Monitoring and Assessment of Urban Stormwater Best Management Practices at Selected Chicago Public Schools in Chicago, Illinois, from September 1, 2016, to July 1, 2017
Links
- Document: Report (9.3 MB pdf) , HTML , XML
- Dataset: USGS National Water Information System database —USGS water data for the Nation
- Download citation as: RIS | Dublin Core
Acknowledgments
The authors wish to acknowledge the Chicago Public Schools for allowing access to the school properties to service and collect data and to the Metropolitan Water Reclamation District of Greater Chicago for including the U.S. Geological Survey in this study.
Abstract
The Space to Grow program helps transform aging and neglected schoolyards of Chicago Public Schools into outdoor community spaces with the goal of promoting health and learning while addressing neighborhood flooding issues. Virgil I. Grissom Elementary School and Donald L. Morrill Math and Science School were selected in 2014 for schoolyard upgrades and the installation of various green infrastructure (GI) improvements. The U.S. Geological Survey installed sensors to measure precipitation, groundwater levels, and stormwater runoff volumes from September 1, 2016, to July 1, 2017.
At Virgil I. Grissom Elementary School, about 933,000 gallons of water fell on the schoolyard during the monitoring period. No discharge was recorded coming from the GI sewer lines, but backflow indicated water was flowing from the sewer line draining the impervious running track into the combined manhole structure and backwards into the GI retention basins (as designed). This design allowed for a 100-percent capture rate. Native soil at Virgil I. Grissom Elementary School also was conducive to rapid infiltration. Soil borings at Virgil I. Grissom Elementary School indicated about 10.5 feet (ft) of fine sand overlying silty clay to a depth of at least 16 ft. At Donald L. Morrill Math and Science School, about 1,120,000 gallons of water fell on the schoolyard during the monitoring period. About 72.5 precent of this water was discharged into the sewer system, and the other 27.5 percent was captured by the GI. Unlike Virgil I. Grissom Elementary School, the soil profile at Donald L. Morrill Math and Science School consisted of about 5 ft of clay loam overlying stiff blue clay to a depth of at least 12 ft. The sewer line coming from the GI under the football field was at the bottom of the reservoir. This design seemed to allow water to flow out of the line before being absorbed by the retention basin.
Introduction
The Metropolitan Water Reclamation District (MWRD) of Greater Chicago has joined with the Chicago Public Schools, City of Chicago Department of Water Management, and the Chicago-based nonprofits Healthy Schools Campaign and Openlands in a program called Space to Grow. The objective of the program is to transform aging and neglected schoolyards into outdoor community spaces for physical activity, outdoor learning, environmental literacy, and engagement with art while addressing neighborhood flooding issues. The program includes replacing playground equipment and uses a wide variety of green infrastructure (GI) improvements and stormwater best management practices to build outdoor classroom areas, turf fields, jogging tracks, basketball courts, and rain and vegetable gardens. The MWRD encourages the projects to maximize the design retention capacity (amount of water held by the GI) of the improvements to direct stormwater into the ground as infiltration rather than as runoff that contributes to local flooding.
Space to Grow began as a pilot program in 2014 with four schools selected for improvements. The program has specific criteria for selecting schoolyards for renovation, which include location in a high flood risk tier, high priority need for a playground, and minimum impermeable surface area of 300,000 square feet (ft2; Space to Grow, 2023). Site selection includes an application process, and review and final selection are done by the Space to Grow partners. During 2015, the MWRD indicated willingness to expand the program to as many as 30 additional schools. The unique partnership of agencies and organizations in the Space to Grow program brings together combined resources to address playground needs of Chicago area schools with enhanced stormwater management and the advancement of GI science and engineering.
The MWRD was interested in determining if the stormwater reduction goals of the individual schoolyard GI improvements were being met and how the GI improvements performed over time. In 2016, the U.S. Geological Survey (USGS), in cooperation with MWRD, began a study with the purpose of monitoring the hydrology in and near Space to Grow projects to help quantify the performance of the stormwater best management practices being used at these program sites. As part of this study, USGS monitored two of the initial four schools in the 2014 Space to Grow pilot: Virgil I. Grissom Elementary School (henceforth known as Grissom) in the Hegewisch community area on the near southeastern side of the city, and Donald L. Morris Math and Science School (henceforth known as Morrill) in the Chicago Lawn community area on the south side of the city (fig. 1, table 1). Using GI engineering design plans and maps of adjacent utilities and site inspection visits, the USGS helped develop monitoring plans for these two school GI project sites, and installation of equipment and monitoring began in late summer 2016. The information gained during this study can be used to inform best management practices for urban stormwater management at other locations where flooding is a concern.
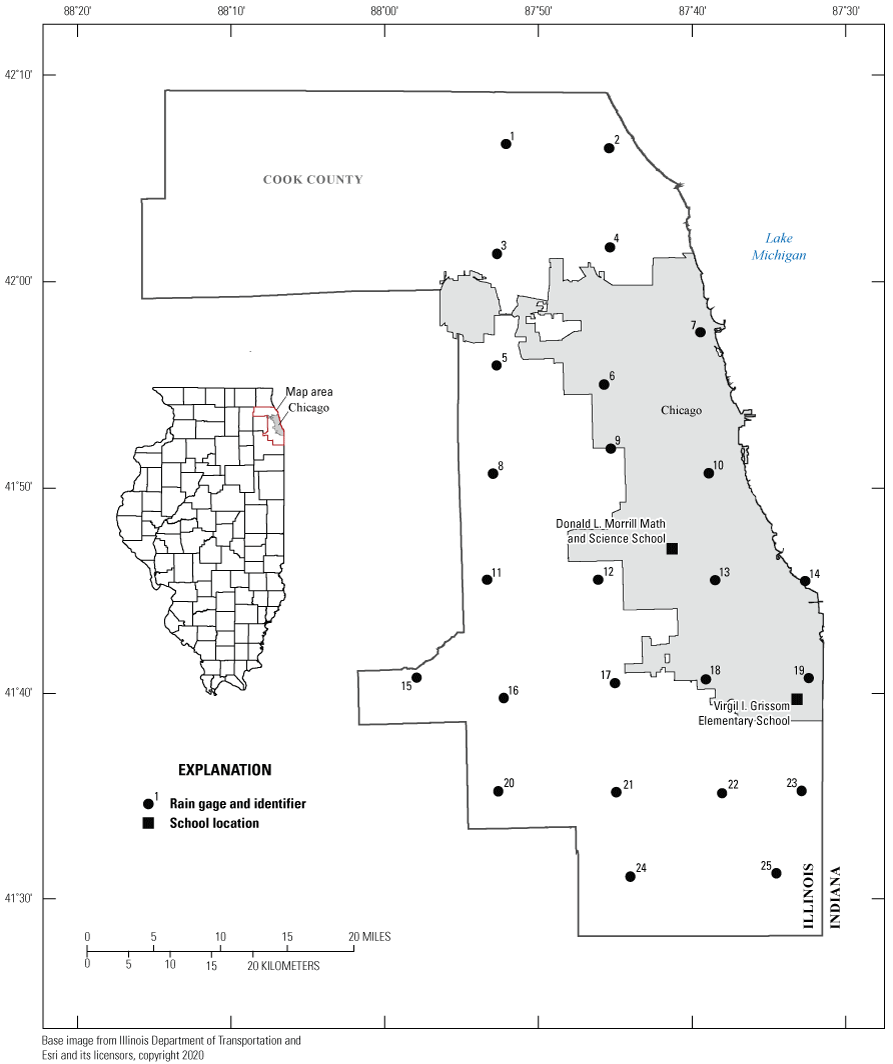
Location of Virgil I. Grissom Elementary School, Donald L. Morrill Math and Science School, and rain gages of the Cook County Precipitation Network in the Chicago, Illinois, metropolitan area.
Table 1.
Site information of Virgil I Grissom Elementary School and Donald L. Morrill Math and Science School sewer and groundwater sites.[Station data from U.S. Geological Survey (2023). USGS, U.S. Geological Survey; IL, Illinois; °, degree; ', minute; “, second; stn, station]
Purpose and Scope
This report describes and summarizes the hydrological monitoring of the GI improvements at two Chicago public schools—Grissom and Morrill—from September 1, 2016, to July 1, 2017. The report includes descriptions of the GI improvements at both facilities, installation of various equipment to monitor the sites, and a summary of data collected including precipitation, groundwater levels, and stormwater runoff volumes. Also included are data from individual storms, estimates of runoff volumes at each site, and a comparison of runoff from GI and non-GI areas.
Site Description
The following sections present descriptions of the sewer infrastructure and soil characteristics at the Grissom and Morrill study areas.
Virgil I. Grissom Elementary School
Unique conditions at Grissom allowed for a side by side or paired area comparison of the newly implemented GI improvements and the adjacent existing impervious running track (fig. 2). The GI area, about 48,300 ft2, was slightly larger than the impervious area of the running track, which is about 43,200 ft2.
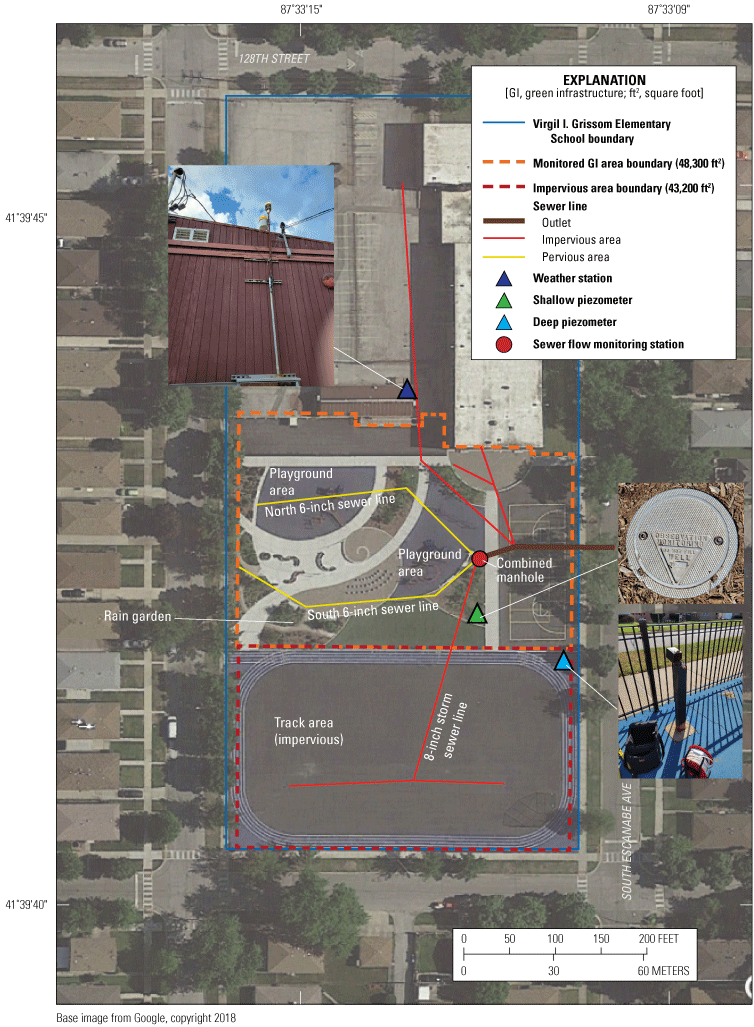
Plan view utility map of Virgil I. Grissom Elementary School.
Storm sewer lines drain the impervious running track and the pervious GI flow from the permeable pavement in the playground and rain garden into a combined manhole structure before exiting the school grounds to a local sewer line (fig. 2). Sewer lines draining the impervious track combine into one 8-inch (in.) line that then enters the combined manhole structure about 5.62 feet (ft) below land surface (fig. 2; John Watson, MWRD, written comm., October 2015). The GI area is drained by two 6-in. sewer lines that also discharge into the combined manhole. The sewer line referred to as “GI north” drains the playground area and enters the combined manhole structure from the northwest about 2 ft below land surface; the line referred to as “GI south” drains the rain garden area and enters the combined manhole structure from the southwest about 2.5 ft below land surface.
The GI was designed to collect water from the surrounding land through infiltration from the surface and as runoff from the impervious track area. The outlet pipe for the combined manhole structure is outfitted with a restrictor to reduce the flow out to the sewer system, so when stormwater from the impervious track area enters the combined manhole, water does not immediately flow into the outlet pipe to the sewer system. As the water level rises within the combine manhole, it reaches the height of the GI north sewer line and starts to fill the retention basin beneath the playground (fig. 3). As the water level in the combined manhole continues to rise, the water level then reaches the height of the GI south sewer line and starts to fill the retention basin beneath the rain garden (fig. 3). After a storm, water is then slowly released into the outlet sewer system.
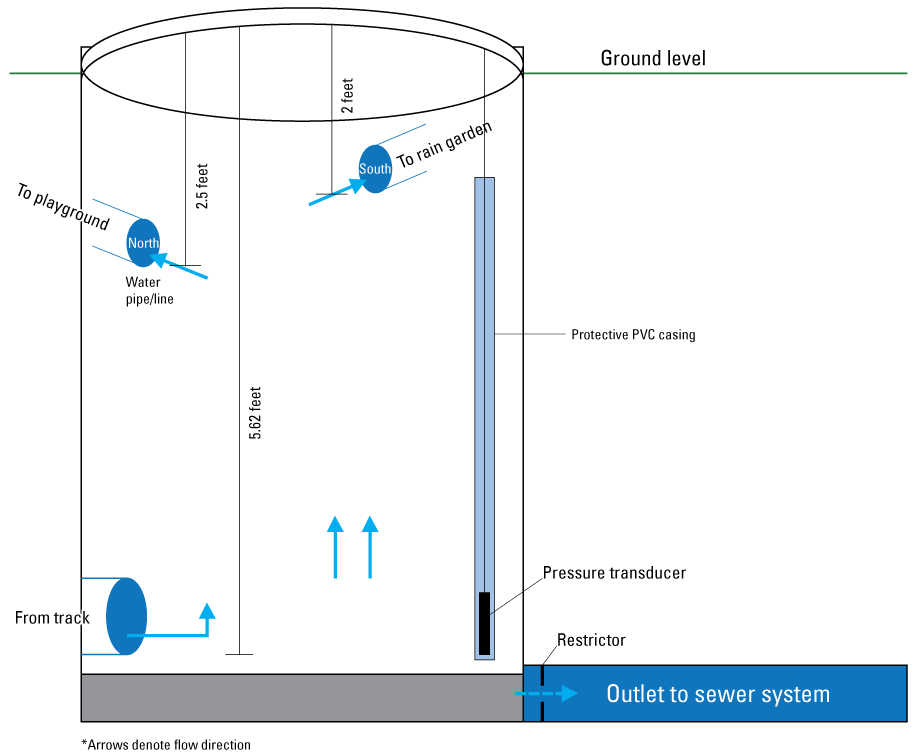
Schematic of the combined manhole structure (not to scale). [PVC, polyvinyl chloride]
Soil borings drilled in 1968 during a land survey before the school was built indicated about 1 ft of black silty-sandy topsoil overlying about 10.5 ft of brown and gray fine sand (appendix 1, fig. 1.1). Beneath the sand was a stiff gray silty clay and a trace of fine sand to about 20 ft (Illinois State Geological Survey, 2023). Cores collected during piezometer installation confirmed the descriptions in the 1968 boring logs.
Donald L. Morrill Math and Science School
Morrill has a large impervious area; however, access to sewer lines suitable for monitoring was limited (fig. 4). The bulk of the GI consisted of the main playground, a football field, and permeable pavers that were all monitored for this study. A rain garden on the east side of the school building was not connected to any of the sewer lines draining the GI and was therefore not directly monitored during this study.
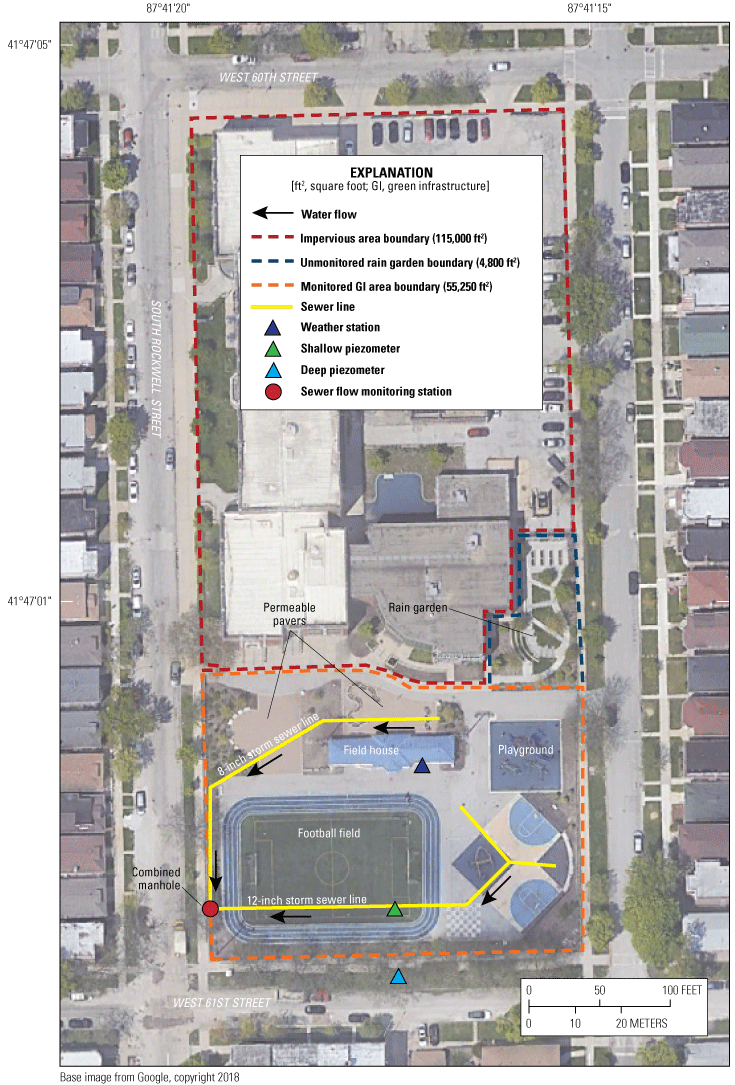
Plan view utility map of Donald L. Morrill Math and Science School.
The monitored part of the schoolyard is drained by two sewer lines that discharge into a combined manhole structure on the western border of the playground (sewer flow monitoring station, fig. 4). Sewer lines draining the basketball courts and play equipment area of the schoolyard combine into a 12-in. sewer line flowing through the engineered retention basin beneath the football field before exiting into the combined manhole structure (fig. 4). A single 8-in. sewer line drains the permeable pavers lining the northern edge of the playground exiting into the combined manhole structure.
Soil borings at Morrill indicated little topsoil and about 5 ft of clay and loam overlying compact blue clay to depths of at least 12 ft (Illinois State Geological Survey, 2023; fig. 1.2). This contrasts the high sand content in the upper 10.5 ft of soil at Grissom. A construction log for the deep piezometer at Morrill could not be located, and the shallow piezometer was installed after playground construction was completed so its log described the 4.6 ft of backfill for the GI retention basin under the football field.
Methods
The following sections present descriptions of the GI improvements and monitoring strategy at Grissom and Morrill. Grissom included GI and impervious surface monitoring whereas Morrill monitored GI only. Monitoring at both locations included runoff, weather conditions, and groundwater levels. Groundwater levels, stormwater flow volumes, precipitation, air pressure, relative humidity, and air temperature data can be accessed through the USGS National Water Information System database (U.S. Geological Survey, 2023) using the station identifiers in table 1.
Virgil I. Grissom Elementary School
HACH FL900 series sewer flow meters (control system) with Sigma Submerged AV900 acoustic Doppler sensors were deployed in each of the three sewer lines draining to the combined manhole structure. The sewer flow meters use acoustic signals to measure the stage and velocity of stormwater flow through the sewer lines and have a thermistor to collect water temperature data. The flow meters use the measured stage and velocity data to calculate an instantaneous discharge. The AV900 sensors measure accurate stage ranging from 0 to 10 ft and velocity/discharge at stages above 0.07 ft. V-notch weirs (Thel-Mar Company) were installed in the two 6-in. GI sewer lines in addition to the acoustic flow meters and Doppler sensors (fig. 5A, B). The installed weirs were to be used as a secondary method for determining discharge based on the stage measured by the HACH FL900. The stage/discharge relation for the weirs was predetermined by the manufacturer. An additional pressure transducer was installed within a protective polyvinyl chloride (commonly known as PVC) pipe inside the manhole structure to monitor stormwater backup.
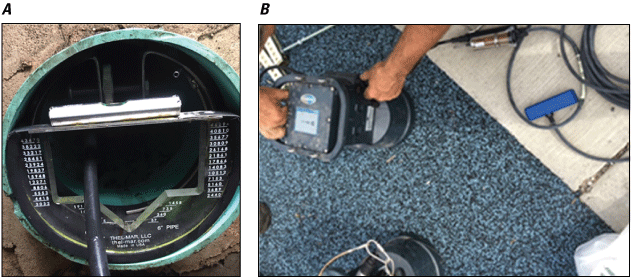
(A) A 6-inch V-notch weir (B) installed in combination with a sewer flow meter in the sewer line draining the green infrastructure at Virgil I. Grissom Elementary School. Photographs by Clint Bailey, U.S. Geological Survey.
Continuous water level and water quality data also were collected. Two 1-in. diameter piezometers were installed at the school in mid-August 2016 (appendix 2). One piezometer was installed on the northeastern corner of the impervious running track and the second within the rain garden part of the GI to compare water level responses of the impervious areas and GI areas (fig. 2). The running track piezometer was installed to a depth of 16 ft, screened from 11.2 to 15.9 ft, and a sand pack around the screen from 6 to 16 ft below land surface (fig. 2.1). The rain garden piezometer was installed at a shallower depth of 3.8 ft with a screen from 3.0 to 3.7 ft that is within the gravel retention basin beneath the rain garden (fig. 2.2). Piezometers were instrumented with an Aqua TROLL 100 pressure transducer to measure changes in groundwater levels and water temperature. In February 2017, the Aqua TROLL 100 deployed in the track piezometer was removed and replaced with an Aqua TROLL 200 to collect continuous specific conductance data in addition to groundwater levels and temperature.
A Vaisala weather station was deployed atop a field house on the northwestern edge of the playground (fig. 2). The weather station was equipped to measure precipitation, humidity, barometric pressure, air temperature, and wind velocity/direction. Data collected from the weather station were stored on a Campbell Scientific CR10 data logger. When USGS weather station data were lost, rainfall data were supplemented with local data collected from the Illinois State Water Survey (ISWS) Cook County Precipitation Network (CCPN).
Donald L. Morrill Math and Science School
Monitoring equipment at Morrill was only installed within the GI part of the school grounds. The 12-in. and 8-in. GI sewer lines were outfitted with HACH FL900 sewer flow meters and AV900 acoustic sensors. The 12-in. sewer line also was outfitted with a V-notch weir in addition to the flow meter described previously.
Groundwater levels and water quality were monitored with 1-in. diameter piezometers installed within the retention basin of the football field and outside the GI along the fence lining the perimeter of the schoolyard (fig. 4). The 4.6-ft deep GI piezometer with a 2-ft screen (fig. 2.1). was installed within the gravel retention basin surrounding the 12-in. sewer line that exits the GI at a depth of 3.25 ft below land surface. Another piezometer installed along the GI perimeter was drilled to a depth of 18 ft below land surface and screened 10–18 ft below land surface in silt and clay (log not available). Piezometers were outfitted with an Aqua TROLL 100 pressure transducer to collect water level and water temperature data. An Aqua TROLL 200 pressure and specific conductance sensor was initially installed within the deep piezometer along the GI perimeter; however, shortly after deployment in August 2016, the piezometer was vandalized and the Aqua TROLL 200 was stolen. A replacement sensor with water level and pressure only was installed in February 2017.
A weather station was installed on top of the field house roof in the center of the monitored GI. When USGS weather station data were lost, rainfall data were supplemented with local data collected from the ISWS CCPN.
Quality Assurance/Quality Control
During site visits, data were downloaded from the weather station, Aqua TROLLs, and sewer meters and checks were completed on the Aqua TROLLS and sewer meters. These data were then uploaded, reviewed, and approved in Aquarius Time-Series and are available from the USGS National Water Information System database (U.S. Geological Survey, 2023). All data are presented in this report in central standard time.
Groundwater Levels
At each piezometer, a measuring point from which groundwater levels were measured was established. These measuring points are in reference to land surface. Groundwater levels are measured using a calibrated electric tape then subtracting the measuring point from it to obtain a water level below land surface (Cunningham and Schalk, 2011). The sensor reading was then compared to this measured water level and if the sensor reading fell outside acceptable limits, the sensor was reset to the measured water level.
Specific Conductance
The Grissom specific conductance sensor in the deep monitoring piezometer in the track was cleaned and calibrated monthly. The sensor was checked against specific conductance standards and sensor data corrected according to the National Field Manual (U.S. Geological Survey, variously dated), Wagner and others (2006), and Mathany and others (2019).
Sewer Meters
Sewer meter data were downloaded during each site visit. The sewer pipes were checked and any debris behind each sensor was removed. If no water was flowing through the sewer line at the time of the field visit, the sensor was checked to ensure it was reading zero. If it was not, then the sensor was reset to zero and the recorded sensor data were corrected accordingly.
Discharge for the sewer lines was computed using the stage-to-discharge relation defined by the sensor manufacturer. Discharge for the sewer lines with weirs installed were computed using the stage-to-discharge relation defined by the weir manufacturer. Installation of the weirs resulted in 0.20 ft of stagnant water pooled behind them, so before calculating discharge, stage data were corrected to remove the 0.20 ft from the stage. However, discharge calculated from the corrected weir rating yielded total discharges greater than the total precipitation recorded at the site during the monitoring period. During several events, some highly anomalous instantaneous discharge values were reported. Discharge reported by the HACH FL900 sewer meters was “flashy” and did not depict a standard stormwater hydrograph for several events. As such, site-specific rating curves were developed for the sewer lines using stage data measured by the HACH FL900 and are described in the “Monitoring and Assessment Results and Discussion” section.
Monitoring and Assessment Results and Discussion
Discussion of the results from the Grissom and Morrill studies with in-depth analysis of specific storms at each location.
Virgil I. Grissom Elementary School
Site-specific rating curves for the Grissom GI sewer lines were not developed. This lack of site-specific rating curves is because no flow was recorded exiting the sewer lines and no weir was installed in the 8-in. sewer line coming from the track because of accessibility issues; this sewer was almost always under water.
Precipitation data collected from the weather station at Grissom revealed likely erroneous precipitation and other climatological data. Precipitation measured by the weather station was determined to underreport the actual precipitation amounts, so hourly precipitation data from September 1, 2016, to July 1, 2017, were interpreted from data collected at rain gage 19 of the ISWS CCPN less than 1.5 miles northeast of Grissom (fig. 1). A total of 30.98 in. of rainfall was recorded at rain gage 19 over the monitoring period (fig. 6). Precipitation data from rain gage 19 of the CCPN were collected and analyzed by Erin Bauer at the ISWS of the Prairie Research Institute at the University of Illinois. The CCPN is funded by the U.S. Army Corps of Engineers.
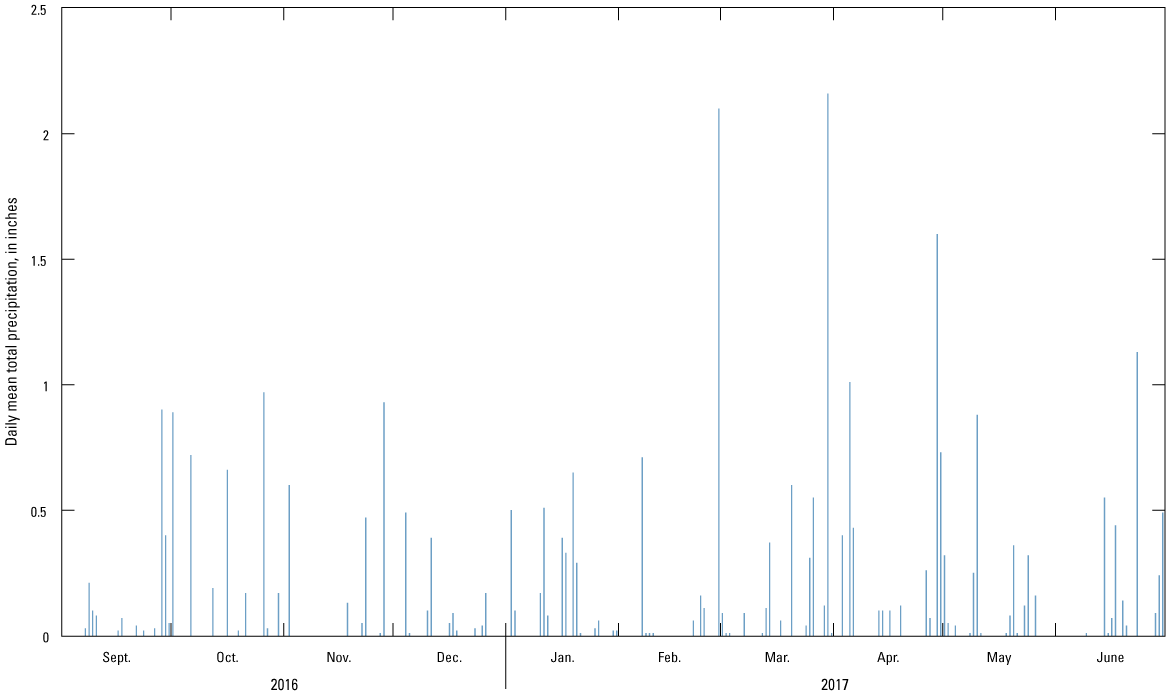
Precipitation data collected at rain gage 19 (fig. 1) of the Illinois State Water Survey (2022) Cook County Precipitation Network from September 1, 2016, to July 1, 2017.
Multiple rainfall events from September 1, 2016, to July 1, 2017, varied in amount and intensity. A total of 12 storms produced enough rainfall to have measurable groundwater levels within the 6-in. diameter GI sewer lines (fig. 7). The same events resulted in fairly similar stage values for each event measured within the GI north and GI south storm sewer lines. Stage from the GI line entering from the north side of the combined manhole structure was consistently about 0.25–0.5 ft higher than that recorded from the GI sewer line entering from the south. This difference in stage is nearly equal to the difference in depth below land surface at which the north and south sewer lines enter the combined manhole.
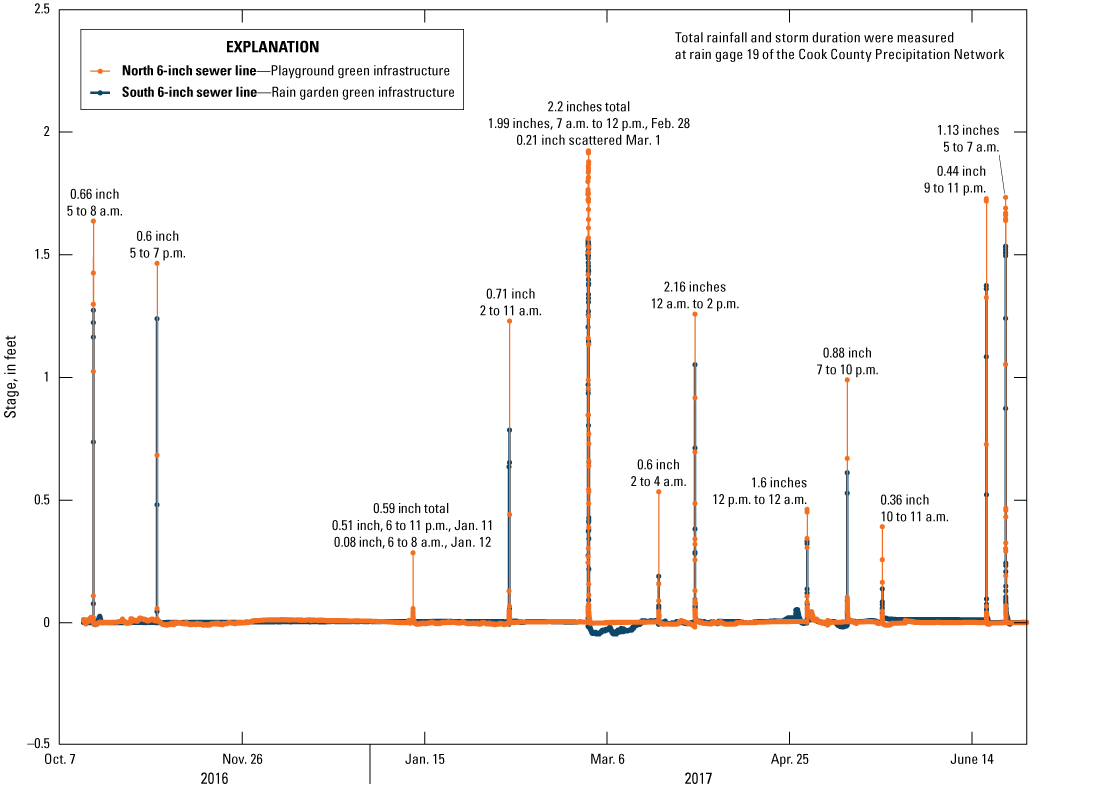
Stage recorded in the 6-inch green infrastructure sewer lines at Virgil I. Grissom Elementary School (station 413943087331301; U.S. Geological Survey, 2023) during major storm events from September 1, 2016, to July 1, 2017.
The same 12 rainfall events also were recorded in the 8-in. sewer line draining the impervious running track (fig. 8). Several additional events also were recorded by the flow meter installed in the 8-in. sewer line. Events that match those recorded in the GI sewer lines were consistently about 4–4.5 ft greater in the track sewer line. MWRD site plans indicated that the track sewer line enters the combined manhole at 3.12–3.62 ft below the depth of the GI sewer lines. Further investigation determined that water velocities recorded in the GI sewer lines during the 12 storm events were primarily negative, indicating that water was backflowing into the sewer lines from the combined manhole structure as opposed to draining from the GI (as designed). Positive velocities occurred during a storm surge when the water level in the combined manhole increased quickly, causing water to flush up into the GI lines and flow back out. Furthermore, comparing recorded stage values within each of the sewer lines during the events revealed that the difference in stage between each of the lines was equivalent to the difference in depth below land surface the sewer line entered into the combined manhole structure.
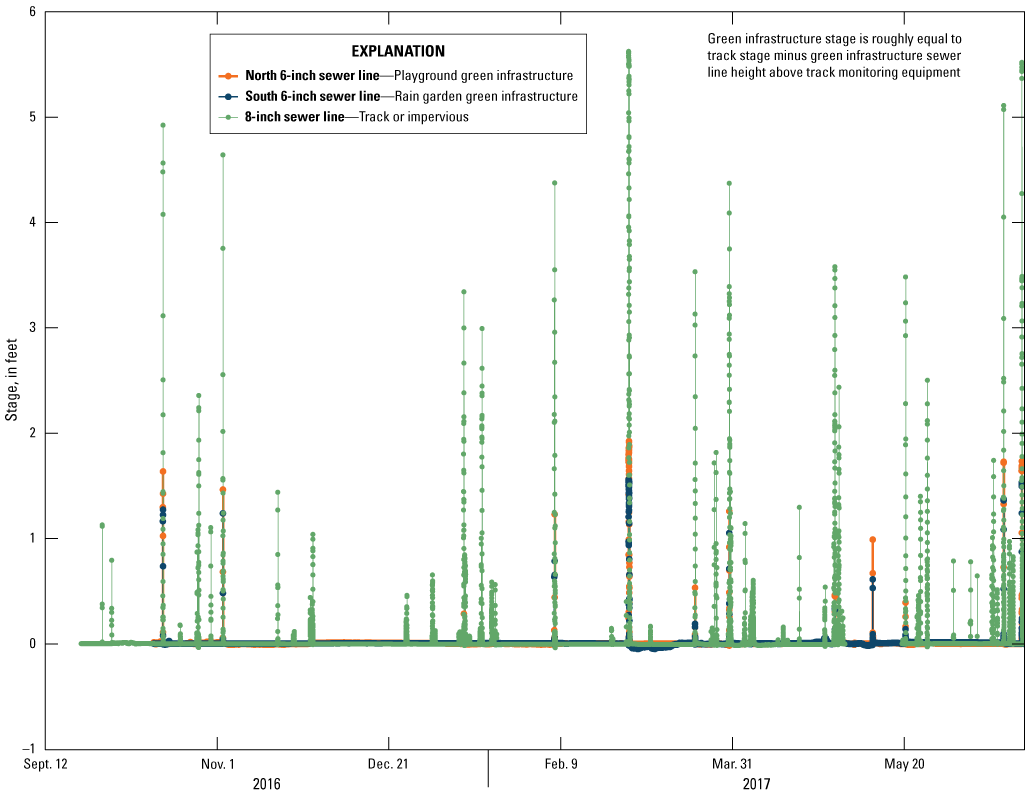
Sewer flow meter stage data collected at Virgil I. Grissom Elementary School (station 413943087331301; U.S. Geological Survey, 2023) over the period of September 1, 2016, to July 1, 2017.
Stormwater quality samples could not be collected at Grissom because, even during minor events, the 8-in. sewer line would be in backwater from the combined manhole within minutes of precipitation starting. Therefore, accurate fresh stormwater samples were impossible to collect during the monitoring period.
Groundwater levels recorded in the deep piezometer in the track indicated responses to each of the 12 major storms, along with increases in water level during smaller precipitation events (fig. 9). No response in water level was observed from the last two storm events because the piezometer had been redeveloped a few days before to remove excess sediment, and the water level had not been recovered (recovery took almost 1 month). The groundwater hydrographs indicate responses to rainfall events with somewhat rapid increases and slow declines back to baseline conditions. Some smaller rainfall events, which were not recorded in the sewer lines (October 1, 2016, and April 5–6, 2017), created substantial increases in groundwater levels, potentially indicating that saturation of surrounding soils from previous events likely plays a key role in water response to any given hydrologic event.
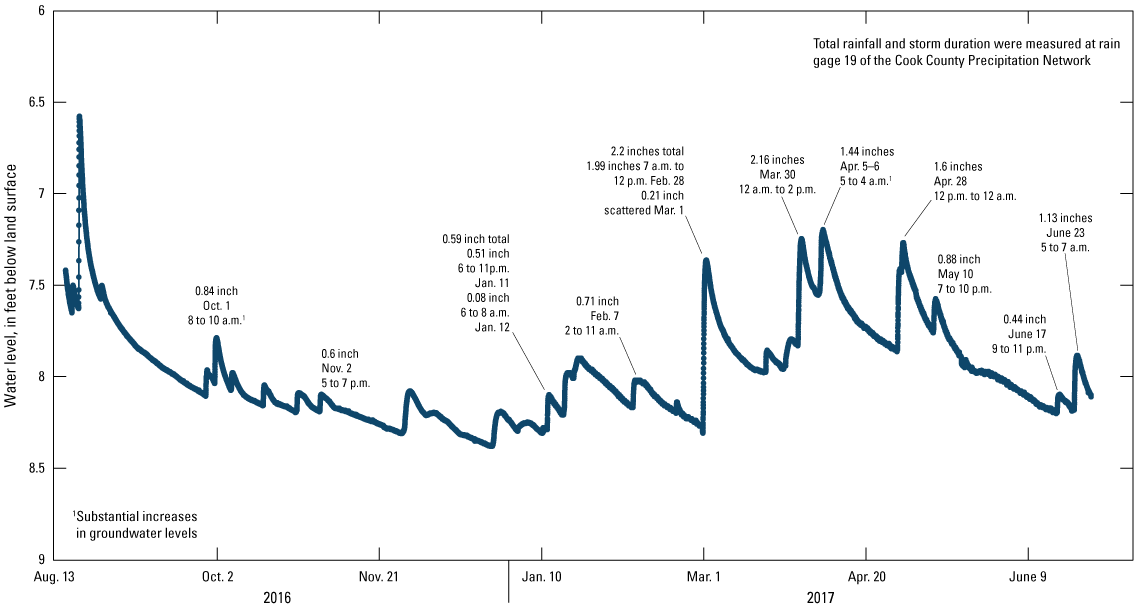
Groundwater levels recorded at the Virgil I. Grissom Elementary School deep piezometer (station 413941087331201; U.S. Geological Survey, 2023) in the track from August 14, 2016, to July 1, 2017.
Two of the major rainfall events were recorded from the shallow piezometer in the rain garden on January 11–12, 2017, and February 28–March 1, 2017 (fig. 10), which caused increases in water level by about 0.5 ft and 1 ft, respectively. A series of small events also were recorded between December 26, 2016, and January 8, 2017; however, these smaller events are likely erroneous because no substantial rainfall or snowmelt was measured during that period. Erroneous data could be a result of condensation freezing in the Aqua TROLL communication cable or ventilation tube. The missing data are a result of depth-to-water values exceeding the depth of the Aqua TROLL. These “negative” depth values are also likely a result of a potential blockage of the Aqua TROLL ventilation tube, which is supposed to allow the pressure sensor to equilibrate with barometric pressure changes. The Aqua TROLL was configured with a depth to water of 3.72 ft. The piezometer was usually dry, so this was the depth to the bottom of the piezometer.
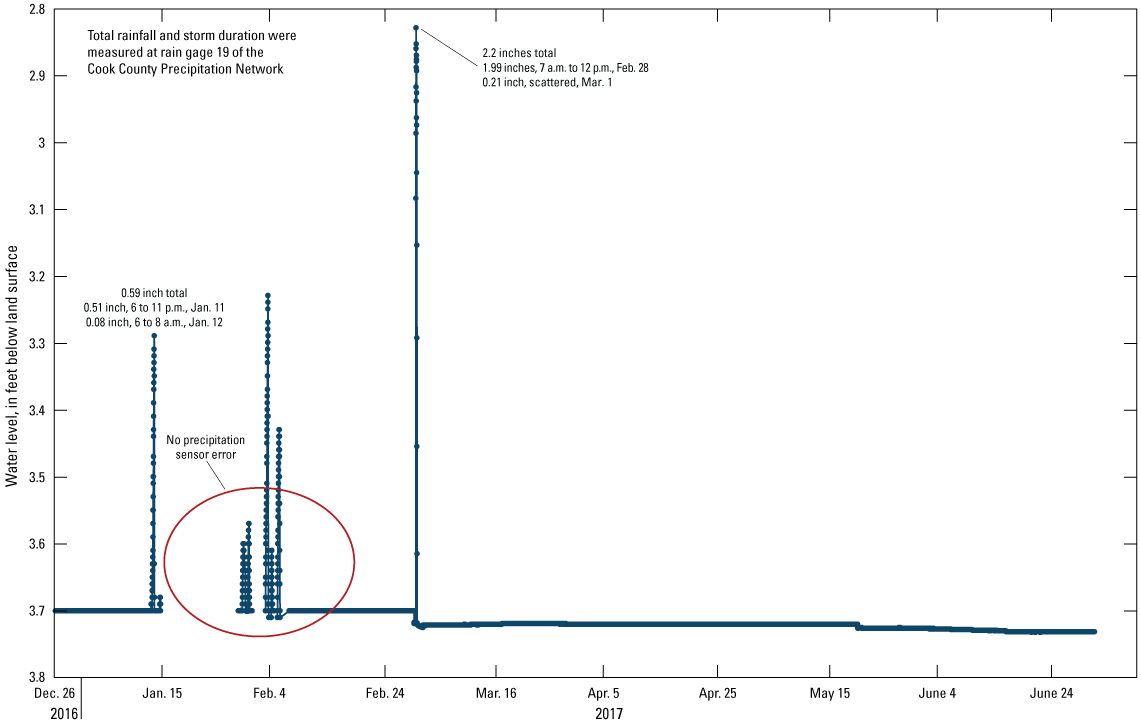
Groundwater levels recorded from the Virgil I. Grissom Elementary School green infrastructure shallow piezometer (station 413942087331301; U.S. Geological Survey, 2023) in the rain garden from December 26, 2016, to July 1, 2017.
Specific conductance data were recorded in the deep piezometer in the track from February 28 to July 1, 2017 (fig. 11). The water quality sensor was cleaned and calibrated monthly. During the May 2017 service visit, the sensor was heavily silted, which caused erroneous specific conductance values. The siltation was likely a result of one of the major storm events flushing silt from the surrounding borehole into the piezometer. The piezometer was repurged on June 28, 2017, and the sensor was redeployed. Specific conductance data collected during the fouled period from May through June 2017 were corrected using USGS standard procedures for data corrections as a result of fouling and calibration data shifts based on field measurements; however, values reported during this period are likely underestimated.
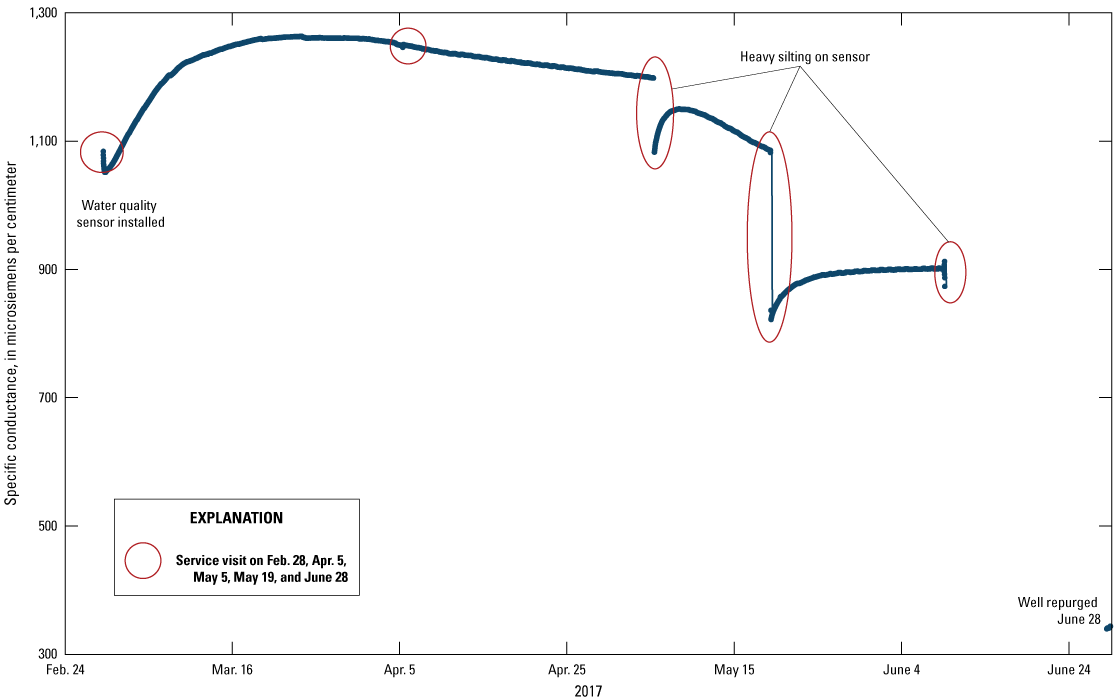
Specific conductance data collected at the Virgil I. Grissom Elementary School deep piezometer (station 413941087331201; U.S. Geological Survey, 2023) in the track from February 28, 2017, to June 28, 2017.
Water temperature data collected from the deep piezometer in the track indicated typical seasonal variations (fig. 12). The shallow piezometer in the rain garden was typically dry, so water temperature data were likely affected by air temperature and therefore are not reported in this summary.
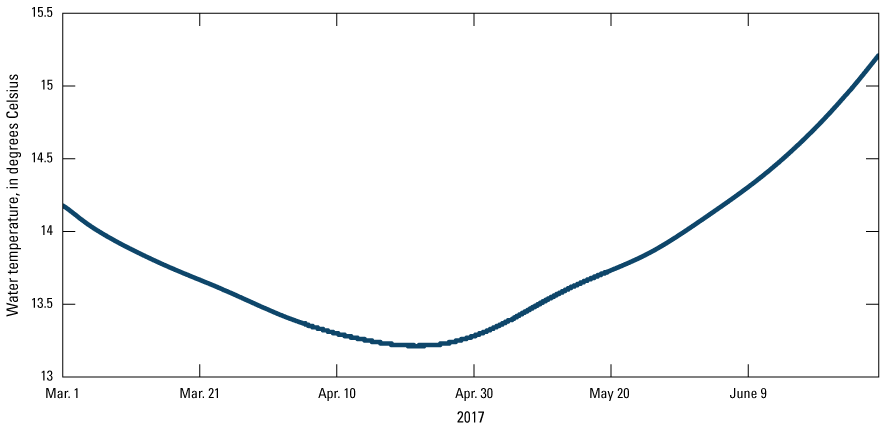
Water temperature data collected at the Virgil I. Grissom Elementary School deep piezometer (station 413941087331201; U.S. Geological Survey, 2023) in the track from February 28, 2017, to June 28, 2017.
Rainfall events on February 28 and June 23, 2017, at Grissom were selected for a more detailed analysis. Hourly precipitation totals were obtained from the rain gage 19 weather station of the ISWS CCPN and compared to stage data measured in the GI and track storm sewer lines. During the February 28 event, precipitation fell over a 6-hour period from 6 p.m. through March 1 at 12 a.m. (fig. 13). Precipitation intensity was greatest at the onset of the event between the hours of 6 p.m. and 7 p.m., when 0.72 in. of rain was recorded. This precipitation created a rapid increase in stage (from 0 to 5.04 ft) in the impervious track sewer line from about 6:25 p.m. to 6:30 p.m. The combined manhole filled within 5 minutes in response to this initial stormwater flush from the impervious track, and between 6:30 p.m. and 6:35 p.m., stormwater was measured in the GI north and GI south (fig. 13) storm sewer lines. The storm intensity ebbed the following hour; stage measured in the GI north line dropped to almost 0 ft, the GI south line stage dropped to 0 ft, and stage measured within the 8-in. track sewer line rapidly dropped to less than 2 ft. As precipitation intensity picked back up over the remainder of the event, similar responses were measured in all three sewer lines. Stormwater levels measured in the combined manhole briefly equaled 5.62 ft above the track sewer line between 6:45 p.m. and 7 p.m., a level that would create stormwater overflow from the manhole. After comparing results from the shallow piezometer in the rain garden, it was determined to be unlikely that substantial stormwater overflowed from the combined manhole because the groundwater levels in the shallow piezometer in the rain garden, less than 50 ft downslope, did not exceed land surface elevation at any point during the event. On February 28, 2017, a rapid increase in water level in the shallow piezometer began at 9:15 p.m. and peaked at 0.89 ft at 10:15 p.m. (fig. 10). The water level quickly decreased, and the piezometer was again dry by 2:15 a.m. on March 1, 2017. On February 28, 2017, the water level in the deep piezometer began to increase at about 6:30 p.m. and peaked on March 1, 2017, at 6 a.m. with a 0.94-ft increase. Unlike the shallow piezometer, the water level in the deep piezometer decreased slowly over the course of the next several days (fig. 9). Event discharge was not calculated from the track stormwater sewer because it became surcharged within minutes of the onset of the storm.
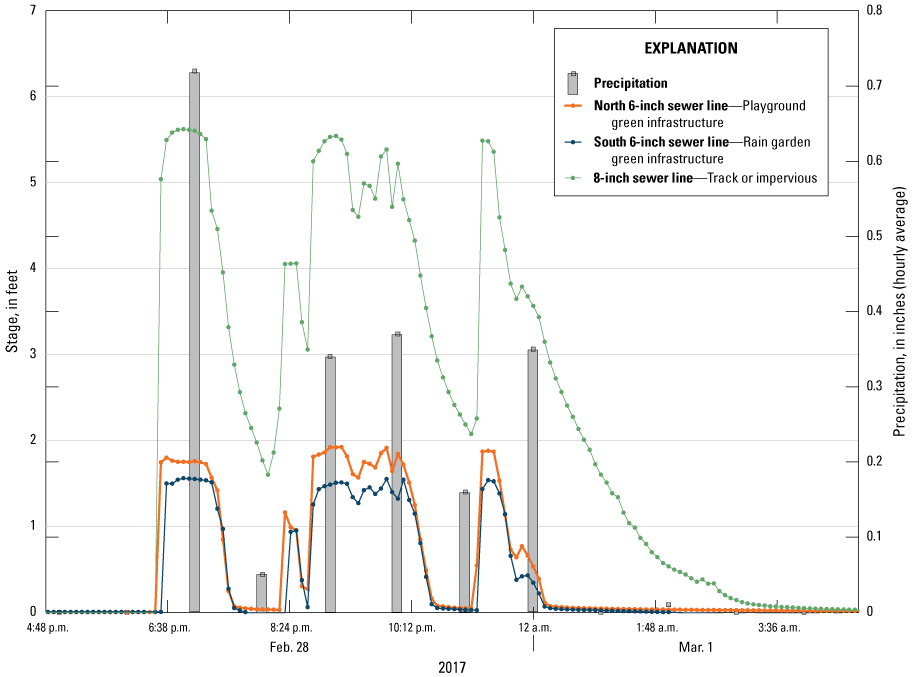
Stage (station 413943087331301; U.S. Geological Survey, 2023) and precipitation data from rain gage 19 of the Illinois State Water Survey (2022) Cook County Precipitation Network from the February 28, 2017, storm event recorded at Virgil I. Grissom Elementary School.
The June 23, 2017, storm occurred over a 3-hour period between 4 a.m. and 7 a.m. (fig. 14). During the first hour of the event, about 0.76 in. of rain fell. The monitored storm sewer lines responded similarly to the February 28 event (fig. 14). A rapid increase in stage was recorded in the track line that peaked at about 5.52 ft. Within 5 minutes of the initial flush recorded in the track sewer line, an increase in stage was measured at both GI sewer lines, which started in the GI north line followed by the GI south line. Stormwater levels in the combined manhole rose within 0.1 ft of the manhole rim but did not exceed overflow levels. No increase in shallow water level was recorded at the shallow piezometer (fig. 10) in the rain garden, which remained dry throughout the duration of the storm. A delayed response was recorded at the deep piezometer in the track (fig. 9); however, compared to the February 28 event, the increase in water level of less than 0.3 ft over the course of several hours was muted.
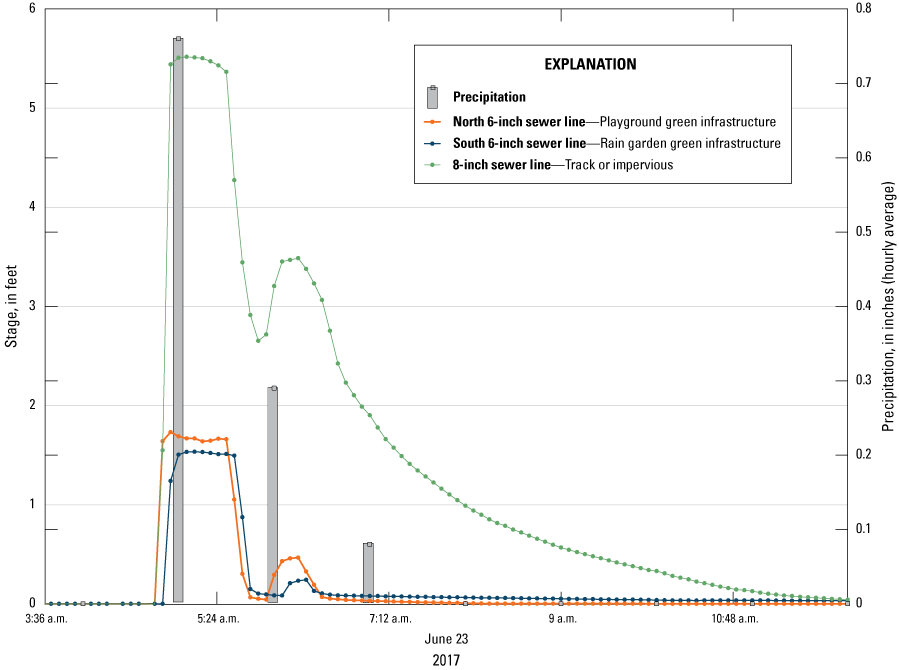
Stage (station 413943087331301; U.S. Geological Survey, 2023) and precipitation data from rain gage 19 of the Illinois State Water Survey (2022) Cook County Precipitation Network from the June 23, 2017, storm event recorded at Virgil I. Grissom Elementary School.
During the study period of September 1, 2016, to July 1, 2017, a total of 30.98 in. of rain fell on the Grissom schoolyard. This equates to roughly 124,700 cubic feet or 932,780 gallons (gal) of water falling on the pervious GI part of the schoolyard. The only recorded stage within the GI sewer lines during the monitoring period was measured during backflow from the combined manhole structure into the GI sewer lines. No discharge was detected from the GI sewer lines during the study period. The lack of discharge from the GI sewer lines indicate that the GI was able to accept and capture 100 percent of nearly 1 million gallons of water during the monitoring period. Additionally, when USGS personnel were completing a service visit during a storm on April 5, 2017, evidence was obtained of stormwater overflowing from the street into the GI (fig. 15). The GI also was able to capture this additional stormwater runoff from the street without creating flow discharging from the GI sewer. Our limited interpretation based on observations indicate that the 100-percent capture rate is likely the result of the effective GI design and the naturally permeable fine sand immediately beneath the GI.
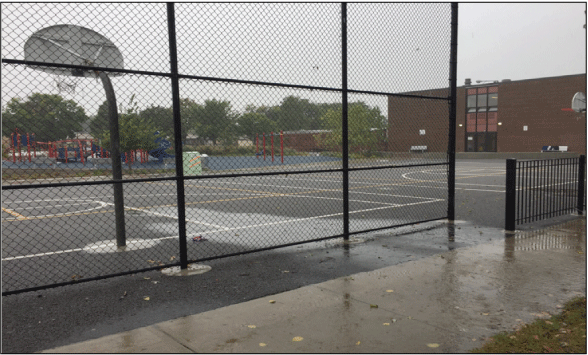
Stormwater overflow from the street flowing into the green infrastructure at Virgil I. Grissom Elementary School on October 6, 2016. Photograph by James Duncker, U.S. Geological Survey.
Donald L. Morrill Math and Science School
Precipitation from September 1, 2016, to July 1, 2017, was estimated as a mean using an inverse distance-weighted method using data from four ISWS CCPN rain gages (number 9, 10, 12, and 13) in proximity to Morrill (figs. 1 and 16). Based on the sum of the weighted daily mean, a total of 29.94 in. of rainfall was recorded over the course of the study.
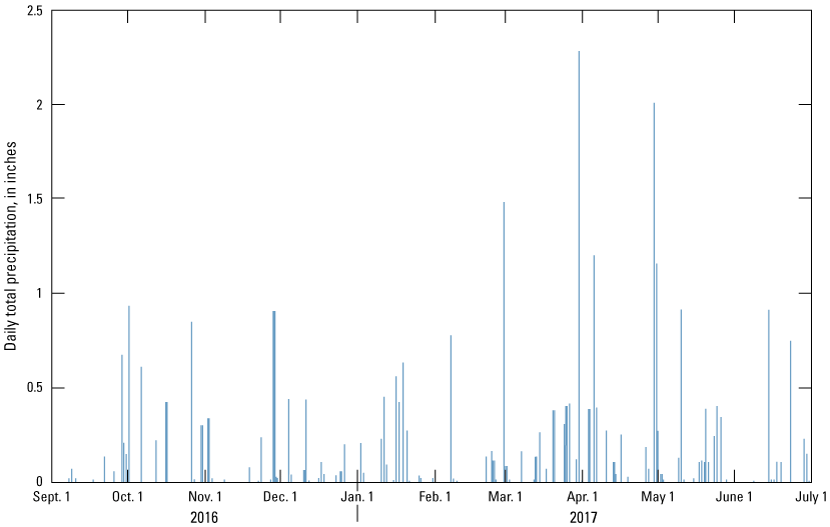
Daily mean precipitation estimated using an inverse distance-weighted method using data collected at rain gages 9, 10, 12, and 13 of the Illinois State Water Survey (2022) Cook County Precipitation Network from September 1, 2016, to July 1, 2017.
Precipitation events that occurred over Morrill were similar in rainfall accumulation and intensity to those recorded near Grissom from September 1, 2016, to July 1, 2017. Most of these events, even small storms, produced measurable stormwater runoff in the 8-in. sewer line (permeable pavers) and 12-in. sewer line (football field). Positive velocities corresponded with all substantial increases in stormwater stage in the 8-in. sewer line (fig. 17). In response to rainfall, stage in the combined manhole ranged from tenths of a foot to nearly 2 ft. Any stage over 8 in. (0.67 ft) exceeded the diameter of the sewer line and represents flooding in the combined manhole structure or elevated pressure in the drain during a substantial storm surge. No negative velocities were recorded in the sewer, unlike at Grissom, indicating that water was collecting in the GI areas and draining to the combined manhole structure.
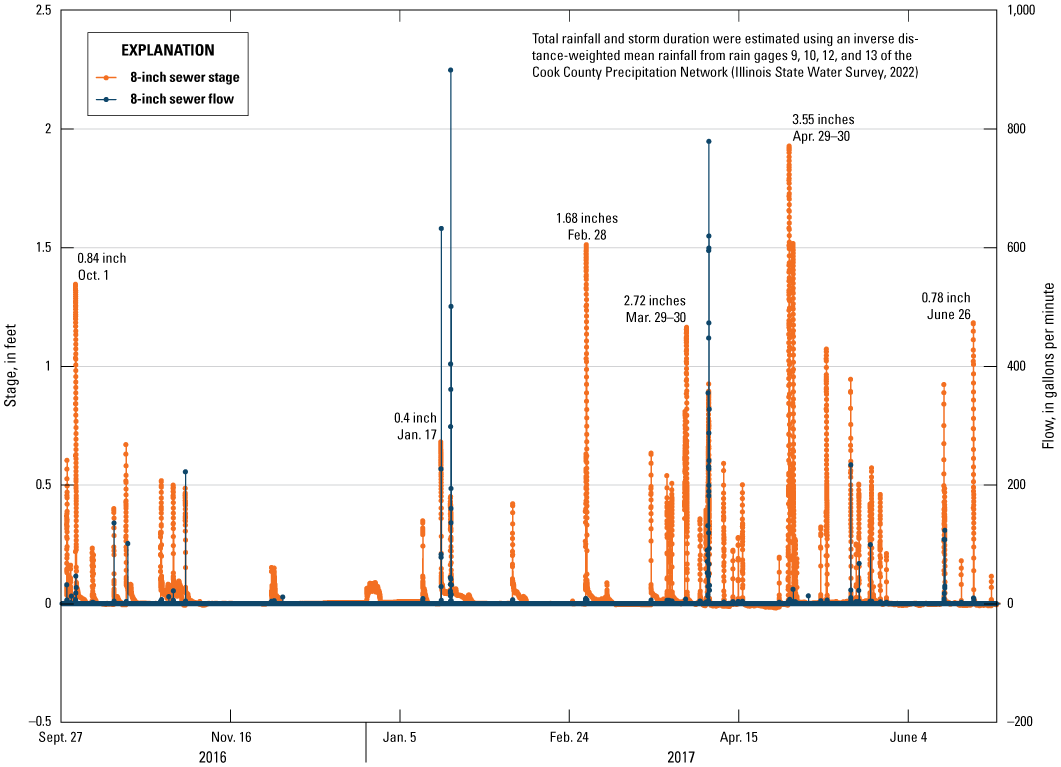
Stage and flow responses (station 414659087411901; U.S. Geological Survey, 2023) to major storm events measured in the 8-inch sewer line draining the permeable paver area at Donald L. Morrill Math and Science School from September 1, 2016, to July 1, 2017.
The responses in stage from major and minor storms measured in the 12-in. sewer line (football field; fig. 18) were similar to those from the permeable paver area. A V-notch weir was installed in the sewer line on October 24, 2016, to improve the accuracy of calculated instantaneous discharge. After the first storm following weir installation (October 26, 2016) a constant backwater was maintained behind the V-notch weir at a stage of about 0.20–0.21 ft. Stormwater flow only occurred when stage exceeded 0.20 ft, so stage data were corrected to remove the 0.20 ft of backwater behind the weir. Stormwater flow through the 12-in. sewer line (football field) was unique compared to the 8-in. sewer line (permeable paver) in that runoff was witnessed during and after precipitation events. The recorded hydrographs of stormwater flow measured in the 12-in. sewer line (football field) were less “flashy” compared to events recorded in the 8-in. sewer line (permeable paver), with a rapid initial increase in stage and a slow decline back to the 0.20-ft baseline.
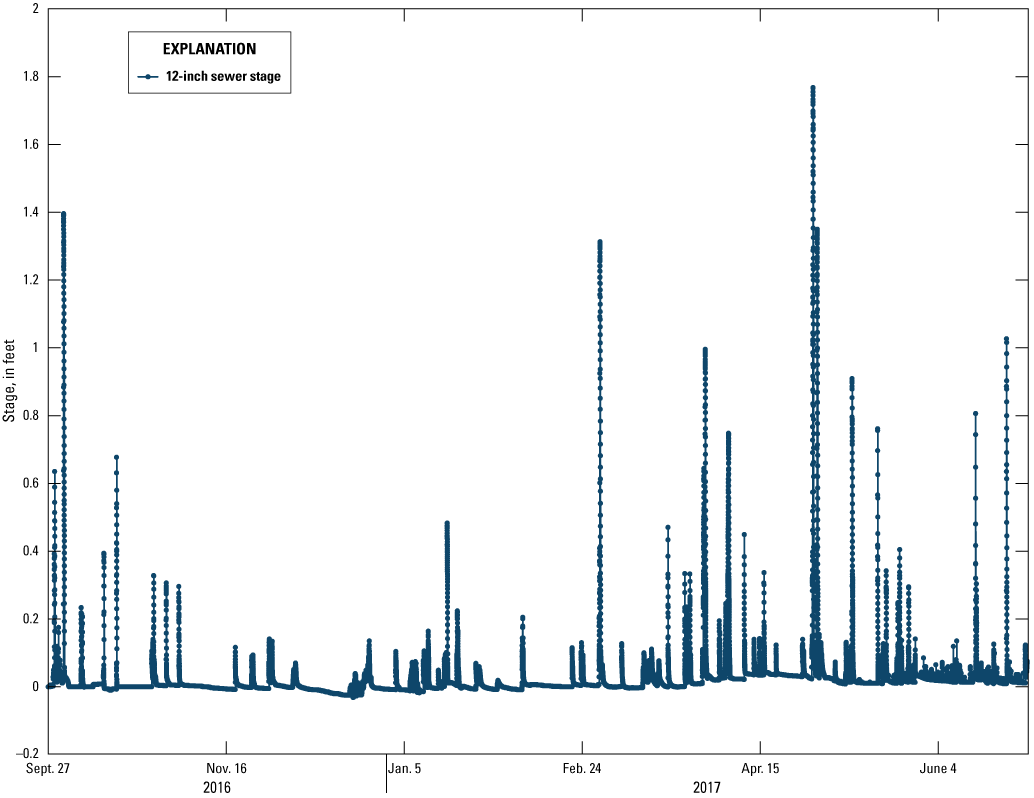
Stormwater stage measured in the 12-inch sewer line (station 414659087411901; U.S. Geological Survey, 2023) in response to various storm events at Donald L. Morrill Math and Science School from September 1, 2017, to July 1, 2017.
Two water quality samples of the stormwater runoff were collected from the 12-in. sewer line (football field) during storms on April 5 (fig. 19A) and May 19, 2017 (fig. 19B). These samples were collected during the falling limb of the hydrograph and analyzed by the USGS National Water Quality Laboratory for specific conductance (Fishman and Friedman, 1989), calcium (Fishman, 1993), chloride (Fishman and Friedman, 1989), nitrogen (Patton and Kryskalla, 2003), phosphorus (Patton and Kryskalla, 2003), and total organic carbon (Standard Methods Committee of the American Public Health Association and others, 2017). Analytical results are reported within each associated hydrograph (fig. 19A, B) (station 414659087411901; U.S. Geological Survey, 2023).
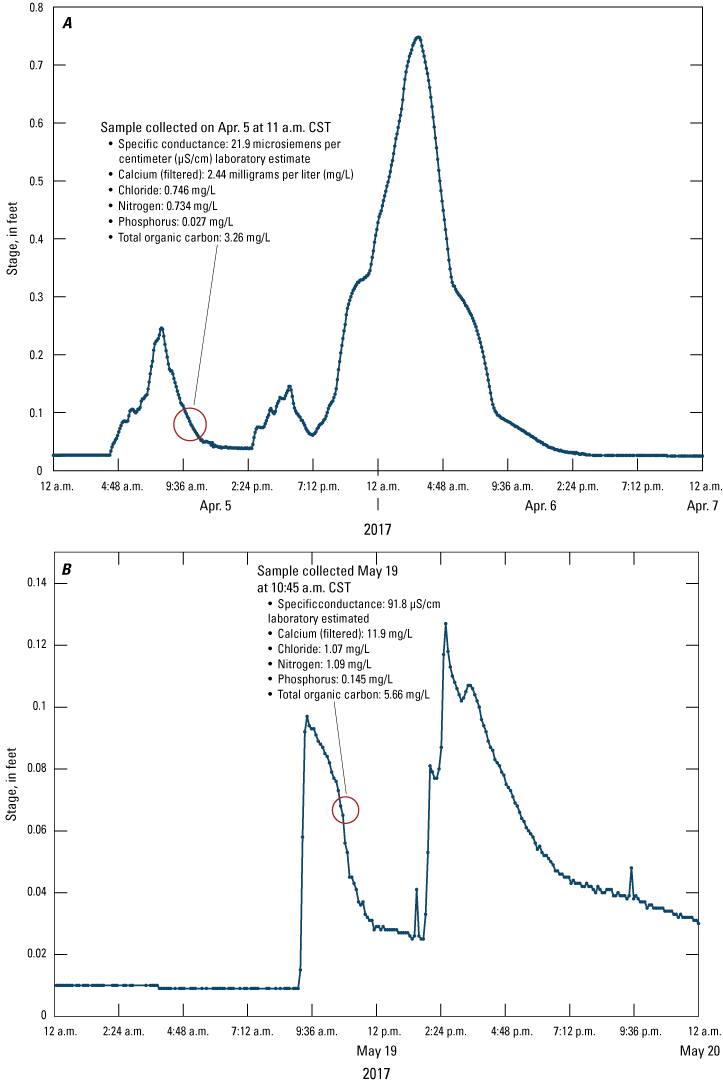
Results from discrete water quality samples (station 414659087411901; U.S. Geological Survey, 2023) collected from stormwater runoff in the 12-inch sewer line (football field) at Donald L. Morrill Math and Science School during (A) April 5, 2017, and (B) May 19, 2017, precipitation events. [CST, central standard time]
Rating curves for the Morrill 8-in. sewer line (permeable paver) were developed and calibrated on the low end by pumping a 50-gal drum of water into the 8-in. sewer line via the first manhole directly north of the monitored combined manhole at varying flow rates. The remaining part of the rating was then derived by taking more than 140 sensor recorded stage and discharge data points from more than 25 storms. The fitted regression equation for the 8-in. sewer line rating curve points results in a coefficient of determination value of greater than 0.95 (fig. 20).
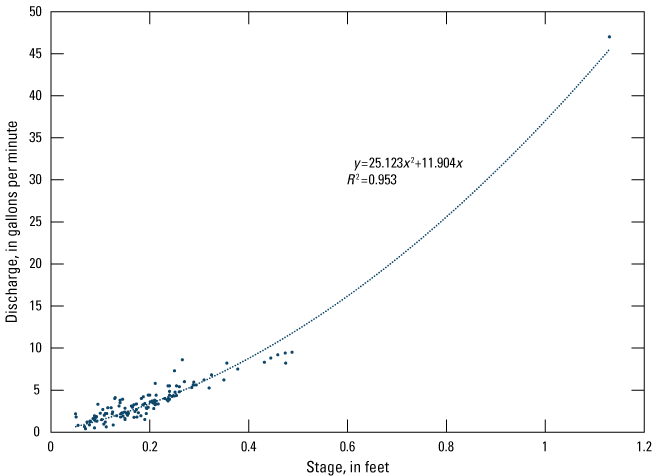
Rating curve for the 8-inch sewer line at Donald L. Morrill Math and Science School. [y, discharge, in gallons per minute; x, stage, in feet ; R2, coefficient of determination]
Discharge from the 12-in. sewer line (football field) was computed using the stage-to-discharge relation defined by the manufacturer for the installed weir (fig. 21A, B). Before calculating discharge, the stage data were corrected to remove the 0.20-ft stagnant water stage that remained behind the weir after October 26, 2016. However, similar to the weir ratings at Grissom, the discharge calculated from the 12-in. sewer calibrated weir rating yielded total discharges greater than the total precipitation recorded at the site during the monitoring period, and during several events, instantaneous discharges exceeded 250 gallons per minute, a highly unlikely value given the recorded rainfall intensities and fairly small drainage area. Discharge measured directly by the HACH FL900 sewer flow meters was “flashy” and did not depict a standard stormwater hydrograph for several events. Discharge values from the sewer meters ranged erroneously as high as 900 gallons per minute. As such, a site-specific rating curve was developed for the 12-in. sewer line at Morrill (fig. 21A, B).
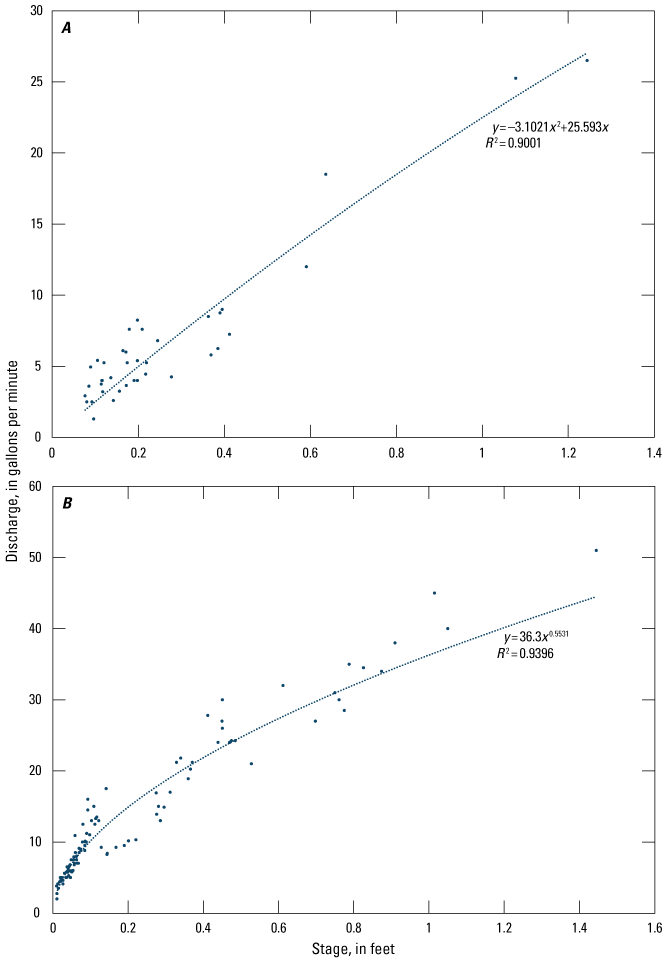
Rating curves for the 12-inch sewer at Donald L. Morrill Math and Science School (A) before and (B) after weir installation on October 24, 2016. [y, discharge, in gallons per minute; x, stage, in feet; R2, coefficient of determination]
Two approaches were used to develop a site-specific rating curve for the 12-in. sewer line. On September 7, 2016, USGS personnel visited the site in an attempt to calibrate the discharge rating of the V-notch weir. A 50-gal drum of water was pumped into the 12-in. sewer line, via the manhole west of the football field, for multiple attempts at varying flow rates. Stage and discharge rates were then recorded within the monitored combined manhole using calibrated 5-gal buckets. Values collected during these tests allowed for the development of a rating curve for stages below 0.05 ft. For stages above 0.05 ft, the rating curve was developed using stage and discharge from the flowmeter. During more than 20 events, hydrographs were calculated for discharge from the sensor. More than 150 stage/discharge data points from the falling limbs of these hydrographs were used to create two rating curves for the 12-in. weir. The first rating curve defined the stage/discharge relation before weir installation (fig. 21A), and the second rating curve defined the stage/discharge relation after weir installation on October 25, 2016 (fig. 21B). Only data from the falling limbs of the events were used because multiple readings were needed for the sensors to adjust to the rapid change from no flow to discharge during events. The falling limbs yielded the most consistent relations between stage and discharge with coefficient of determination values for each curve exceeding 0.9 (fig. 21A, B).
Groundwater levels were measured from February 28 to July 1, 2017, within the deep piezometer at the perimeter of the Morrill schoolyard. Groundwater levels responded to major storm events by increasing as much as 2 ft (fig. 22). During at least two instances, increases in groundwater levels were measured with no corresponding precipitation data (May 11 and June 17, 2017), possibly attributed to localized precipitation over the school and not recorded at the CCPN rain gage. A rapid increase in water level measured in February at the start of the record was the result of the sensor equilibrating after deployment.
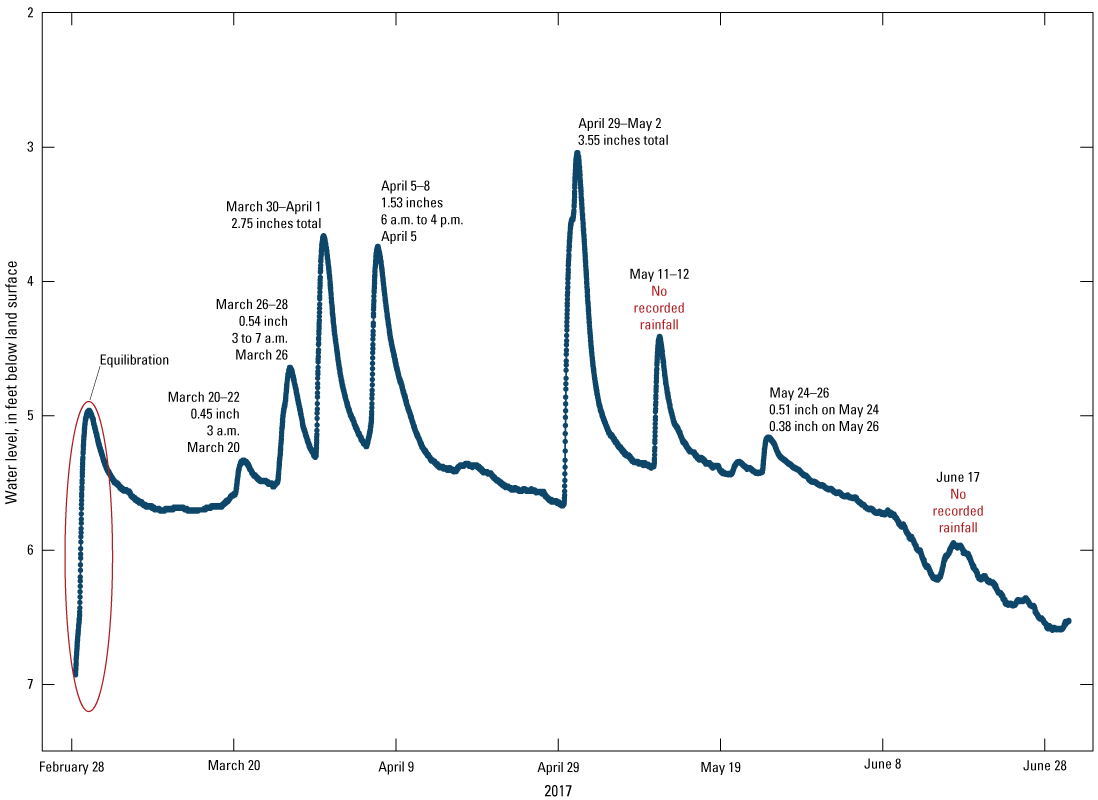
Groundwater levels recorded in the deep piezometer (station 414659087411701; U.S. Geological Survey, 2023) at the perimeter of Donald L. Morrill Math and Science School from February 28, 2017, to July 1, 2017.
Groundwater levels collected from the shallow piezometer within the retention basin beneath the football field revealed a steady increase in shallow groundwater starting in March 2017 (fig. 23). Water level fluctuated daily even without the effect of precipitation. During precipitation events, response in water level did not have the same sharp increase as observed in the deep piezometer. Large amounts of precipitation would only increase the water level by about 0.25 ft, and smaller amounts of precipitation are harder to discern amongst the natural “noise” of the data.
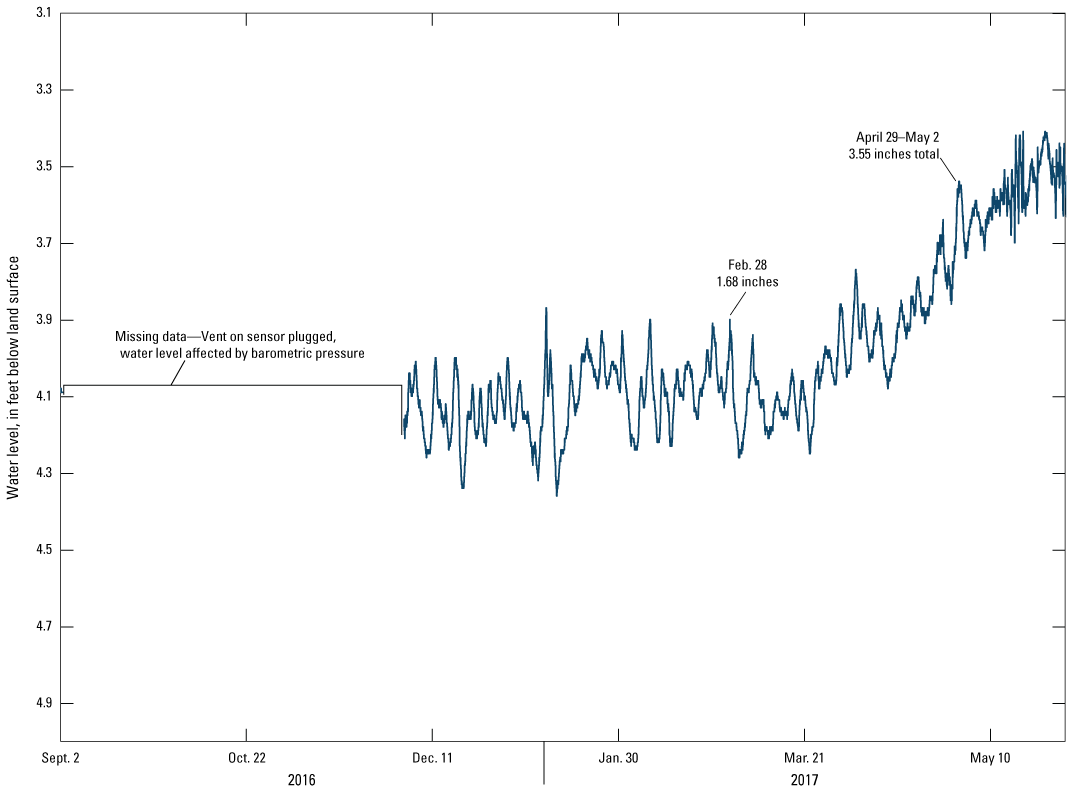
Groundwater levels recorded in the shallow piezometer (station 414659087411702; U.S. Geological Survey, 2023) in the football field at Donald L. Morrill Math and Science School from September 1, 2016, to July 1, 2017.
Events on February 28 and April 29, 2017, at Morrill were selected for a more detailed analysis of precipitation and stormwater runoff. Not all of the CCPN sites used in calculating total precipitation for the analysis period had high density data, so hourly precipitation data were obtained from rain gage 13 of the ISWS CCPN and compared to stage data measured in the 8-in. and 12-in. GI storm sewers. During the February 28, 2017, event, precipitation fell over a 7-hour period from 6 p.m. through March 1, 2017, at 1 a.m. (fig. 24). A total of 0.3 in. of rain fell during the first hour of the event, causing a rapid 0.4-ft increase in stage within the 8-in. storm sewer (permeable paver, fig. 24) and a less than 0.2-ft increase in stage within the 12-in. sewer (football field, fig. 24). Storm intensity ebbed the following hour and then steadily increased from 8 p.m. through 1 a.m. on March 1, 2017, when maximum hourly precipitation of 0.72 in. fell between midnight and 1 a.m. The 0.72 in. that fell in this 1-hour period caused a rapid increase in stage that surcharged the 8-in. and 12-in. sewer lines. The maximum stage recorded in the storm sewer lines was 1.52 ft and 1.27 ft in the 8-in. and 12-in. sewer lines, respectively. After the storm passed, stage in both sewer lines took several hours to return to baseline (dry) conditions. A total of 3,300 gal and 2,600 gal were discharged from the 8-in. and 12-in. sewer lines, respectively, during the event. Some stormwater continued to slowly trickle after the event ceased on March 1 around 1 a.m. No change in groundwater levels was recorded within the deep piezometer at the perimeter during the event. There are no background groundwater levels at the deep piezometer before the storm because the equipment had just been installed; however, recorded groundwater levels increased by at least 2 ft, peaking at about midnight on March 2, 2017 (fig. 22).
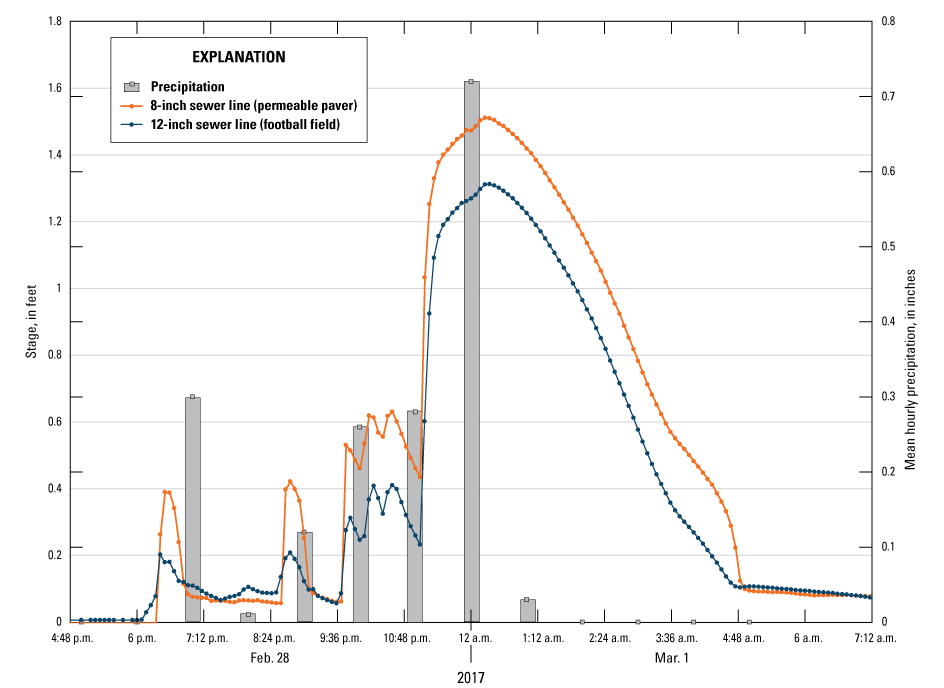
Stage from the 8-inch and 12-inch sewer lines (station 414659087411901; U.S. Geological Survey, 2023) and precipitation from site 13 of the Cook County Precipitation Network (Illinois State Water Survey, 2022) for the February 28, 2017, storm event recorded at Donald L. Morrill Math and Science School.
Similar trends were recorded during the April 29, 2017, event at Morrill (fig. 25). Precipitation fell over a 12-hour period from 11 a.m. to 11 p.m. Precipitation gradually increased from the start of the event, peaking at about 6 p.m. with 0.46 in. of rainfall recorded, and then steadily fell until the end of the event at about 11 p.m. Stormwater stage measured within the storm sewer lines steadily increased following the storm intensity. Peak stage within both sewer lines was recorded at about 8:20 p.m. during a secondary peak in storm intensity during the falling limb of the storm. Maximum stage recorded during the event was measured at 1.93 ft and 1.77 ft within the 8-in. and 12-in. storm sewer lines, respectively, during the event. A total of about 5,340 gal and 4,100 gal were discharged from the 8-in. and 12-in. storm sewer lines, respectively. Some stormwater continued to discharge after the event, which ended at about 11 p.m. Groundwater levels measured within the shallow piezometer in the football field recorded a small increase in groundwater levels of about 0.3 ft; however, the accuracy of the increase in water level is difficult to discern from possible effects of barometric pressure. An increase in water level of about 2 ft was recorded at the deep piezometer. Groundwater levels began to increase at about 7 p.m. on April 29, 2017, and peaked at about 8 p.m. on May 1, 2017 (fig. 22).
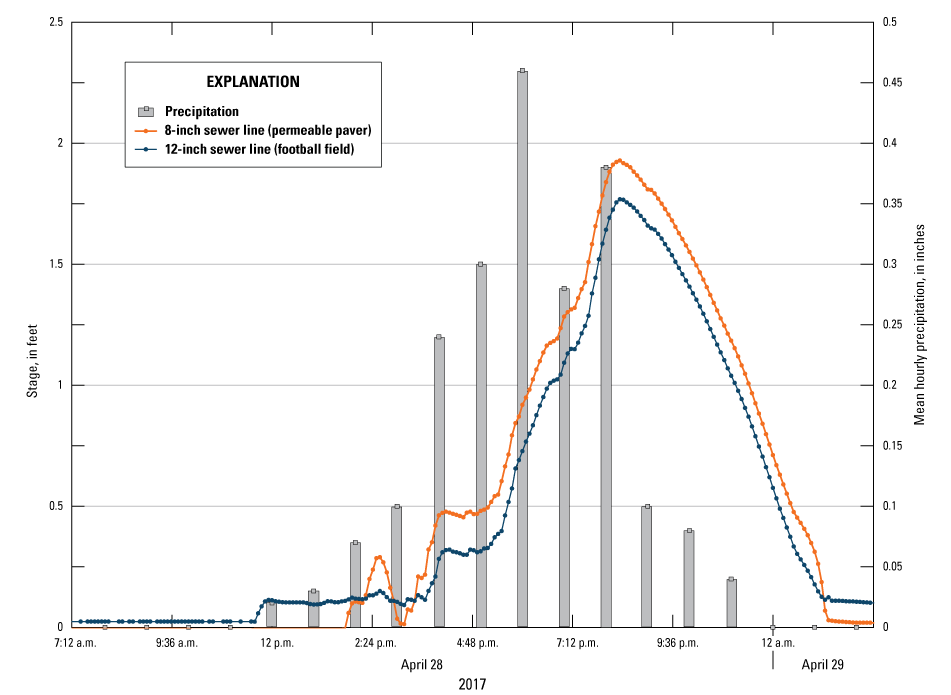
Stage from the 8-inch and 12-inch sewer lines (station 414659087411901; U.S. Geological Survey, 2023) and precipitation from site 13 of the Cook County Precipitation Network (Illinois State Water Survey, 2022) for the April 29, 2017, storm event recorded at Donald L. Morrill Math and Science School.
Minor precipitation events generated measurable runoff in both GI sewer lines at Morrill, and both sewer lines recorded surcharged stage values greater than the pipe diameter for multiple events. During the study period of September 1, 2016, to July 1, 2017, a total of 29.94 in. of rain fell on the Morrill schoolyard. This equates to about 150,000 cubic feet or 1,120,000 gal of water falling on the pervious GI part of the schoolyard. The USGS developed site-specific rating curves for each storm sewer line based on field calibrations and sensor recorded stage/discharge data from the falling limbs of ideal event hydrographs. Total discharge from the 8-in. sewer line during the monitoring period was about 178,000 gal. Total discharge from the 12-in. storm sewer line was about 635,000 gal. There was a range of 0.01–0.02 ft of corrected stage when stormwater did not flow over the V-notch weir because of the presence of debris. This caused discharge calculated from these low stages to be “noisy,” negative, or unreliable, and the values were therefore not included in the total. Total stormwater discharged from the site during the monitoring period was about 813,000 gal and was subtracted from the total amount of precipitation calculated to have fallen in the study area to yield about 309,000 gal that were captured in the surrounding ground and not discharged into the sewer from the collective storms. This equates to about a 72.5-percent discharge into the sewer system and 27.5 percent captured by the surrounding ground. This low capture rate may be due to the soil at Morrill being primarily clay material, thus not allowing water to infiltrate out of the GI into the surrounding ground. In addition, the sewer line coming from the GI under the football field was at the bottom of the engineered reservoir, and this design seemed to allow water to flow out of the line before being stored and absorbed by the retention basin. Analysis of the selected events on February 28 and April 29, 2017, indicated that retention time of stormwater discharged from the Morrill GI is limited. A response in stage to precipitation, in most cases, occurs within 30 minutes, and peak discharge trails peak precipitation by about 1 hour or less.
Summary
The Chicagoland area has issues with flooding because of the large amounts of impervious surfaces that cover the area. Many of these surfaces include schoolyards. The Space to Grow program helps transform aging and neglected schoolyards into outdoor community spaces with the goal of promoting health and learning while addressing neighborhood flooding issues. In cooperation with the Metropolitan Water Reclamation District of Greater Chicago, the U.S. Geological Survey deployed equipment at two Chicago Public Schools, Virgil I. Grissom Elementary School and Donald L. Morrill Math and Science School, to measure precipitation, groundwater levels, and stormwater runoff volumes from September 1, 2016, to July 1, 2017.
Two of the largest storms that occurred during the monitoring period (February 28 and June 23, 2017, at Grissom and February 28 and April 29, 2017, at Morrill) were further analyzed. The February 28 and June 23, 2017, events at Grissom indicated a rapid increase in stage coming from the impervious running track, and the combined manhole structure was filled within 5 minutes in response to this initial flush of water. If intense rain falls over a longer period of time, the sewer can become overtopped before the green infrastructure (GI) reservoirs are full, as observed during the February 28, 2017, event. The surge into the GI reservoirs could not keep up with the rapid amount of water entering the combined manhole structure from the impervious running track. The February 28 and April 29, 2017, events at Morrill recorded a steady increase in sewer stage following storm intensity, and stormwater continued to discharge from the GI sewer lines even after the storm ended.
A total of 30.98 inches (in.) of rain was recorded to have fallen over the Grissom schoolyard. This equates to roughly 933,000 gallons (gal) of water falling on the schoolyard. No discharge was measured coming from the GI sewer lines, which indicated that the lines were able to accept 100 percent of stormwater during the monitoring period. Native soil beneath Grissom consisted of about 10.5 feet of sandy deposits that allowed rapid infiltration of water captured by the GI. A total of 29.94 in. of rain was recorded to have fallen over the Morrill schoolyard. This equates to roughly 1,120,000 gal of water falling on the schoolyard. Total discharge from the 8-in. and 12-in. sewer lines coming from the GI parts of the schoolyard amounted to about 178,000 gal and 635,000 gal, respectively. This means that from more than 1 million gallons of water that had fallen on the schoolyard, only 27.5 percent was captured and stored by the GI. The remaining 72.5 percent discharged into the sewer system. Unlike Virgil I. Grissom Elementary School, the soil profile at Donald L. Morrill Math and Science School consisted of about 5 ft of clay loam overlying stiff blue clay to a depth of at least 12 ft. Additionally, at Morrill, the sewer line coming from the GI under the football field was at the bottom of the reservoir. This design seemed to allow water to flow out of the line before being absorbed by the retention basin. Analysis of individual storms indicated that retention time of stormwater is limited where peak discharge occurred about 1 hour or less after peak precipitation.
References Cited
Cunningham, W.L., and Schalk, C.W., comps., 2011, Groundwater technical procedures of the U.S. Geological Survey: U.S. Geological Survey Techniques and Methods, book 1, chap. A1, 151 p., accessed July 2023 at https://doi.org/10.3133/tm1A1.
Fishman, M.J., ed., 1993, Methods of analysis by the U.S. Geological Survey National Water Quality Laboratory—Determination of inorganic and organic constituents in water and fluvial sediments: U.S. Geological Survey Open-File Report 1993–125, 217 p. [Also available at https://doi.org/10.3133/ofr93125.]
Fishman, M.J., and Friedman, L.C., 1989, Methods for determination of inorganic substances in water and fluvial sediments: U.S. Geological Survey Techniques of Water-Resources Investigations, book 5, chap. A1, 545 p. [Also available at https://doi.org/10.3133/twri05A1.]
Illinois State Geological Survey, 2023, ILWATER: Illinois State Geological Survey digital data, accessed August 4, 2023, at https://prairie-research.maps.arcgis.com/apps/webappviewer/index.html?id=e06b64ae0c814ef3a4e43a191cb57f87.
Illinois State Water Survey, 2022, Cook County Precipitation Network—Data collection: Illinois State Water Survey database, accessed April 24, 2023, at https://www.isws.illinois.edu/data/ccprecipnet/gageloc.asp.
Mathany, T.M., Saraceno, J.F., and Kulongoski, J.T., 2019, Guidelines and standard procedures for high-frequency groundwater-quality monitoring stations—Design, operation, and record computation: U.S. Geological Survey Techniques and Methods, book 1, chap. D7, 54 p., accessed July 2023 at https://doi.org/10.3133/tm1D7.
Patton, C.J., and Kryskalla, J.R., 2003, Methods of analysis by the U.S. Geological Survey National Water Quality Laboratory—Evaluation of alkaline persulfate digestion as an alternative to Kjeldahl digestion for determination of total and dissolved nitrogen and phosphorus in water: U.S. Geological Survey Water-Resources Investigations Report 2003–4174, 33p. [Also available at https://doi.org/10.3133/wri034174.]
Space to Grow, 2023, Space to Grow: Space to Grow web page, accessed January 9, 2023, at https://www.spacetogrowchicago.org.
Standard Methods Committee of the American Public Health Association, American Water Works Association, and Water Environment Federation, 2017, 5310 total organic carbon, in Lipps, W.C., Baxter, T.E., and Braun-Howland, E., eds., Standard methods for the examination of water and wastewater: Washington, D.C., American Public Health Association Press, [variously paged]. [Also available at https://doi.org/10.2105/SMWW.2882.104.]
U.S. Geological Survey, 2023, USGS water data for the Nation: U.S. Geological Survey National Water Information System database, accessed September 2023 at https://doi.org/10.5066/F7P55KJN.
U.S. Geological Survey, [variously dated], National field manual for the collection of water-quality data: U.S. Geological Survey Techniques and Methods, book 9, chaps. A1–A9, [variously paged], accessed April 5, 2023, at https://water.usgs.gov/owq/FieldManual/.
Wagner, R.J., Boulger, R.W., Jr., Oblinger, C.J., and Smith, B.A., 2006, Guidelines and standard procedures for continuous water-quality monitors—Station operation, record computation, and data reporting: U.S. Geological Survey Techniques and Methods, book 1, chap. D3, 51 p., accessed July 2023 at https://doi.org/10.3133/tm1D3.
Appendix 1. Selected Boring Logs Obtained from the Illinois State Geological Survey
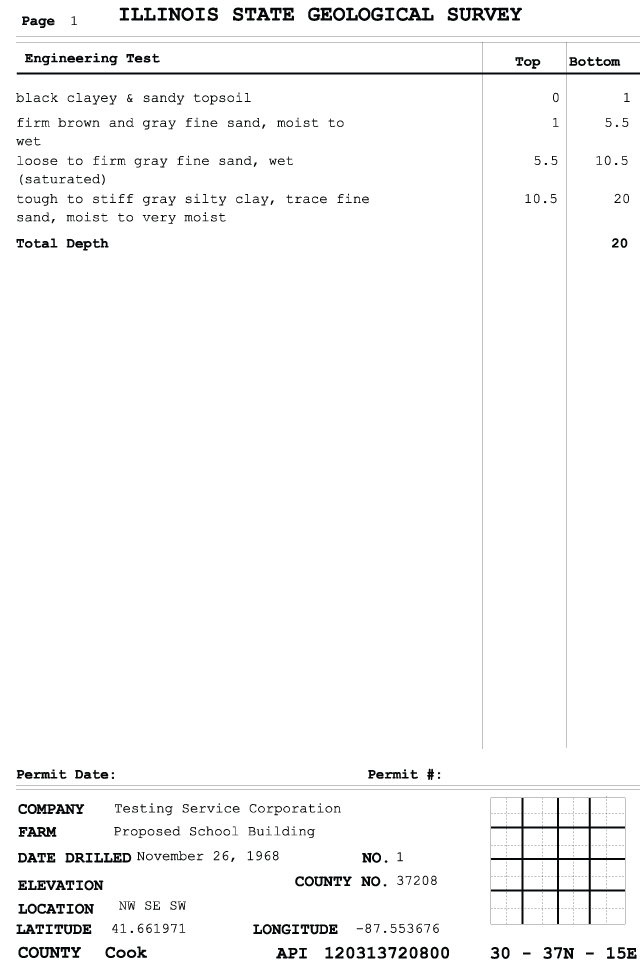
Example geological well log from Virgil I. Grissom Elementary School (Illinois State Geological Survey, 2023).
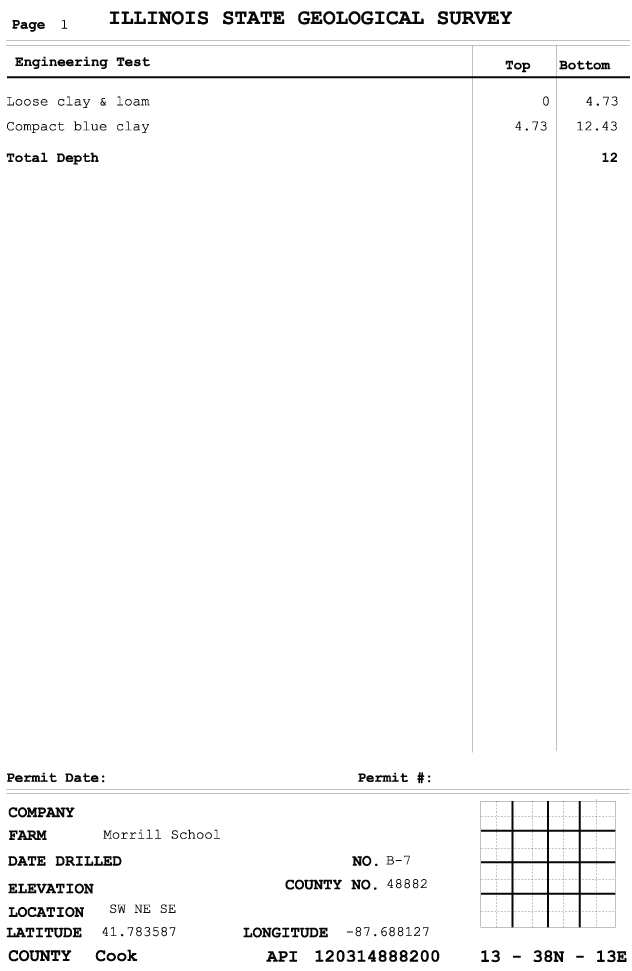
Example geological well log from Donald L. Morrill Math and Science School (Illinois State Geological Survey, 2023).
Reference Cited
Illinois State Geological Survey, 2023, ILWATER: Illinois State Geological Survey digital data, accessed August 4, 2023, at https://prairie-research.maps.arcgis.com/apps/webappviewer/index.html?id=e06b64ae0c814ef3a4e43a191cb57f87.
Appendix 2. Piezometer Construction Logs
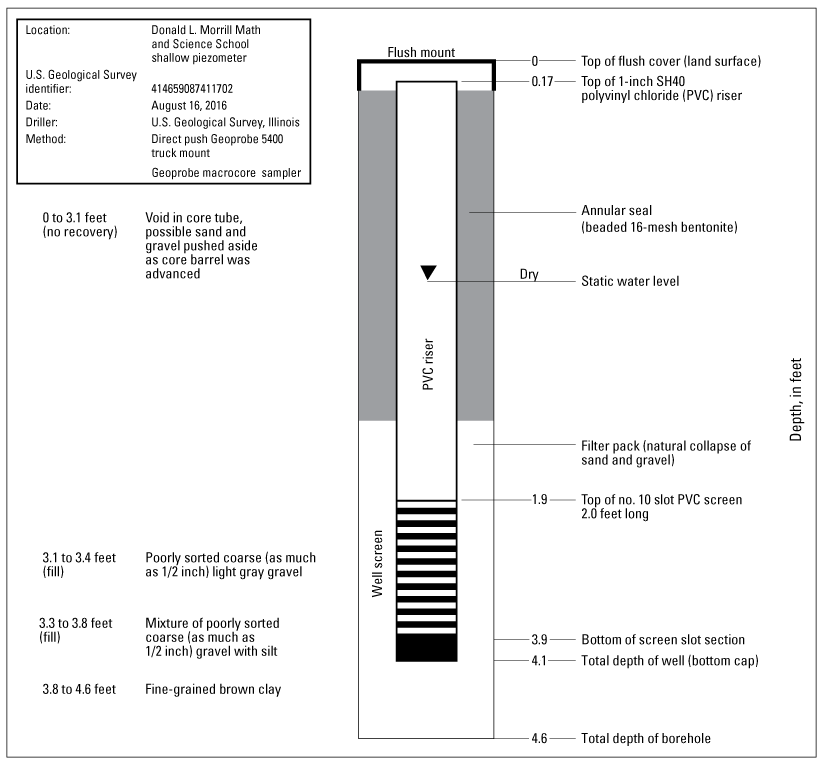
Construction diagram of the Virgil I. Grissom Elementary School deep piezometer.
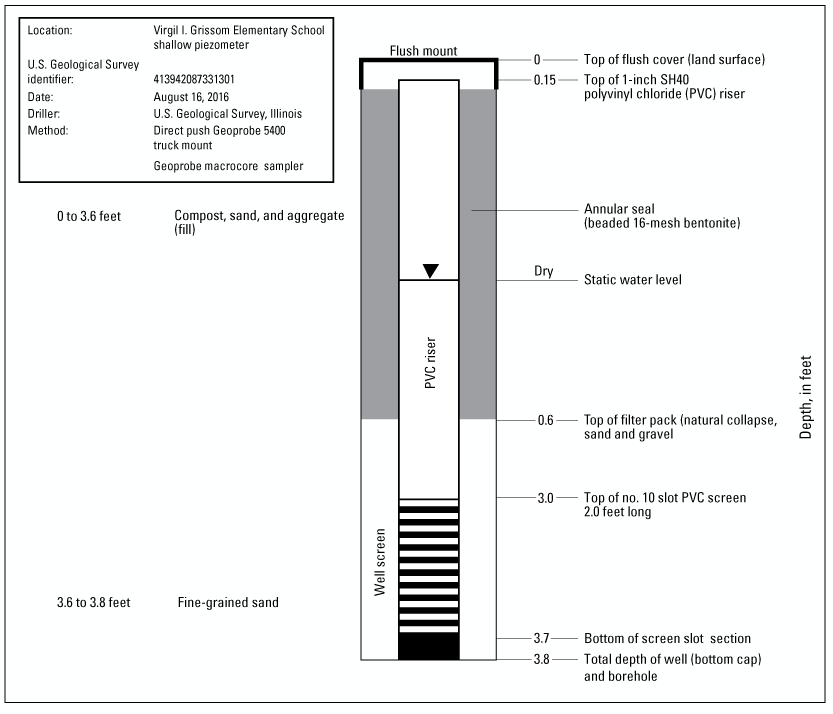
Construction diagram of the Virgil I. Grissom Elementary School shallow piezometer.
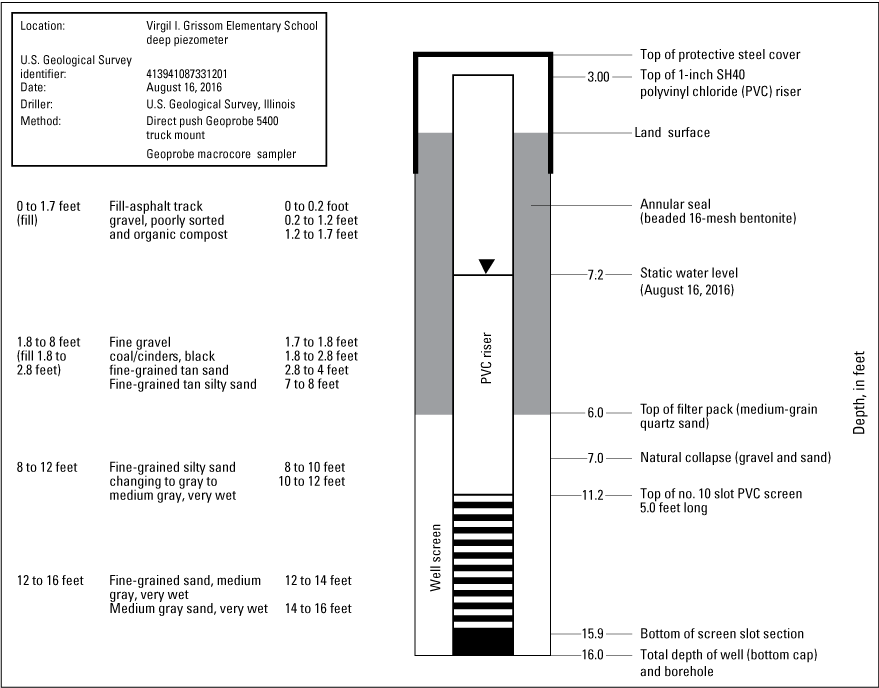
Construction diagram of the Donald L. Morrill Math and Science School shallow piezometer.
Conversion Factors
U.S. customary units to International System of Units
Temperature in degrees Celsius (°C) may be converted to degrees Fahrenheit (°F) as follows:
°F = (1.8 × °C) + 32.
Datums
Vertical coordinate information is referenced to the North American Vertical Datum of 1988 (NAVD 88).
Horizontal coordinate information is referenced to the North American Datum of 1983 (NAD 83).
Supplemental Information
Specific conductance is given in microsiemens per centimeter at 25 degrees Celsius (µS/cm at 25 °C).
Concentrations of chemical constituents in water are given in either milligrams per liter (mg/L) or micrograms per liter (µg/L).
For more information about this publication, contact:
Director, USGS Central Midwest Water Science Center
1400 Independence Road
Rolla, MO 65401
573–308–3667
For additional information, visit: https://www.usgs.gov/centers/cm-water
Publishing support provided by the
Rolla Publishing Service Center
Disclaimers
Any use of trade, firm, or product names is for descriptive purposes only and does not imply endorsement by the U.S. Government.
Although this information product, for the most part, is in the public domain, it also may contain copyrighted materials as noted in the text. Permission to reproduce copyrighted items must be secured from the copyright owner.
Suggested Citation
Bailey, C.R., Soderstrom, C.M., and Duncker, J.J., 2024, Monitoring and assessment of urban stormwater best management practices at selected Chicago public schools in Chicago, Illinois, from September 1, 2016, to July 1, 2017: U.S. Geological Survey Scientific Investigations Report 2024–5036, 40 p., https://doi.org/10.3133/sir20245036.
ISSN: 2328-0328 (online)
Study Area
Publication type | Report |
---|---|
Publication Subtype | USGS Numbered Series |
Title | Monitoring and assessment of urban stormwater best management practices at selected Chicago public schools in Chicago, Illinois, from September 1, 2016, to July 1, 2017 |
Series title | Scientific Investigations Report |
Series number | 2024-5036 |
DOI | 10.3133/sir20245036 |
Year Published | 2024 |
Language | English |
Publisher | U.S. Geological Survey |
Publisher location | Reston, VA |
Contributing office(s) | Central Midwest Water Science Center |
Description | Report: viii, 40 p.; Dataset |
Country | United States |
State | Illinois |
County | Cook County |
City | Chicago |
Online Only (Y/N) | Y |
Additional Online Files (Y/N) | N |
Google Analytic Metrics | Metrics page |