Bathymetric, Hydrodynamic, Biological, and Water-Quality Characteristics of a Nearshore Area of the Laguna Madre Near South Padre Island, Texas, 2021–22
Links
- Document: Report (2.45 MB pdf) , HTML , XML
- Data Releases:
- USGS data release —Bathymetric data for a near-shore area of the Laguna Madre near South Padre Island, Texas, July 2021
- USGS data release —Hydrodynamic data for a near-shore area of the Laguna Madre near South Padre Island, Texas, August 2021 to April 2022
- USGS data release —Seagrass, benthic invertebrate, and water-quality data for a near-shore area of the Laguna Madre near South Padre Island, Texas, September 2021
- USGS water data for the Nation —U.S. Geological Survey National Water Information System database
- Download citation as: RIS | Dublin Core
Acknowledgments
This study was conducted in cooperation with the City of South Padre Island. Funding was provided by the National Oceanic and Atmospheric Administration Living Shorelines program through a grant to the City of South Padre Island. The authors gratefully acknowledge the assistance of Kristina Boburka and Erika Hughston from the Shoreline Department for the City of South Padre Island. The authors also wish to offer a special thanks to Mr. Rick Kalke of the Harte Research Institute at Texas A&M University Corpus Christi for his assistance with identifying benthic invertebrates.
The authors would also like to acknowledge assistance from U.S. Geological Survey colleagues MaryKate Higginbotham and Sarah Reynolds for documenting benthic invertebrates, Jaimie Ingold for enumerating seagrass shoot density, and George Umphres, Brian Petri, and Patrick Bryan for contributing their expertise in the field, often during adverse conditions.
Abstract
A variety of data were collected by the U.S. Geological Survey, in cooperation with the City of South Padre Island, to better understand the physical and biological habitat in Tompkins Channel and adjacent seagrass beds in the lower Laguna Madre, Texas, where the construction of berms has been proposed in the City of South Padre Island’s Shoreline Master Plan. These berms would be used to create living shorelines, defined as shorelines that “connect the land and water to stabilize the shoreline, reduce erosion, and provide ecosystem services, like [maintaining] valuable habitat, that enhances coastal resilience.” Bathymetric surveys show variability in the lagoon depth within the study area and clearly delineate the relatively shallow bay from the deeper Tompkins Channel. There were seasonal patterns in daily mean tidal water-surface elevations, with higher mean tides in the fall and spring and lower mean tides in the winter and summer. Seasonal differences in mean tidal water-surface elevations were primarily caused by astronomical forces. Shorter-term (daily and weekly) variations in tidal water-surface elevations were produced by meteorological forcing resulting from high- and low-pressure systems, the passage of fronts, local storms, and tropical storms. The tide driven currents typically were toward the north-northwest during flood tide or toward the south-southeast during ebb tide, roughly parallel to the general South Padre Island shoreline orientation. Prevailing water current velocity (current speed and direction) indicates that there usually is a net inflow into the lower Laguna Madre from the Gulf of Mexico and a net outflow at locations north of the study area. The overall seagrass density was generally lowest in the northeast section of the transect. Areas of low seagrass density were also most commonly observed close to the channel edge of the transect. Overall, Syringodium filiforme was considerably more abundant than Thalassia testudinum and Halodule wrightii. Amphipods from the families Ampithoidae and Melitidae were the most abundant benthic invertebrates, followed by gastropods in the family Calyptraeidae. Higher abundances of benthic invertebrates were recorded at sites farther away from Tompkins Channel than at sites closer to it, perhaps because of the sensitivity of these organisms to disturbances caused by erosional forces in the channel. Changes in water-quality measured during a 2-day survey were consistent with patterns observed in prior studies, indicating biological processes likely play an important role in the physicochemical environment of the study area. Collectively, the data provide baseline information that can be used as reference conditions prior to any shoreline enhancement activities in the lower Laguna Madre.
Introduction
South Padre Island, Texas, is a segment of the longest barrier island in the world (The Nature Conservancy, 2020) and is an integral part of the unique coastal system of south Texas (fig. 1). The island is between the western Gulf of Mexico and the Laguna Madre, which is one of only five hypersaline lagoons in the world (Javor, 1989). The Laguna Madre is divided physically into the upper Laguna Madre and lower Laguna Madre by a land bridge (specifically, a large mud flat) that extends from South Padre Island to the mainland. Upon completion of the Gulf Intracoastal Waterway in 1949, the upper and lower Laguna Madre were permanently connected by a dredged canal that came to be known as the Land Cut (Tunnel and others, 2001) (fig. 1).
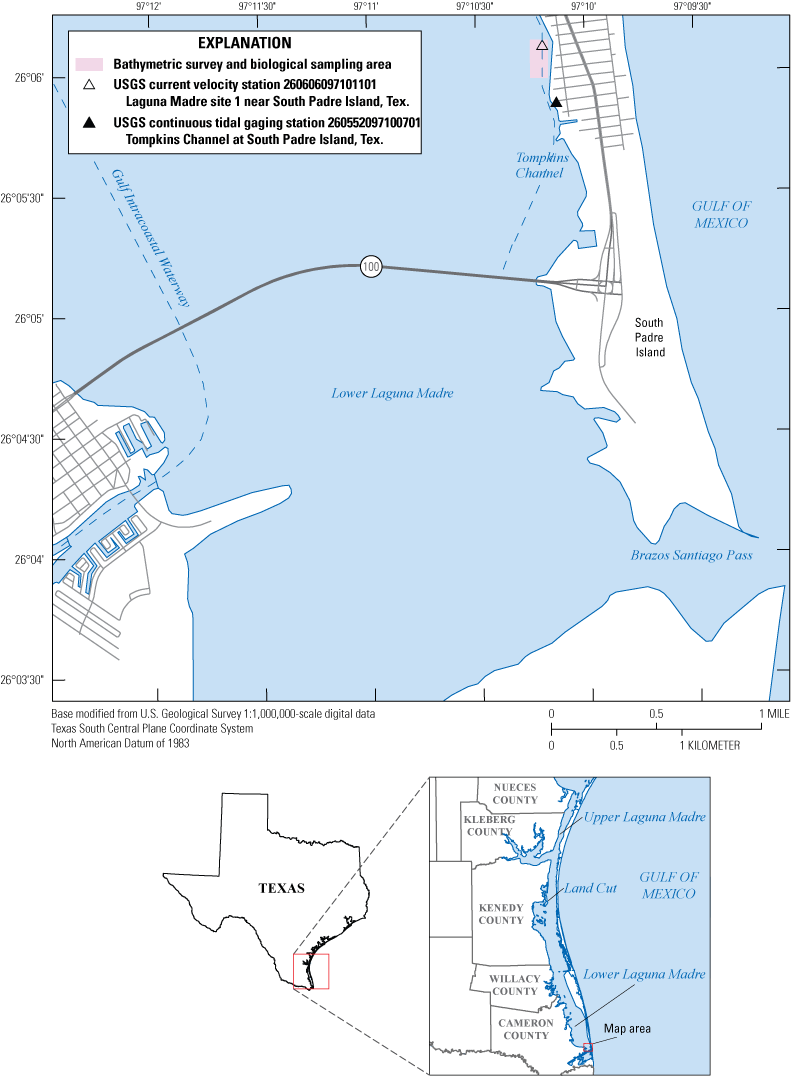
Hydrologic setting and study area on South Padre Island and the lower Laguna Madre, south Texas.
The region’s economy is largely supported by coastal recreational activities and by the Port of Brownsville, which contributes approximately 3 billion dollars annually in economic revenue for the State of Texas (Port of Brownsville, 2022). To protect and sustain this natural asset, the City of South Padre Island’s Shoreline Master Plan (City of South Padre Island, 2018) was developed to promote bay restoration and the development of a living shoreline. Living shorelines “connect the land and water to stabilize the shoreline, reduce erosion, and provide ecosystem services, like [maintaining] valuable habitat, that enhances coastal resilience” (National Oceanic and Atmospheric Administration [NOAA], 2022a, “What is a Living Shoreline” section, second paragraph).
South Padre Island is subject to many coastal risks that underscore the need for shoreline-conservation activities. As a barrier island, South Padre Island is subject to flooding and shoreline erosion during wind and storm surge events associated with tropical storms that sweep in from the south and during wind and large wave events associated with cold fronts that sweep in from the north or west; these storm events adversely affect the physical habitat and biological communities within the lower Laguna Madre (Morton and Holmes, 2009). In 2009, the City of South Padre Island began a program to rebuild dunes that were lost or damaged by Hurricanes Dolly and Ike. Increasing the size and extent of dunes and adding shoreline protection berms along the island beaches has proven to be an effective tool for minimizing erosion caused by tropical storm surges from the Gulf of Mexico (Ravella and others, 2012).
The bayside shoreline of South Padre Island is susceptible to erosional forces from both natural and human causes (Morton and Holmes, 2009). Strong cold fronts in the winter cause high wind velocities from the north and west, and the resulting large waves can adversely affect the bayside shoreline of South Padre Island. Large waves are common on the bayside shoreline throughout the lower Laguna Madre, where recreational and commercial boating activities abound. Habitat such as the seagrass beds in the lower Laguna Madre also are susceptible to the erosional forces associated with large waves, especially along deeper canals, and in waterways with frequent boat traffic and the dredging associated with the maintenance of those waterways.
Much of the lower Laguna Madre is covered by seagrasses that form the foundation of a highly productive aquatic ecosystem (Onuf, 2006). Seagrasses provide physical structure in the shallow waters of the lower Laguna Madre. The seagrass facilitates the settling of sediment and thus contributes to the characteristically high water clarity found throughout the lagoon (Short and Short, 1984). Seagrass blades are a substrate for the growth of nutritious epiphytic algae that support ecologically rich and diverse communities of invertebrate and vertebrate aquatic species (Kitting and others, 1984). Dense seagrass beds also serve as nursery grounds that offer sanctuary for juvenile fish and shellfish, including many commercially important fish (Rooker and others, 1998). The Laguna Madre provides the largest overwintering habitat in the world for the Aythya americana (redhead duck), which relies on the roots and rhizomes of the seagrass Halodule wrightii (shoalweed [commonly referred to as “shoalgrass”]) for food (Weller, 1964). Much of the region’s economy is dependent on outdoor recreational activities and commerce, both of which benefit from maintaining the healthy seagrass-based coastal ecosystem in the lower Laguna Madre. Quammen and Onuf (1993) compared vegetation maps of the lower Laguna Madre from the mid-1960s to the late 1980s and documented a 140-square-kilometer increase in bare seabed in areas that had previously been covered by seagrass; they reported that the loss in seagrass cover was confined to deeper parts of the lagoon and suggested the loss of seagrass was likely caused by maintenance dredging that was done to keep the deep-channel area passable for boat traffic (Quammen and Onuf, 1993).
A major component of the City of South Padre Island’s Shoreline Master Plan is the establishment of intertidal berms to create natural bulkheads that will reduce wave energy along the shoreline of the bay and decrease bay flooding from storm surges (Texas General Land Office, 2022). The proposed shoreline protection berms also are intended to support bay restoration efforts by improving habitat quality. For example, the placement of berms is intended to assist with the control of erosion caused by vessel-generated waves, a common phenomenon in intracoastal waterways during low tides (Sanchez, 2018); the Gulf Intracoastal Waterway is a heavily used corridor for barge and boat traffic. The berms are expected to facilitate development of additional wetland intertidal zones offering juvenile nurseries, foraging areas for migratory birds, and improved water quality (NOAA, 2022a; Ravella and others, 2012). To support the establishment of living shoreline habitat at South Padre Island, the U.S. Geological Survey (USGS), in cooperation with the City of South Padre Island, collected different types of data during 2021–22, including bathymetric, hydrodynamic, biological, and water-quality data. The bathymetric and hydrodynamic data were collected to help characterize the physical forces (tides and currents) that drive coastal erosion. Biological data were collected to help characterize seagrass density and benthic invertebrate community structure; these data serve as indicators of ecosystem health. To help characterize the water-quality, water-quality properties and sediment data were also collected.
Purpose and Scope
The purpose of this report is to provide an assessment of bathymetric, hydrodynamic (tidal and current), biological (seagrass and benthic invertebrate), and water-quality data, including sediment data to characterize recent (2022) baseline conditions in a 21-acre nearshore area of the Laguna Madre adjacent to the bayside shoreline of South Padre Island. Data were collected from shallow and deep parts of the study area. Summary statistics and data correlation metrics were determined for water-quality properties collected during the study. Determining the extent to which the data collected from the study were typical of the surrounding area within the lower Laguna Madre was beyond the scope of this study.
Data Availability
The sediment data (suspended-sediment concentration and sand-fine particle size separation data) produced during this study are available from the USGS National Water Information System (NWIS) (U.S. Geological Survey, 2022). The hydrodynamic data from August 19, 2021, through April 27, 2022, are available in a companion data release (Ockerman, 2022b). The remaining data are available from associated data releases describing the bathymetry (Ockerman, 2022a), seagrass abundance and distribution, benthic invertebrate abundance, and water-quality properties (Opsahl and others, 2022). Tidal water-surface elevation data from August 19, 2021, through August 19, 2022, are also available in NWIS.
Methods
Bathymetric, hydrodynamic (tidal water-surface elevation and current velocity), biological (seagrass and benthic invertebrate), and water-quality data (selected water-quality properties and suspended-sediment concentration and sand-fine particle size separation analyses) were collected during fall 2021 through spring 2022. Bathymetric data were collected from sites in the study area, a 21-acre nearshore area of the Laguna Madre, near South Padre Island, during July 13 and 15, 2021 (Ockerman, 2022a) (figs. 1 and 2). The study area includes a shallow-water area and a deep-channel area. The approximately 14-acre shallow area is farther from shore and ranges from about 1 to 4 feet (ft) deep, depending on tidal conditions. The approximately 7-acre deep-channel area is closer to shore and consists of a dredged channel approximately 6–11 ft deep (Tompkins Channel) that can accommodate boat traffic. The monitoring sites are on and adjacent to the western shoreline of South Padre Island in the shallow-water area and a deep-channel area waters of the lower Laguna Madre (fig. 1; table 1). Different bathymetric data-collection methods were used for the shallow and deep-channel areas.
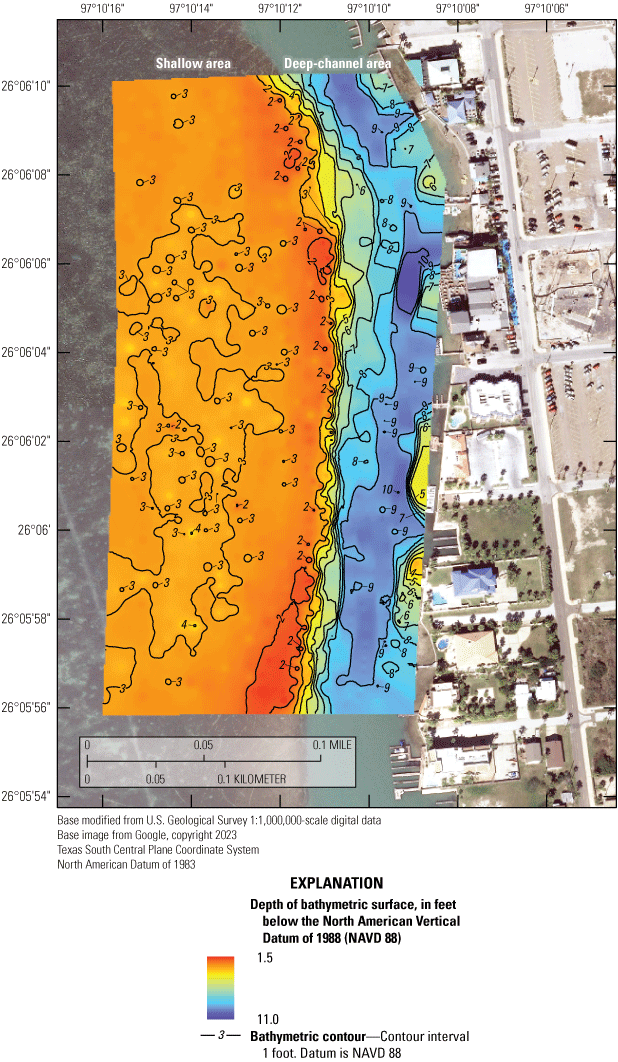
Bathymetry of the Laguna Madre study area, South Padre Island, Texas, July 13–15, 2021.
Table 1.
Site locations for U.S. Geological Survey (USGS) continuous monitoring tidal water-elevation station and current velocity station, Laguna Madre, South Padre Island, Texas, 2021–22.[dd, decimal degrees referenced to North American Datum of 1983]
Site type | USGS station number (fig. 1) | USGS station name (fig. 1) |
Latitude (dd) |
Longitude (dd) |
Data type |
---|---|---|---|---|---|
Tidal gaging station | 260552097100701 | Tompkins Channel at South Padre Island, Tex. | 26.09792 | −97.16883 | Tidal water-surface elevation |
Current velocity station | 260606097101101 | Laguna Madre Site 1 near South Padre Island, Tex. | 26.10181 | −97.16986 | Current velocity |
Shallow-area data were collected July 13–15, 2021, by using a Real Time Network (RTN) Global Navigation Satellite System (GNSS), which included a Leica rover antenna that uses network reference station data and navigation satellite data to determine real-time horizontal and vertical position (Leica Geosystems, 2010). The rover antenna was mounted on a 2-meter rod and placed directly from the boat onto the bay bottom to measure the bottom elevation.
Before each measurement, the boat was positioned and anchored to the seabed to secure the boat from currents for steady positioning of the GNSS antenna. Measurements were collected at a spatial density of approximately one measurement per 850 square feet of area. Measurements were distributed roughly uniformly over the shallow area. Each measurement was an instantaneous (1-second) measurement. The general survey quality is classified as a level IV survey (Rydlund and Densmore, 2012). The GNSS system used was a Leica Viva series receiver (GS14 antenna) and Data Collector (CS20 Field Controller). The Leica GNSS system provides outputs of the estimated coordinate quality (CQ) associated with each measured position. There are three CQ outputs, expressed as distance (Leica Geosystems, 2010):
-
• CQ3D—three-dimensional coordinate quality of reported position
-
• CQ2D—two-dimensional (horizontal) coordinate quality of reported position
-
• CQ1D—one-dimensional (vertical) coordinate quality of reported position
Deep-channel area data were collected by using an RTN GNSS Trimble R10 rover antenna (Trimble Navigation Limited, 2015) connected to an underwater SonarMite single-beam echo sounder (Ohmex Instrumentation, 2016). The rover-echo sounder system was deployed from a moving boat used to collect bathymetric data along transects parallel to the channel. Measurements were collected at a density of approximately 1 measurement per 190 square feet of area. However, measurements were not uniformly distributed but, rather, concentrated in transects oriented north-south, parallel to the channel direction. Data collection was more concentrated where the channel began to deepen to provide greater resolution in the transition area between the deeper channel and the shallow bay. This transition area between the deeper channel and the shallow bay will be altered during berm placement, and greater bathymetric detail will help guide this process (City of South Padre Island, 2018). Each measurement was an instantaneous (1-second) measurement. The GNSS system used to collect data in the deeper-channel area was a Trimble GNSS system (R10 antenna and TSC7 Field Controller) and SonarMite v. 5 echo sounder. The Trimble system (Trimble Navigation Limited, 2015) provides outputs of the estimated horizontal and vertical precision of each measured point (Rydlund and Densmore, 2012). Measured echo-sounder depths are referenced to the Trimble antenna measure point. Those depths were subtracted from the GNSS measured elevation to determine the elevation of the seabed referenced to the North American Vertical Datum of 1988 (NAVD 88).
Error estimates of individual echo-sounder depth measurements were not available. Manufacturer estimates of echo-sounder accuracy are 1 centimeter, or 0.1 percent of measured depth. Coordinate accuracy of the Trimble GNSS measurements (estimated horizontal precision and vertical precision of each measured point) are considered indicative of the overall coordinate accuracy of the deep-channel area data.
Survey data were used as inputs for an interpolation process to create a digital elevation model surface. This process creates a gridded continuous surface, with each grid cell containing a bottom elevation value. Surveyed values were used as inputs, and various methods were applied to estimate elevation values in areas where elevation was not measured. The bathymetric surface raster was constructed by means of inverse distance-weighted interpolation as applied through the Spatial Analyst toolbox extension of Esri’s ArcGIS desktop software (Esri, 2022).
Summary statistics were computed for the water-quality properties for each of the two days of sampling (minimum, maximum, median, and mean values). The Spearman's rank correlation coefficient (Helsel and others, 2020) was used to determine the strength and direction of the relation between each water-quality property and the local time (hours and minutes of the day) when a given water-quality property was collected.
Instantaneous RTN GNSS measurements using the real-time Leica equipment were made at a reference mark established on the seawall adjacent to the study area. The elevation of the reference mark was determined from a separate level II GNSS survey, which has a precision of 0.03 ft and an uncertainty greater than or equal to 0.1 ft (Rydlund and Densmore, 2012). The instantaneous GNSS measurements each were made before and after the daily survey of bathymetric points using the Leica GNSS system on July 15, 2021. The mean elevation of four measurements (two measurements before and two measurements after the daily bathymetric data collection) was −0.008 ft different from the elevation determined from the level II survey.
To test for consistency between the two GNSS measurement systems, a one-time (July 13, 2021), in situ comparison of the Leica GNSS measurements and the Trimble/SonarMite GNSS measurements was made on a stable test surface established at a location in the shallow bay area. A large flat rock served as a temporary monument and was placed on the bay bottom at a depth of about 2.5 ft. Measurements of the elevation of the top of this surface were made with each of the GNSS (Leica and Trimble/SonarMite) systems and are available in Ockerman (2022b). The difference between two measurements made with each system was 0.01 ft and indicated measurements made by the two GNSS systems were consistent with each other.
Continuous (15-minute interval) tidal water-surface elevation data were collected at USGS station 260552097100701, Tompkins Channel at South Padre Island, Tex. (Ockerman, 2022b) (fig. 1; table 1) between August 19, 2021, and August 19, 2022. Tidal water-surface elevation data were collected by using a nonsubmersible pressure transducer referenced to the North American Vertical Datum of 1988 (NAVD 88). Data were recorded every 15 minutes, transmitted hourly to the Geostationary Operational Environmental Satellite, and downloaded to the NWIS database (U.S. Geological Survey, 2022). The site was visited periodically to verify the recorded stage was accurate (Rantz and others, 1982; Turnipseed and Sauer, 2010). The tidally filtered daily mean stage was calculated to help detect any long-term trends by using the Godin filter to remove tidal signals (the effects of daily tides on the recorded stage) (Godin, 1972).
Current velocity (speed and direction) data were collected from August 19, 2021, to April 27, 2022, at USGS station 260606097101101, Laguna Madre Site 1 near South Padre Island, Tex., approximately 300 ft west of the shoreline (Ockerman, 2022b) (fig. 1; table 1). Current data were collected by using a Nortek ECO acoustic Doppler current profiler (ADCP) (Nortek, 2022) during six deployments. In between each deployment, the ADCP was cleaned, the data were downloaded, and the instrument battery was recharged. Between deployments there were gaps of missing data that ranged from 12 to 24 hours. All water elevations were referenced to NAVD 88. The ADCP measured current speed and direction hourly at three different water elevations: 0.49 ft (lower elevation), 0.98 ft (middle elevation), and 1.48 ft (upper elevation) above the top of the ADCP (the top of the ADCP was set just above the seabed at an elevation of −1.4 ft, NAVD 88) (Ockerman, 2022b).
To inventory the extent of biological resources in the study area, an array of eight transects with three biological-sampling sites along each transect was established by using a hand-held Global Positioning System unit. The location of the transects coincides with the planned location of berms that will be constructed in association with the City of South Padre Island. The fixed grid of eight transects with three sampling locations on each transect, all evenly spaced, was used to provide adequate coverage for representing the biological communities within the study area.
Three 10-centimeter-diameter cores were collected at each station for a total of 72 cores. Seagrass shoot abundance in each core was determined by sieving all sediment from plant material, separating live plant material by species, and counting the total live above-ground shoot numbers according to species (Onuf and Ingold, 2007). Each core was visually inspected for seagrass species, which, for this survey, included Syringodium filiforme (manatee grass), Thalassia testudinum (turtlegrass), and Halodule wrightii (shoalgrass) and the abundance of each type was recorded. The abundance of the three seagrass species in each core was recorded.
Benthic invertebrate samples were collected concurrently with seagrass samples to assess abundance and determine which species were present. The benthic macroinvertebrate samples were collected from the sediment surface by using a Petite Ponar sampler (U.S. Environmental Protection Agency, 2020). After the samples were collected, they were placed into a plastic 17.5-liter holding tote box on a metal culling board. The samples were then strained through a 2.00-millimeter (mm), size 10 mesh sieve, and any visible organisms were placed into site-specific sampling bottles. The remaining water, sediment, vegetation, and organisms were then strained again through a 0.45-mm, size 35 mesh sieve to ensure organisms that were not readily visible or attached to vegetation were collected (U.S. Environmental Protection Agency, 2016). Benthic samples were preserved on site in a solution of 70-percent ethyl alcohol.
Benthic macroinvertebrate samples were further processed after returning from the field. The larger specimens were sorted by hand prior to the use of additional sample sieving procedures. The remaining vegetation and sediment were rinsed into the 17.5-liter holding tote boxes positioned above the size 35 sieve and subsequently the size 10 sieve to isolate the smaller specimens, which were collected with forceps and added to the respective sampling bottles for each site. Individuals were isolated on an Olympus SZX16 stereo microscope for photographs and identification. Individuals were identified by use of a microscope and online photographs and resources including those from Harte Research Institute for Gulf of Mexico Studies (2022), Bowling (2021), Global Biodiversity Information Facility (2022), WoRMS Editorial Board, (2022), and Barcode of Life Data Systems (2022). Individuals that could not be identified by means of photographs and available resources were sent for identification by researchers at the Harte Research Institute at Texas A&M University Corpus Christi. The family, genus, and species of each individual invertebrate were recorded. All individuals in each sample were identified to avoid skewed data that could result from random subsampling of benthic samples with few invertebrate individuals.
All individuals identified down to the species level were verified by using information from the Integrated Taxonomic Information System (ITIS) database (ITIS, 2022). The results are included in the “Bathymetric, Hydrodynamic, Biological, and Water-Quality Characteristics” section of this report and in the companion USGS data release (Opsahl and others, 2022). Individuals were identified down to the family or genus level by using information from ITIS. If individuals were missing head capsules or were damaged, they were not identified at the family level; however, they were included in the dataset because they represented additional benthic invertebrates that were present in each sample.
Water-quality measurements were made at each station (fig. 1; table 1) before samples were collected for seagrass and benthic invertebrate species identification and abundance quantification. Water depth, temperature, specific conductance, dissolved oxygen, pH, and turbidity were measured before and after the biological samples were collected. A YSI EXO2 sonde (Xylem, 2023) was used to make the water-quality measurements onsite following USGS guidelines (U.S. Geological Survey, variously dated). Ancillary water-quality information, including air temperature, wind speed, and wind direction, were measured by using a Kestrel 5500 weather meter (Kestrel, 2022). Air temperature, wind speed, and wind direction are recognized drivers of water quality in Gulf of Mexico estuaries (McCarthy and others, 2018; Paul and others, 2019).
Suspended-sediment grab samples were collected during November 2021–May 2022 following standard USGS methods described in Edwards and Glysson (1999). A total of nine environmental samples and one replicate were collected and analyzed by the USGS New Mexico Sediment Laboratory for sediment mass, sediment concentration, sediment mass greater than 0.0625 millimeter (mm) (sand-sized particles), and sediment mass less than 0.0625 mm (silt- and clay-sized particles) using methods described in Guy (1969). Tidal conditions were noted at the time of sample collection.
Bathymetric, Hydrodynamic, Biological, and Water-Quality Characteristics
Understanding the interplay of bathymetry within the study area and driving forces, such as wind and boat traffic, provides a framework for evaluating the type and configuration of any berms that might be constructed to reduce shoreline erosion. The bathymetric surveys show variability in bottom contours within the study area and clearly delineate the relatively shallow area from the deep-channel area known as the Tompkins Channel (fig. 2). Depths for the shallow bay survey ranged from 1.46 to 4.70 ft (median depth 2.87 ft) with progressively shallower elevations located westward of, and farther away from, Tompkins Channel. The depth range reported from the shallow bay survey of the seagrass beds near Tompkins Channel is typical of much of the lower Laguna Madre (Onuf, 2006). Depths for the deep-channel area survey ranged from 2.67 to 11.01 ft (median depth 7.81 ft), with the deepest values near the centroid of Tompkins Channel. The sharp dropoff that occurs at the interface of shallow bay area and deep-channel area of Laguna Madre (Tompkins Channel) is controlled by periodic dredging of the deep-channel area to support boat travel.
The bathymetry surveys provide context for understanding seasonal variations in shoreline erosion. In warmer months, the predominant wind direction is from the southeast, and the western side of South Padre Island along Tompkins Channel is protected from the formation of waves and wind-driven erosion under these conditions. The distance travelled by wind over open water, across Tompkins Channel to the seagrass beds, is relatively small (approximately 200–300 ft), and this reduces the formation of large wind-driven waves reaching the edge of the seagrass beds during periods of southeast winds. In contrast, winds from the north and west in the cooler months generate much larger waves on the eastern side of the lower Laguna Madre that cause erosional problems on the western shore of South Padre Island. In addition to wind-driven waves, the waves caused by commercial and recreational boating also contribute to erosion on the western side of South Padre Island.
Tidal water-surface elevations in the Laguna Madre are important in geomorphological and biological processes; notably, meteorological forces (wind, air pressure, and weather patterns) are more influential on tides than astronomical forces (solar and lunar gravity) (Rusnak, 1960). Between August 19, 2021, and August 19, 2022, tidal water-surface elevations at the Tompkins Channel station ranged from −1.64 ft to 1.93 ft (NAVD 88), with a mean of 0.26 ft (fig. 3). Tidally filtered daily means (Godin, 1972) ranged from −0.70 to 1.2 ft (NAVD 88), with a mean of 0.26 ft (fig. 3). The tidal water-surface elevation range in the study area is smaller in magnitude compared to that of the adjacent Gulf of Mexico because tidal forces from the Gulf of Mexico are dampened as they enter the relatively shallow lower Laguna Madre through nearby tidal inlets, including Brazos Santiago Pass (NOAA, 2022b) (fig. 1). Daily mean tidal water-surface elevations changed seasonally and were highest in fall 2021 and spring 2022, peaked in October, and lowest values were observed in winter and summer 2022. These seasonal patterns of generally higher tidal water-surface elevations in the fall and lower elevations in winter and summer are consistent with previous observations of tidal water-surface elevations in Laguna Madre (Smith, 1978) and are driven primarily by astronomical forces. Shorter-term (daily and weekly) variations in tidal water-surface elevations are produced from meteorological forcing caused by high- and low-pressure systems, the passage of fronts, local storms, and large tropical storms, including hurricanes. During these times, astronomical tides appear as relatively minor features in tidal water-surface elevations. Overall, tidal patterns in the lower Laguna Madre are characterized as having diurnal, semidiurnal, and mixed tide cycles, which may include one high and one low tide of nearly equal magnitude per lunar day, two high and two low tides per lunar day, or an irregular pattern of high and low tides of different magnitudes, respectively (Gill and others, 1995). These patterns, in turn, are superimposed on the larger seasonal temporal pattern caused by astronomical factors. Although tidal patterns in the lower Laguna Madre are difficult to predict given the complexity of the various forces involved, observations from the Tompkins Channel station are consistent with nearby tidal measurements made at the Brazos Santiago Pass (NOAA, 2022b) and accurately reflect local tidal conditions near the study area.
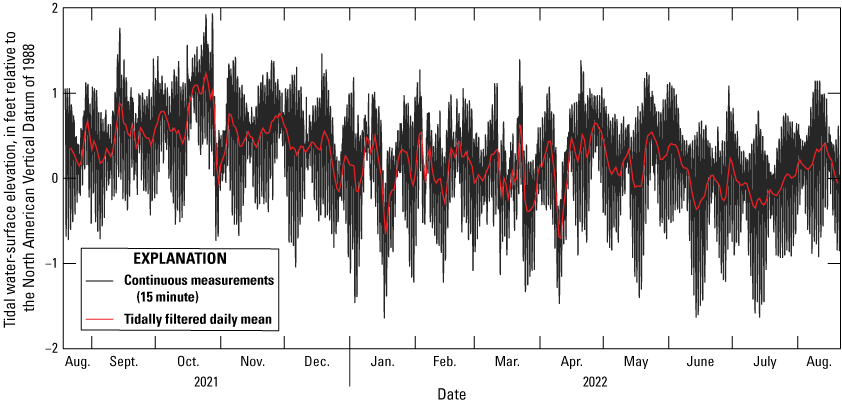
Continuous (15-minute) and tidally filtered daily mean tidal water-surface elevations measured at the Tompkins Channel at South Padre Island, Texas (U.S. Geological Survey gaging station 260552097100701), August 19, 2021−August 19, 2022.
Water-current velocity (speed and direction) affects seagrass bed health. Water-current velocity affects the transport of nutrients, sediment stability, water chemistry, and seagrass canopy configuration (Fonseca and others, 2019). ADCP depth-averaged water-current velocity measurements depict the integrated water-column motion (fig. 4). Water speed ranged from 0 to 1.67 feet per second (ft/s). The tide-driven currents typically were toward the north-northwest, between 315° and 360° (which corresponds to 0° on fig. 4), during flood tide into the Laguna Madre, or toward the south-southeast, between 135° and 180°, during ebb tide when water flows out of the Laguna Madre (roughly parallel to the general South Padre Island shoreline orientation). During this study, the maximum flood tide current was 1.67 ft/s, whereas the maximum ebb tide current was 1.28 ft/s (fig. 4). The shoreline trends northwest-southeast in the study area. The speed of currents parallel to the shoreline was typically more than 0.6 ft/s, whereas the speed of currents that were not parallel the shoreline was typically less than 0.6 ft/s. Overall, the prevailing water current velocity (current speed and direction) determined during this study coincides with the findings of previous studies (East and others, 1998; Hedgpeth, 1967), indicating that there is a net inflow into the lower Laguna Madre from the Gulf of Mexico and a net outflow at locations north of the study area.
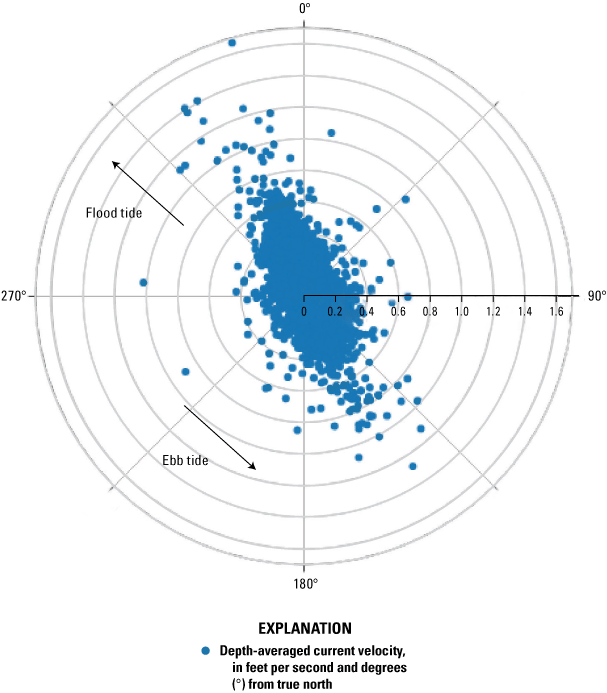
Scatterplot in polar coordinates depicting depth-averaged current velocity (current speed and direction), in degrees from true north, at the Laguna Madre Site 1 near South Padre Island, Texas (USGS station 260606097101101), August 18, 2021–April 27, 2022.
Understanding the distribution and abundance of seagrass in areas adjacent to dredged channels such as Tompkins Channel can aid evaluation of the effectiveness of management actions related to shoreline stability. The seagrass survey conducted within the study area documented the presence of three seagrass species: Syringodium filiforme, Halodule wrightii, and Thalassia testudinum (fig. 5; table 2). Shoot counts for individual species indicate that Syringodium filiforme was considerably more abundant in the study area than Thalassia testudinum or Halodule wrightii (fig. 5B–D). Syringodium filiforme shoot counts ranged from 0 to 20.8 shoots per 100 square centimeters (cm2), whereas shoot counts ranged from 0 to 4.67 and 0 to 14.9 shoots per 100 cm2 for Thalassia testudinum and Halodule wrightii, respectively. Median shoot counts were considerably higher for Syringodium filiforme (12.1 shoots per 100 cm2) compared to 1.70 and 0 shoots per cm2 for Thalassia testudinum and Halodule wrightii, respectively. Although Halodule wrightii was particularly abundant at site 6C, there were no Halodule wrightii shoots at more than half the sites, resulting in a median value of 0 shoots per 100 cm2 and making Halodule wrightii the least abundant seagrass species on an overall basis.
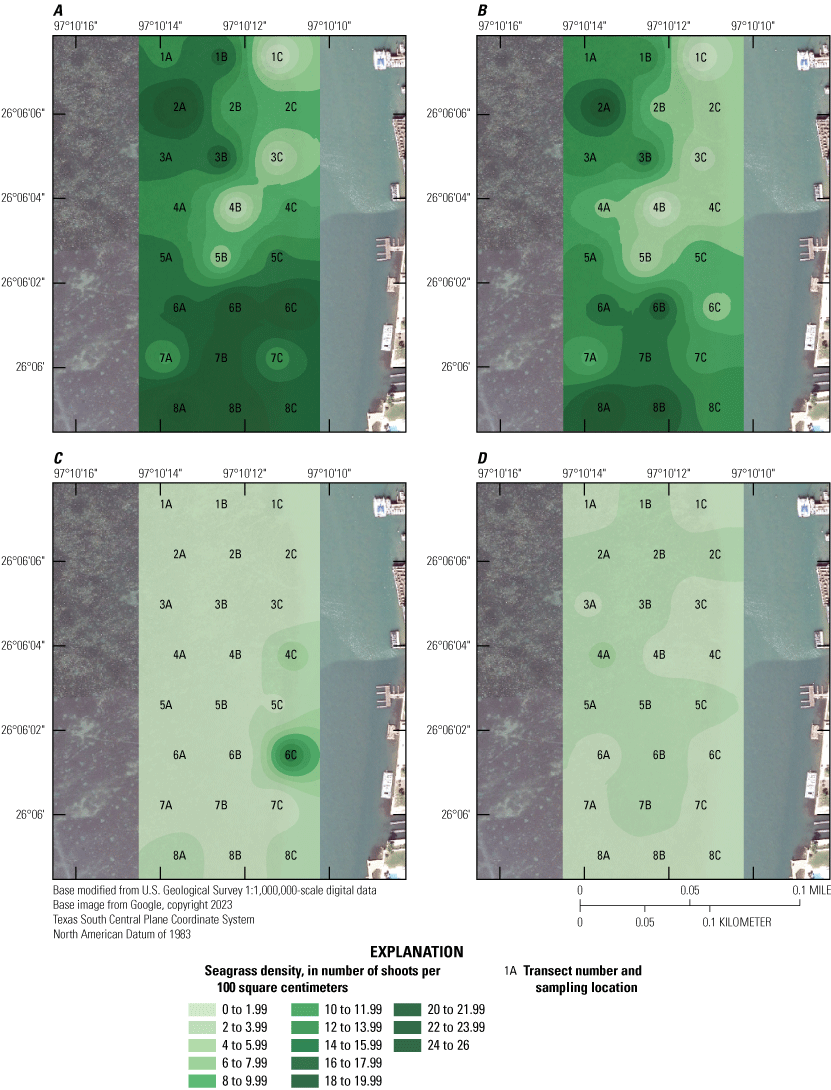
Seagrass shoot density shown within the transect study area for A, summation of all species, B, Syringodium filiforme, C, Halodule wrightii, and D, Thalassia testudinum.
Table 2.
Transect site information, seagrass data, and benthic invertebrate data from U.S. Geological Survey transect surveys in the Laguna Madre, South Padre Island, Texas, September 8–9, 2021.[Dates shown as month, day, year. dd, decimal degrees referenced to North American Datum of 1983; cm2, square centimeter; NA, not applicable]
Combining shoot data from all three species provides an overview of the distribution of seagrass in the study area. The combined sum of shoot counts from all three species ranged from 0 to 25.5 shoots per 100 cm2 (fig. 5A; table 2). Seagrass density was generally lowest in the northeast section of the transect. Areas of low seagrass density were also most commonly observed close to the channel edge of the transect. Overall, Syringodium filiforme was considerably more abundant than Thalassia testudinum and Halodule wrightii. The results of this seagrass survey document recent (2022) conditions for the area of interest along Tompkins Channel where berms may be placed to reduce shoreline erosion.
A total of 366 individual organisms were identified as benthic invertebrates. There were 18 individuals that could not be identified at the family level. For the remainder of the sample set, 32 different families were represented. Amphipods were the most abundant group, with the family Ampithoidae accounting for 113 of the total number of individuals, Melitidae accounting for 50 of the total number of individuals, and Calyptraeidae (gastropods) accounting for 24 of the total number of individuals. In many instances, organisms were not identified down to the genus or species level, although 36 different genera and 34 different species were documented. The total number of individuals and taxonomic identification of each are documented in Opsahl and others (2022).
The total number of individuals was used to assess the spatial distribution of benthic invertebrates within the study area (fig. 6; table 2). Numbers of individuals among sites ranged from 0.65 to 14.4 individuals per 100 cm2. Higher abundances were recorded at sites farther away from Tompkins Channel and farther south within the study area. A lower density of individuals was evident in the northeastern part of the study area. Lower numbers of benthic invertebrates near Tompkins Channel could result from disturbances in the channel. Benthic invertebrates in the Laguna Madre were reported to be sensitive to disturbances from dredging and recovered more slowly than seagrass and fish communities (Sheridan, 2004). Thus, benthic invertebrates could serve as good indicator organisms to evaluate the effects of activities related to shoreline erosion that are being considered at or near the study area.
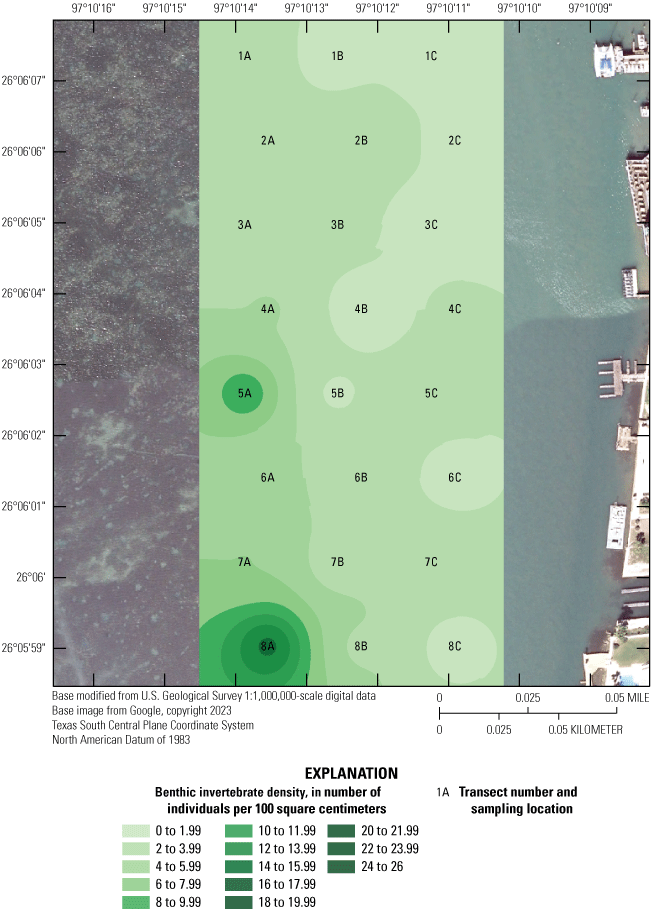
Benthic invertebrate density based on the total number of all individuals shown within the transect study area.
Water-quality data were collected to help characterize the physicochemical framework in which biological communities exist. Temperatures during the 2-day survey (September 8–9, 2021), ranged from 29.4 to 32.5 degrees Celsius (°C; median 30.8 °C), (table 3). Specific conductance provides an indirect measure of salinity because it represents the sum of dissolved ions in solution (Hem, 1982; 1985); specific conductance ranged from 55,200 to 56,000 microsiemens per centimeter at 25 degrees Celsius (µS/cm at 25 °C; median 55,500 µS/cm at 25 °C). Specific conductance was high for saline waters (with most seawater measuring approximately 35,000 µS/cm at 25 °C), consistent with the hypersaline conditions characteristic of the lower Laguna Madre (Javor, 1989). pH ranged from 7.1 to 9.0 (median 8.5), indicating slightly basic conditions characteristic of seawater (NOAA, 2018). The dissolved oxygen concentration ranged from 2.9 to 10.3 milligrams per liter (mg/L; median 6.4 mg/L), indicating conditions ranging from less than 50 percent saturation to near 100 percent saturation can occur in the study area. Turbidity values ranged from 1 to 34 nephelometric turbidity units (median 10.4 nephelometric turbidity units) indicating relatively low turbidity in the waters of the lower Laguna Madre during calm conditions (Short and Short, 1984). Overall, the water-quality data collected during the 2-day survey for this assessment were consistent with hypersaline calm water conditions.
Table 3.
Summary statistics for select water-quality parameters from the U.S. Geological Survey water-quality transect survey in the Laguna Madre, South Padre Island, Texas, September 8–9, 2021.[HH, hours; MM, minutes; UTC, coordinated universal time; NA, not applicable; n.d., not determined]
Given the small size and relatively shallow water in the study area, characterizing the possible causes of any spatiotemporal variations in water-quality properties was not possible. Previous studies have indicated that spatiotemporal variations in water-quality properties are often driven by diurnal processes and biological activity (Ziegler and Benner, 1998). Where water-quality measurements were made, the water was shallow (4 ft deep or less) and likely well-mixed. Hence, measurements made at individual sampling points in the shallow part of the study area were assumed to be comparable. Changes over the course of the 6 hours that were sampled on both day 1 and day 2 were evident for all constituents except turbidity (table 3). Increases in temperature between midmorning and midafternoon were not surprising, given there were no large shifts in weather conditions during this 2-day survey. Increases in pH and corresponding increases in dissolved oxygen are consistent with increased plant productivity, primarily by seagrasses as well as algae and phytoplankton (Ziegler and Benner, 1998). As sunlight increases, photosynthetic processes in seagrasses become active, resulting in the uptake of dissolved carbon dioxide and production of dissolved oxygen.
Suspended-sediment concentration data and sand-fine size separation data obtained by separating the sand-size suspended sediment from fine (silt and clay) sized suspended sediment (Guy, 1969) provides insights into water-quality conditions within the lower Laguna Madre. Suspended sediment concentrations in this study ranged from 2 to 28 mg/L (table 4). The low concentrations and narrow range in sediment concentrations are consistent with calm conditions within the Laguna Madre at the time of sampling; notably, no samples were collected during periods of high winds or cold fronts. Sand-sized particles are greater than or equal to 0.0625 mm in diameter or larger, whereas fine-sized particles are less than 0.0625 mm in diameter (Guy, 1969). The percentage of fine-sized suspended sediment ranged from 55 to 89 percent. Sand-sized particles are heavier than fine-sized particles, so the high percentages of fine-size particles are not surprising given the relatively calm conditions. Wind and currents are major factors that can cause resuspension and redistribution of sediments from the bottom of the bay system. New sources of sediment can also be introduced into the Laguna Madre from inland sources or by inflows of water from the Gulf of Mexico that transport suspended material from offshore sources (Morton and others, 2000). A loss of suspended material may occur through transport processes causing water and its associated load of suspended material to leave the system through exchanges with the Gulf of Mexico or by transport into Corpus Christi Bay (Morton and others, 2000). Human factors associated with dredging and boat traffic also influence suspended-sediment dynamics. Changes in suspended-sediment concentrations associated with human activities is an important variable that can be used to differentiate and evaluate the effects of management practices when establishing stabilizing structures such as intertidal berms within the Laguna Madre system.
Table 4.
Suspended-sediment concentration and summary statistics for samples collected at Laguna Madre Site 1 near South Padre Island, Texas (U.S. Geological Survey station 260606097101101).[Dates shown as month, day, year. HH, hours; MM, minutes; UTC, coordinated universal time; <, less than; ≥, greater than or equal to; mg/L, milligram per liter; mm, millimeter; R, replicate sample not used in calculation of summary statistics; NA, not applicable; n.d., not determined]
Implications for Shoreline Conservation Activities
The data in this report were collected from Tompkins Channel and adjacent seagrass beds in the lower Laguna Madre to support efforts to protect and restore shoreline habitat and resources as they relate to the construction of a living shoreline. The types of data described in this report are intended for use in shoreline conservation activities such as determining the optimal size and shape of any berms that might be constructed to reduce shoreline erosion caused by waves. Bathymetric data from the study area were used to create a high-resolution map of the bottom topography of the study area to provide needed information about the physical structure of the areas selected for berm placement. Tide and current data describe the velocity (speed and direction) of water movement in the shallow seagrass beds and can be used to help understand and predict transport and deposition of suspended sediment during periods of disturbance. Seagrass shoot density, diversity and abundance of benthic invertebrates, and water quality were evaluated as metrics to enumerate biological characteristics of most importance to maintaining ecosystem community structure in areas undergoing disturbance associated with dredging and deposition of dredged material. Collectively, the data provide recent (2022) baseline information that can be used for reference prior to any new shoreline conservation activities.
Summary
South Padre Island, Texas, is a segment of the longest barrier island in the world and is an integral part of the unique coastal system of south Texas. The Laguna Madre extends from South Padre Island to the mainland and is one of only five hypersaline lagoons in the world. As a barrier island, South Padre Island is subject to flooding and erosion of its shorelines during wind and storm surge events; these storm events adversely affect the physical habitat and biological communities within the lower Laguna Madre. The City of South Padre Island’s Shoreline Master Plan was developed to promote bay restoration and the development of living shorelines—shorelines that “connect the land and water to stabilize the shoreline, reduce erosion, and provide ecosystem services, like [maintaining] valuable habitat, that enhances coastal resilience.” To improve and protect habitats, the City of South Padre Island’s Shoreline Master Plan proposed to establish intertidal berms to reduce wave energy and reduce erosion.
Bathymetric and hydrodynamic data were collected by the U.S. Geological Survey, in cooperation with the City of South Padre Island, to characterize physical forces that drive coastal erosion. Bathymetric surveys clearly delineate the sharp change in elevation from the shallow bay to the deep area associated with Tompkins Channel maintained by periodic dredging to support boat traffic in the channel. Seasonally, the seagrass beds in the shallow bay reduce the formation of large wind-driven waves and associated erosion on the western shoreline of South Padre Island. Tidal water-surface elevations affected by astronomical forces exhibit a seasonal pattern with higher elevations in fall and spring and lower elevations in winter and summer. Superimposed onto the large seasonal tidal water-surface elevation pattern are smaller variations that are due to meteorological forcing caused by high- and low-pressure systems, as well as the passage of fronts, local storms, and tropical storms. Tidally driven currents roughly parallel the South Padre Island shoreline, typically toward the north-northwest during flood tide and toward the south-southeast during ebb tide. Prevailing water current velocity (current speed and direction) data indicate a net inflow into the lower Laguna Madre from the Gulf of Mexico and a net outflow north of the study area.
Biological data and water-quality properties were collected as indicators of ecosystem health. Seagrass and benthic invertebrate densities were generally lower closer to the deep-channel area and higher farther away from the channel edge. Syringodium filiforme was considerably more abundant than Thalassia testudinum and Halodule wrightii. Amphipods from the families Ampithoidae and Melitidae were the most abundant benthic invertebrates, followed by gastropods from the family Calyptraeidae. Higher abundances of benthic invertebrates were recorded at sites farther away from Tompkins Channel, perhaps because of their sensitivity to disturbances from erosional forces in the channel. Water-quality data collected during the 2-day survey for this assessment were consistent with hypersaline calm water conditions featuring low concentrations of suspended sediment. This report provides recent (2022) baseline physical characteristics and ecosystem health indicators that inform shoreline conservation activities.
References Cited
Barcode of Life Data Systems, 2022, BOLD systems—Taxonomy browser: Barcode of Life Data Systems database, accessed January 4, 2022, at https://boldsystems.org.
Bowling, B., 2021, Identification guide to marine organisms of Texas: Marine Species Identification Project website, accessed November 24, 2021, at https://txmarspecies.tamug.edu/index.cfm.
City of South Padre Island, 2018, South Padre Island Shoreline Master Plan: South Padre Island, Texas, City of South Padre Island, 134 p., accessed October 18, 2022, at https://www.myspi.org/egov/documents/1590608913_55466.pdf.
East, J.W., Solis, R.S., and Ockerman, D.J., 1998, Computed discharges at five sites in lower Laguna Madre near Port Isabel, Texas, June 1997: U.S. Geological Survey Fact Sheet 167–98, 6 p., accessed March 28, 2023, at https://doi.org/10.3133/fs16798.
Edwards, T.K., and Glysson, G.D., 1999, Field methods for measurement of fluvial sediment (revised 1999): U.S Geological Survey Techniques of Water-Resource Investigations, book 3, chap. C2, 89 p., accessed March 28, 2023, at https://doi.org/10.3133/twri03C2.
Esri, 2022, ArcMap—IDW, overview of the raster interpolation toolset: Esri ArcGIS for Desktop web page, accessed November 30, 2022, at https://desktop.arcgis.com/en/arcmap/10.3/tools/3d-analyst-toolbox/idw.htm.
Fonseca, M.S., Fourqurean, J.W., and Koehl, M.A.R., 2019, Effect of seagrass on current speed—Importance of flexibility vs. shoot density: Frontiers in Marine Science, v. 6, no. 376, 13 p., accessed December 22, 2022, at https://doi.org/10.3389/fmars.2019.00376.
Gill, S.K., Hubbard, J.R., and Dingle, G., 1995, Tidal characteristics and datums of Laguna Madre, Texas: National Oceanic and Atmospheric Administration Technical Memorandum NOS OES 008, 57 p., accessed December 22, 2022, at https://repository.library.noaa.gov/view/noaa/2667.
Global Biodiversity Information Facility, 2022, Free and open access to biodiversity data: Global Biodiversity Information Facility database, accessed January 3, 2022, at https://www.gbif.org.
Guy, H.P., 1969, Laboratory theory and methods for sediment analysis: U.S. Geological Survey Techniques of Water-Resources Investigations, book 5, chap. C1, 58 p., accessed March 28, 2023, at https://doi.org/10.3133/twri05C1.
Harte Research Institute for Gulf of Mexico Studies, 2022, Gulfbase—Search the database: Corpus Christi, Texas, Texas A&M University, Harte Research Institute for Gulf of Mexico Studies database, accessed January 3, 2022, at https://www.gulfbase.org.
Helsel, D.R., Hirsch, R.M., Ryberg, K.R., Archfield, S.A., and Gilroy, E.J., 2020, Statistical methods in water resources: U.S. Geological Survey Techniques and Methods, book 4, chapter A3, 458 p., accessed April 4, 2023, at https://doi.org/10.3133/tm4A3. [Supersedes USGS Techniques of Water-Resources Investigations, book 4, chapter A3, version 1.1.]
Hem, J.D., 1985, Study and interpretation of the chemical characteristics of natural water (3d ed.): U.S. Geological Survey Water-Supply Paper 2254, 264 p., accessed September 11, 2022, at https://pubs.usgs.gov/wsp/wsp2254/.
ITIS, 2022, Integrated Taxonomic Information System: ITIS database, accessed October 18, 2022, at https://www.itis.gov.
Kestrel, 2022, Kestrel 5500 weather meter: Kestrel Weather & Environmental Meters web page, accessed March 31, 2022, at https://kestrelmeters.com/products/kestrel-5500-weather-meter.
Kitting, C.L., Fry, B., and Morgan, M.D., 1984, Detection of inconspicuous epiphytic algae supporting food webs in seagrass meadows: Oecologia, v. 62, no. 2, p. 145–149, accessed March 28, 2023, at https://doi.org/10.1007/BF00379006.
Leica Geosystems, 2010, Leica Viva series—Technical reference manual (ver. 1.0): Leica Geosystems web page, accessed June 6, 2022, at https://www.gefos-leica.cz/ftp/GPS/Navody/EN_Originaly/Viva_GNSS/Viva_Technical_reference.pdf.
McCarthy, M.J., Otis, D.B., Méndez-Lázaro, P., and Muller-Karger, F.E., 2018, Water quality drivers in 11 Gulf of Mexico estuaries: Remote Sensing, v. 10, no. 2, 15 p., accessed April 6, 2023, at https://doi.org/10.3390/rs10020255.
Morton, R.A., Ward, G.H., and White, W.A., 2000, Rates of sediment supply and sea-level rise in a large coastal lagoon: Marine Geology, v. 167, nos. 3–4, p. 261–284, accessed March 28, 2023, at https://doi.org/10.1016/S0025-3227(00)00030-X.
National Oceanic and Atmospheric Administration [NOAA], 2018, Ocean acidification: National Oceanic and Atmospheric Administration web page, accessed June 13, 2022, at https://www.noaa.gov/education/resource-collections/ocean-coasts/ocean-acidification.
National Oceanic and Atmospheric Administration [NOAA], 2022a, Habitat blueprint—Living shorelines: National Oceanic and Atmospheric Administration web page, accessed June 13, 2022, at https://www.habitatblueprint.noaa.gov/living-shorelines/.
National Oceanic and Atmospheric Administration [NOAA], 2022b, Tides and currents—SPI Brazos Santiago, TX—Station ID 8779749: National Oceanic and Atmospheric Administration web page, accessed December 19, 2022, at https://tidesandcurrents.noaa.gov/stationhome.html?id=8779749.
Nortek, 2022, Eco current profiler: Nortek web page, accessed October 18, 2022, at https://www.nortekgroup.com/products/eco.
Ockerman, D.J., 2022a, Bathymetric data for a near-shore area of the Laguna Madre near South Padre Island, Texas, July 2021: U.S. Geological Survey data release, https://doi.org/10.5066/P9X7QKBF.
Ockerman, D.J., 2022b, Hydrodynamic data for a near-shore area of the Laguna Madre near South Padre Island, Texas, August 2021 to April 2022: U.S. Geological Survey data release, https://doi.org/10.5066/P9AUKOC8.
Ohmex Instrumentation, 2016, Sonarmite v5.xx BTX/SPX single beam portable Bluetooth echo sounder: Ohmex Ltd. web page, accessed on June 6, 2022, at http://www.ohmex.com/BTXhardware.pdf.
Onuf, C., 2006, Laguna Madre, in Handley, L., Altsman, D., and DeMay, R., eds., Seagrass status and trends in the northern Gulf of Mexico—1940–2002: U.S. Geological Survey Scientific Investigations Report 2006–5287, p. 29–40, accessed on December 20, 2022, at https://doi.org/10.3133/sir20065287.
Onuf, C.P., and Ingold, J.J., 2007, A GIS analysis of seagrass resources and condition withing Padre Island National Seashore, Texas: U.S. Geological Survey Open-File Report 2007–1261, 34 p., accessed September 5, 2023, at https://doi.org/10.3133/ofr20071261.
Opsahl, S.P., Ingold, J.J., Higginbotham, M., and Reynolds, S.M., 2022, Seagrass, benthic invertebrate, and water-quality data for a near-shore area of the Laguna Madre near South Padre Island, Texas, September 2021: U.S. Geological Survey data release, https://doi.org/10.5066/P9GRJA3X.
Paul, M.J., Coffey, R., Stamp, J., and Johnson, T., 2019, A review of water quality responses to air temperature and precipitation changes 1—Flow, water temperature, saltwater intrusion: Journal of the American Water Resources Association, v. 55, no. 4, p. 824–843, accessed April 5, 2023, at https://doi.org/10.1111/1752-1688.12710.
Port of Brownsville, 2022, Overview—Economic impact statement: Port of Brownsville web page, accessed December 19, 2022, at https://www.portofbrownsville.com/about/.
Quammen, M.L., and Onuf, C.P., 1993, Laguna Madre—Seagrass changes continue decades after salinity reduction: Estuaries, v. 16, no. 2, p. 302–310, accessed April 2, 2023, at https://doi.org/10.2307/1352503.
Rantz, S.E., and others, 1982, Measurement and computation of streamflow—Volume 1. Measurement of stage and discharge: U.S. Geological Survey Water-Supply Paper 2175, 284 p., accessed January 23, 2020, at https://doi.org/10.3133/wsp2175.
Ravella, P.A., Worsham, B., Mann, R.E., and Trevino, R., 2012, City of South Padre Island Erosion Response Plan: Texas General Land Office, 51 p., accessed October 17, 2022, at https://www.myspi.org/egov/documents/1376428264_16955.pdf.
Rooker, J.R., Holt, G.J., and Holt, S.A., 1998, Vulnerability of newly settled red drum (Sciaenops oscellatus) to predatory fish—Is early life history enhanced by seagrass meadows?: Marine Biology, v. 131, no. 1, p. 145–151, accessed January 23, 2023, at https://doi.org/10.1007/s002270050305.
Rusnak, G.A., 1960, Sediments of Laguna Madre, Texas, in SP 21—Recent sediments, northwest Gulf of Mexico: American Association of Petroleum Geologists, p. 153–196, accessed December 22, 2022, at https://archives.datapages.com/data/specpubs/sedimen1/images/a143/a1430001/0150/01530.pdf.
Rydlund, P.H., Jr., and Densmore, B.K., 2012, Methods of practice and guidelines for using survey-grade global navigation satellite systems (GNSS) to establish vertical datum in the United States Geological Survey: U.S. Geological Survey Techniques and Methods, book 11, chap. D1, 102 p., 4 app., accessed April 4, 2023, at https://doi.org/10.3133/tm11D1.
Sanchez, M.L., 2018, Effect of vessel-generated waves in near low tide conditions on shorelines in the Intracoastal Waterways: Jacksonville, Fla., University of North Florida, master’s thesis, Graduate Theses and Dissertations no. 789, 43 p., accessed April 4, 2023, at https://digitalcommons.unf.edu/etd/789.
Sheridan, P., 2004, Recovery of floral and faunal communities after placement of dredged material on seagrasses in Laguna Madre, Texas: Estuarine, Coastal and Shelf Science, v. 59, no. 3, p. 441–458, accessed April 4, 2023, at https://doi.org/10.1016/j.ecss.2003.10.004.
Smith, N.P., 1978, Intracoastal tides of upper Laguna Madre, Texas: Texas Journal of Science, v. 30, no. 1, p. 85–95, accessed April 4, 2023, at https://fau.digital.flvc.org/islandora/object/fau%3A32697.
Texas General Land Office, 2022, Texas Coastal Resiliency Master Plan: Texas General Land Office web page, accessed December 5, 2022, at https://www.glo.texas.gov/coast/coastal-management/coastal-resiliency/index.html.
The Nature Conservancy, 2020, Safeguarding South Padre Island—The world’s largest barrier island: The Nature Conservancy web page, accessed June 13, 2022, at https://www.nature.org/en-us/about-us/where-we-work/united-states/texas/stories-in-texas/south-padre-island/#:~:text=Bordered%20by%20the%20Laguna%20Ma dre,federal%20or%20state%20conservation%20status.
Trimble Navigation Limited, 2015, Trimble R10 GNSS receiver—User guide (ver. 1.10, rev. B, November 2014): Trimble Navigation Limited web page, accessed on June 6, 2022, at https://receiverhelp.trimble.com/r10-gnss/R10%20UserGuide.pdf.
Tunnel, J.W., Judd, F.W., and Bartlett, R.C., 2001, The Laguna Madre of Texas and Tamaulipas: Texas A&M University Press, 346 p.
Turnipseed, D.P., and Sauer, V.B., 2010, Discharge measurements at gaging stations: U.S. Geological Survey Techniques and Methods, book 3, chap. A8, 87 p., accessed January 1, 2023, at https://doi.org/10.3133/tm3A8.
U.S. Environmental Protection Agency, 2016, Standard operating procedure for benthic invertebrate field sampling (rev. 11, March 2016): U.S. Environmental Protection Agency web page, accessed March 31, 2021, at https://www.epa.gov/sites/default/files/2017-01/documents/sop-for-benthic-invertebrate-field-sampling-201603-8pp.pdf.
U.S. Environmental Protection Agency, 2020, Sediment sampling: U.S. Environmental Protection Agency, Laboratory Services and Applied Science Division, LSASDPROC–200–R4, 17 p., accessed March 4, 2022, at https://www.epa.gov/sites/default/files/2015-06/documents/Sediment-Sampling.pdf.
U.S. Geological Survey, 2022, USGS water data for the Nation: U.S. Geological Survey National Water Information System database, accessed July 1, 2022, at https://doi.org/10.5066/F7P55KJN.
U.S. Geological Survey, [variously dated], National field manual for the collection of water-quality data: U.S. Geological Survey Techniques of Water-Resources Investigations, book 9, chaps. A1–A10, accessed January 1, 2023, at https://doi.org/10.3133/twri09.
WoRMS Editorial Board, 2022, World Register of Marine Species: WoRMS website, accessed January 3, 2023, at https://www.marinespecies.org.
Xylem, 2023, EXO2s multiparameter sonde: Washington, D.C., Xylem, Inc., web page accessed March 10, 2023, at https://www.ysi.com/exo2s?gclid=Cj0KCQiAx6ugBhCcARIsAGNmMbgdobQAvWRLuTwb0R8Ju9VglsbqdHon0YsSnAtXgIKtn4miLPxhqoUaAt9MEALw_wcB.
Ziegler, S., and Benner, R., 1998, Ecosystem metabolism in a subtropical seagrass-dominated lagoon: Marine Ecology Progress Series, v. 173, p. 1–12, accessed March 10, 2023, at https://www.int-res.com/articles/meps/173/m173p001.pdf.
Conversion Factors
Datums
Vertical coordinate information is referenced to the North American Vertical Datum of 1988 (NAVD 88).
Horizontal coordinate information is referenced to the North American Datum of 1983 (NAD 83).
Elevation, as used in this report, refers to distance above the vertical datum.
Supplemental Information
Specific conductance is given in microsiemens per centimeter at 25 degrees Celsius (µS/cm at 25 °C).
Concentrations of chemical constituents in water are given in milligrams per liter (mg/L).
Abbreviations
ADCP
acoustic Doppler current profiler
CQ
estimated coordinate quality for Leica GNSS system
GNSS
Global Navigation Satellite System
ITIS
Integrated Taxonomic Information System
NOAA
National Oceanic and Atmospheric Administration
NWIS
National Water Information System
RTN
Real Time Network
USGS
U.S. Geological Survey
For more information about this publication, contact
Director, Oklahoma-Texas Water Science Center
U.S. Geological Survey
1505 Ferguson Lane
Austin, TX 78754–4501
For additional information, visit
https://www.usgs.gov/centers/ot-water
Publishing support provided by
Lafayette Publishing Service Center
Disclaimers
Any use of trade, firm, or product names is for descriptive purposes only and does not imply endorsement by the U.S. Government.
Although this information product, for the most part, is in the public domain, it also may contain copyrighted materials as noted in the text. Permission to reproduce copyrighted items must be secured from the copyright owner.
Suggested Citation
Opsahl, S.P., Beltran, J.I., and Ockerman, D.J., 2023, Bathymetric, hydrodynamic, biological, and water-quality characteristics of a nearshore area of the Laguna Madre near South Padre Island, Texas, 2021–22: U.S. Geological Survey Scientific Investigations Report 2023–5111, 19 p., https://doi.org/10.3133/sir20235111
ISSN: 2328-0328 (online)
Study Area
Publication type | Report |
---|---|
Publication Subtype | USGS Numbered Series |
Title | Bathymetric, hydrodynamic, biological, and water-quality characteristics of a nearshore area of the Laguna Madre near South Padre Island, Texas, 2021–22 |
Series title | Scientific Investigations Report |
Series number | 2023-5111 |
DOI | 10.3133/sir20235111 |
Year Published | 2023 |
Language | English |
Publisher | U.S. Geological Survey |
Publisher location | Reston, VA |
Contributing office(s) | Oklahoma-Texas Water Science Center |
Description | Report: viii, 18 p.; 4 Data Releases |
Country | United States |
State | Texas |
Other Geospatial | South Padre Island |
Online Only (Y/N) | Y |
Google Analytic Metrics | Metrics page |