Status of Water-Level Altitudes and Long-Term and Short-Term Water-Level Changes in the Chicot and Evangeline (Undifferentiated) and Jasper Aquifers, Greater Houston Area, Texas, 2022
Links
- Document: Report (13.5 MB pdf) , HTML , XML
- Dataset: USGS National Water Information System database —USGS water data for the Nation
- Data Releases:
- USGS data release —Depth to groundwater measured from wells completed in the Chicot and Evangeline (undifferentiated) and Jasper aquifers, greater Houston area, Texas, 2022
- USGS data release —Groundwater-level altitudes and long-term groundwater-level changes in the Chicot and Evangeline (undifferentiated) and Jasper aquifers, greater Houston area, Texas, 2022
- Download citation as: RIS | Dublin Core
Abstract
Since the early 1900s, groundwater withdrawn from the primary aquifers that compose the Gulf Coast aquifer system—the Chicot, Evangeline, and Jasper aquifers—has been an important source of water in the greater Houston area, Texas. This report, prepared by the U.S. Geological Survey in cooperation with the Harris-Galveston Subsidence District, City of Houston, Fort Bend Subsidence District, Lone Star Groundwater Conservation District, and Brazoria County Groundwater Conservation District, is one in an annual series of reports depicting the status of water-level altitudes and water-level changes in these aquifers in the greater Houston area.
In this report, the Chicot and Evangeline aquifers are treated as a single hydrogeologic unit for the purposes of providing annual assessments of regional-scale water-level altitudes and changes over time. In 2022, shaded depictions of water-level altitudes for the Chicot and Evangeline aquifers (undifferentiated) ranged from about 270 feet (ft) below the North American Vertical Datum of 1988 (NAVD 88) to about 195 ft above NAVD 88. The largest decline in water-level altitudes indicated by the 1977–2022 long-term water-level-change map was southeast of The Woodlands. In comparison, the 1990–2022 long-term water-level-change map depicts declines in water-level altitudes in localized areas at or near certain wells in parts of northwestern Harris County and southern Montgomery County. The largest rise in water-level altitudes for 1977–2022 was observed in a relatively large area in southeastern Harris County, whereas the largest rise in water-level altitudes for 1990–2022 was observed in a relatively small area in central Harris County. The 5-year short-term water-level-change map depicts the largest declines at three wells in northern Fort Bend County and the largest rises at five wells in southwestern and central Harris County. The 1-year short-term water-level-change map depicts the largest decline at a well in north-central Harris County and the largest rises at a well in north-central Fort Bend County and a well in southwestern Harris County.
In 2022, shaded depictions of water-level altitudes for the Jasper aquifer ranged from about 213 ft below NAVD 88 to about 285 ft above NAVD 88. The 2000–22 long-term water-level-change map depicts water-level declines throughout most of the study area where water-level-measurement data from the aquifer were collected, with the largest decline in north-central Harris County and south-central Montgomery County south of The Woodlands. The 5-year short-term water-level-change map depicts the largest decline at a well in Conroe and the largest rise at a well in south-central Montgomery County. The 1-year short-term water-level-change map depicts the largest decline at a well in south-central Montgomery County east of The Woodlands and the largest rise at a well in Conroe.
Introduction
The greater Houston area in Texas as defined in this report includes approximately 11,000 square miles and encompasses all or part of 11 counties (Harris, Galveston, Fort Bend, Montgomery, Brazoria, Chambers, Grimes, Liberty, San Jacinto, Walker, and Waller). Since the early 1900s, an important source of groundwater withdrawals in the study area has been from three of the hydrogeologic units that compose the Gulf Coast aquifer system—the Chicot, Evangeline, and Jasper aquifers; more recently, groundwater withdrawals have also been from the Catahoula confining unit (fig. 1) (Kasmarek and Robinson, 2004; Ellis and others, 2023). Withdrawals from these hydrogeologic units are used for municipal supply, commercial and industrial use, and irrigation purposes (Kasmarek and Robinson, 2004). Early development of the Gulf Coast aquifer system, which began before 1900 in the greater Houston area, resulted in rapid declines in groundwater levels in some areas; by 1946, as much as 50 percent of the eventual groundwater-level minimums had already been measured in many wells in the area, and by 1977, these withdrawals led to declines in water levels resulting in water-level altitudes of more than 300 feet (ft) below the North American Vertical Datum of 1988 (NAVD 88) in the Chicot and Evangeline aquifers in southeastern Harris County (Gabrysch, 1979). These groundwater-level declines also led to as much as 10 ft of land-surface subsidence in some parts of the greater Houston area—historically in central and southeastern Harris County and in Galveston County but more recently in the northern and western parts of the greater Houston area—from depressurization and compaction of clay and silt layers interbedded in the aquifer sediments (Winslow and Doyel, 1954; Winslow and Wood, 1959; Gabrysch and Bonnet, 1975; Gabrysch, 1984; Holzer and Bluntzer, 1984; Coplin and Galloway, 1999; Kasmarek, Gabrysch, and Johnson, 2009; Ellis and others, 2023).
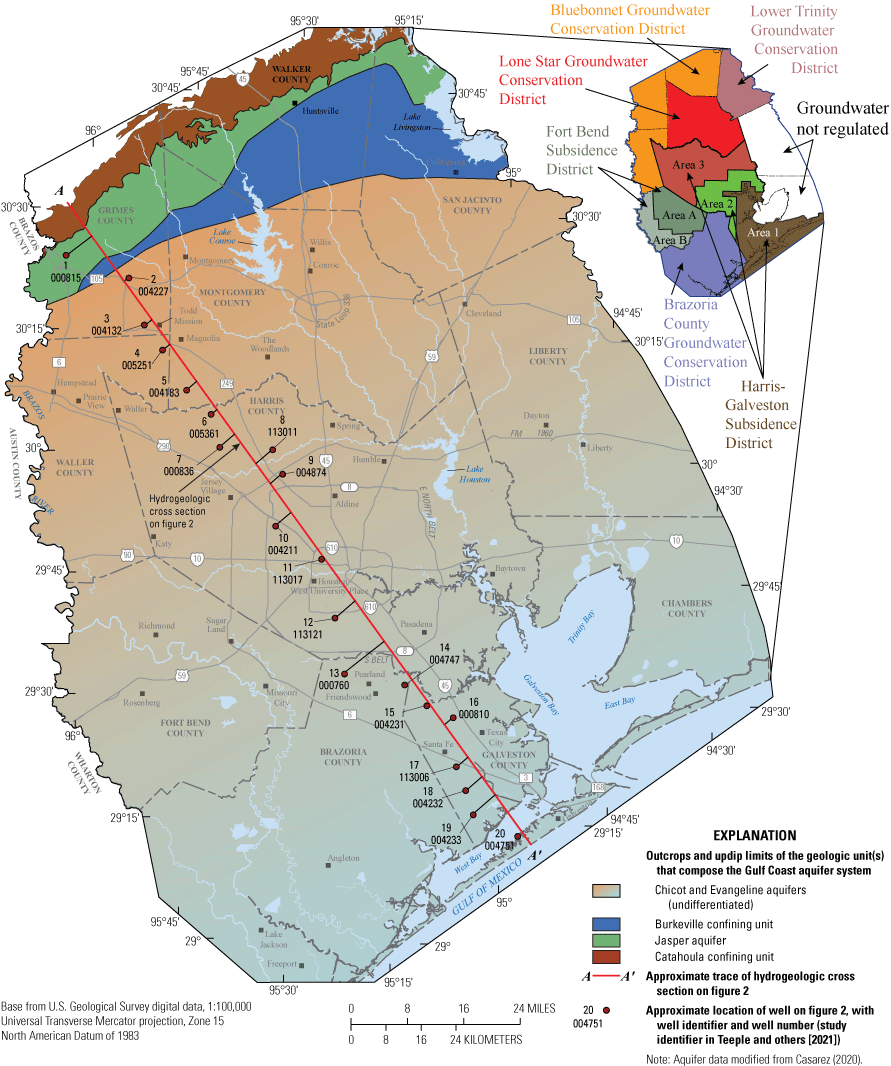
Locations of groundwater regulatory districts; approximate trace of hydrogeologic cross section A–A´; and outcrops and updip limits of the hydrogeologic units that compose the Gulf Coast aquifer system in the greater Houston study area, Texas.
To regulate and reduce groundwater withdrawals in Harris and Galveston Counties, the 64th Texas State Legislature authorized the establishment of the Harris-Galveston Subsidence District (HGSD) in 1975 (Harris-Galveston Subsidence District, 2021). After establishing the HGSD, the Texas State Legislature established an additional subsidence district (Fort Bend Subsidence District [FBSD]) and four groundwater conservation districts (Lone Star Groundwater Conservation District [LSGCD], Bluebonnet Groundwater Conservation District, Brazoria County Groundwater Conservation District [BCGCD], and Lower Trinity Groundwater Conservation District) in the greater Houston area (fig. 1) to enable the regulation of groundwater withdrawals within their respective jurisdictions. The FBSD was established by the 71st Texas State Legislature in 1989 (Fort Bend Subsidence District, 2021), the LSGCD and Bluebonnet Groundwater Conservation District were established by the 77th Texas State Legislature in 2001 (Bluebonnet Groundwater Conservation District, 2021; Lone Star Groundwater Conservation District, 2021), the BCGCD was established by the 78th Texas State Legislature in 2003 (Brazoria County Groundwater Conservation District, 2021), and the Lower Trinity Groundwater Conservation District was established by the 79th Texas State Legislature in 2005 (Lower Trinity Groundwater Conservation District, 2021). Regulatory plans to gradually decrease groundwater withdrawals (in conjunction with increased use of alternative surface-water supplies) are currently (2022) being phased in throughout most of the study area (fig. 1). The current groundwater management plans of each district are available on their respective websites (Fort Bend Subsidence District, 2013; Harris-Galveston Subsidence District, 2013; Brazoria County Groundwater Conservation District, 2017; Bluebonnet Groundwater Conservation District, 2018; Lower Trinity Groundwater Conservation District, 2019; Lone Star Groundwater Conservation District, 2020). Groundwater withdrawals are currently (2022) not regulated by a groundwater conservation district in two counties in the greater Houston area: Liberty and Chambers Counties (fig. 1).
Since the 1970s, the U.S. Geological Survey (USGS), in cooperation with the HGSD, has monitored water-level altitudes and published reports on the status of water-level altitudes and long-term water-level changes in the greater Houston area; following their establishment by the Texas State Legislature, the FBSD, LSGCD, and BCGCD became cooperative participants in the study as well. An extensive network of wells was established by the USGS in 1977, which led to the first in an annual series of published water-level-altitude maps of the Chicot and Evangeline aquifers in 1979 for the greater Houston area (Gabrysch, 1979). A comprehensive report for the Chicot and Evangeline aquifers depicting 1990 water-level altitudes in each aquifer unit was first published by the USGS in 1991 (Barbie and others, 1991). The USGS also first published a map of the Jasper aquifer water-level altitudes in 2000 for the greater Houston area (Coplin, 2001). Additional information on the history of water-level-altitude monitoring and of the USGS reports published to document water-level altitudes and water-level changes in the greater Houston area is provided in Ellis and others (2023).
The decision to treat the Chicot and Evangeline aquifers as a single hydrogeologic unit in the greater Houston area when providing water-level altitudes and water-level changes was made starting with the 2021 annual report (Braun and Ramage, 2022) and continues in this report. The reasoning and nature of the decision are described in detail in Ramage and others (2022). In brief, the rationale for not differentiating the Chicot aquifer from the Evangeline aquifer includes the absence of a confining unit between the two aquifers. Previous investigators have noted that the absence of a confining unit between the Chicot and Evangeline aquifers implies that the two aquifers are hydraulically connected, allowing groundwater to flow between them (Liu and others, 2019). Because of this hydraulic connection, water-level changes that occur in one aquifer can affect groundwater levels in the adjoining aquifer (Kasmarek and others, 2010). Additionally, previous investigators have noted similarity in geochemical signatures between the Chicot and Evangeline aquifers (Borrok and Broussard, 2016), as well as in hydraulic properties (Meyer and Carr, 1979; Carr and others, 1985). The decision was also made in response to the findings from Young and others (2017) and Young and Draper (2020), which led to the reclassification of the hydrogeologic units for a large proportion of the wells in the groundwater-monitoring network used to collect long-term groundwater levels for the annual series of reports. When considered together, the various lines of evidence that suggest a hydraulic connection between the Chicot and Evangeline aquifers provide sufficient justification for treating the Chicot and Evangeline aquifers as a single hydrogeologic unit for the purposes of providing annual assessments of regional-scale water-level altitudes and changes over time.
This report, prepared by the USGS in cooperation with the HGSD, City of Houston, FBSD, LSGCD, and BCGCD, is one in an annual series of reports depicting the status of water-level altitudes and water-level changes in the Chicot and Evangeline (undifferentiated) and Jasper aquifers in the greater Houston area.
Purpose and Scope
The purpose of this report, prepared by the USGS in cooperation with the HGSD, City of Houston, FBSD, LSGCD, and BCGCD, is to depict water-level altitudes and long-term and short-term water-level changes in the Chicot and Evangeline (undifferentiated) and Jasper aquifers in the greater Houston area, Texas. An overview of the hydrogeology of the study area is provided, and regional-scale maps depicting approximate water-level altitudes for 2022 (represented by measurements made during November 2021 through March 2022) (Ramage and Adams, 2023a), long-term water-level changes in the Chicot and Evangeline aquifers (undifferentiated) (1977–2022 and 1990–2022) and the Jasper aquifer (2000–22) (Ramage and Adams, 2023b), and short-term water-level changes for the Chicot and Evangeline (undifferentiated) and Jasper aquifers (2017–22 and 2021–22) (Ramage and Adams, 2023b) are featured. Except where noted, the text and figures in this report were modified from the previous report in this annual series of reports (Braun and Ramage, 2022).
Hydrogeology of the Study Area
The following overview of the hydrogeology of the study area is summarized from Kasmarek and Ramage (2017). From land surface down, the hydrogeologic units in the study area include the Chicot and Evangeline aquifers (undifferentiated), Burkeville confining unit, Jasper aquifer, and Catahoula confining unit (figs. 2 and 3). The three primary aquifers in the Gulf Coast aquifer system in the study area (the Chicot, Evangeline, and Jasper aquifers) are composed of laterally discontinuous deposits of gravel, sand, silt, and clay (Baker, 1979). The percentage of clay and other fine-grained, clastic material generally increases downdip (Baker, 1979). The two uppermost of the three aquifers, the Chicot and Evangeline aquifers (undifferentiated), are contained in Holocene- and Pleistocene-age (Quaternary-age) sediments and Pliocene- and Miocene-age (Tertiary-age) sediments (Baker, 1979, 1986; Young and others, 2012, 2014; Casarez, 2020; Young and Draper, 2020; Teeple and others, 2021) (figs. 2 and 3). The more deeply buried Jasper aquifer is contained in Miocene-age sediments (Baker, 1979, 1986; Young and others, 2012, 2014; Casarez, 2020; Young and Draper, 2020; Teeple and others, 2021) (figs. 2 and 3). The stratigraphic relations between these hydrogeologic units are shown on hydrogeologic cross section A–A´ (figs. 1 and 2) of the Gulf Coast aquifer system, which extends through the greater Houston area from northwestern Grimes County southeastward through Waller, Montgomery, Harris, and Brazoria Counties before terminating at the Gulf of Mexico coast in Galveston County.
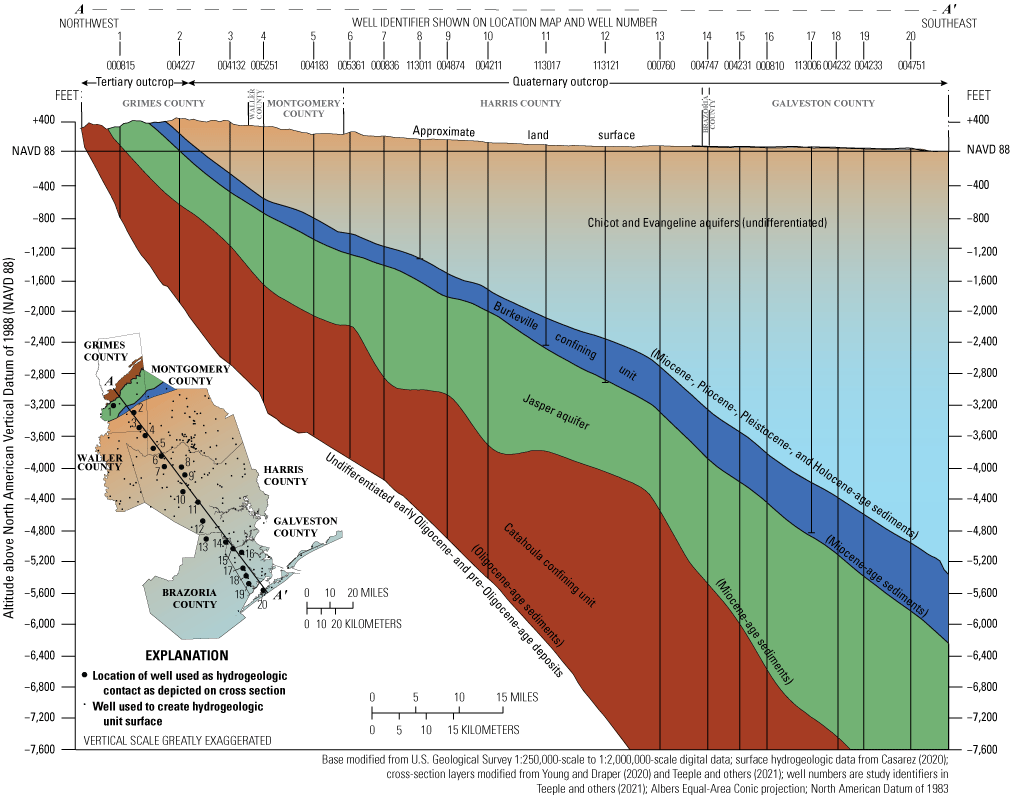
Hydrogeologic cross section A–A´ of the Gulf Coast aquifer system in Grimes, Waller, Montgomery, Harris, Brazoria, and Galveston Counties, Texas.
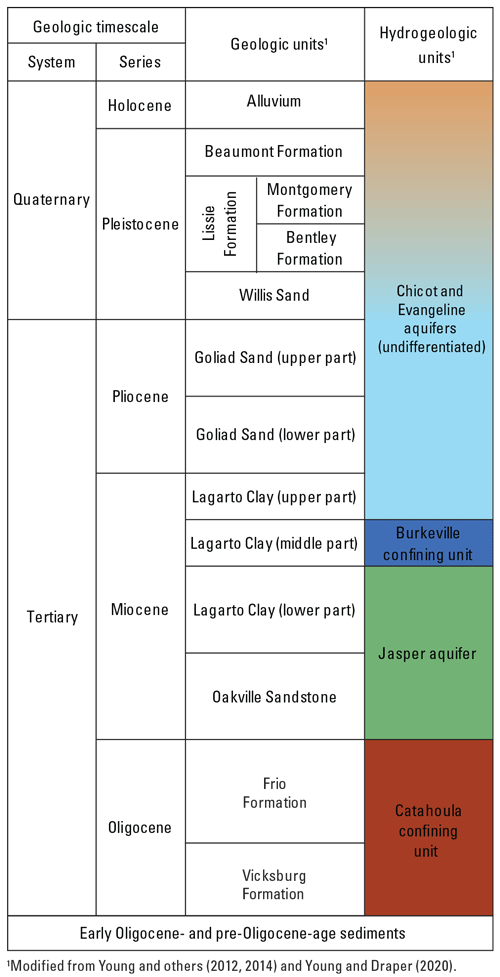
Depiction of the geologic and hydrogeologic units of the Gulf Coast aquifer system in the greater Houston study area, Texas.
Recent updates to the hydrogeologic framework are detailed in Ramage and others (2022), which summarized the work of Young and others (2012, 2014) and Young and Draper (2020). Young and Draper (2020) updated the Gulf Coast aquifer system geologic stratigraphy and hydrogeologic unit thicknesses depicted for the study area (figs. 2 and 3) by using a coupled chronostratigraphic and lithostratigraphic approach. The coupled chronostratigraphic and lithostratigraphic approach used by Young and Draper (2020) was based on a hydrogeologic framework proposed by Young and others (2014), which in turn was based on earlier work by Young and others (2012). Young and Draper (2020) subdivided the Lagarto Clay into upper, middle, and lower parts; in their revised hydrogeologic framework, the entirety of the upper part of the Lagarto Clay was included in the sediments that contain the Evangeline aquifer. The lower part of the Lagarto Clay was also included in the revised definition of the sediments that contain the Jasper aquifer, similar to Turcan and others (1966). Additionally, the Goliad Sand was subdivided into upper and lower parts following Young and others (2012).
Through time, geologic and hydrologic processes created accretionary sediment wedges (stacked sequences of sediments) more than 7,600 ft thick at the coast (fig. 2) (Chowdhury and Turco, 2006). These sediments, which compose the Gulf Coast aquifer system, were deposited by fluvial-deltaic processes and subsequently were eroded and redeposited by worldwide episodic changes in sea level that occurred as a result of oscillations between glacial and interglacial climate conditions (Lambeck and others, 2002). The Gulf Coast aquifer system consists of hydrogeologic units that dip and thicken from northwest to southeast; the hydrogeologic units representing the aquifers and confining units thus crop out in bands inland from, and approximately parallel to, the coast and become progressively more deeply buried and confined toward the coast (figs. 1 and 2) (Kasmarek, 2012). The aquifers receive recharge where the hydrogeologic units composing the aquifers crop out (Kasmarek and Robinson, 2004) (figs. 1 and 2). The Burkeville confining unit is stratigraphically positioned between the Chicot and Evangeline aquifers (undifferentiated) and the Jasper aquifer (fig. 2), thereby restricting groundwater flow between the Chicot and Evangeline aquifers (undifferentiated) and the Jasper aquifer. Supporting evidence of the interaction of groundwater flow between the Chicot and Evangeline aquifers includes the similarities between long-term water-level-change maps for each aquifer (for example, figs. 5 and 7 in Braun and others [2019] and figs. 5 and 7 in Braun and Ramage [2020]), which indicate that the areas where groundwater levels have risen or declined are approximately spatially coincident. Additional evidence of the hydraulic connection between the Chicot and Evangeline aquifers was provided by Borrok and Broussard (2016, p. 330); their geochemical evaluation of the Chicot aquifer system from 1993 to 2015 in Louisiana indicated that in some years (1998, 2008, and 2011) certain wells screened in the Chicot aquifer “appeared to be tapping water with a geochemistry (temperature, salinity, alkalinity, [and so forth]) matching the underlying Evangeline aquifer.”
Hydraulic properties of the Chicot aquifer do not differ appreciably from those of the hydrogeologically similar Evangeline aquifer (Carr and others, 1985, p. 10). From aquifer-test data, Meyer and Carr (1979) estimated that the transmissivity of the Chicot aquifer ranges from 3,000 to 25,000 square feet per day (ft2/d) and that the transmissivity of the Evangeline aquifer ranges from 3,000 to 15,000 ft2/d. Proceeding updip and inland from the Quaternary- and Tertiary-age sediments containing the Chicot and Evangeline aquifers (undifferentiated), the older geologic units (containing the Burkeville confining unit, the Jasper aquifer, and the Catahoula confining unit) sequentially crop out (figs. 1 and 2). Additionally, in the downdip parts of the aquifer system, the Jasper aquifer can be differentiated from the overlying Chicot and Evangeline aquifers (undifferentiated) on the basis of stratigraphic position relative to the altitude of the Burkeville confining unit (figs. 2 and 3).
Precipitation falling on the land surface overlying these aquifers returns to the atmosphere as evapotranspiration, becomes surface runoff until it reaches streams, or infiltrates as groundwater recharge to the unconfined updip sediments composing the aquifers (Williamson and others, 1990; Healy, 2010). The infiltrating water moves downgradient toward the coast, reaching the intermediate and deep zones of the aquifers southeastward of the outcrop areas, where it can be withdrawn and discharged by wells or is naturally discharged by diffuse upward leakage in topographically low areas near the coast (Kasmarek and Robinson, 2004). Water in the coastal, deep zones of the aquifers is denser, and this higher density water causes the fresher, lower density water that has not been captured and withdrawn by wells to be redirected as diffuse upward leakage to shallow zones from the confined downdip areas of the aquifer system (Kasmarek and Robinson, 2004).
Previous Studies
From the early 1900s until about 1930, periodic measurements of groundwater levels were documented in the greater Houston area. Groundwater-level data became more widely available beginning in December 1930 when systematic USGS groundwater measurement surveys began in cooperation with the Texas State Board of Water Engineers (predecessor to the Texas Water Commission) and the City of Houston (White and others, 1939, 1944). From these data, the first water-level-altitude maps of the Chicot and Evangeline aquifers in 1928 and 1931 in the Houston area were published (figs. 1 and 2 in White and others, 1939). USGS groundwater measurements have continued since 1930 as part of a groundwater-level monitoring network of wells in the Houston area. Gabrysch (1967, 1972, 1980) published water-level-altitude maps of the Chicot and Evangeline aquifers for 1957, 1966, 1970, and 1975. As described in the “Introduction” section of this report, the USGS established an extensive network of wells in 1977, and groundwater-level data collected during springs of 1977 and 1978 were used to construct water-level-altitude maps of the Chicot and Evangeline aquifers in the greater Houston area, the first to be published in an annual series, in 1979 (Gabrysch, 1979). In cooperation with the FBSD, which adopted its groundwater management plan in 1990 (Fort Bend Subsidence District, 2013), an increased number of wells were inventoried by the USGS in Fort Bend, Harris, Brazoria, and Waller Counties in 1989 and 1990. A more comprehensive water-level-altitude report for the Chicot and Evangeline aquifers depicting water-level altitudes for 1990 was published by the USGS in 1991 (Barbie and others, 1991) and was revised in 1997 when updated well data became available (Kasmarek, 1997). Similarly, after the establishment of the LSGCD in 2001, the USGS began publishing water-level-altitude maps of the Jasper aquifer in the greater Houston area (primarily Montgomery County) (Coplin, 2001). In 2004, 2006, and 2007, as additional wells with reliable water-level data were inventoried, revised water-level-altitude maps for the Jasper aquifer were published (Kasmarek and Lanning-Rush, 2004; Kasmarek and others, 2006; Kasmarek and Houston, 2007). Since 2007, comprehensive maps for the Jasper aquifer have been included in the annual series of reports that depict water-level altitudes and water-level changes in the greater Houston area (Kasmarek and Houston, 2007, 2008; Kasmarek, Houston, and Ramage, 2009; Kasmarek and others, 2010, 2012, 2013, 2014, 2015, 2016; Johnson and others, 2011; Kasmarek and Ramage, 2017; Shah and others, 2018; Braun and others, 2019; Braun and Ramage, 2020, 2022).
Methods
This section describes the methods used to (1) collect and process water-level data used in this report; (2) apply kriging processes used to depict water-level altitudes and water-level changes, which are described briefly herein and in detail in Ramage and others (2022); (3) determine water-level altitudes; and (4) depict long-term and short-term water-level changes in the study area. The data collection methods are similar to those described in previous reports by Gabrysch (1979), Kasmarek and Houston (2007), and Kasmarek and Ramage (2017).
Water-Level Measurements
Water-level measurements were made at 670 wells in the study area by USGS personnel during November 2021 through March 2022 (Ramage and Adams, 2023a). At each well, a measuring point was established (if one had not been previously established) to determine the depth to water. The height above land surface of the measuring point at each well was measured with an engineering ruler. The depth to water in each well was then measured by using a calibrated steel tape, airline, or electric groundwater-level tape in accordance with methods described in Cunningham and Schalk (2011). Each well was measured during the November 2021 through March 2022 time period to provide a “snapshot” of water-level altitudes in the aquifers associated with individual wells.
In 2022, 15 additional water-level measurements representing about 2 percent of the total number of water-level measurements were provided by municipal utility districts, industrial entities, and powerplants. Municipal utility districts supply water to the businesses and residents of the greater Houston area (Municipal District Services, 2022). Water is used by oil and gas industrial entities during hydrocarbon processing (Allison and Mandler, 2018) and by powerplants to generate electrical power (Torcellini and others, 2003). The provided water levels were typically measured by using air pressure to determine the saturated thickness above the pump intake, and multiple pressure measurements were usually made as a quality-control measure. Air pressure measurements tend to provide less precision than do measurements made with either a calibrated steel tape or electric tape; therefore, all measurements provided by other entities were rounded to the nearest foot. Groundwater levels that were provided by municipal utility districts, industrial entities, or powerplants are identified as such in the “source” field in Ramage and Adams (2023a); additional information about these wells can be obtained from the USGS National Water Information System (NWIS) (U.S. Geological Survey, 2022b).
Most of the measured wells were being pumped at least once daily (and some more frequently) during the study period. Therefore, well pumps were turned off for at least 1 hour when possible before the water-level measurements were made in order to obtain a water-level measurement that approximated the static conditions within the aquifer. Antecedent withdrawal rates and pumping status of nearby wells were not always known and, in such instances, could have affected the representativeness of the water-level data that were collected. To ensure that the recorded water-level measurement was accurate, at least two water-level measurements were made at each well while the well was not being pumped. After the water-level-measurement data were collected, they were thoroughly evaluated and incorporated into a geographic information system as point-data layers and subsequently used for the construction of water-level-altitude and water-level-change maps. The water-level measurements made or provided for this study were carefully reviewed by USGS personnel and were compiled in a companion data release (Ramage and Adams, 2023a). All collected water-level measurements were uploaded into NWIS (U.S. Geological Survey, 2022b); the provided water-level measurements were not uploaded into NWIS.
Kriging
This section is modified from the “Use of Universal Kriging Geostatistical Interpolation Methods To Depict Water-Level Altitudes” section of Ramage and others (2022). Kriging was used to grid water-level-measurement data from 2022 and to grid long-term water-level changes for the Chicot and Evangeline (undifferentiated) and Jasper aquifers. Kriging has long been recognized as an effective method for interpolating hydrologic data, particularly water-level data, as an alternative to contouring methods (Varouchakis and others, 2012) and is a well-established geostatistical method (Matheron, 1963; Paramasivam and Venkatramanan, 2019) that provides an optimized unbiased linear prediction with a minimum mean estimation error. Kriging, like other interpolation methods, uses a weighted average of all measured values to predict values at unmeasured locations. The weights assigned to measured values are spatially dependent. The spatial dependency is quantified by the spatial correlation of the measured locations and is assumed to be more similar in spatial proximity and diverge as spatial distance increases. Correlation between measured locations and their spatial distance relative to one another is defined by the experimental semivariogram and the best-fit model that attempts to minimize the estimation error (Olea, 1975, 2009). Kriging methods for depicting water-level altitudes and water-level changes provide several advantages compared to manual contouring methods, such as those described in Kasmarek and Ramage (2017). One of the advantages of kriging over traditional, manual contouring methods is that kriging provides estimated values of the variable of interest at unmeasured locations. Kriging also provides a means of quantifying the estimation uncertainty (by standard deviation, the square root of the computed variance), allowing for evaluation of the estimation reliability (Todini, 2001). This estimation uncertainty can be useful for determining the spatial variability of the data and allowing for possible optimization of the network of wells. Additional details related to the kriging processes used to depict shaded gridded representations of water-level altitudes in the Chicot and Evangeline (undifferentiated) and Jasper aquifers are described in Ramage and others (2022).
Determination of Water-Level Altitudes
The 2022 regional-scale depictions of water-level altitudes presented in this report were derived from water-level measurements made throughout the 11-county study area from November 2021 through March 2022 (water levels usually are higher because groundwater use is at its lowest during these months compared to the rest of the year). Water-level altitudes were calculated by subtracting the water-level measurement made at each well from the land-surface elevation at that well referenced to NAVD 88 (National Ocean Service, 2021). Prior to 2016, water-level altitudes published in this annual series of USGS reports were referenced to either the National Geodetic Vertical Datum of 1929 or NAVD 88, whereas all water-level altitudes published in 2016 and after are referenced to NAVD 88.
The accuracy of land-surface-elevation data at wells used in the annual series of reports has gradually improved over time as digital elevation models (DEMs) progressively replaced traditional methods of determining land-surface elevations from topographic maps. The most accurate land-surface-elevation data available were used by the USGS for each historical annual depiction of water-level altitudes in the study area. To determine land-surface elevations in 2022, a corresponding land-surface datum was obtained for each well by using a USGS National Geospatial Program 1-meter DEM that provides three-dimensional elevation values referenced to NAVD 88 (Arundel and others, 2015; U.S. Geological Survey, 2022a). In 1977, land-surface-elevation data were extracted from USGS 1:24,000-scale 7.5-minute topographic quadrangle maps for the Gulf of Mexico coastal area, which have a 5-ft contour as described in Gabrysch (1979) and thereby provide an accuracy of approximately 2.5 ft. The DEM used to determine land-surface elevations in 2022 provides an accuracy of 0.5 ft.
Color-shaded gridded representations (shaded grids) of the 2022 water-level altitudes in the Chicot and Evangeline (undifferentiated) and Jasper aquifers were constructed; each color shade represents increments or “bins” of water-level altitudes (50-ft range). To depict the upper and lower bounds of the data on each map, the water-level-altitude increment for the first bin and for the last bin for a given map extends to the lowest and highest estimated water-level-altitude value, respectively. The shaded grids are published in a companion data release (Ramage and Adams, 2023b). The water-level-altitude grids are approximate, regional-scale depictions of the water-level altitudes in the Chicot and Evangeline (undifferentiated) and Jasper aquifers. The areal extents and locations of the shaded areas represented by these grids represent the combined effects of total groundwater withdrawals from all wells screened in the Gulf Coast aquifer system within the study area.
Depicting Long-Term Water-Level Changes
Maps depicting long-term water-level changes in the Chicot and Evangeline (undifferentiated) and Jasper aquifers were constructed for the period of record available for each of the aquifers; data are from 1977 to 2022 for the Chicot and Evangeline aquifers (undifferentiated) and from 2000 to 2022 for the Jasper aquifer. A long-term change map for the period from 1990 to 2022 for the Chicot and Evangeline aquifers (undifferentiated) was also constructed to highlight changes in groundwater levels that may have occurred since the establishment of the FBSD in 1989 and the subsequent increase in the number of wells that were inventoried by the USGS in Fort Bend, Harris, Brazoria, and Waller Counties in 1989 and 1990. Long-term water-level-change maps were constructed from gridded water-level-measurement data from the current year (2022) and from each respective beginning year for the period of record (1977 and 1990, respectively, for the Chicot and Evangeline aquifers [undifferentiated] and 2000 for the Jasper aquifer). Each color shade on the maps represents increments or “bins” of water-level changes (20- or 40-ft range).
A mask was applied to the gridded 2022 water-level altitudes to retain only those grid cells with variance values less than or equal to the mean variance of the 2022 grid (as a whole) as a method for either removing potential outliers or hiding grid cells with insufficient data density. Water-level-measurement data from wells measured in the historical year (1977, 1990, or 2000) were gridded using the same methods that were used to generate the 2022 water-level-altitude grids, and a mask was applied to the gridded historical altitudes to retain only those grid cells with variance values less than or equal to the mean variance of the historical grid (as a whole). The resulting grids derived from water-level-measurement data from 2022 and from 1977, 1990, or 2000 were then cropped to a common geospatial extent before subtracting the historical grid from the 2022 grid. (The grids were cropped to a common geospatial extent because the network of wells where groundwater levels were measured in each aquifer changed over time as wells were added in response to expansion of development throughout the greater Houston area or were taken out of service in certain parts of the study area as surface-water usage increased and groundwater usage decreased, among other reasons.) The area of water-level change shown on the maps is based primarily on the availability of groundwater-level data in both 2022 and the historical year. Another factor when determining the area of mapped water-level change is the computed variance associated with each estimated water-level altitude at each grid cell for both the current year water-level-altitude and historical year water-level-altitude gridded surfaces. If the computed variance at any given grid cell is less than or equal to the overall mean variance for each respective gridded surface for the current year and historical year, the data are deemed valid for inclusion in the mapped area depicting water-level change (Ramage and others, 2022). The datasets of water-level-change values (difference between 2022 and historical year water-level-altitude values) are available in Ramage and Adams (2023b); the grids and their associated variance distribution maps that were produced from the water-level data collected from the network of wells (along with a description of the processes by which these datasets were produced) are included in Ramage and Adams (2023b).
Depicting Short-Term Water-Level Changes
Maps depicting short-term water-level changes in the Chicot and Evangeline (undifferentiated) and Jasper aquifers were constructed to represent changes during the past 5 years (2017–22) and during the past year (2021–22). Because these change maps represent a much shorter time period than do the long-term change maps, changes at the local scale (individual wells or small groups of proximal wells) can be more pronounced in them than in long-term change maps, which are more representative of regional-scale variations in groundwater levels. As a result, using a shaded grid to represent these short-term changes tends to lead to pronounced concentric patterns (bullseyes) in the short-term change maps. Therefore, short-term water-level changes were instead depicted by shading a circular symbol at each of the wells measured during both years representing the short-term interval in question, where the shading represents water-level rises or declines at 10-ft intervals. Kriging was not used to depict the short-term water-level changes. Instead, water-level change determined by direct subtraction of water levels at each well measured at both the beginning and ending of the periods of interest (2017 and 2022 for the 5-year maps; 2021 and 2022 for the 1-year maps) is represented by the shaded symbol for each well in the short-term change maps.
Water-Level Altitudes and Long-Term and Short-Term Water-Level Changes
Maps of water-level altitudes and long-term and short-term water-level changes were constructed for the Chicot and Evangeline (undifferentiated) and Jasper aquifers in the study area (figs. 4–12). The maps in this report depict approximate water-level altitudes for the Chicot and Evangeline aquifers (undifferentiated) in 2022 by using shaded grids where each shade represents a 50-ft interval (fig. 4) and approximate long-term (1977–2022 and 1990–2022) water-level changes in the Chicot and Evangeline aquifers (undifferentiated) by using shaded grids where each shade represents a 40-ft interval (figs. 5 and 6). Approximate short-term (2017–22 and 2021–22) water-level changes in the Chicot and Evangeline aquifers (undifferentiated) are represented by shaded symbols for each well where a water-level measurement was made both in 2022 and in either 2017 or 2021 (figs. 7 and 8). For approximate water-level altitudes for the Jasper aquifer in 2022, shaded grids representing 50-ft intervals were used (fig. 9), and for approximate long-term (2000–22) water-level changes in the Jasper aquifer, shaded grids representing 20-ft intervals were used (fig. 10). Approximate short-term (2017–22 and 2021–22) water-level changes in the Jasper aquifer are represented by shaded symbols for each well where a water-level measurement was made both in 2022 and in either 2017 or 2021 (figs. 11 and 12).
Locations of wells used to construct the 2022 water-level-altitude maps for the Chicot and Evangeline (undifferentiated) and Jasper aquifers are presented in Ramage and Adams (2023a, b). Certain water-level-altitude measurements made during November 2021 through March 2022 were not used to construct the water-level-altitude maps presented in this report. Many of the wells that were excluded when constructing the water-level-altitude maps were colocated wells in nests. It is not possible to establish a variance-distance relation between observations made at the same location (variability of observations over zero distance) during the kriging process; therefore, prior to the kriging process, colocated wells must be “declustered.” When two or more wells were colocated, data from the well where the water level was deepest were retained. The reason that the data from the well with the deepest water level were retained for colocated well sites is because that well was expected to have a water level that was most analogous to nearby production wells, which tend to be deeper and have deeper water levels than do domestic wells or piezometers. Production wells account for most of the groundwater-monitoring network, particularly in Harris County, where most of the colocated wells are located. A much smaller proportion of the wells were excluded when constructing the water-level-altitude maps because they were not consistent with water-level altitudes obtained from nearby wells screened in the same aquifer for one of the following reasons: (1) the water level measured in the well in question differed appreciably from the water levels measured in wells in the surrounding area and was deemed to be erroneous or (2) water had been withdrawn recently from the well in question, temporarily lowering its static water-level altitude. The companion data release by Ramage and Adams (2023b) identifies the water-level-altitude measurements that were used to construct the maps of water-level altitudes and water-level changes for each aquifer. Of the 670 wells where water-level measurements were made in the study area, measurements from 574 wells were used in the construction of the water-level-altitude maps. Individual water-level altitudes measured at each well for the Chicot and Evangeline (undifferentiated) and Jasper aquifers and associated metadata are provided in the companion data releases (Ramage and Adams, 2023a, b).
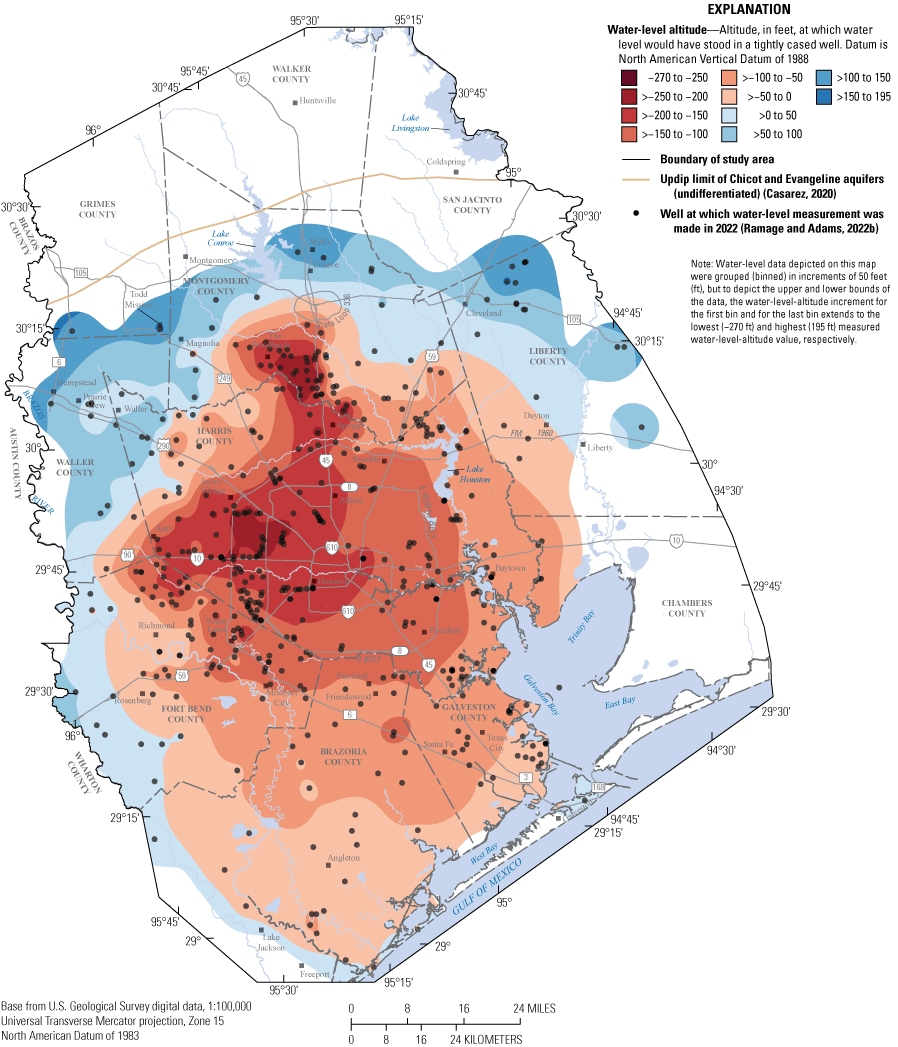
Approximate 2022 water-level altitudes in the Chicot and Evangeline aquifers (undifferentiated), greater Houston study area, Texas (water-level-measurement data collected during November 2021 through March 2022).
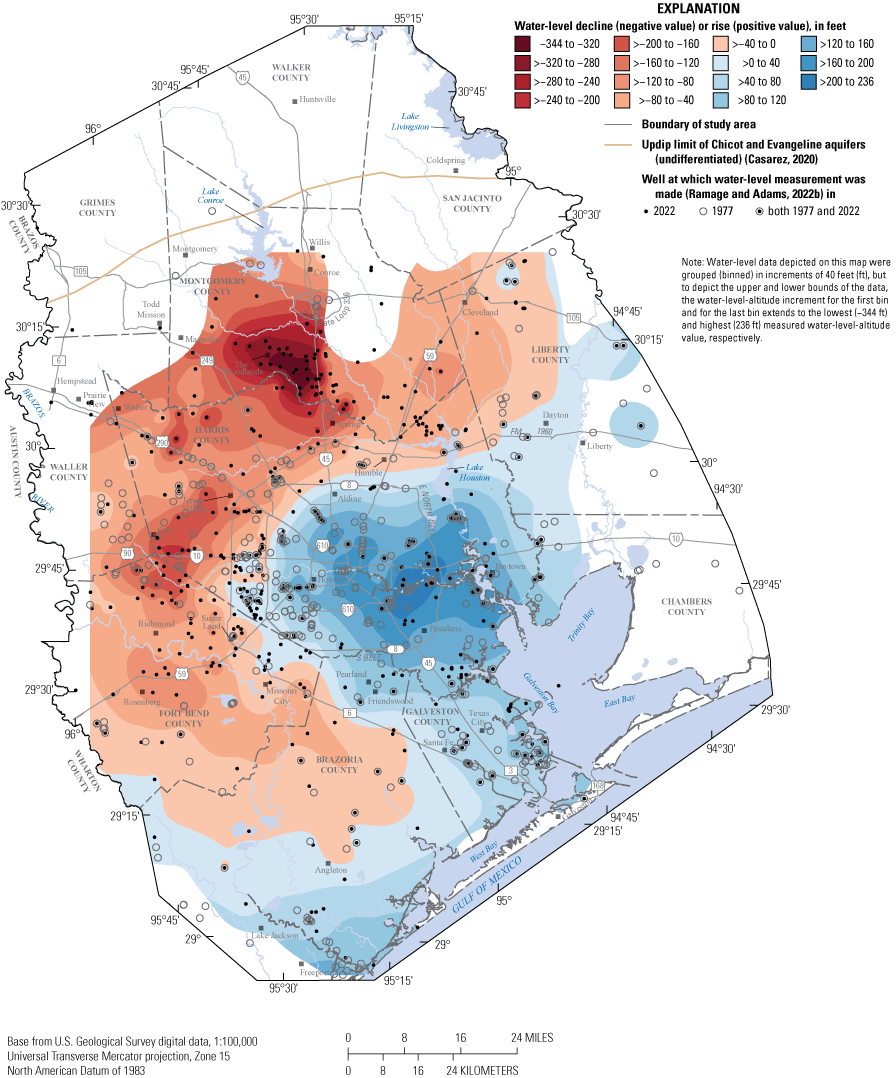
Approximate 1977–2022 water-level changes in the Chicot and Evangeline aquifers (undifferentiated), greater Houston study area, Texas.
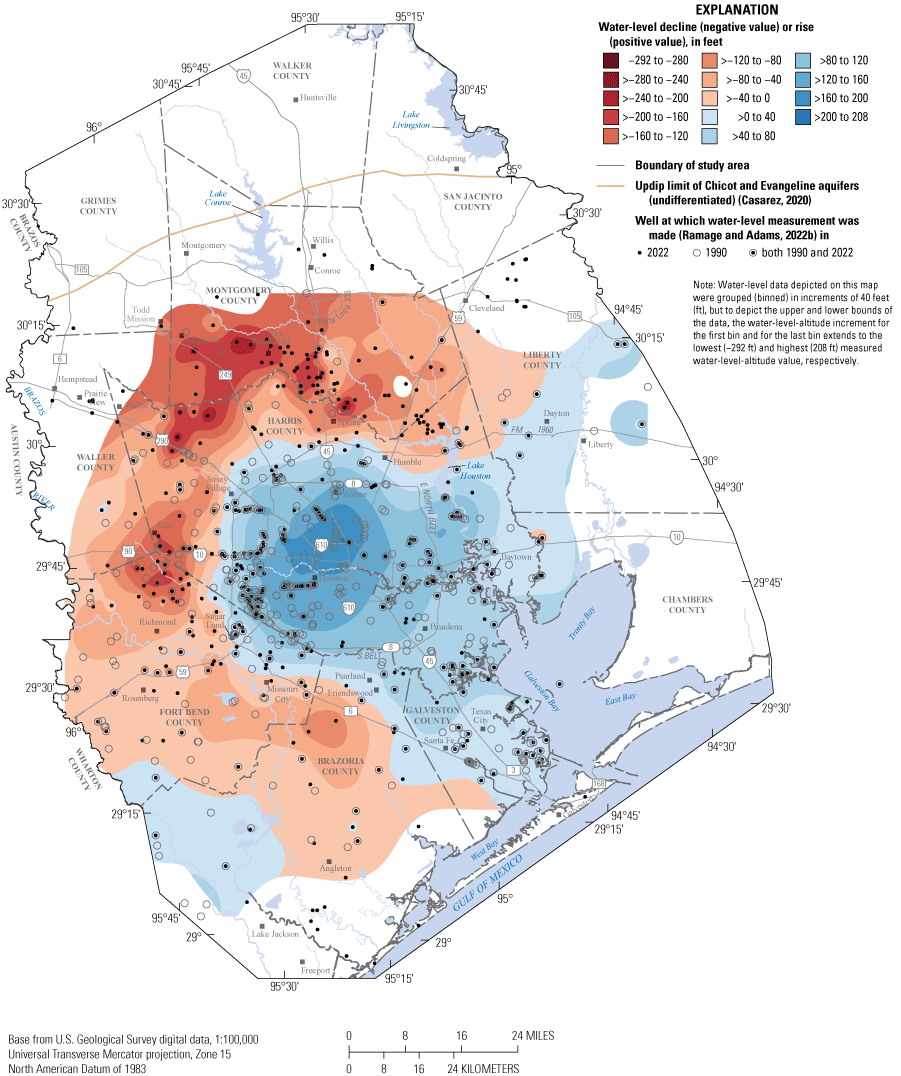
Approximate 1990–2022 water-level changes in the Chicot and Evangeline aquifers (undifferentiated), greater Houston study area, Texas.
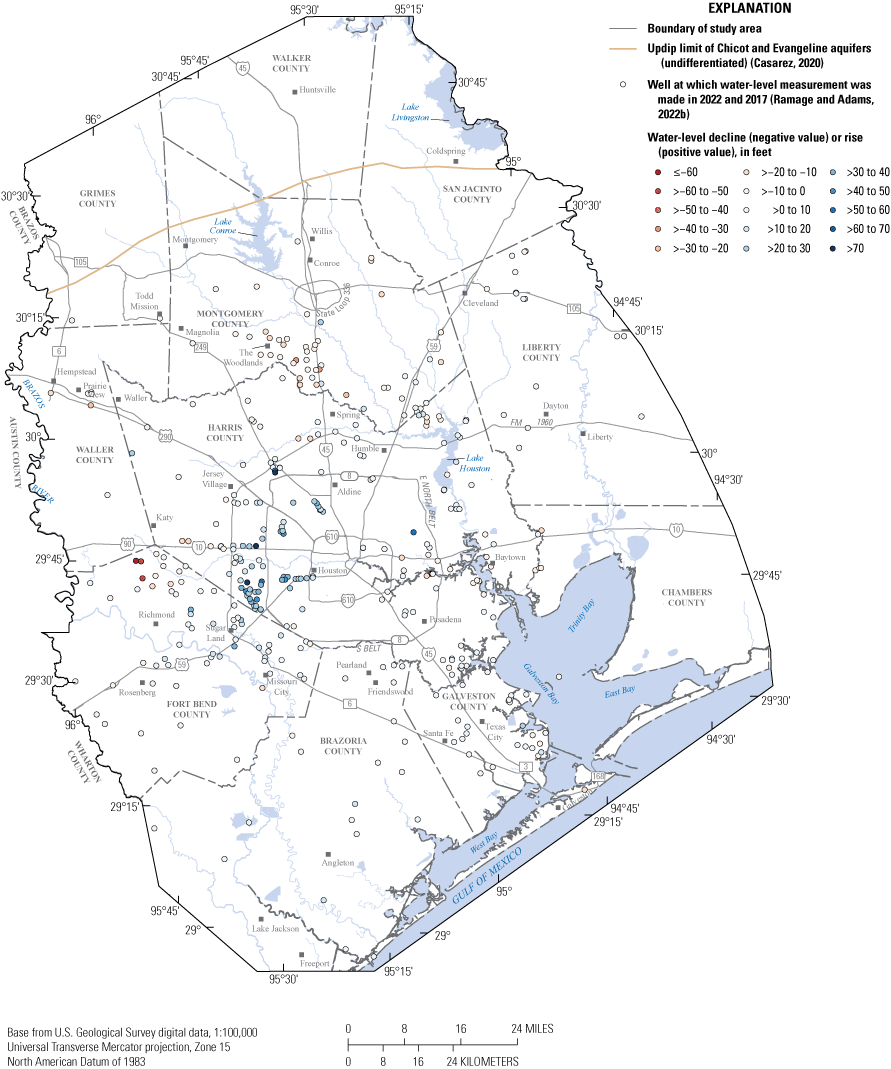
Approximate 2017–22 water-level changes at wells screened in the Chicot and Evangeline aquifers (undifferentiated), greater Houston study area, Texas.
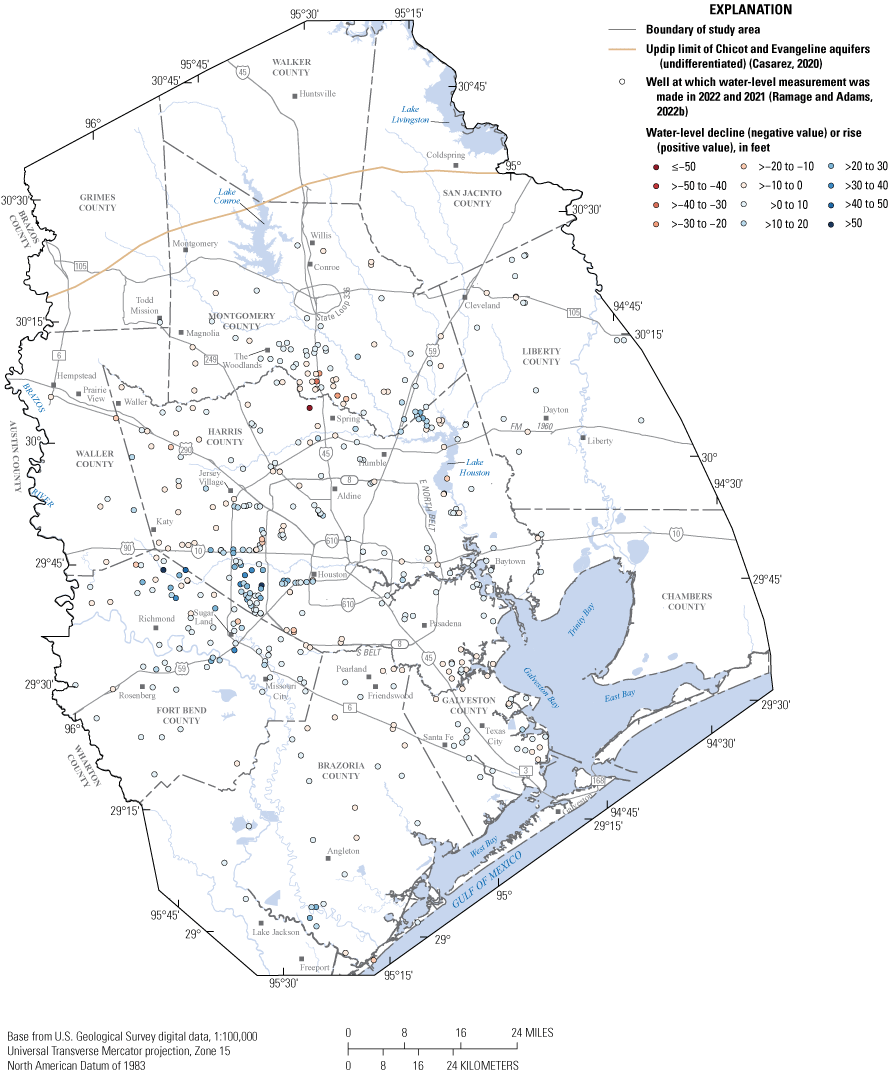
Approximate 2021–22 water-level changes at wells screened in the Chicot and Evangeline aquifers (undifferentiated), greater Houston study area, Texas.

Approximate 2022 water-level altitudes in the Jasper aquifer, greater Houston study area, Texas (water-level-measurement data collected during November 2021 through March 2022).
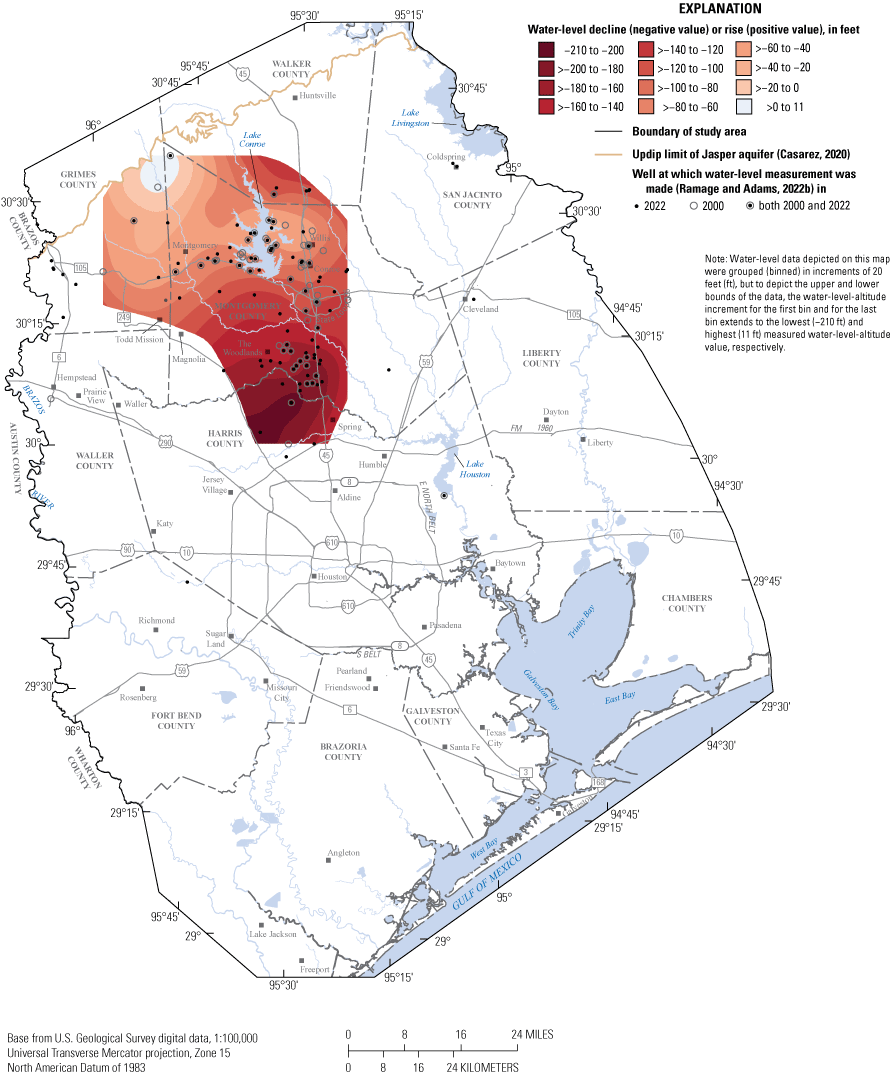
Approximate 2000–22 water-level changes in the Jasper aquifer, greater Houston study area, Texas.
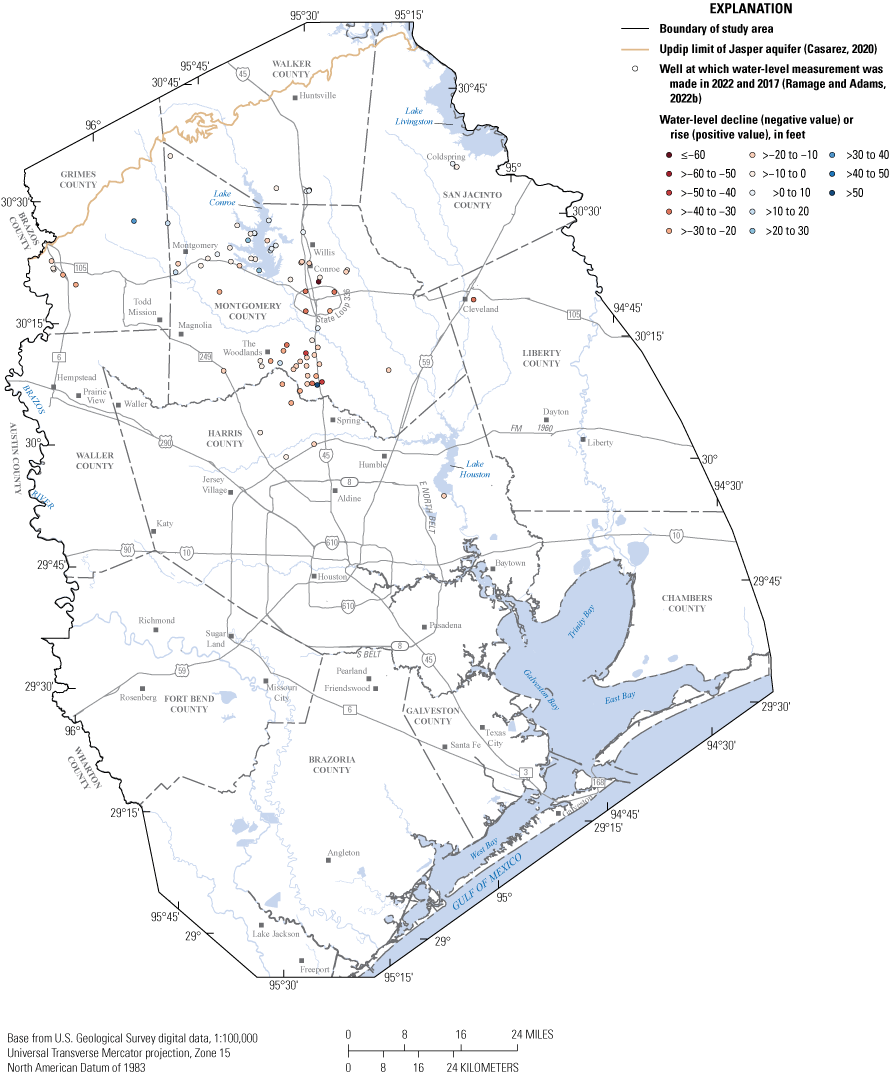
Approximate 2017–22 water-level changes at wells screened in the Jasper aquifer, greater Houston study area, Texas.
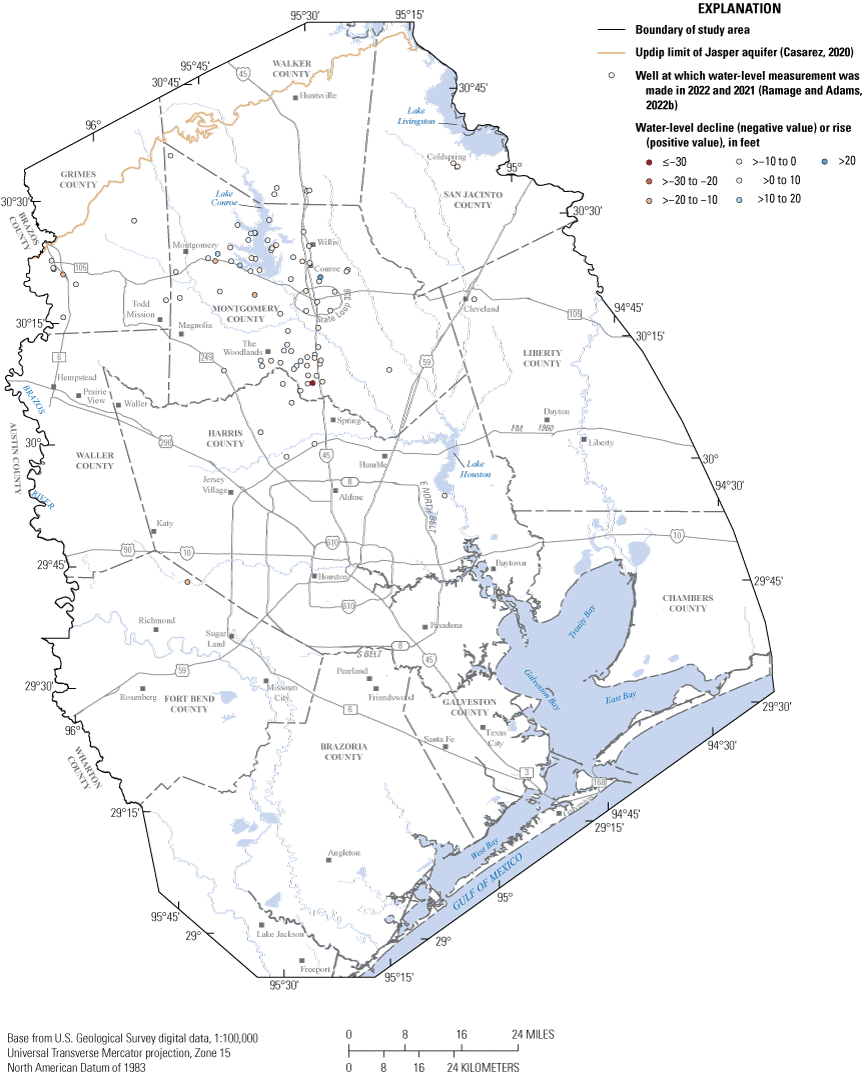
Approximate 2021–22 water-level changes at wells screened in the Jasper aquifer, greater Houston study area, Texas.
Chicot and Evangeline Aquifers (Undifferentiated)
Water-level-measurement data from 470 wells (Ramage and Adams, 2023b) were used to depict the 2022 water-level altitudes for the Chicot and Evangeline aquifers (undifferentiated) (fig. 4). In 2022, observed water-level altitudes for the Chicot and Evangeline aquifers (undifferentiated) were lowest (250–270 ft below NAVD 88, which corresponds to the interval from −270 to −250 ft in fig. 4) at and near a few clusters of wells, which are obscured by the well symbols on the map, in relatively small areas in the south-central part of Montgomery County between The Woodlands and Spring and in an area of west-central Harris County between Interstate 10 and U.S. Highway 290. The highest observed water-level altitudes (150–195 ft above NAVD 88, which corresponds to the interval from greater than [>] 150 to 195 ft in fig. 4) for the Chicot and Evangeline aquifers (undifferentiated) in 2022 were in an area west of Todd Mission, near the western border of Grimes County.
The largest decline in water-level altitudes (320–344 ft, which corresponds to the interval from −344 to −320 ft in fig. 5) indicated by the 1977–2022 long-term water-level-change map for the Chicot and Evangeline aquifers (undifferentiated) was southeast of The Woodlands (fig. 5), whereas the 1990–2022 long-term water-level-change map for the Chicot and Evangeline aquifers (undifferentiated) depicts the largest declines in water-level altitudes (280–292 ft, which corresponds to the interval from −292 to −280 ft in fig. 6) in localized areas at or near certain wells, often obscured by the well symbols on the map, in parts of northwestern Harris County and southern Montgomery County (fig. 6). The largest rise in water-level altitudes in the Chicot and Evangeline aquifers (undifferentiated) (200–236 ft, which corresponds to the interval from >200 to 236 ft in fig. 5) was observed in a relatively large area in southeastern Harris County for 1977–2022, whereas the largest rise in water-level altitudes for 1990–2022 (200–208 ft, which corresponds to the interval from >200 to 208 ft in fig. 6) was observed in a relatively small area in central Harris County.
The 5-year (2017–22) short-term water-level-change map for the Chicot and Evangeline aquifers (undifferentiated) depicts the largest declines (greater than or equal to [≥] 50 ft) at three wells in northern Fort Bend County and the largest rises (>50 ft) at five wells in southwestern and central Harris County (fig. 7). The 1-year (2021–22) short-term water-level-change map for the Chicot and Evangeline aquifers (undifferentiated) depicts the largest decline (≥50 ft) at a well in north-central Harris County and the largest rises (>50 ft) at a well in north-central Fort Bend County and a well in southwestern Harris County (fig. 8).
Jasper Aquifer
Water-level-measurement data from 104 wells (Ramage and Adams, 2023b) were used to depict the approximate 2022 water-level altitudes for the Jasper aquifer (fig. 9). In 2022, water-level altitudes for the Jasper aquifer were lowest (200–213 ft below NAVD 88, which corresponds to the interval from −213 to −200 ft in fig. 9) in an area of south-central Montgomery County and north-central Harris County between The Woodlands and Spring, whereas water-level altitudes for the Jasper aquifer were highest (250–285 ft above NAVD 88, which corresponds to the interval from >250 to 285 ft in fig. 9) in northeastern Grimes, northwestern Montgomery, and southwestern Walker Counties (fig. 9).
Annual water-level-measurement data have been collected from wells completed in the Chicot and Evangeline aquifers (undifferentiated) since 1977, whereas annual water-level-measurement data have been collected from wells completed in the Jasper aquifer since only 2000. The 2000–22 long-term water-level-change map for the Jasper aquifer (fig. 10) depicts water-level declines throughout most of the study area where water-level-measurement data from the Jasper aquifer were collected, with the largest decline (≥200 ft, which corresponds to the interval −210 to −200 ft in fig. 10) in north-central Harris County and south-central Montgomery County south of The Woodlands. One small area of the map in northwestern Montgomery County and east-central Grimes County depicts slight rises of >0 ft (which corresponds to the interval >0 to 11 ft in fig. 10).
The 5-year (2017–22) short-term water-level-change map for the Jasper aquifer depicts the largest decline (≥60 ft) at a well in Conroe and the largest rise (>50 ft) at a well in south-central Montgomery County (fig. 11). The 1-year (2021–22) short-term water-level-change map for the Jasper aquifer depicts the largest decline (≥30 ft) at a well in south-central Montgomery County east of The Woodlands and the largest rise (>20 ft) at a well in Conroe (fig. 12).
Data Limitations
As described in the “Determination of Water-Level Altitudes” section, the accuracy of the land-surface-elevation data has gradually improved over time. Any changes in land-surface elevations, such as inaccuracies associated with the DEM source data, could affect the accuracy of the long-term water-level-change maps depicting the differences between the current year (2022) and the historical year (1977, 1990, or 2000) altitudes.
Shaded representations of water-level altitudes and water-level changes should be viewed in a regional context because water-level altitudes in the Chicot and Evangeline aquifers (undifferentiated) and in the Jasper aquifer are dynamic and can change rapidly in individual wells and spatially over larger areas encompassing many wells as a result of changes in local and regional groundwater withdrawals and different antecedent conditions when groundwater-level measurements were made. Although the shaded gridded surfaces provide more information than did the previously used contours and are based on geostatistical interpolation, the resulting data between groundwater-level measurements are estimated data. Users should be aware that water-level changes may have occurred since the water-level altitudes were determined for the report. Inherent uncertainties exist in these data such as antecedent withdrawal rates and the pumping status of nearby wells. When evaluating the gridded depictions of water-level altitudes and water-level changes (figs. 4–6, 9, and 10), users should exercise discretion and know the limitations of these data when drawing conclusions or making policy decisions. These data should not be used for engineering applications.
Summary
The greater Houston area, Texas, as defined in this report consists of Harris, Galveston, Fort Bend, Montgomery, Brazoria, Chambers, Grimes, Liberty, San Jacinto, Walker, and Waller Counties. Since the early 1900s, groundwater withdrawn from the primary aquifers that compose the Gulf Coast aquifer system—the Chicot, Evangeline, and Jasper aquifers—has been an important source of water for municipal supply, commercial and industrial use, and irrigation in the greater Houston area. This report, prepared by the U.S. Geological Survey in cooperation with the Harris-Galveston Subsidence District, City of Houston, Fort Bend Subsidence District, Lone Star Groundwater Conservation District, and Brazoria County Groundwater Conservation District, is one in an annual series of reports depicting the status of water-level altitudes and water-level changes in these aquifers in the greater Houston area. Groundwater levels in wells screened in the aquifers were measured during November 2021 through March 2022 (water-level altitudes usually are higher during these months compared to the rest of the year).
In this report, the Chicot and Evangeline aquifers are treated as a single hydrogeologic unit for the purposes of providing annual assessments of regional-scale water-level altitudes and changes over time. This report contains regional-scale maps depicting approximate 2022 water-level altitudes and both long-term (1977, 1990, or 2000 to 2022) and short-term (1-year and 5-year) water-level changes in the Chicot and Evangeline (undifferentiated) and Jasper aquifers. Water-level measurements from 470 and 104 wells were used to depict the approximate 2022 water-level altitudes for the Chicot and Evangeline (undifferentiated) aquifers and for the Jasper aquifer, respectively.
In 2022, shaded depictions of water-level altitudes for the Chicot and Evangeline aquifers (undifferentiated) ranged from about 270 feet (ft) below the North American Vertical Datum of 1988 (NAVD 88) to about 195 ft above NAVD 88. The largest decline in water-level altitudes (about 344 ft) indicated by the 1977–2022 long-term water-level-change map for the Chicot and Evangeline aquifers (undifferentiated) was southeast of The Woodlands, whereas the 1990–2022 long-term water-level-change map for the Chicot and Evangeline aquifers (undifferentiated) depicts the largest declines in water-level altitudes (about 292 ft) in localized areas at or near certain wells in parts of northwestern Harris County and southern Montgomery County. The largest rise in water-level altitudes in the Chicot and Evangeline aquifers (undifferentiated) (about 236 ft) was observed in a relatively large area in southeastern Harris County for 1977–2022, whereas the largest rise in water-level altitudes for 1990–2022 (about 208 ft) was observed in a relatively small area in central Harris County. The 5-year (2017–22) short-term water-level-change map for the Chicot and Evangeline aquifers (undifferentiated) depicts the largest declines (greater than or equal to [≥] 50 ft) at three wells in northern Fort Bend County and the largest rises (greater than [>] 50 ft) at five wells in southwestern and central Harris County. The 1-year (2021–22) short-term water-level-change map for the Chicot and Evangeline aquifers (undifferentiated) depicts the largest decline (≥50 ft) at a well in north-central Harris County and the largest rises (>50 ft) at a well in north-central Fort Bend County and a well in southwestern Harris County.
In 2022, shaded depictions of water-level altitudes for the Jasper aquifer ranged from about 213 ft below NAVD 88 to about 285 ft above NAVD 88. The 2000–22 long-term water-level-change map for the Jasper aquifer depicts water-level declines throughout most of the study area where water-level-measurement data from the Jasper aquifer were collected, with the largest decline (≥200 ft) in north-central Harris County and south-central Montgomery County south of The Woodlands. One small area of the map in northwestern Montgomery County and east-central Grimes County depicts slight rises of >0 (as much as about 11) ft. The 5-year (2017–22) short-term water-level-change map for the Jasper aquifer depicts the largest decline (≥60 ft) at a well in Conroe; the largest rise (>50 ft) was at a well in south-central Montgomery County. The 1-year (2021–22) short-term water-level-change map for the Jasper aquifer depicts the largest decline (≥30 ft) at a well in south-central Montgomery County east of The Woodlands and the largest rise (>20 ft) at a well in Conroe.
Any changes in land-surface elevations could affect the accuracy of the long-term water-level-change maps depicting the differences between the current year (2022) and the historical year (1977, 1990, or 2000) altitudes. Users should be aware that water-level changes may have occurred since the water-level altitudes were determined for this report. Inherent uncertainties exist in these data such as antecedent withdrawal rates and the pumping status of nearby wells. When evaluating the gridded depictions of water-level altitudes and water-level changes, users should exercise discretion and know the limitations of these data when drawing conclusions or making policy decisions. These data should not be used for engineering applications.
References Cited
Allison, E., and Mandler, B., 2018, Water in the oil and gas industry—An overview of the many roles of water in oil and gas operations, part 2 of Petroleum and the environment: American Geosciences Institute, 2 p., accessed August 25, 2022, at https://www.americangeosciences.org/geoscience-currents/water-oil-and-gas-industry.
Arundel, S.T., Archuleta, C.M., Phillips, L.A., Roche, B.L., and Constance, E.W., 2015, 1-meter digital elevation model specification: U.S. Geological Survey Techniques and Methods, book 11, chap. B7, 25 p. with appendixes, accessed August 25, 2022, at https://doi.org/10.3133/tm11B7.
Baker, E.T., Jr., 1979, Stratigraphic and hydrogeologic framework of part of the Coastal Plain of Texas: Texas Department of Water Resources Report 236, 43 p., accessed August 25, 2022, at https://www.twdb.texas.gov/publications/reports/numbered_reports/doc/R236/R236.pdf.
Baker, E.T., Jr., 1986, Hydrology of the Jasper aquifer in the southeast Texas Coastal Plain: Texas Water Development Board Report 295, 64 p., accessed August 25, 2022, at https://www.twdb.texas.gov/publications/reports/numbered_reports/doc/R295/R295.pdf.
Barbie, D.L., Coplin, L.S., and Locke, G.L., 1991, Approximate altitude of water levels in wells in the Chicot and Evangeline aquifers in the Houston area, Texas, January–February 1990: U.S. Geological Survey Open-File Report 91–240, 2 sheets, accessed August 25, 2022, at https://doi.org/10.3133/ofr91240.
Bluebonnet Groundwater Conservation District, 2018, Bluebonnet Groundwater Conservation District groundwater management plan: Bluebonnet Groundwater Conservation District, 179 p., accessed August 25, 2022, at http://www.bluebonnetgroundwater.org/wp-content/uploads/2019/10/ADOPTED-MGMT-Plan-10-108-v2.pdf.
Bluebonnet Groundwater Conservation District, 2021, District history: Bluebonnet Groundwater Conservation District web page, accessed August 25, 2022, at http://www.bluebonnetgroundwater.org/district/history/.
Borrok, D.M., and Broussard, W.P., III, 2016, Long-term geochemical evaluation of the coastal Chicot aquifer system, Louisiana, USA: Journal of Hydrology, v. 533, p. 320–331, accessed August 25, 2022, at https://doi.org/10.1016/j.jhydrol.2015.12.022.
Braun, C.L., and Ramage, J.K., 2020, Status of groundwater-level altitudes and long-term groundwater-level changes in the Chicot, Evangeline, and Jasper aquifers, Houston-Galveston region, Texas, 2020: U.S. Geological Survey Scientific Investigations Report 2020–5089, 18 p., accessed August 25, 2022, at https://doi.org/10.3133/sir20205089.
Braun, C.L., and Ramage, J.K., 2022, Status of water-level altitudes and long-term water-level changes in the Chicot and Evangeline (undifferentiated) and Jasper aquifers, greater Houston area, Texas, 2021 (ver. 1.1, August 19, 2022): U.S. Geological Survey Scientific Investigations Report 2022–5065, 25 p., accessed August 25, 2022, at https://doi.org/10.3133/sir20225065.
Braun, C.L., Ramage, J.K., and Shah, S.D., 2019, Status of groundwater-level altitudes and long-term groundwater-level changes in the Chicot, Evangeline, and Jasper aquifers, Houston-Galveston region, Texas, 2019: U.S. Geological Survey Scientific Investigations Report 2019–5089, 18 p., accessed August 25, 2022, at https://doi.org/10.3133/sir20195089.
Brazoria County Groundwater Conservation District, 2017, Brazoria County Groundwater Conservation District groundwater management plan: Brazoria County Groundwater Conservation District, 130 p., accessed August 25, 2022, at https://www.bcgroundwater.org/images/bcg/documents/2017/Groundwater-Management-Plan-2017.pdf.
Brazoria County Groundwater Conservation District, 2021, About the district: Brazoria County Groundwater Conservation District web page, accessed August 25, 2022, at https://www.bcgroundwater.org/bcg/About_the_District.asp.
Carr, J.E., Meyer, W.R., Sandeen, W.M., and McLane, I.R., 1985, Digital models for simulation of ground-water hydrology of the Chicot and Evangeline aquifers along the Gulf Coast of Texas: Texas Department of Water Resources Report 289, 101 p., accessed August 25, 2022, at https://www.twdb.texas.gov/publications/reports/numbered_reports/doc/R289/Report289.pdf.
Casarez, I.R., 2020, Aquifer extents in the coastal lowlands aquifer system regional groundwater availability study area in Texas, Louisiana, Mississippi, Alabama, and Florida: U.S. Geological Survey data release, accessed August 25, 2022, at https://doi.org/10.5066/P9BH2KG2.
Chowdhury, A.H., and Turco, M.J., 2006, Geology of the Gulf Coast aquifer, Texas, chap. 2 of Mace, R.E., Davidson, S.C., Angle, E.S., and Mullican, W.F., eds., Aquifers of the Gulf Coast of Texas: Texas Water Development Board Report 365, p. 23–50, accessed August 25, 2022, at https://www.twdb.texas.gov/publications/reports/numbered_reports/doc/R365/ch02-Geology.pdf.
Coplin, L.S., 2001, Water-level altitudes in wells completed in the Jasper aquifer, greater Houston area, Texas, spring 2000: U.S. Geological Survey Open-File Report 01–147, 2 p., accessed August 25, 2022, at https://doi.org/10.3133/ofr01147.
Coplin, L.S., and Galloway, D., 1999, Houston-Galveston, Texas—Managing coastal subsidence, in Galloway, D., Jones, D.R., and Ingebritsen, S.E., eds., Land subsidence in the United States: U.S. Geological Survey Circular 1182, p. 35–48, accessed April 30, 2021, at https://doi.org/10.3133/cir1182.
Cunningham, W.L., and Schalk, C.W., comps., 2011, Groundwater technical procedures of the U.S. Geological Survey: U.S. Geological Survey Techniques and Methods, book 1, chap. A1, 151 p., accessed August 25, 2022, at https://doi.org/10.3133/tm1A1.
Ellis, J.H., Knight, J.E., White, J.T., Sneed, M., Hughes, J.D., Ramage, J.K., Braun, C.L., Teeple, A., Foster, L., Rendon, S.H., and Brandt, J., 2023, Hydrogeology, land-surface subsidence, and documentation of the GULF model, southeast Texas, 1897–2018: U.S. Geological Survey Professional Paper 1877, 407 p., accessed January 23, 2023, at https://doi.org/10.3133/pp1877.
Fort Bend Subsidence District, 2013, Fort Bend Subsidence District 2013 regulatory plan [amended 2007, 2009, and 2013]: Fort Bend Subsidence District, 14 p., accessed August 25, 2022, at http://fbsubsidence.org/wp-content/uploads/2020/07/FBSD-Regulatory-Plan-Amended-2013.pdf.
Fort Bend Subsidence District, 2021, Fort Bend Subsidence District—District regulatory plan: Fort Bend Subsidence District, accessed August 25, 2022, at https://fbsubsidence.org/planning/regulatory-plan/.
Gabrysch, R.K., 1967, Development of ground water in the Houston district, Texas, 1961–65: Texas Water Development Board Report 63, 35 p., accessed August 25, 2022, at https://www.twdb.texas.gov/publications/reports/numbered_reports/doc/R63/Report63.asp.
Gabrysch, R.K., 1972, Development of ground water in the Houston district, Texas, 1966–69: Texas Water Development Board Report 152, 24 p., accessed August 25, 2022, at https://www.twdb.texas.gov/publications/reports/numbered_reports/doc/R152/R%20152.pdf.
Gabrysch, R.K., 1979, Approximate altitude of water levels in wells in the Chicot and Evangeline aquifers in the Houston area, Texas, spring 1977 and spring 1978: U.S. Geological Survey Open-File Report 79–334, 4 sheets, accessed August 25, 2022, at https://doi.org/10.3133/ofr79334.
Gabrysch, R.K., 1980, Development of ground water in the Houston district, Texas, 1970–74: Texas Water Development Board Report 241, 49 p., accessed August 25, 2022, at https://www.twdb.texas.gov/publications/reports/numbered_reports/doc/R241/report241.asp.
Gabrysch, R.K., 1984, Ground-water withdrawals and land surface subsidence in the Houston-Galveston region, Texas, 1906–80: Texas Department of Water Resources Report 287, 64 p., accessed January 10, 2023, at https://www.twdb.texas.gov/publications/reports/numbered_reports/doc/R287/R287.pdf.
Gabrysch, R.K., and Bonnet, C.W., 1975, Land-surface subsidence in the Houston-Galveston region, Texas: Texas Water Development Board Report 188, 19 p., accessed January 10, 2023, at https://www.twdb.texas.gov/publications/reports/numbered_reports/doc/R188.pdf.
Harris-Galveston Subsidence District, 2013, Regulatory plan 2013: Harris-Galveston Subsidence District, 14 p., accessed August 25, 2022, at http://hgsubsidence.org/wp-content/uploads/2013/07/HGSD-2013-Regulatory-Plan-with-Amendment.pdf.
Harris-Galveston Subsidence District, 2021, Legislative authority: Harris-Galveston Subsidence District web page, accessed August 25, 2022, at https://hgsubsidence.org/about/legislative-authority/.
Healy, R.W., 2010, Estimating groundwater recharge, with contributions by B.R. Scanlon: Cambridge, U.K., Cambridge University Press, 245 p., accessed January 10, 2023, at https://doi.org/10.1017/CBO9780511780745.
Holzer, T.L., and Bluntzer, R.L., 1984, Land subsidence near oil and gas fields, Houston, Texas: Ground Water, v. 22, p. 450–459, accessed January 10, 2023, at https://doi.org/10.1111/j.1745-6584.1984.tb01416.x.
Johnson, M.R., Ramage, J.K., and Kasmarek, M.C., 2011, Water-level altitudes 2011 and water-level changes in the Chicot, Evangeline, and Jasper aquifers and compaction 1973–2010 in the Chicot and Evangeline aquifers, Houston–Galveston region, Texas: U.S. Geological Survey Scientific Investigations Map 3174, 17 p., 16 sheets, accessed August 25, 2022, at https://doi.org/10.3133/sim3174.
Kasmarek, M.C., 1997, Water-level altitudes in wells completed in the Chicot and Evangeline aquifers, Fort Bend County and adjacent areas, Texas, January–February 1990: U.S. Geological Survey Open-File Report 97–784, 2 sheets, accessed August 25, 2022, at https://doi.org/10.3133/ofr97784.
Kasmarek, M.C., 2012, Hydrogeology and simulation of groundwater flow and land-surface subsidence in the northern part of the Gulf Coast aquifer system, Texas, 1891–2009 (ver. 1.1, December 2013): U.S. Geological Survey Scientific Investigations Report 2012–5154, 55 p., accessed August 25, 2022, at https://doi.org/10.3133/sir20125154.
Kasmarek, M.C., Gabrysch, R.K., and Johnson, M.R., 2009, Estimated land-surface subsidence in Harris County, Texas, 1915–17 to 2001 (ver. 1.1., February 8, 2010): U.S. Geological Survey Scientific Investigations Map 3097, 2 sheets, accessed January 12, 2023, at https://doi.org/10.3133/sim3097.
Kasmarek, M.C., and Houston, N.A., 2007, Water-level altitudes 2007 and water-level changes in the Chicot, Evangeline, and Jasper aquifers and compaction 1973–2006 in the Chicot and Evangeline aquifers, Houston-Galveston region, Texas: U.S. Geological Survey Scientific Investigations Map 2968, 159 p., 18 sheets, accessed August 25, 2022, at https://doi.org/10.3133/sim2968.
Kasmarek, M.C., and Houston, N.A., 2008, Water-level altitudes 2008 and water-level changes in the Chicot, Evangeline, and Jasper aquifers and compaction 1973–2007 in the Chicot and Evangeline aquifers, Houston-Galveston region, Texas: U.S. Geological Survey Scientific Investigations Map 3031, 4 p., 17 sheets, accessed August 25, 2022, at https://pubs.usgs.gov/sim/3031.
Kasmarek, M.C., Houston, N.A., and Brown, D.W., 2006, Water-level altitudes 2006 and water-level changes in the Chicot, Evangeline, and Jasper aquifers and compaction 1973–2005 in the Chicot and Evangeline aquifers, Houston-Galveston region, Texas: U.S. Geological Survey Open-File Report 2006–1079, 15 sheets, accessed August 25, 2022, at https://doi.org/10.3133/ofr20061079.
Kasmarek, M.C., Houston, N.A., and Ramage, J.K., 2009, Water-level altitudes 2009 and water-level changes in the Chicot, Evangeline, and Jasper aquifers and compaction 1973–2008 in the Chicot and Evangeline aquifers, Houston-Galveston region, Texas (ver. 1.3, March 15, 2011): U.S. Geological Survey Scientific Investigations Map 3081, 3 p., 16 sheets, 2 app., accessed August 25, 2022, at https://doi.org/10.3133/sim3081.
Kasmarek, M.C., Johnson, M.R., and Ramage, J.K., 2010, Water-level altitudes 2010 and water-level changes in the Chicot, Evangeline, and Jasper aquifers and compaction 1973–2009 in the Chicot and Evangeline aquifers, Houston-Galveston region, Texas: U.S. Geological Survey Scientific Investigations Map 3138, 17 p., 16 sheets, 1 app., accessed August 25, 2022, at https://doi.org/10.3133/sim3138.
Kasmarek, M.C., Johnson, M.R., and Ramage, J.K., 2012, Water-level altitudes 2012 and water-level changes in the Chicot, Evangeline, and Jasper aquifers and compaction 1973–2011 in the Chicot and Evangeline aquifers, Houston-Galveston region, Texas: U.S. Geological Survey Scientific Investigations Map 3230, 18 p., 16 sheets, accessed August 25, 2022, at https://doi.org/10.3133/sim3230.
Kasmarek, M.C., Johnson, M.R., and Ramage, J.K., 2013, Water-level altitudes 2013 and water-level changes in the Chicot, Evangeline, and Jasper aquifers and compaction 1973–2012 in the Chicot and Evangeline aquifers, Houston-Galveston region, Texas: U.S. Geological Survey Scientific Investigations Map 3263, 19 p., 16 sheets, accessed August 25, 2022, at https://doi.org/10.3133/sim3263.
Kasmarek, M.C., Johnson, M.R., and Ramage, J.K., 2014, Water-level altitudes 2014 and water-level changes in the Chicot, Evangeline, and Jasper aquifers and compaction 1973–2013 in the Chicot and Evangeline aquifers, Houston-Galveston region, Texas: U.S. Geological Survey Scientific Investigations Map 3308, 16 sheets, 31-p. pamphlet, accessed August 25, 2022, at https://doi.org/10.3133/sim3308.
Kasmarek, M.C., and Lanning-Rush, J., 2004, Water-level altitudes 2004 and water-level changes in the Chicot, Evangeline, and Jasper aquifers and compaction 1973–2003 in the Chicot and Evangeline aquifers, Houston-Galveston region, Texas: U.S. Geological Survey Open-File Report 2004–1084, 13 sheets, accessed August 25, 2022, at https://doi.org/10.3133/ofr20041084.
Kasmarek, M.C., and Ramage, J.K., 2017, Water-level altitudes 2017 and water-level changes in the Chicot, Evangeline, and Jasper aquifers and compaction 1973–2016 in the Chicot and Evangeline aquifers, Houston-Galveston region, Texas: U.S. Geological Survey Scientific Investigations Report 2017–5080, 32 p., accessed August 25, 2022, at https://doi.org/10.3133/sir20175080.
Kasmarek, M.C., Ramage, J.K., Houston, N.A., Johnson, M.R., and Schmidt, T.S., 2015, Water-level altitudes 2015 and water-level changes in the Chicot, Evangeline, and Jasper aquifers and compaction 1973–2014 in the Chicot and Evangeline aquifers, Houston-Galveston region, Texas: U.S. Geological Survey Scientific Investigations Map 3337, 16 sheets, scale 1:100,000, 35-p. pamphlet, accessed August 25, 2022, at https://doi.org/10.3133/sim3337.
Kasmarek, M.C., Ramage, J.K., and Johnson, M.R., 2016, Water-level altitudes 2016 and water-level changes in the Chicot, Evangeline, and Jasper aquifers and compaction 1973–2015 in the Chicot and Evangeline aquifers, Houston-Galveston region, Texas: U.S. Geological Survey Scientific Investigations Map 3365, 16 sheets, scale 1:100,000, 53-p. pamphlet, accessed August 25, 2022, at https://doi.org/10.3133/sim3365.
Kasmarek, M.C., and Robinson, J.L., 2004, Hydrogeology and simulation of ground-water flow and land-surface subsidence in the northern part of the Gulf Coast aquifer system, Texas: U.S. Geological Survey Scientific Investigations Report 2004–5102, 111 p., accessed August 25, 2022, at https://doi.org/10.3133/sir20045102.
Lambeck, K., Esat, T.M., and Potter, E.K., 2002, Links between climate and sea levels for the past three million years: Nature, v. 419, no. 6903, p. 199–206, accessed August 25, 2022, at https://doi.org/10.1038/nature01089.
Liu, Y., Li, J., and Fang, Z.N., 2019, Groundwater level change management on control of land subsidence supported by borehole extensometer compaction measurements in the Houston-Galveston region, Texas: Geosciences, v. 9, no. 5, 19 p., accessed August 25, 2022, at https://doi.org/10.3390/geosciences9050223.
Lone Star Groundwater Conservation District, 2020, Groundwater management plan—Adopted April 14, 2020: Lone Star Groundwater Conservation District, 114 p., accessed August 25, 2022, at https://static1.squarespace.com/static/58347802cd0f6854e2f90e45/t/5f5aa373fc64123d4cd68a44/1599775678824/LSGCD+MP+%28Final+Clean%29_Adopted+5.15.20.pd f.
Lone Star Groundwater Conservation District, 2021, Our history: Lone Star Groundwater Conservation District web page, accessed August 25, 2022, at https://www.lonestargcd.org/history.
Lower Trinity Groundwater Conservation District, 2019, Groundwater management plan: Lower Trinity Groundwater Conservation District, 106 p., accessed April 30, 2021, at https://4596a5d4-d630-4058-8899-b9dd610c192c.filesusr.com/ugd/125bf6_b1700eade64040d7a3791979b153f1d3.pdf.
Lower Trinity Groundwater Conservation District, 2021, What is the Lower Trinity Groundwater Conservation District?: Lower Trinity Groundwater Conservation District web page, accessed August 25, 2022, at https://www.waterwells.info/.
Matheron, G., 1963, Principles of geostatistics: Economic Geology and the Bulletin of the Society of Economic Geologists, v. 58, no. 8, p. 1246–1266, accessed August 25, 2022, at https://pubs.geoscienceworld.org/segweb/economicgeology/article/58/8/1246/17275/Principles-of-geostatistics.
Meyer, W.R., and Carr, J.E., 1979, A digital model for simulation of ground-water hydrology in the Houston area, Texas: Texas Department of Water Resources LP–103, 27 p., accessed August 25, 2022, at http://www.twdb.texas.gov/publications/reports/limited_printing/doc/LP-103/LP-103%20a.pdf.
Municipal District Services, 2022, About Municipal District Services: Municipal District Services web page, accessed August 25, 2022, at https://www.mdswater.com/.
National Ocean Service, 2021, What is geodesy?—Global positioning tutorial: National Oceanic and Atmospheric Administration, National Ocean Service web page, accessed August 25, 2022, at https://oceanservice.noaa.gov/education/tutorial_geodesy/geo01_intro.html.
Olea, R.A., 1975, Optimum mapping techniques using regionalized variable theory: Lawrence, Kansas Geological Survey, Number 2 Series on Spatial Analysis, 137 p., accessed August 25, 2022, at https://www.kgs.ku.edu/Publications/Bulletins/SpA2/SpatialAnalysis2.pdf.
Olea, R.A., 2009, A practical primer on geostatistics (ver. 1.4, December 2018): U.S. Geological Survey Open-File Report 2009–1103, 346 p., accessed August 25, 2022, at https://doi.org/10.3133/ofr20091103.
Paramasivam, C.R., and Venkatramanan, S., 2019, An introduction to various spatial analysis techniques: GIS and Geostatistical Techniques for Groundwater Science, p. 23–30, accessed August 25, 2022, at https://doi.org/10.1016/B978-0-12-815413-7.00003-1.
Ramage, J.K., and Adams, A.C., 2023a, Depth to groundwater measured from wells completed in the Chicot and Evangeline (undifferentiated) and Jasper aquifers, greater Houston area, Texas, 2022: U.S. Geological Survey data release, https://doi.org/10.5066/P967ZHTU.
Ramage, J.K., and Adams, A.C., 2023b, Groundwater-level altitudes and long-term groundwater-level changes in the Chicot and Evangeline (undifferentiated) and Jasper aquifers, greater Houston area, Texas, 2022: U.S. Geological Survey data release, https://doi.org/10.5066/P9F32XDT.
Ramage, J.K., Braun, C.L., and Ellis, J.H., 2022, Treatment of the Chicot and Evangeline aquifers as a single hydrogeologic unit and use of geostatistical interpolation methods to develop gridded surfaces of water-level altitudes and water-level changes in the Chicot and Evangeline aquifers (undifferentiated) and Jasper aquifer, greater Houston area, Texas, 2021: U.S. Geological Survey Scientific Investigations Report 2022–5064, 51 p., accessed August 25, 2022, at https://doi.org/10.3133/sir20225064.
Shah, S.D., Ramage, J.K., and Braun, C.L., 2018, Status of groundwater-level altitudes and long-term groundwater-level changes in the Chicot, Evangeline, and Jasper aquifers, Houston-Galveston region, Texas, 2018: U.S. Geological Survey Scientific Investigations Report 2018–5101, 18 p., accessed August 25, 2022, at https://doi.org/10.3133/sir20185101.
Teeple, A.P., Foster, L.K., Lindaman, M.A., Duncan, L.L., and Casarez, I., 2021, Hydrogeologic data for the development of the hydrogeologic framework of the coastal lowlands aquifer system regional groundwater availability study area in Texas, Louisiana, Mississippi, Alabama, and Florida: U.S. Geological Survey data release, accessed August 25, 2022, at https://doi.org/10.5066/P9PEFY11.
Todini, E., 2001, Influence of parameter estimation uncertainty in kriging—Part 1 - Theoretical development: Hydrology and Earth System Sciences, v. 5, no. 2, p. 215–223, accessed August 25, 2022, at https://doi.org/10.5194/hess-5-215-2001.
Torcellini, P.N., Long, N., and Judkoff, R., 2003, Consumptive water use for U.S. power production: National Renewable Energy Laboratory report no. NREL/TP–550–33905, 18 p., accessed August 25, 2022, at https://www.nrel.gov/docs/fy04osti/33905.pdf.
Turcan, A.N., Jr., Wesselman, J.B., and Kilburn, C., 1966, Interstate correlation of aquifers, southwestern Louisiana and southeastern Texas: U.S. Geological Survey Professional Paper 550–D, p. D1–D6, accessed April 30, 2021, at https://doi.org/10.3133/pp550D.
U.S. Geological Survey, 2022a, About 3DEP products & services: U.S. Geological Survey, 3D Elevation Program web page, accessed August 25, 2022, at https://www.usgs.gov/3d-elevation-program/about-3dep-products-services.
U.S. Geological Survey, 2022b, USGS water data for the Nation: U.S. Geological Survey National Water Information System database, accessed August 25, 2022, at https://doi.org/10.5066/F7P55KJN.
Varouchakis, E.A., Hristopulos, D.T., and Karatzas, G.P., 2012, Improving kriging of groundwater level data using nonlinear normalizing transformations—A field application: Hydrological Sciences Journal, v. 57, no. 7, p. 1404–1419, accessed August 25, 2022, at https://doi.org/10.1080/02626667.2012.717174.
White, W.N., Livingston, P.P., and Turner, S.F., 1939, Ground-water resources of the Houston-Galveston area, Texas: Texas Board of Water Engineers Report 130, 16 p., accessed August 25, 2022, at https://www.twdb.texas.gov/publications/reports/historic_groundwater_reports/doc/M130.pdf.
White, W.N., Rose, N.A., and Guyton, W.F., 1944, Ground-water resources of the Houston district, Texas: U.S. Geological Survey Water-Supply Paper 889–C, accessed August 25, 2022, at https://doi.org/10.3133/wsp889C.
Williamson, A.K., Grubb, H.F., and Weiss, J.S., 1990, Groundwater flow in the Gulf Coast aquifer systems, south central United States—A preliminary analysis: U.S. Geological Survey Water-Resources Investigations Report 89–4071, 124 p., accessed August 25, 2022, at https://doi.org/10.3133/wri894071.
Young, S.C., and Draper, C., 2020, The delineation of the Burkeville confining unit and the base of the Chicot aquifer to support the development of the Gulf 2023 groundwater model: Harris-Galveston Subsidence District and Fort Bend Subsidence District report, prepared by INTERA, Inc., 75 p., accessed August 25, 2022, at https://hgsubsidence.org/wp-content/uploads/2021/06/Final_HGSD_FBSD_Burkeville_Report_final.pdf.
Young, S.C., Ewing, T., Hamlin, S., Baker, E., and Lupton, D., 2012, Final report—Updating the hydrogeologic framework for the northern portion of the Gulf Coast aquifer system: Texas Water Development Board report, prepared by INTERA, Inc., and Frontera Exploration Consultants, 285 p., accessed August 25, 2022, at https://www.twdb.texas.gov/publications/reports/contracted_reports/doc/1004831113_GulfCoast.pdf.
Young, S.C., Kelley, V.A., Deeds, N., Hudson, C., Piemonti, D., Ewing, T.E., Banerji, D., Seifert, J., and Lyman, P., 2017, Report on the delineation of fresh, brackish and saline groundwater resources based on interpretation of geophysical logs: Harris-Galveston Subsidence District Scientific Research Report 2018–001, prepared by Intera, Inc., LBG-Guyton Associates, and the University of Texas Bureau of Economic Geology, 216 p., accessed August 25, 2022, at https://hgsubsidence.org/wp-content/uploads/2021/11/HGSD-Scientific-Report-2018-001_Report-on-the-Delineation-of-Fresh-Brackish-and-Saline-Groundwater -Resources-Based-on-Interpretation-of-Geophysical-Logs.pdf.
Young, S.C., Pinkard, J., Basset, R.L., and Chowdhury, A.H., 2014, Final report—Hydrogeochemical evaluation of the Texas Gulf Coast aquifer system and implications for developing groundwater availability models: Texas Water Development Board report no. 1148301233, prepared by INTERA, Inc., and Tetra Tech, 375 p., accessed August 25, 2022, at https://www.twdb.texas.gov/publications/reports/contracted_reports/doc/1148301233.pdf.
Datum
Vertical coordinate information is referenced to the North American Vertical Datum of 1988 (NAVD 88).
Horizontal coordinate information is referenced to the North American Datum of 1983 (NAD 83).
Abbreviations
>
greater than
≥
greater than or equal to
BCGCD
Brazoria County Groundwater Conservation District
DEM
digital elevation model
FBSD
Fort Bend Subsidence District
HGSD
Harris-Galveston Subsidence District
LSGCD
Lone Star Groundwater Conservation District
NAVD 88
North American Vertical Datum of 1988
NWIS
National Water Information System
USGS
U.S. Geological Survey
For more information about this publication, contact
Director, Oklahoma-Texas Water Science Center
U.S. Geological Survey
1505 Ferguson Lane
Austin, TX 78754–4501
For additional information, visit
https://www.usgs.gov/centers/ot-water
Publishing support provided by
Lafayette Publishing Service Center
Disclaimers
Any use of trade, firm, or product names is for descriptive purposes only and does not imply endorsement by the U.S. Government.
Although this information product, for the most part, is in the public domain, it also may contain copyrighted materials as noted in the text. Permission to reproduce copyrighted items must be secured from the copyright owner.
Suggested Citation
Ramage, J.K., and Braun, C.L., 2023, Status of water-level altitudes and long-term and short-term water-level changes in the Chicot and Evangeline (undifferentiated) and Jasper aquifers, greater Houston area, Texas, 2022: U.S. Geological Survey Scientific Investigations Report 2023–5007, 26 p., https://doi.org/10.3133/sir20235007.
ISSN: 2328-0328 (online)
Study Area
Publication type | Report |
---|---|
Publication Subtype | USGS Numbered Series |
Title | Status of water-level altitudes and long-term and short-term water-level changes in the Chicot and Evangeline (undifferentiated) and Jasper aquifers, greater Houston area, Texas, 2022 |
Series title | Scientific Investigations Report |
Series number | 2023-5007 |
DOI | 10.3133/sir20235007 |
Year Published | 2023 |
Language | English |
Publisher | U.S. Geological Survey |
Publisher location | Reston, VA |
Contributing office(s) | Oklahoma-Texas Water Science Center |
Description | Report: v, 26 p.; 2 Data Releases; Dataset |
Country | United States |
State | Texas |
City | Houston |
Online Only (Y/N) | Y |
Google Analytic Metrics | Metrics page |