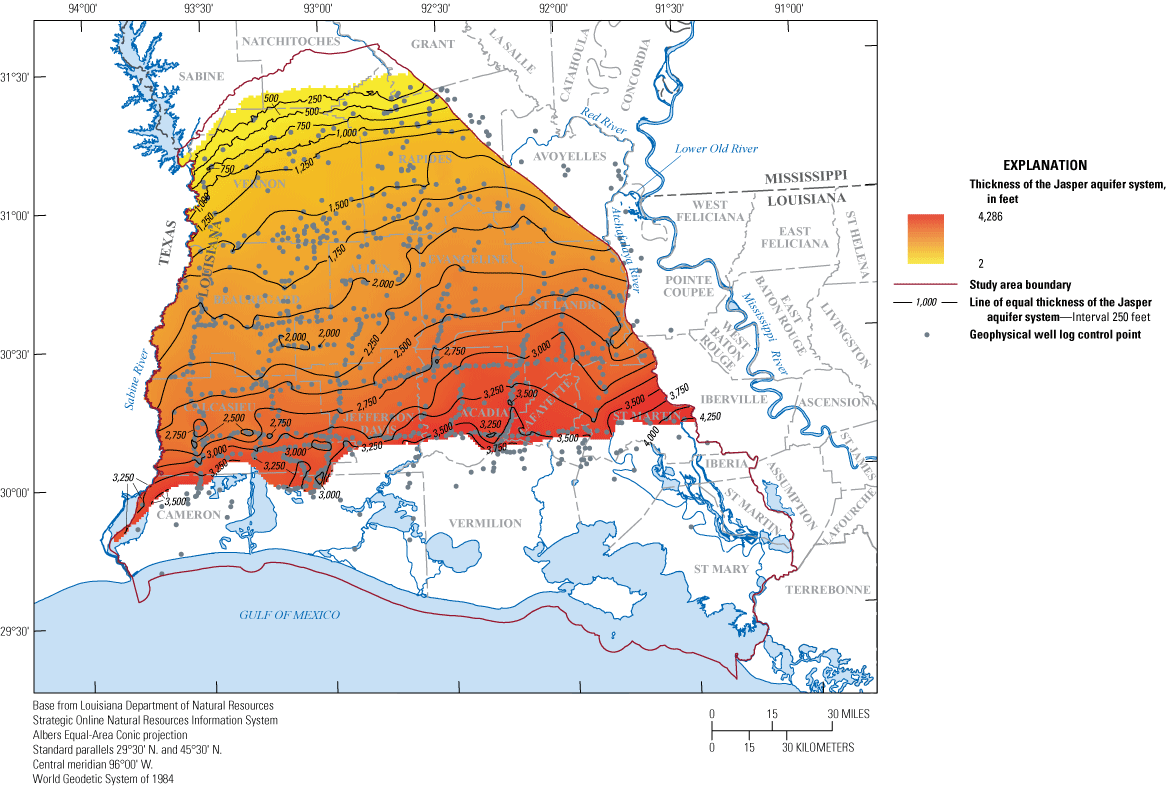
Thickness of the Jasper aquifer system in southwestern Louisiana.
Catahoula Aquifer
The altitude of the base of the Catahoula aquifer ranges from a maximum of about 374 ft above NAVD 88 near its outcrop to about 10,229 ft below NAVD 88 (fig. 17) before it begins to intersect the top of the zone of overpressure, where it reaches a maximum thickness of about 3,307 ft (fig. 18). Within the study area, freshwater in the aquifer is limited to areas in Vernon Parish and in northern Rapides Parish east of the Mississippi alluvial valley (figs. 5–10). Because the top of the Catahoula aquifer was placed at the top of the Anahuac Formation, the Catahoula aquifer also includes an increasingly large percentage of silt and clay towards the south as the Anahuac Formation thickens from its subsurface pinchout.
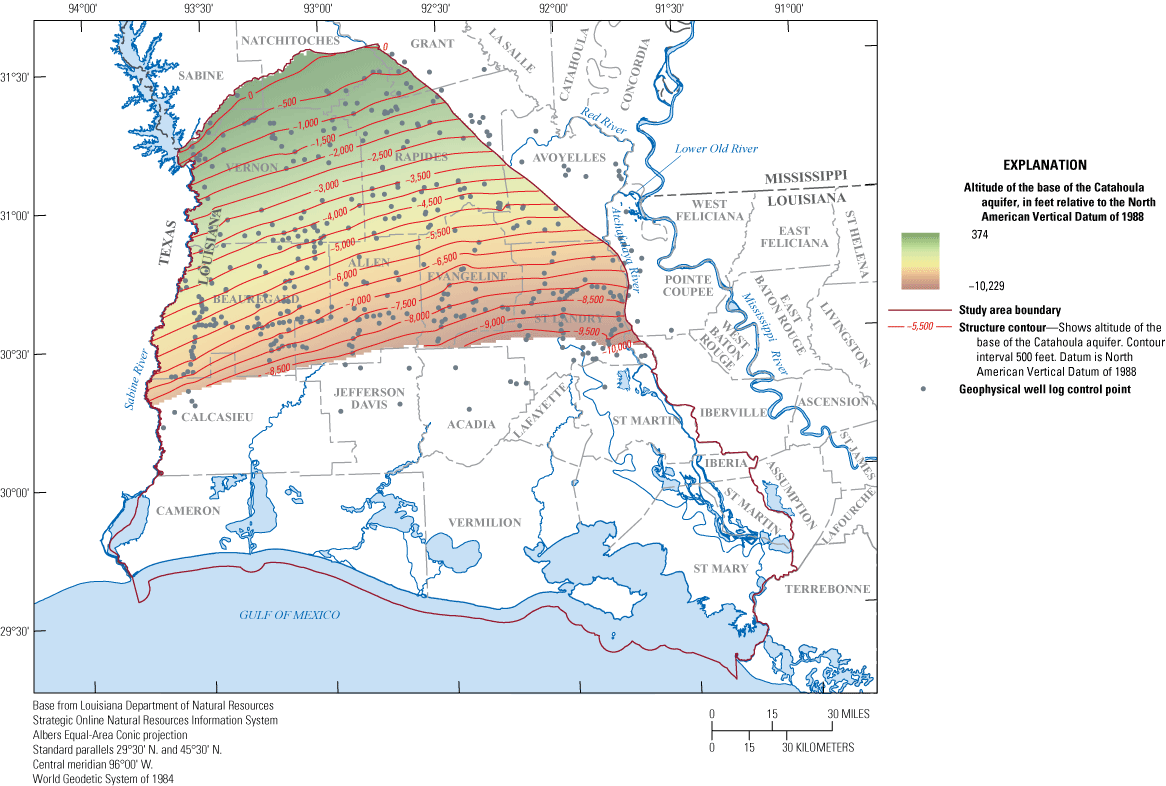
Altitude of the base of the Catahoula aquifer in southwestern Louisiana.
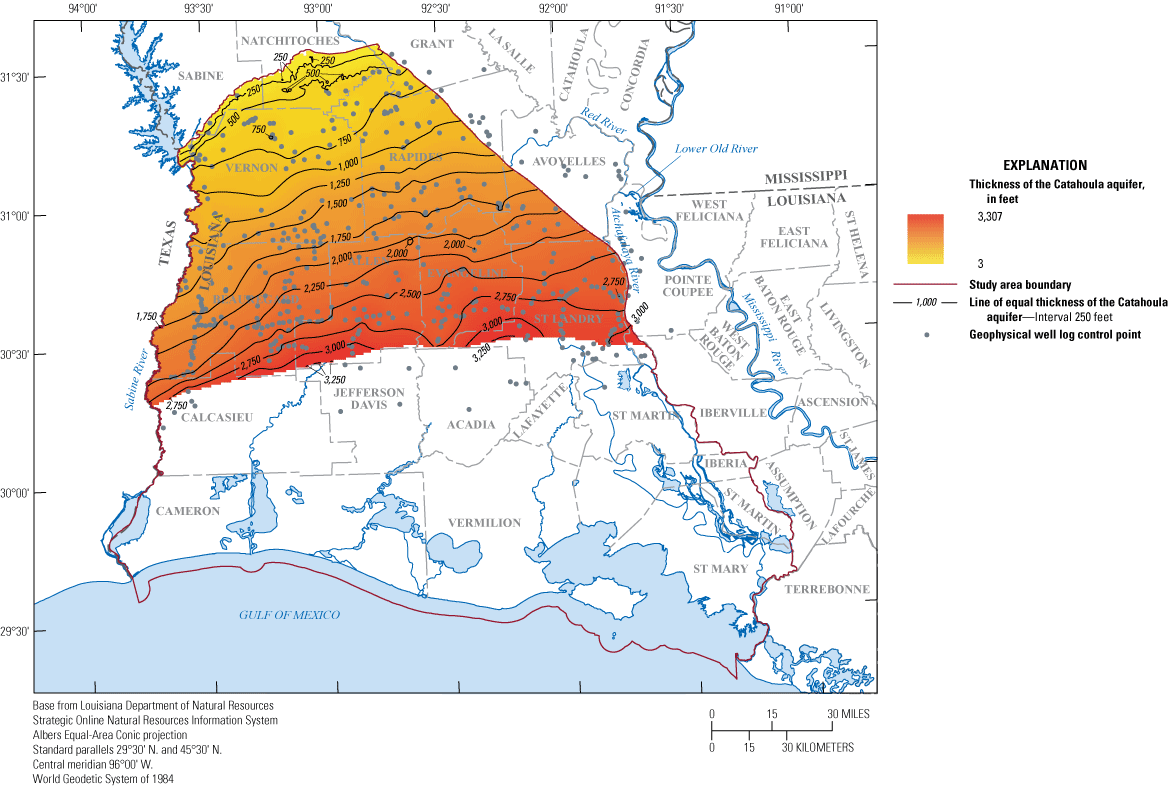
Thickness of the Catahoula aquifer in southwestern Louisiana.
Summary
The Coastal Lowlands aquifer system in southwestern Louisiana comprises a thick wedge-shaped sequence of heterogeneous unconsolidated to semiconsolidated sediments that were deposited in shifting coastal environments over millions of years. The resulting mass of sediments is characterized by complex interbedding, facies changes, and structural deformation that complicates differentiation into a conventional sequence of aquifers and confining units. Through time, locally defined stratigraphic or hydrogeologic units have been grouped together into four broad hydrogeologic units in southwestern Louisiana—the Chicot aquifer system, Evangeline aquifer, Jasper aquifer system, and Catahoula aquifer. Data and interpretations from previous geologic and hydrogeologic publications were synthesized and expanded to map these four units across southwestern Louisiana by using 2,242 geophysical logs. Hydrogeologic unit boundaries in the updip area defined by stratigraphic formation contacts were merged with the upper boundaries of marine shales in the downdip area to better constrain the overall structure of the aquifer system. Raster surfaces were created for the base and thickness of each of the four hydrogeologic units. The interpreted extents of the raster surfaces end where the base of each unit fell below the top of the zone of overpressure in the deep subsurface, which is conceptualized as an impediment to groundwater flow. A table of point data and the derived raster surfaces are available in an accompanying data release and are intended to provide a generalized hydrogeologic framework to support regional groundwater studies.
References Cited
Bureau of Safety and Environmental Enforcement, 2019, BSEE Data Center, accessed October 20, 2017, at https://www.data.bsee.gov/.
Burke, L.A., Kinney, S.A., Russell, D.F., and Pitman, J.K., 2013, Series of five maps characterizing geopressure gradients based on mud weight measurements covering part of southern Louisiana, State and Federal waters: American Association of Petroleum Geologists Spatial Library GIS Open File, 5 p., 5 maps.
Clark, B.R., Barlow, P.M., Peterson, S.M., Hughes, J.D., Reeves, H.W., and Viger, R.J., 2018, National-scale grid to support regional groundwater availability studies and a national hydrogeologic database: U.S. Geological Survey data release, accessed July 7, 2021, at https://doi.org/10.5066/F7P84B24.
Collier, A.L., 2018, Water withdrawals by source and category in Louisiana Parishes, 2014–2015: U.S. Geological Survey data release, accessed October 13, 2020, at https://doi.org/10.5066/F78051VM.
Huffman, A.C., Jr., Kinney, S.A., Biewick, L., Mitchell, H.R., and Gunther, G.L., 2004, Gulf Coast Geology (GCG) online—Miocene of southern Louisiana, version 1.0: U.S. Geological Survey Data Series 90–A, accessed May 18, 2020, at https://doi.org/10.3133/ds90A.
Lindaman, M.A., 2023, Altitudes and thicknesses of hydrogeologic units of southwestern Louisiana: U.S. Geological Survey data release, https://doi.org/10.5066/P9OLQLPP.
Louisiana Department of Natural Resources [Louisiana DNR], 2020, Strategic Online Natural Resources Information System (SONRIS): Louisiana Department of Natural Resources database, accessed from January 2017 through March 2020 at https://www.sonris.com/.
Lovelace, J.K., Fontenot, J.W., and Frederick, C.P., 2004, Withdrawals, water levels, and specific conductance in the Chicot aquifer system in southwestern Louisiana, 2000–03: U.S. Geological Survey Scientific Investigations Report2004–5212, 56 p. [Also available at https://wise.er.usgs.gov/dp/pdfs/SIR_2004-5212.pdf.]
Martin, A., Jr., and Whiteman, C.D., Jr., 1999, Hydrology of the coastal lowlands aquifer system in parts of Alabama, Florida, Louisiana, and Mississippi: U.S. Geological Survey Professional Paper 1416–H, 51 p., 8 pls. [Also available at https://doi.org/10.3133/pp1416H.]
McCulloh, R.P., and Heinrich, P.V., 1999, Geology of the Fort Polk region, Sabine, Natchitoches, and Vernon Parishes, Louisiana: Louisiana State University, Geological Survey, Final report prepared for the U.S. Army Corps of Engineers, Fort Worth district, under contract no. DACA63-95-D-0051, delivery order no. 0008, 119 p., plus appendixes.
National Oceanic and Atmospheric Administration, National Ocean Service, Office for Coastal Management, 2014, Topobathymetric model of the northern Gulf of Mexico, 1888 to 2013, accessed December 12, 2020, at https://coast.noaa.gov/dataviewer/#/.
Nyman, D.J., 1984, The occurrence of high concentrations of chloride in the Chicot aquifer system of southeastern Louisiana: Louisiana Department of Transportation and Development Water Resources Technical Report no. 33, 75 p. [Also available at https://wise.er.usgs.gov/dp/pdfs/TR33.pdf.]
Nyman, D.J., Halford, K.J., and Martin, A., Jr., 1990, Geohydrology and simulation of flow in the Chicot aquifer system of southwestern Louisiana: Louisiana Department of Transportation and Development Water Resources Technical Report no. 50, 58 p. [Also available at https://wise.er.usgs.gov/dp/pdfs/TR50.pdf.]
Prakken, L.B., and Griffith, J.M., 2000, Analysis of geophysical log data from the Fort Polk Military Reservation, Vernon Parish, Louisiana: U.S. Geological Survey Water-Resources Investigations Report 00–4111, 50 p. [Also available at: https://wise.er.usgs.gov/dp/pdfs/WRI_00-4111.pdf.]
Sargent, B.P., Frederick, C.P., and Roberts, F.C., 2004, Thickness data for the Chicot aquifer system surficial confining unit: Louisiana Department of Transportation and Development Water Resources Basic Records Report no. 22, 91 p. [Also available at https://wise.er.usgs.gov/dp/pdfs/BR22.pdf.]
Schiebout, J.A., Ting, S., Williams, M., Boardman, G., Gose, W., Wilhite, R., White, P.D., and Kilbourne, B., 2004, Paleofaunal and environmental research on Miocene fossil sites TVOR SE and TVOR S on Fort Polk, Louisiana, with continued survey, collection, processing, and documentation of other Miocene localities: U.S. Army Corps of Engineers, Fort Worth District, contract no. DACA63-00-D-006, delivery order no. 0015, 46 p.
Smoot, C.W., 1988, Louisiana hydrologic atlas map no. 3—Altitude of the base of freshwater in Louisiana: U.S. Geological Survey Water-Resources Investigations Report 86–4184, 1 sheet. [Also available at https://doi.org/10.3133/wri864314.]
Smoot, C.W., 1989, Louisiana hydrologic atlas map no. 4—Geohydrologic sections of Louisiana: U.S. Geological Survey Water-Resources Investigations Report 87–4288, 1 sheet. [Also available at https://doi.org/10.3133/wri874288.]
U.S. Geological Survey, 2013, National Elevation Dataset, 1/3 arc-second resolution, accessed April 12, 2018, at https://viewer.nationalmap.gov/basic/.
Weiss, J.S., 1992, Geohydrologic units of the Coastal Lowlands aquifer system, south-central United States: U.S. Geological Survey Professional Paper1416–C, 32 p. [Also available at https://doi.org/10.3133/pp1416C.]
Williamson, A.K., and Grubb, H.F., 2001, Ground-water flow in the Gulf Coast aquifer systems, south-central United States: U.S. Geological Survey Professional Paper 1416–F, 173 p. [Also available at https://doi.org/10.3133/pp1416F.]
Wilson, T.A., and Hosman, R.L., 1988, Geophysical well-log data base for the Gulf Coast aquifer systems, south-central United States: U.S. Geological Survey Open-File Report 87–677, 213 p. [Also available at https://doi.org/10.3133/ofr87677.]
Conversion Factors
U.S. customary units to International System of Units
Datum
Vertical coordinate information is referenced to the North American Vertical Datum of 1988 (NAVD 88).
Horizontal coordinate information is referenced to the World Geodetic System of 1984 (WGS 84).
Altitude, as used in this report, refers to distance above or below the vertical datum.
For more information about this publication, contact
Director, Lower Mississippi-Gulf Water Science Center
U.S. Geological Survey
640 Grassmere Park, Suite 100
Nashville, TN 37211
For additional information, visit
https://www.usgs.gov/centers/lmg-water/
Publishing support provided by
Lafayette Publishing Service Center
Disclaimers
Any use of trade, firm, or product names is for descriptive purposes only and does not imply endorsement by the U.S. Government.
Although this information product, for the most part, is in the public domain, it also may contain copyrighted materials as noted in the text. Permission to reproduce copyrighted items must be secured from the copyright owner.
Suggested Citation
Lindaman, M.A., 2023, Hydrogeologic framework of southwestern Louisiana: U.S. Geological Survey Scientific Investigations Report 2023–5004, 31 p., https://doi.org/10.3133/sir20235004.
ISSN: 2328-0328 (online)
Study Area
Publication type | Report |
---|---|
Publication Subtype | USGS Numbered Series |
Title | Hydrogeologic framework of southwestern Louisiana |
Series title | Scientific Investigations Report |
Series number | 2023-5004 |
DOI | 10.3133/sir20235004 |
Year Published | 2023 |
Language | English |
Publisher | U.S. Geological Survey |
Publisher location | Reston, VA |
Contributing office(s) | Lower Mississippi-Gulf Water Science Center |
Description | Report: viii, 31 p.; Data Release |
Country | United States |
State | Louisiana |
Online Only (Y/N) | Y |
Google Analytic Metrics | Metrics page |