Nitrogen Load Estimates From Six Nonpoint Sources on Long Island, New York, From 1900 To 2019
Links
- Document: Report (4.25 MB pdf) , HTML , XML
- Data Release: USGS data release - Annual nitrogen load estimates from six nonpoint sources on Long Island, New York, from 1900 to 2019
- Download citation as: RIS | Dublin Core
Abstract
Estimates of nitrogen loading from nonpoint sources on Long Island, New York, at or just below the land surface, are essential for assessing the current and future effects of nitrogen on the island’s drinking water and fresh and marine surface receiving waters. Annual estimates of nitrogen loading for the 120 years from 1900 to 2019 for major nonpoint nitrogen sources—septic systems, residential fertilizer, agricultural fertilizer, livestock waste, pet waste, and atmospheric deposition—were made by using a geographic information system to analyze, visualize, and process data sources, and format output data. This analysis provided spatial and temporal estimates of nitrogen loading derived from each nonpoint source at a 500- by 500-foot gridded resolution and represents the total mass of nitrogen applied on, or just below, the land surface annually from 1900 to 2019. These mass estimates are considered unattenuated as they do not reflect the various mechanisms of nitrogen loss, such as plant uptake, overland runoff, and chemical transformations in the soil and unsaturated zones that likely reduce the amount of nitrogen that reaches the water table.
Island-wide and countywide summaries of the estimated nitrogen loading were analyzed to describe the long-term average nitrogen totals and the contributions from the six nonpoint sources. The island-wide average annual nitrogen load from 1900 to 2019 was 14.92 million kilograms (Mkg) nitrogen, which represents the aggregate of individual contributions from septic systems (4.15 Mkg), residential fertilizer (3.28 Mkg), agricultural fertilizer (3.19 Mkg), livestock waste (1.22 Mkg), pet waste (0.98 Mkg), and atmospheric deposition (2.10 Mkg). The island-wide average annual nitrogen load, normalized by area, was 4,100 kilograms nitrogen per square kilometer (kg N/km2), which represents the aggregate of individual contributions from septic systems (1,100 kg N/km2), residential fertilizer (910 kg N/km2), agricultural fertilizer (880 kg N/km2), livestock waste (340 kg N/km2), pet waste (270 kg N/km2), and atmospheric deposition (580 kg N/km2).
Introduction
The aquifer system beneath Long Island, New York, has been designated a sole-source aquifer by the U.S. Environmental Protection Agency (EPA). Human activities on the land surface can affect the water quality of the aquifer system and in surface waters that receive groundwater discharge. Nitrate concentrations that exceed the national drinking-water standard of 10 milligrams per liter as nitrogen (mg/L as N) in some areas (Scorca and Monti, 2001; Monti and Scorca, 2003) and eutrophication from excess nitrogen have been observed in the region’s coastal waters. Historical estimates of nitrogen contributions to the aquifer from nonpoint sources—septic systems, residential fertilizer use, agricultural fertilizer use, livestock waste, pet waste, and atmospheric deposition—are essential for assessing the effects of nitrogen on drinking water and on nutrient loading to fresh and marine waters.
In 2016, the U.S. Geological Survey (USGS) National Water-Quality Assessment Program began an investigation of the susceptibility to contamination of the North Atlantic Coastal Plain aquifer system, which includes Long Island, N.Y. As part of this larger effort, the annual load of nitrogen was estimated for six nonpoint sources that may have contributed nitrogen to the Long Island aquifer system between 1900 and 2019. These loading estimates of nitrogen are unattenuated and represent the mass of nitrogen that could potentially enter the aquifer system each year and do not account for processes that may attenuate or decrease loading in the soil and unsaturated zones prior to entering the aquifer at the water table. These estimates were derived from various data sources to quantify the loading from the six nonpoint sources.
Location and Physical Setting
Long Island is in the southeasternmost part of New York State and extends east-northeastward roughly parallel to the Connecticut coastline. It is surrounded by salt water and is bounded by the Atlantic Ocean on its eastern and southern shore, the Long Island Sound on its northern shore, and New York Bay and the East River on its western shore (fig. 1). The total length of Long Island is about 193 kilometers (km), and its maximum width is 37 km. The total land area (including smaller islands within the administrative boundaries of the island but excluding the bordering bays) is about 3,608 square kilometers (km2; Cohen and others, 1968).
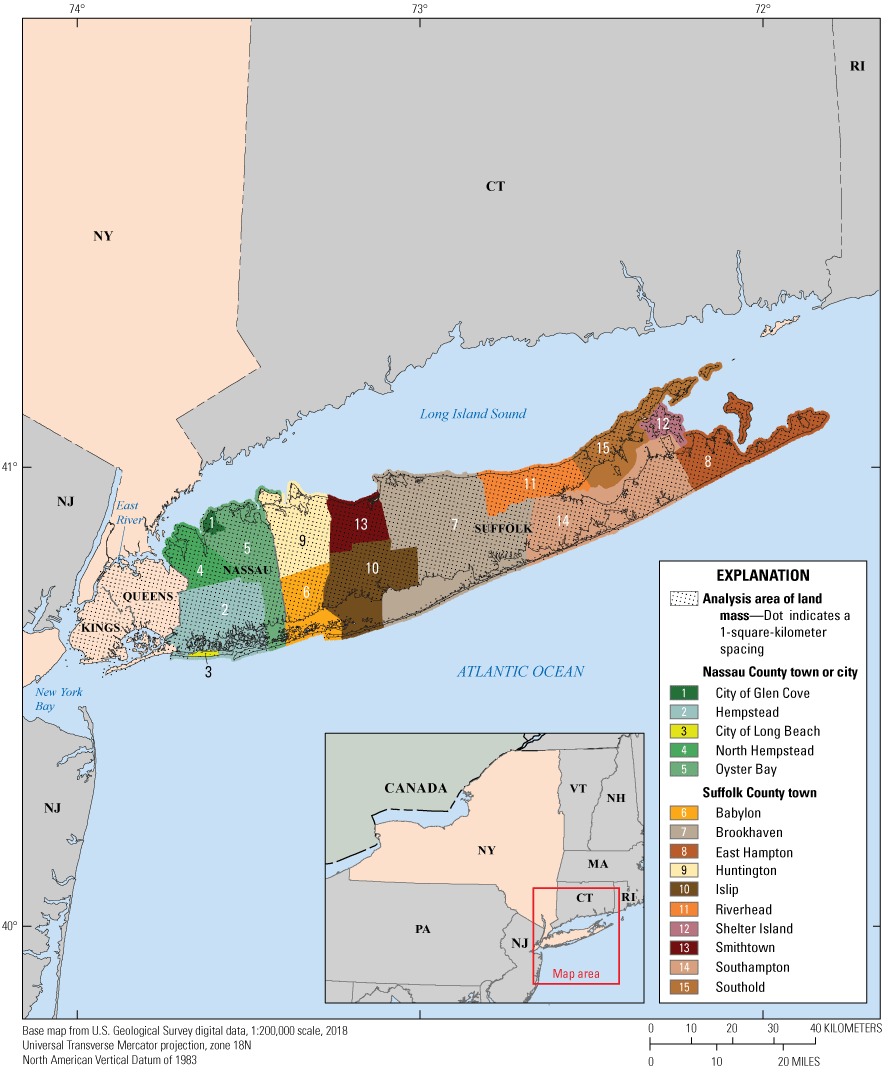
Map showing location of study area and land area on Long Island, New York.
Kings and Queens Counties, on the western end of Long Island, are densely populated. Kings County is the westernmost county and has a land area of about 184 km2. Queens County is bordered by Kings County on the west and Nassau County on the east and has a greater land area than Kings County, about 282 km2. Nassau County has about 762 km2 of land area (26 km2 of which is marshland) and is divided into three towns—Hempstead, North Hempstead, and Oyster Bay—and two cities—Glen Cove (within Oyster Bay Township) and Long Beach (within Hempstead Township). Suffolk County, the easternmost county, shares a border with Nassau County and has about 2,380 km2 of land area (18 km2 of which is marshland); Suffolk County is divided into 10 towns—Babylon, Brookhaven, East Hampton, Huntington, Islip, Riverhead, Shelter Island, Smithtown, Southampton, and Southold (fig. 1).
In 2020, Kings, Queens, and Nassau Counties had population densities that ranked in the top 20 in the entire United States (U.S. Census Bureau, 2021a). However, 100 years ago, more than 40 percent of Long Island was farms and pastures, and the urbanized areas were primarily in Kings and western Queens Counties (U.S. Department of Agriculture, 2019; National Agricultural Statistics Service [NASS], 2020).
Purpose and Scope
This report presents the data and methods used to estimate annual nitrogen loading from 1900 to 2019 from available land use and land cover data, population and agricultural census data, and sewer area information. The methods described in this report made the diverse sources of information comparable and useful to consistently estimate annual nitrogen load for the major nonpoint sources throughout the analysis. The results of this analysis include both island-wide and countywide trends and annual nonpoint source nitrogen loads from 1900 to 2019. The results also include estimates of the spatial variability of each of the six nitrogen source types across Long Island. Examples of this spatial variability for selected years are included in this report. The complete dataset of spatially variable nitrogen sources for each year are documented in a U.S. Geological Survey data release (Monti, 2024), which includes comma delimited files of gridded nitrogen data at a resolution of 500 feet (ft).
Previous Studies
Previous studies of the occurrence and concentration of nitrogen in the groundwater and surface waters of Long Island include Perlmutter and Koch (1975), Katz and others (1980), Ragone and others (1980), and Bleifuss and others (2012). The relationship between land use and water quality was assessed by Eckhardt and others (1989), LeaMond and others (1992), Eckhardt and Stackelberg (1995), Stackelberg (1995), and Pearsall (1996). Estimates of nonpoint source nitrogen loads to Long Island Sound were developed by using watershed models (Georgas and others, 2009). Scorca and Monti (2001) and Monti and Scorca (2003) estimated total nitrogen loads from groundwater and surface water entering Long Island Sound and the south shore estuaries.
Related studies in the Chesapeake Bay watershed are applicable to the Long Island aquifer system. Bachman and Phillips (1996) sampled the base flow of streams representing a variety of watershed characteristics during selected seasons and estimated nitrogen loads from the calculated median instantaneous yields. Phillips and others (1999) evaluated nitrate loads discharged from groundwater to streams in a wide range of hydrogeomorphic regions throughout the Chesapeake Bay watershed and estimated the residence time of groundwater in the aquifer system prior to discharging to streams. Bachman and others (1998) also developed relationships between groundwater discharge, base-flow nitrate yields, and hydrogeomorphic regions in the Chesapeake Bay watershed.
Nitrogen loads from agricultural fertilizer have been described in several reports that include each county for the contiguous United States: Alexander and Smith (1990) focused on the agricultural use of fertilizer from 1945 to 1985, Gronberg and Spahr (2012) estimated both agriculture and residential use of commercial fertilizer from 1987 to 2012, Cao and others (2018) provided county-level estimates of nitrogen for the contiguous United States from 1850 to 2015, and Falcone (2021) estimated nitrogen and phosphorus from fertilizer and manure from 1950 to 2017, also at the county level.
Method and Approach
Estimates of annual nitrogen load for the 120 years from 1900 to 2019 were made by using a geographic information system (GIS). The GIS incorporated spatial data available as either raster grids, polygon outlines, or tabular data. The spatial data available as a raster grid included land use and land cover, impervious surfaces, and atmospheric deposition (table 1). Polygon outlines represented the areas covered by sewer system areas, building footprints, golf courses, and administrative boundaries of counties, towns, and cities. Tabular data from the U.S. Census Bureau (2016, 2021a) and U.S. Department of Agriculture (USDA; 2019) collections were coupled to polygon outlines and raster grids by the use of tabular joins and GIS overlay functions.
Table 1.
Raster data available for each category and time period in this analysis.[m, meter; —, not used; NASS, National Agricultural Statistics Service]
Raster cell resolution (m) | Time periods covered | Data source | ||
---|---|---|---|---|
1900–37 | 1938–2005 | 2006–19 | ||
250 | 1938 | 1938–92 | — | Sohl and others (2016, 2018a) |
250 | — | 1993–2005 | — | Sohl and others (2014, 2018b) |
30 | — | — | 2006 (for 2006–07) | Fry and others (2011) |
30 | — | — | 2008–19 | NASS (2019)1 |
60 | 1974 | 1974 | — | Falcone (2017) |
60 | — | 1982 | — | Falcone (2017) |
60 | — | 1992 | — | Falcone (2017) |
60 | — | 2002 | — | Falcone (2017) |
60 | — | 2012 | 2012 | Falcone (2017) |
1,000 | 1900–37 | 1938–99 | — | Hember (2018a, b) |
4,000 | — | 2000–05 | 2006–19 | Schwede and Lear (2014) |
The land use and land cover data changed spatially and temporally during the 120-year period. Human activity was the primary driver behind the changes in spatial distribution of nitrogen loading from anthropogenic sources: septic systems, residential fertilization, agricultural fertilization, pet waste, and livestock waste. The sixth nonpoint source of nitrogen—atmospheric deposition—was also estimated by using GIS extrapolation functions to expand on previously published results (table 1).
The annual nitrogen load represents the sum of nitrogen sources, in kilograms per year from people, pets, and livestock, or in kilograms per hectare per year from atmospheric deposition, agriculture, and residential fertilization. Although the loading of nitrogen from septic systems, residential fertilization, pets, and livestock sources likely changed over the 120 years of the analysis period, those variations would be small relative to the much larger changes in total population and land use. Therefore, the changes were deemed not significant at the scale of this analysis, and their loading was held constant throughout the period. As a result, the estimated nitrogen load was calculated as a constant function of the changing land use, population, and livestock data. In contrast, agricultural fertilization rates likely varied more throughout the study period. Although there are many unknowns, including changes in fertilizer type and application rates and methods, estimates of agricultural nitrogen loading were developed to account for the transition from organic (manure) to inorganic (synthetic) fertilizer in the mid-20th century. These varying application rates were applied to each of the major crops grown on Long Island between 1900 and 2019.
The administrative boundaries of each county, town, or city were used to define the regions included in this analysis. County regions were joined to a formatted table of agricultural data from the U.S. Department of Agriculture (NASS, 2020), and town and city regions were joined to a formatted table of decennial census data (U.S. Census Bureau, 2016, 2021a). The annual nitrogen loading was calculated by processing data described in the “Description of Datasets Used in the Analysis” section of this report and using a sequence of procedures, described in “Estimating Nitrogen Loads” section, that combined the average nitrogen loading with the attributes for each region. This information was further combined with the corresponding land use category, imperviousness coefficients, and existing sewer system data. This set of procedures was performed for each annual dataset, generating an estimated nitrogen load from the six nonpoint sources at each land use raster cell.
The results of this analysis provided spatially distributed estimates of nitrogen loading for each nonpoint source (septic systems, residential fertilizer, agricultural fertilizer, livestock waste, pet waste, and atmospheric deposition) for each year of the study. This estimated nitrogen load was gridded at a resolution of 500 ft, representing the annual nitrogen load within each 250,000-ft2 cell (fig. 2). These cell values were aggregated to create island-wide and countywide regional nitrogen load estimates. Each regional load was divided by the area of the respective region to calculate an area-normalized load for each year between 1900 and 2019. These annual estimates do not account for the various mechanisms of nitrogen loss, such as plant uptake, other chemical transformations in the soil, and unsaturated zones that likely attenuate the amount of nitrogen that reaches the water table. Therefore, these loads should be considered maximum potential loads that require adjustment before use in estimating actual nitrogen loads to wells and receiving waters.
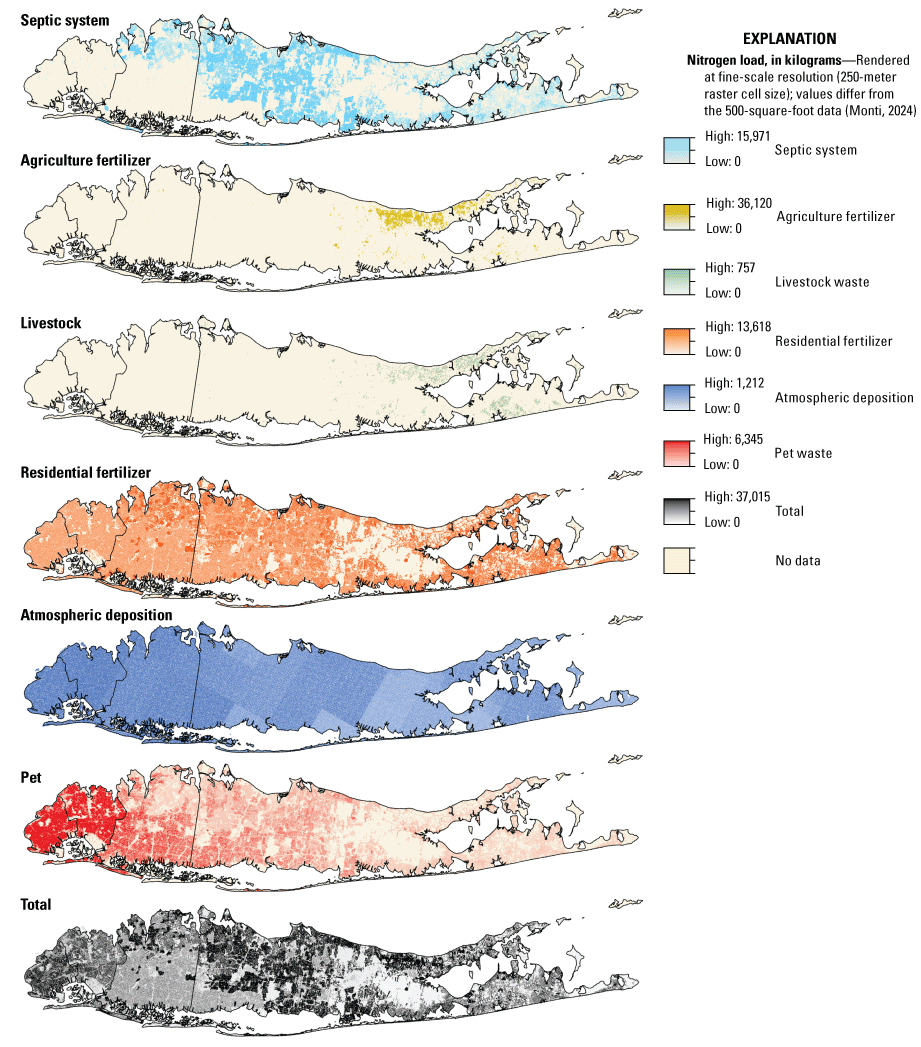
Nitrogen loads on Long Island, New York, in 2019, identifying individual component contributions to the total load.
Description of Datasets Used in the Analysis
The datasets were compiled and synthesized to provide a spatial and temporal distribution of nitrogen entering the subsurface from the six nonpoint sources. The spatial distribution of these sources is dependent on the land use and land cover category represented for each year. The annual loading of nitrogen was calculated by multiplying the annual application rates of nitrogen by the respective land areas over which application occurred or by multiplying the annual per capita nitrogen waste by the number of people, livestock, or pets in each specific area. A more detailed discussion of the functions used to calculate the mass for each nonpoint source is described in the “Estimating Nitrogen Loads” section of this report.
Land Use and Land Cover
Land use classifications designate the human activities or decisions determining how the land is used. Land cover describes the physical land type, such as developed (urban), forested, agricultural, or wetland areas. Because the data for land use and land cover (often found in tandem) were derived from multiple sources, the resolutions varied, and the land use categories changed over time (tables 1 and 2).
Table 2.
Reclassified values representing land use category data sources used for estimating nitrogen loading for Long Island, New York.[Not all crops listed were grown on Long Island, N.Y. The 1938–2005 land use and land cover data are from Sohl and others (2018a, b); the 2006 National Land Cover Dataset is from Fry and others (2011); the 2008–19 cropland data layer is from National Agricultural Statistics Service (2019)]
Land use and land cover data used in this analysis were obtained from several sources to enable analysis for the period from 1900 to 2019. The primary datasets (Sohl and others, 2014, 2016, 2018a, b) consisted of 13 land use categories available as annual raster grids for 1938–2005 at a 250-meter (m) resolution that were derived from modeled historical land use and land cover for the conterminous United. These datasets are thematically and spatially consistent with the land cover projections produced as part of the National Assessment of Ecosystem Carbon and Greenhouse Gas Fluxes project (Zhu and Reed, 2014).
Although several land use models are available for the years prior to 1938, the resolutions were too large to be appropriate for this analysis. These land use models are Steyaert and Knox (2008) at 10-km resolution, University of Illinois at Urbana-Champaign (2012) at 0.5-degree resolution, and Goldewijk and others (2007) at a 0.5-degree latitude and 1-degree longitude resolution. Therefore, the 1938 land use and land cover raster grid from Sohl and others (2016, 2018a) was used to represent land use conditions from 1900 to 1938 to maintain a consistent resolution throughout the 1900–2005 period. The use of the data from 1938 to represent all the prior years of the analysis does introduce a level of uncertainty in the estimates. Although the changes in land use categories prior to 1938 were primarily in Kings and Queens Counties, where populations increased during this period, more detailed spatial information about the extent of those changes was limited.
Two additional sources were used for land use and land cover categories from 2006 to 2019. The National Land Cover Database (NLCD) provided data for 2006 and 2007 (Fry and others, 2011). The NLCD uses a raster grid with a 30-m resolution with 15 land use and land cover categories for Long Island. The USDA cropland data layer was used for land use from 2008 to 2019 (NASS, 2019). The datasets were downloaded from the interactive CropScape mapping application.
The various land use and land cover categories from these sources were reclassified into five categories for the purposes of this analysis: developed, crop, pasture, undeveloped, and water (fig. 3; table 2). This reclassification simplified the land use and land cover categories in each raster grid and provided a means to consistently calculate the nitrogen load from year to year. The spatial distribution of the five categories and the shift from agricultural and undeveloped lands in 1938 to mostly developed lands in 2019 are illustrated in figures 3A and 3B respectively. Land use changes from 1900 to 2019 represent a transition from extensive agricultural use in 1900, when 40 percent of the land area was used for crops, to predominantly developed (residential) land use. Developed areas increased from less than 27 percent in 1938 to more than 68 percent of the total land mass in 2019.
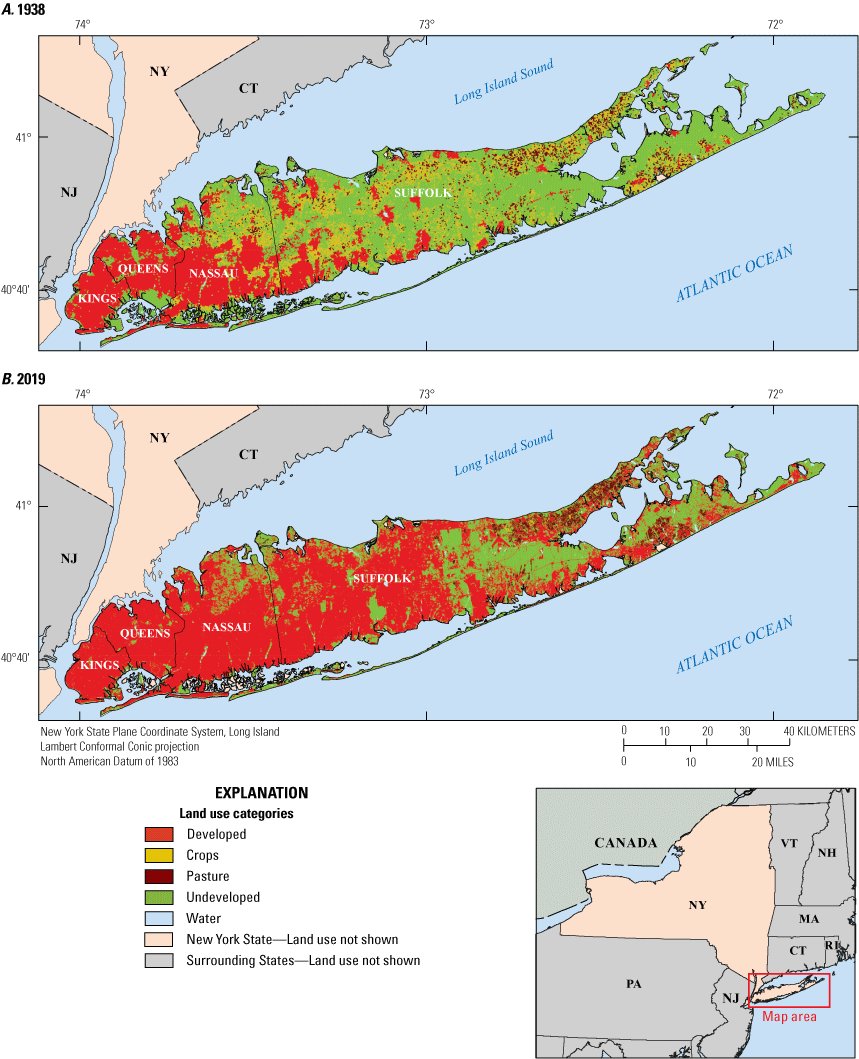
Map showing land use categories for Long Island, New York, representing conditions in A, 1938 and B, 2019. Land use categories are reclassified from the original source values, as shown in table 2.
Groundwater Flow Model Polygon Grid
A polygon grid was developed with 348 rows and 1,309 columns with each 500- by 500-ft cell representing a 250,000-ft2 area. This polygon grid was chosen because it is the same grid used in a regional groundwater flow model for Long Island described in Walter and others (2020). Although the flow model grid extends beyond the land area of Long Island and into the surrounding saline water bodies, nitrogen loads were considered to be zero in those areas. Conditions at the center point of each land cell polygon were used to estimate nitrogen loads for each 500- by 500-ft cell and nonpoint source from 1900 to 2019 (Monti, 2024).
Building Footprints
Building footprints were downloaded from the New York State Building Footprints website (State of New York, 2023). Although these polygons represent the outlines of all the structures in New York, only those for the four counties on Long Island were used in this study. The building footprints were used to distribute population and household estimates for the developed land use areas. Building footprints with an area less than 500 ft2, such as garages and sheds, were omitted to avoid assigning population values to those structures. This data source and others were used to estimate the septic and pet waste nitrogen loads.
Golf Courses
Golf courses were delineated from tax parcel boundaries or drawn from Streets and Imagery Hybrid maps (Esri, 2013, 2015). The golf course areas were applied in this analysis to calculate the nitrogen fertilizer use from golf courses. There were approximately 51 golf course polygon cells in Nassau, and 78 in Suffolk County that were potential sources of nitrogen over the course of the analysis period. The total golf course area in Nassau and Suffolk Counties was 75 km2, with 33 km2 in Nassau County and 42 km2 in Suffolk County, representing about 4 and 2 percent of the total land area in the two counties, respectively.
Impervious Surfaces
Estimates of impervious surfaces on Long Island were used to eliminate areas from the calculations of residential fertilizer application. Raster grids are available for 1974, 1982, 1992, 2002, and 2012 (Falcone, 2017) and provided the percent of impervious land cover for each raster cell. The mapping of these surfaces was derived by comparing anthropogenic impervious surfaces from the 2011 version of the NLCD (Fry and others, 2011) with the national land use dataset developed from an assessment of human-influenced land use trends for the conterminous United States in 2012 (Falcone, 2015). The raster grids provide an estimated imperviousness (humanmade surfaces as a percentage of the total land use) at a 60-m resolution. The impervious surfaces available for 1974, 1982, 1992, 2002, and 2012 were used to represent the imperviousness of the developed land use category for 1900–74, 1975–82, 1983–92, 1993–2002, and 2003–19, respectively. Using the 1974 impervious surface data for a period as long as 1900 to 1974 was not ideal; however, impervious surface information did not exist before 1974. It is possible, therefore, that the imperviousness during the earlier part of the study period has been overestimated and, in turn, residential fertilizer use, which was calculated from the developed land use data, has been underestimated.
Census of Population
The U.S. Census Bureau has enumerated the country’s population every decade since 1790. These data are available at different levels of geographical detail (U.S. Census Bureau, 2002); however, for this study, the town and city population data were useable because the administrative boundaries on Long Island did not change between 1900 and 2019. Town and city populations from 1790 to 1980 were inventoried by Shupe and others (1982) using data from the U.S. decennial census collections. This dataset, combined with the U.S. Census Bureau decennial collections for 1990, 2000, 2010, and 2020 (U.S. Census Bureau, 2021b), were merged into a single table of decennial populations at the town and city level for 1790 to 2020. The population estimates for the years in between the decennial collections were calculated by linear interpolation (fig. 4A).
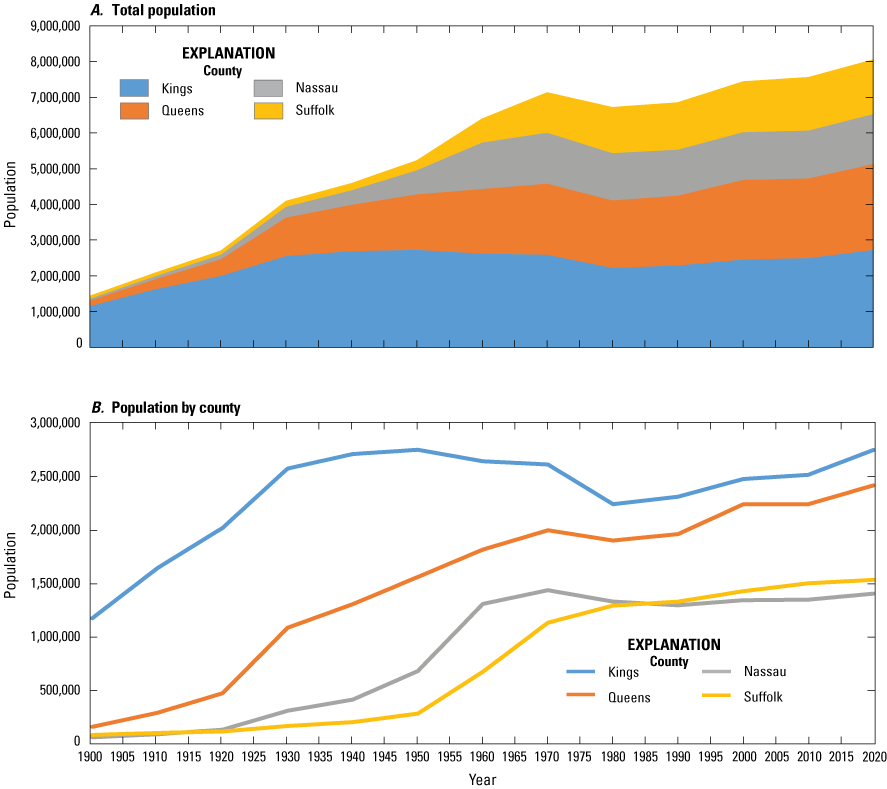
Graphs showing population on Long Island, New York, from 1900 to 2020 for A, the entire island, by county, and B, each individual county.
In 1900, more than 1 million people resided in Kings County. By 1930, Kings County had 2.5 million residents and Queens County exceeded 1 million residents (fig. 4B). Even as population densities were increasing in the westernmost parts of Long Island, population densities in Nassau and Suffolk County remained low until 1945, when suburban communities began to develop, and populations increased eastward (fig. 4B). The populations in Nassau and Suffolk Counties exceeded 1 million residents in 1955 and 1968, respectively (fig. 4B). By 2020, the estimated population of Long Island exceeded 8 million people (fig. 4A), representing a more than fivefold increase since 1900.
Census of Agriculture
Agricultural information, including farm acreage, livestock count, and crop type, is collected by the USDA as part of the census of agriculture (USDA, 2019) and is provided at both State and county levels. The census of agriculture originated as part of the 1820 decennial census, when agricultural pursuits within each household were added to the census questionnaire. A separate agricultural census began in 1840 on the same schedule as the decennial census of population until legislation in 1919 (The Act Providing for the Fourteenth Census, Public Law 65–325, 40 Stat. 1291) approved an additional mid-decennial census of agriculture taken in 1925 and every 10 years afterwards until 1950. Between 1954 and 1974, the U.S. Census Bureau conducted the census of agriculture in years ending in “4” and “9.” In 1997, the census of agriculture responsibility was transferred to the NASS, which began conducting this census in years ending in “2” and “7” (USDA, 2019).
Historical census of agriculture publications used in this study are available for 1900, 1910, 1920, 1925, 1930, 1935, 1940, 1945, 1950, 1954, 1959, 1964, 1974, 1978, 1982, 1987, 1992, 1997, 2002, 2007, 2012, and 2017 (NASS, 2020). The census of agriculture provides the best available data on total farm acreage, crop acreage, and livestock enumerations for each county on Long Island. These data were tabulated by county. Estimates for the years between census collections were calculated by using linear interpolation between collection dates to generate a table of estimated annual farm acreage (fig. 5A), crop acreage (fig. 5B), and livestock counts in each county. The 2017 census was the last census available at the time of this analysis, and those values were used for the years 2017–19.
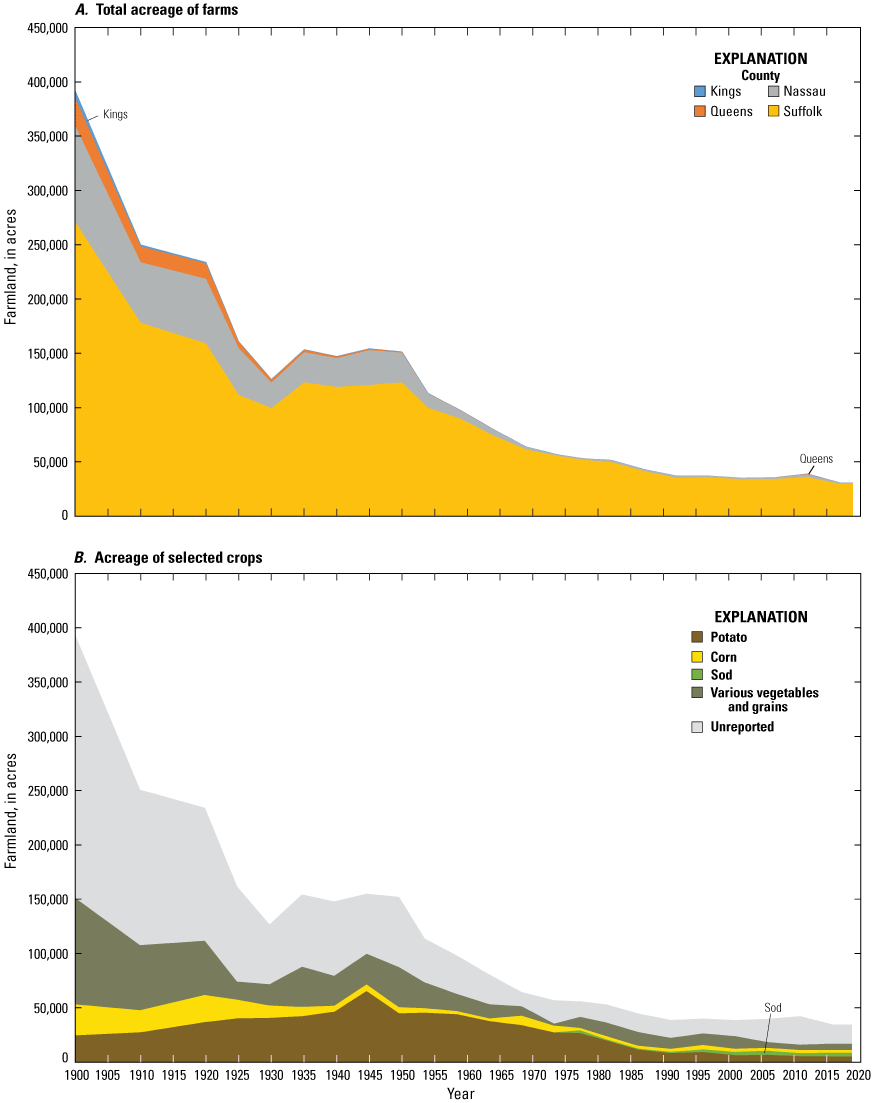
Graphs showing A, total acreage of farms, by county, and B, total acreage of selected crops categories on Long Island, New York, 1900–2019.
Farmed lands in the four counties exceeded 391,000 acres in 1900, or about 40 percent of the total land area on Long Island. Farm acreage decreased substantially from 1900 to 2019, when less than 3 percent of Long Island was used for agriculture. Most of this remaining agricultural land was on the peninsulas in eastern Suffolk County (fig. 3B).
Individual crop types used in this analysis included corn, potato, various vegetables, orchards, vineyards, sod, hay, and grains. The crop names were not consistent across census years. For instance, potatoes were sometimes called “potatoes” and sometimes “Irish potatoes.” These crop types with multiple names were grouped together to create consistency across census collections. When summing the acres of individual crop types, the total acreage of specified crops was less than the total acreage classified as farmed. This difference was noted, and the acreage was assigned as an unreported crop category in this analysis (fig. 5B).
Livestock enumerations from agricultural collections used in this analysis included horses, cattle, hogs, pigs, swine, sheep, goats, ducks, geese, chickens, and turkeys. To account for inconsistencies in how these counts were taken from census to census, this analysis grouped livestock by type, creating categories such as pigs and hogs, or goats and sheep.
Sewer System Areas
Human waste enters the environment through either an onsite septic system or a sewer system. Most areas begin with onsite septic systems and transition to a sewer system as the area develops. There are three principal types of sewer systems on Long Island—sanitary, storm, and combined sewers. Construction of sewer systems in New York City began in the mid-1800s (Veatch and others, 1906), and the New York State legislature established the Brooklyn Board of Sewer Commissioners in 1857. These early sewer systems were combined sewer systems that collected rainwater and human waste. Storm sewers primarily collect rainwater, but not human waste, and are not represented as a sewer system in this report. For this analysis, sanitary and combined system areas together are referred to as sewer system areas. It was assumed in this analysis that the entire populations in Kings and Queens Counties used sewer systems that discharge treated wastewater directly into the surrounding surface-water bodies for the whole study period.
For Nassau and Suffolk Counties, sewer data were obtained from the Long Island Index (2020), which provided maps of sewer districts. These maps were then georeferenced to create digital sewer system outlines for this analysis. By 2019, the cumulative area serviced by sewer systems was approximately 1,254 km2, or about 35 percent of the entire Long Island area (fig. 6). The year that an area switched from onsite septic systems to a centralized sewer system was estimated and assigned to each digitized outline by using previously published reports (Suter, 1937; Reilly and others, 1983; Monti and Scorca, 2003; Busciolano, 2005), descriptive data of municipal wastewater treatment plants (State of New York, 2020), and a timeline of estimated community connections (Peter E. Pyne, Jr., Nassau County Division of Environmental Health, written commun., 2017). A start year could not be determined for approximately 47 km2 of sewer system areas, in which cases a year was estimated from the “developed” reclassification code of the land use and land cover grid (table 2). The assigned start year for these areas was the year when 75 percent of the sewer system area overlapped the aggregate of the developed grid cell area (fig. 6).
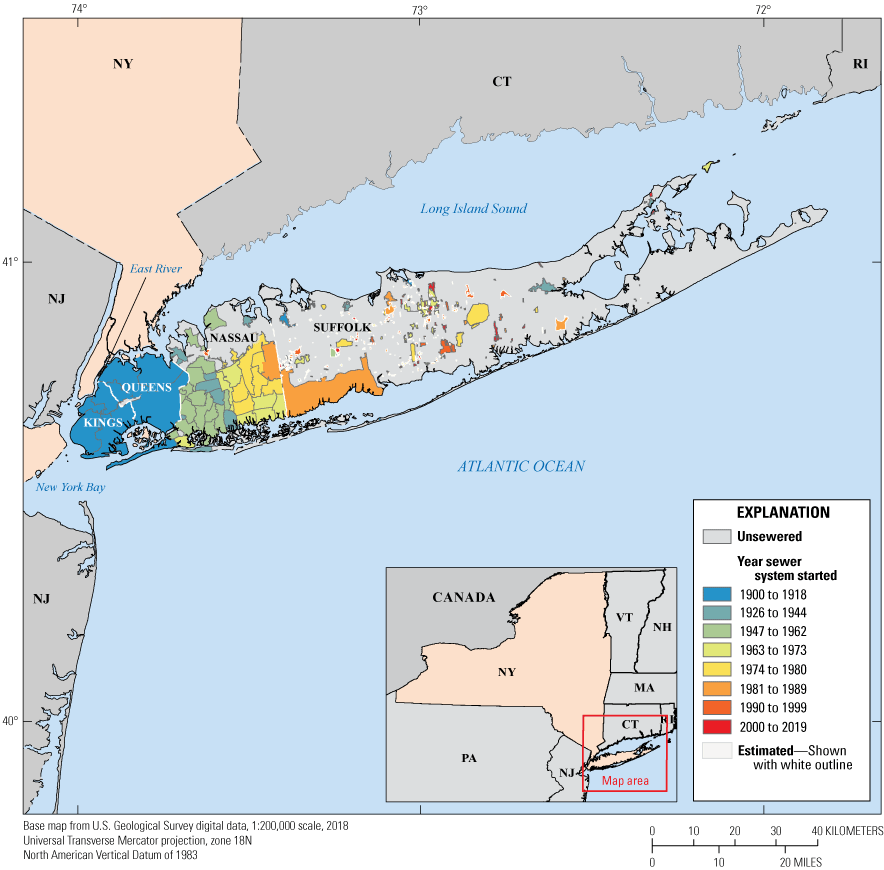
Map showing boundaries of sewer system areas and the years when the systems started for Long Island, New York.
Atmospheric Deposition of Nitrogen
Data sources for both wet and dry atmospheric annual deposition of nitrogen are available as raster grids for 1900 to 2019 from two sources. The first data source is the 1-km-resolution North American Climate Integration and Diagnostics (NACID) Nitrogen Deposition Version 1 database (Hember, 2018a, b). These raster grid units are in kilograms nitrogen per hectare per year and are available for each year from 1900 to 2013. The second data source is from the National Atmospheric Deposition Program (NADP) National Trends Network (Schwede and Lear, 2014; NADP, 2021). The NADP Total Deposition Science Committee developed total (wet plus dry) deposition raster grids at about a 4-km resolution, also in kilograms nitrogen per hectare per year, for each year from 2000 to 2019. Combining these two datasets into one required additional processing steps because neither dataset extent completely covered the land area of Long Island. These additional steps are discussed further in the “Estimating Nitrogen Loads” section.
Annual Nitrogen Loading
Annual nitrogen loading is the mass of nitrogen, in kilograms per head count of humans and livestock per year, per hectare per year for agriculture or residential fertilization, or per household per year for pets. Loadings used for this analysis are from previous publications, as described in the following subsections and summarized in table 3 and were held constant throughout the 120-year period, with the exception of atmospheric deposition and agricultural fertilization rates, which varied during the analysis period.
Human Waste
The estimated per capita annual nitrogen load from human waste applied in this analysis was 4.8 kg (Valiela and others, 1997). This value was derived from Watson and others (1967) and has a standard deviation of 2.3 kg. This value was used throughout the 120-year analysis period.
Table 3.
Nitrogen loading from nonpoint sources other than atmospheric on Long Island, New York, 1900–2019.[Some groupings of the type of animal and its nitrogen contributions were created to account for variations in the reported data. kg N, kilogram of nitrogen]
Source of nitrogen | Loading | Reference |
---|---|---|
Humans | 4.80 | Valiela and others, 1997 |
Residential | 100 | Lloyd and others, 2016 |
Agricultural | See fig. 7 and “Fertilizer Rates” section | |
Golf course | 146 | Lloyd and others, 2016 |
Cow1 | 89.8 | Boyer and others, 2002 |
Chicken2 | 0.17 | Boyer and others, 2002 |
Horse | 40.0 | Boyer and others, 2002 |
Pig and hog3 | 5.84 | Boyer and others, 2002 |
Sheep and goat4 | 5.00 | Boyer and others, 2002 |
Turkey | 0.39 | Boyer and others, 2002 |
Duck | 0.77 | American Society of Agricultural Engineers, 2003 |
Dog | 1.95 | CDM Smith, 2020 |
Cat | 1.46 | CDM Smith, 2020 |
Fertilizer
Koppelman (1978) estimated an annual rate of lawn fertilizer application of 49 kilograms nitrogen per hectare (kg N/ha) on Long Island. Valiela and others (1997) provided annual rates of fertilizer application to lawns and golf courses that range from 49 to 227 kg N/ha on Cape Cod. Lloyd and others (2016) applied an annual rate of 100 kg N/ha in a nitrogen land use model on the north shore of Long Island to represent the residential fertilizer application rate. In this report, annual rates of 100 and 146 kg N/ha were used to represent the residential fertilizer and golf course application rates, respectively, which represent values typical of those found in the literature.
Valiela and others (1997) estimated an annual agricultural fertilizer application rate used on different crop types of 136 kg N/ha which was derived by averaging a variety of crop types using information provided by Loehr (1974), Stanley (1988), Hayes and others (1990), and Correll and others (1992). This rate overestimates agricultural fertilization for the early part of the 20th century, when organic fertilizers predominated. Organic fertilizers, such as animal manure, generally have a lower nitrogen per ton than modern synthetic fertilizers (Ross and Mehring, 1938), which were not available in the early 1900s.
Synthetic inorganic fertilizers originated in the early part of the 20th century by using the Haber-Bosch chemical-processing method developed in 1909 at the University of Karlsruhe in Germany (Ross and Mehring, 1938). As more processing facilities became active and farmers realized the potential benefits, the use of this type of fertilizer predominated by the middle part of the 20th century (Mehring and others, 1957). The USDA (1966) reported the average nutrient content of fertilizer in the United States from 1850 to 1964, showing it increased substantially between 1930 and 1950. The fertilizer application rates used in this analysis reflect this transition in agricultural areas from organic to inorganic nitrogen and the corresponding increase in nitrogen content, which was estimated to occur in the early 1940s to mid-1950s.
A set of curves were constructed to represent a nitrogen application rate for agricultural areas that varies over time to reflect the change from an organic to an inorganic fertilizer with increasing nutrient content. The curves were structured by using four intervals that represent the estimated changes. The first interval, from 1900 to 1919, represents an organic source of fertilizer with an average increase of less than 0.6 percent in nitrogen per unit volume applied during these 20 years. The second interval, from 1920 to 1930, represents the period when the invention of the Haber-Bosch chemical-processing method to make synthetic fertilizer and its initial adoption provided an average increase of 1.8 percent of nitrogen per unit volume applied from fertilizer above the previous interval. During the third period, from 1931 to 1957, the rate of transition from organic to synthetic fertilizer accelerated as both the popularity of synthetic fertilizers and their content of nitrogen increased, providing an average increase of 3.6 percent of nitrogen per unit of fertilizer applied. The period from 1958 to 2019 represents a plateau in the nitrogen content of synthetic fertilizers as they increased less than 0.01 percent above the previous interval.
These varying application rate curves were applied to three demand categories representing crops with low, medium, or high nitrogen demands (fig. 7). A fourth category was computed as the average of the three demand categories. A current range of nitrogen needs for specific crops was determined from a series of guidelines published by Cornell University (Reiners and others, 2019). With the assistance of the regional Cornell Cooperative Extension specialist (Nora Catlin, Agriculture Program Director/Floriculture Specialist, written commun., 2021), the guidelines provided estimated ranges for the current nitrogen application rates for each crop type. The dashed lines in figure 7 represent the minimum and maximum guideline of the crops in each demand category. High-demand crop types include potatoes and sod; medium-demand crop types include sweet corn and other corn varieties; low-demand crop types include vegetables, grains, and vineyards. The curves were constructed such that they plateau within the minimum and maximum guidelines published between 1960 and 2019; the high and medium demand curves are closer to the minimum guideline rates, and the low demand curve is about midway between the minimum and maximum.
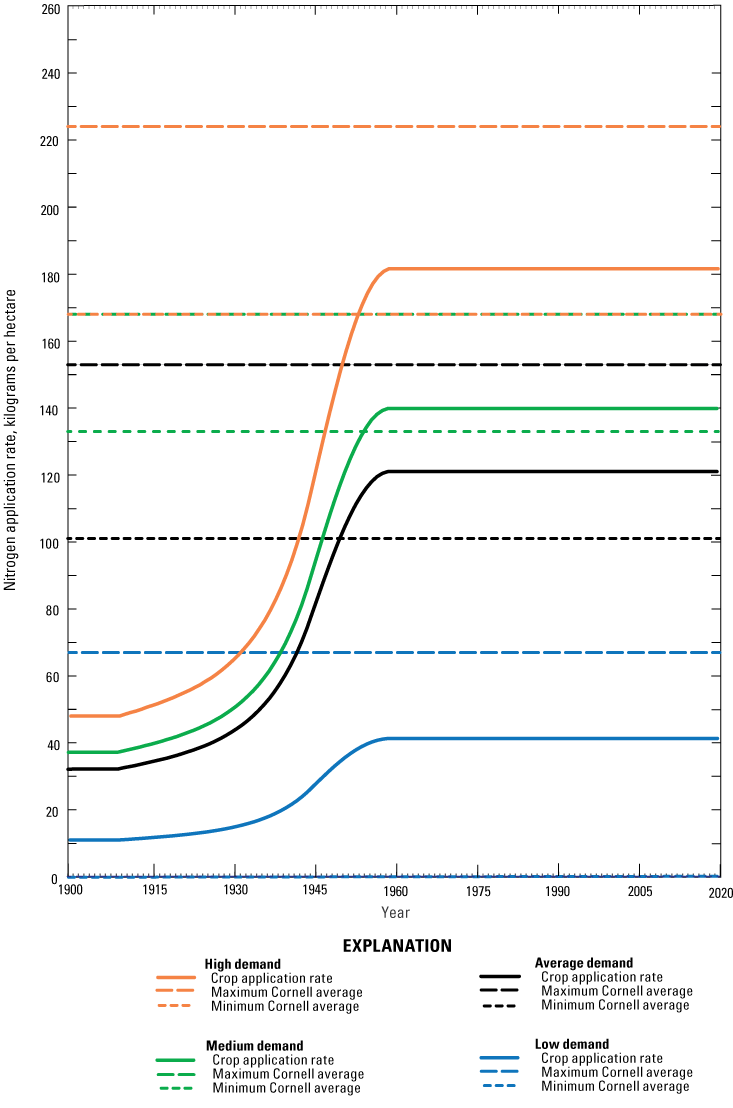
Graph showing agricultural curve application rates for high, medium, low, and average nitrogen demand categories. Cornell guidelines from February 5, 2019.
Livestock and Pet Waste
Annual nitrogen loading from livestock waste, in units of kg of N per animal, is available in Boyer and others (2002) for cows, chickens, horses, swine, sheep, goats, and turkeys, and from the American Society of Agricultural Engineers, (2003) for ducks (table 3). Annual nitrogen loading from pet waste, in units of kg of N per household, was estimated assuming dogs and cats made up the majority of domestic pets in the study area (table 3; CDM Smith, 2020).
Estimating Nitrogen Loads
The compiled datasets were used to calculate an annual nitrogen load for each nonpoint source from 1900 to 2019. The nitrogen estimates were determined for the center of each 500- by 500-ft cell of the regional groundwater flow model polygon grid (or “polygon grid”) for Long Island. The land use and land cover categories were the primary basis for assigning the loads from each anthropogenic source (all sources except atmospheric deposition). For instance, the reclassified land use category of developed was used to estimate the nitrogen loads from residential fertilizer use, whereas the crop category was used to estimate agricultural nitrogen loads. Overlaying county, town, or city boundaries onto the land use for each year allowed the calculation of total area per land use category per year. Although the cell size representing the land use raster changed from 250 m to 30 m in 2006, the aggregation of the area for each land use category was calculated from its respective land use cell size.
The region’s total aggregated land use area was used to distribute the estimated nitrogen load equally across the area. For example, if the tabular data were available by county, the nitrogen load for each land use category was distributed across the whole county. The various land use raster grid sources and their respective cells did not align with the Long Island polygon grid cells because the polygon grid was rotated for the purpose of simulating groundwater flow (Walter and others, 2020). An overlay analysis was used to distribute the nitrogen estimates from each raster grid onto the Long Island polygon grid by apportioning the load using the overlapping area within each 500- by 500-ft polygon grid cell.
The land use and land cover data for 1938 were used for the period from 1900 to 1937 because land use and land cover gridded raster data were not available for those years, at a 250 m resolution. As a result, the number of land use and land cover cells in each region remained constant during those years. Land use data after 1938 shows that most of the transition from agricultural to residential use in Nassau County occurred after 1945, suggesting that the assumption of using the land use data from 1938 for the period from 1900 to1937 was reasonable. The estimated per capita or per hectare loading (table 3) remained constant during this period, whereas agricultural fertilizer application rates, human and livestock population, the distribution of sewer system areas, number of households, atmospheric deposition, crop type, and acreage, and the number of land use and land cover cells in each of the five reclassified categories changed over time.
Septic System Nitrogen Source
Septic system nitrogen loading from 1900 to 2019 was calculated annually using a subset of building footprints that excluded garages and sheds (buildings less than 500 ft2). This dataset does not include the years buildings were built in Nassau and Suffolk Counties and therefore required correlating the building data with the developed land use category. The first year a building footprint overlapped with a developed category of the land use and land cover raster grid was the year assigned to that building footprint. In some instances, this method did not yield a result because the land use was never categorized as developed. In these cases, a year was assigned on the basis of proximity to other buildings to which a year had been assigned.
The differing resolutions of the raster grids used by the historical land use and land cover, NLCD, and cropland layers were transferred to the building footprints to do the overlay analysis. A second overlay using the centers of the building footprints and the polygon grid assigned a row and column from the nitrogen loading grid for each building. The two overlays result in the estimated year a building was constructed and a consistent 250,000-ft2 area from the grid cells, minimizing resolution differences in land use for developed areas.
The annual town or city population counts were used to distribute population among the building footprints in order to calculate an average population per building. Summarizing the building footprint row and column of each polygon grid resulted in a population per grid cell from 1900 to 2019. This combination of data—the number of buildings and the number of people within them for each grid cell—allowed for a consistent and detailed representation of the annual shifts in population densities in the developed areas, including residential, commercial, and industrial areas. This approach assumed that most of the town and city residents were near the commercial and industrial areas where they worked.
For each year of the study, 4.8 kg N/person (table 3) was multiplied by the aggregated population per developed polygon cell, which resulted in a human waste nitrogen load for each cell. This approach distributed the load equally within the census town, tract, or block geographic area. When a building on a developed land use and land cover cell was contained within an active sewer system extent area (fig. 6), the waste load of nitrogen for that cell was set to zero and only the developed cells outside the sewer system extent were used to estimate nitrogen from septic systems for that particular year.
Residential Fertilization Nitrogen Source
The mass of nitrogen from residential fertilization was calculated from the land use and land cover raster cells that were categorized as developed from 1938 to 2019. This calculation required converting the raster cell area to hectares because estimated residential fertilization application rates in Lloyd and others (2016) are reported as kilograms nitrogen per hectare per year. Multiplying the estimated fertilizer application rate (table 3) by the raster cell area provided the residential nitrogen load. This approach alone likely overestimated residential loading because each developed land use and land cover cell contained some impervious areas that were not fertilized and not every developed area or homeowner applies fertilizer. To adjust for this overestimation, the impervious surfaces within the developed raster cells were used to calculate a mean percent imperviousness for each developed land use and land cover category and the pervious surface percentage was then used to calculate the residential fertilization nitrogen load for only the pervious areas of the developed cells. Another adjustment was based on an estimated percentage of homeowners who use fertilizer. Giblin and Gaines (1990) estimated that the percentage of households on Cape Cod that used fertilizers was about 34 percent. In this analysis, we rounded the Giblin and Gaines (1990) estimate to 30 percent, assuming that household fertilizer may be used more frequently in some communities than in others, so 30 percent seemed a reasonable estimate for Long Island. Multiplying the residential fertilization nitrogen load for only the pervious areas by 0.30 resulted in the final residential fertilizer nitrogen load for each year analyzed.
A different approach was used to calculate the residential fertilizer nitrogen load prior to 1938 because the developed area category of the land use and land cover data was held constant during this period and therefore the residential load also remained constant, which was considered unrealistic. An average ratio of annual residential nitrogen load to septic system load of 0.53, computed based on the period 1938 to 2019, was multiplied by the human waste nitrogen load at each developed cell to compute annual residential fertilizer nitrogen load for the years prior to 1938.
Golf course areas were included in the residential fertilizer estimates. Each golf course was assigned a start and end year, and an annual application rate of 146 (kg N/ha)/yr was applied to the entire golf course area during the years it was active (table 3).
Agricultural Fertilization Nitrogen Source
The land use and land cover cells categorized as cropland were used to calculate the distribution and annual nitrogen load from agricultural fertilization. The most refined geographic level of data collected by the USDA is at the county scale; therefore, a countywide agricultural fertilizer nitrogen load was calculated from the USDA total farm and reported crop acreage (NASS, 2020), converted into hectares, and then multiplied by a variable application rate that represents the transition from organic to inorganic fertilizer and the nitrogen demand category by crop type (fig. 7).
The most commonly cultivated crop on Long Island was the potato. In 1945, potato farms covered more than 65,000 acres and represented about 42 percent of inventoried crops. In 1969, about 34,000 acres were used for potatoes, constituting the greatest proportion of all the inventoried crops at almost 53 percent of the total farmed acres, primarily in Suffolk County (figs. 5A and 5B). Corn farming used more than 28,000 acres in 1900, accounting for about 7 percent of the total farmed acres. In 1969, the acres of corn had fallen to just under 9,000 acres, but still accounted for about 13 percent of the total farmed acres. Other crops, including vegetables and grains, used about 98,000 acres in 1900, or 25 percent of farmed acres; however, by 2002, only about 12,000 acres were used, accounting for about 33 percent of all the farmed acres on Long Island. The total farmed acres recorded as individual crops was less than the total reported farm acreage in the agricultural census data. This difference was categorized as unreported crops and accounted for more than 240,000 acres in 1900, about 62 percent of the total farmed acres. In 2012, about 26,000 acres were unreported, which was about 66 percent of the total farmed acres (fig. 5B).
The USDA agricultural census collection reports of crop and farmed acreage were inventoried by Brakebill and Gronberg (2017) and, in this report, were reclassified into four categories according to the low, medium, high, or average nitrogen demand of the crop (fig. 7). The high-demand application rates were applied to the acres of potatoes and sod. The medium-demand application rates were applied to the acres of corn and the low-demand application rates were applied to all other reported crop acres. The unreported acres of crops received an application rate that is the average of the low, medium, and high rates.
The specific locations of crop cultivation could not be determined; therefore, for this analysis, the combination of crops and their nitrogen application rates were aggregated to yield a county load for each year. This county load was uniformly distributed across the crop land use and land cover cells within the county boundaries. This provided the annual load of nitrogen from agricultural fertilization at each crop land use and land cover cell. Loads at each land use and land cover raster cell were then overlaid onto the polygon grid to apportion the load to each polygon cell.
Livestock Waste Nitrogen Source
Livestock waste was also considered as a source of agricultural nitrogen in this analysis. The nitrogen load from livestock waste, particularly if animals grazed on pastureland or were fed from local food sources, may be overestimated in this analysis because the nitrogen in their feces would have been derived from the atmosphere or from fertilizers applied to these pastures (Valiela and others, 1997), not from external sources. Quantifying this potential overestimate of nitrogen loading from livestock waste would require a detailed time-varying, nitrogen-source analysis for the pastures throughout Long Island, for which data are unavailable. Instead, a nitrogen load from livestock waste was calculated by using a livestock type count (NASS, 2020) and corresponding nitrogen loading (table 3) which were summed at the county level. Except for waterfowl, annual livestock nitrogen loads were reduced by 50 percent to account for the assumption that half of the year their food is locally grown, and the nitrogen content of the feed is already accounted for in other source categories. The calculated waterfowl load was reduced by 75 percent as the waterfowl headcount is based on the number brought to market annually and not the number on farms at any point in time. Other potential overestimates of nitrogen loading from livestock are discussed in the “Uncertainties and Limitations” section.
Estimates of nitrogen load from livestock varied based on the loading of nitrogen per head for each type of animal (table 3). The land use and land cover grid cells categorized as pasture (table 2; fig. 3) were overlaid with county boundaries to determine the number of pastureland grid cells in each county, the assumption being that the livestock contributions should be applied only to pasture lands. The county livestock nitrogen load, not including waterfowl, was then distributed uniformly to the appropriate land use and land cover cells by dividing this county nitrogen load by the number of pasture-land grid cells in each county for each year of the study. Waterfowl loads from ducks and geese were similarly distributed equally but only to locations along the shoreline and streams, where duck farming was known to have occurred.
Pet Waste Nitrogen Source
Annual nitrogen loads from pet waste were calculated by using the same nitrogen loading of 1.95 kg N/household/yr and 1.46 kg N/household/yr (table 3) for dogs and cats, respectively, as reported in the Suffolk County subwatersheds wastewater plan (CDM Smith, 2020). In New York, in 2018, the average numbers of dogs and cats per household were 0.43 and 0.38, respectively (American Veterinary Medical Association, 2018); these numbers were assumed to be constant throughout the study period. The number of households per 500-ft polygon grid cell was determined from U.S. Census Bureau (2022) estimates of the national average number of people per household from 1960 to 2019 and the 1900–2019 population estimates per grid cell. The average number of people per household in 1960 was used to estimate the number of households from 1900 to 1959. Multiplying the pet waste nitrogen loading per household (table 3) by the number of households in each grid cell resulted in an annual pet waste nitrogen load from 1900 to 2019, for each grid cell.
Atmospheric Deposition Nitrogen Source
An annual atmospheric nitrogen deposition rate was applied to all land area grid cells, regardless of land use and land cover category. Deposition rates are from the North American Climate Integration and Diagnostics (NACID) Nitrogen Deposition Version 1 database (NDEP1) for the years 1900–2013 (Hember 2018a, b) and the NADP (2021) National Trends Network for the years 2000–19 (Schwede and Lear, 2014); both raster grids are reported in kilograms nitrogen per hectare per year. These data were multiplied by the cell area to generate the atmospheric deposition nitrogen loading at each cell. Extrapolations of the raster grids were necessary because atmospheric deposition data from the two sources did not completely overlap the land area across Long Island. The extrapolation process used a mean 3 by 3 focal statistic GIS tool that targeted grid cells with no defined data. A value was calculated by using the surrounding grid cells to produce raster grids that completely covered the land area of Long Island. For the years when the two data sources overlapped (2000–13), a ratio of NADP values to NACID–NDEP1 values was calculated and was used to adjust the NACID–NDEP1 data.
Calculating a ratio for the overlapping period 2000–13 was necessary because the NADP data are updated annually and Schwede and Lear (2014) included estimates for several nitrogen species that were not considered by Hember (2018a, b) in the NACID–NDEP1 database, thereby providing a more accurate representation of the atmospheric load. Using the extrapolated raster grids allowed for a total island-wide load to be calculated annually from 2000 to 2013 from both data sources. The ratio calculated for these 14 years was 0.58, indicating that, on average, the NACID–NDEP1 data for island-wide load estimates were 73 percent higher than the NADP data because of differences in the approaches used to create the raster grids. The datasets used different interpolation models, but the model used for NADP is the more current one. The ratio (0.58) was used to adjust the extrapolated 1900–99 NACID–NDEP1 raster grids and, together with the extrapolated 2000–19 NADP raster grids, provided a continuous annual representation of the atmospheric deposition nitrogen load from 1900 to 2019.
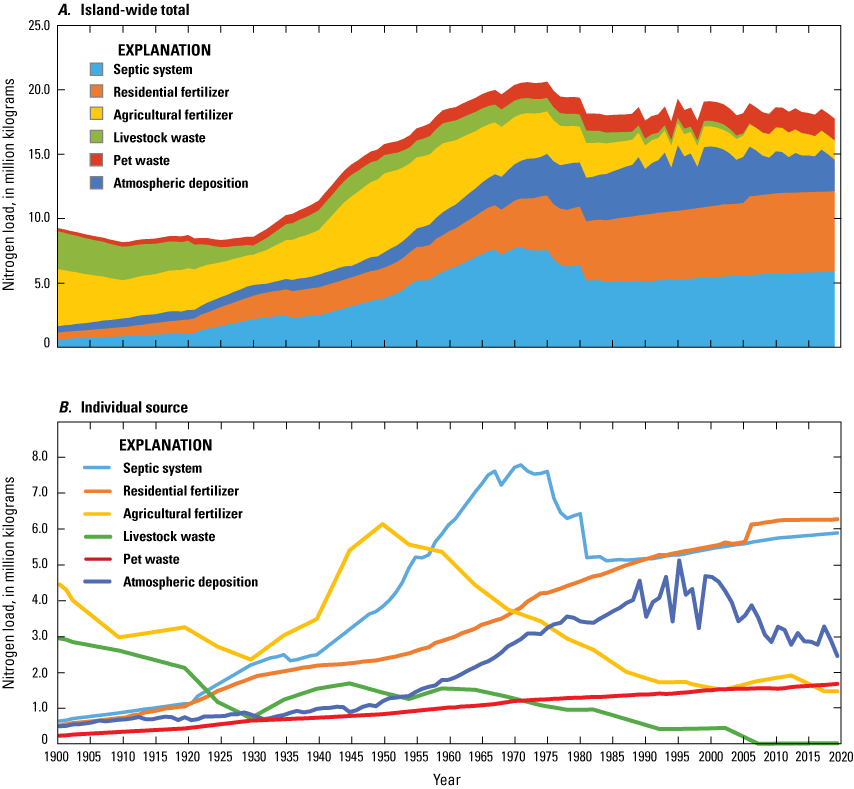
Graphs showing A, nitrogen loads from nonpoint sources as part of the total load for Long Island (stacked) and B, individual loads for each nonpoint source, on Long Island, New York, 1900–2019.
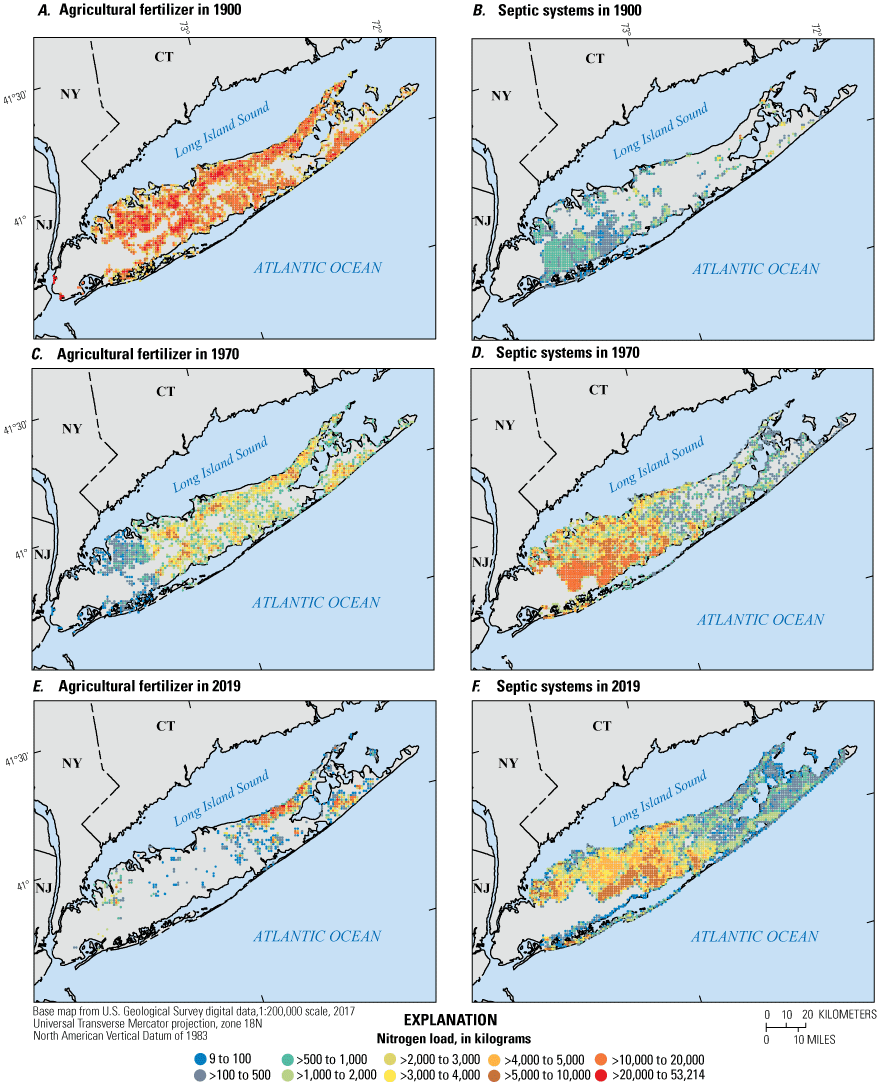
Maps showing nitrogen loads in 1900 from A, agricultural fertilizer and B, septic systems; in 1970 from C, agricultural fertilizer and D, septic systems; and in 2019 from E, agricultural fertilizer, and F, septic systems on Long Island, New York.
Trends in Nitrogen Load From Nonpoint Sources
Nitrogen loads presented in this report are unattenuated loads, or the loads that could potentially enter the aquifer system each year from 1900 to 2019 if the nitrogen mass at land surface was directly transported to the water table, without consideration of the various mechanisms of nitrogen loss, such as plant uptake, overland runoff, and chemical transformations in the soil and unsaturated zone. The annual loads generated by this approach for nitrogen load entering the 3,608-km2 land area of Long Island changed substantially from 1900 to 2019 (fig. 8). Spatial differences over time are evident in the nitrogen loads from agricultural fertilization and septic system sources as land use and land cover areas transitioned from agricultural or undeveloped into urban areas (figs. 3 and 9).
The annual countywide, nonpoint source, nitrogen loads from septic systems (fig. 10), residential fertilization (fig. 11), agricultural fertilization (fig. 12), livestock waste (fig. 13), pet waste (fig. 14) and atmospheric deposition (fig. 15) were divided by the area of the county, resulting in area-normalized loads in kilograms nitrogen per square kilometer that can be used to compare temporal changes for each county, as well as changes in annual nitrogen load by source type between counties. The annual nitrogen loads, and area-normalized nitrogen loads from 1900 to 2019 were used to calculate a long-term average nitrogen load (table 4) and an area-normalized nitrogen load (table 5) for each nonpoint source for both island and county areas.
Table 4.
Average annual nitrogen loads for Long Island, New York, 1900–2019.[Because of rounding, sums of the values may not equal the totals shown. Component values are in million kilograms of nitrogen. km2, square kilometer]
Table 5.
Area-normalized average annual nitrogen loads for Long Island, New York, 1900–2019.[Because of rounding, sums of the values may not equal the totals shown. Component values are in kilograms of nitrogen per square kilometer. km2, square kilometer]
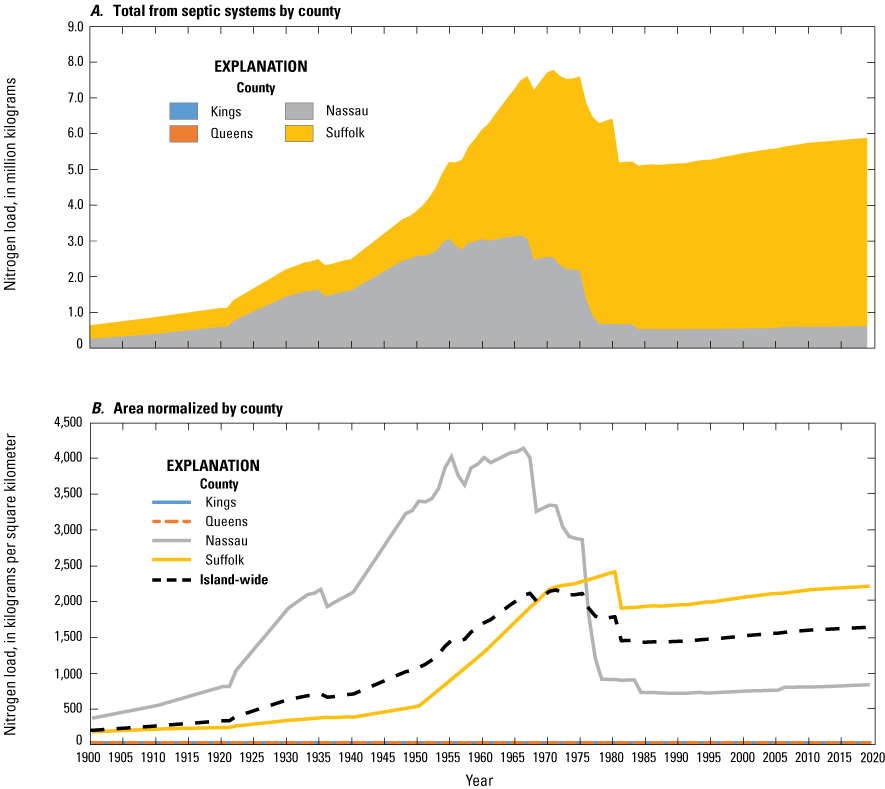
Graphs showing nonpoint, island-wide nitrogen loads from septic systems as A, totals from each county and B, area-normalized loads per square kilometer for each county on Long Island, New York, 1900–2019.
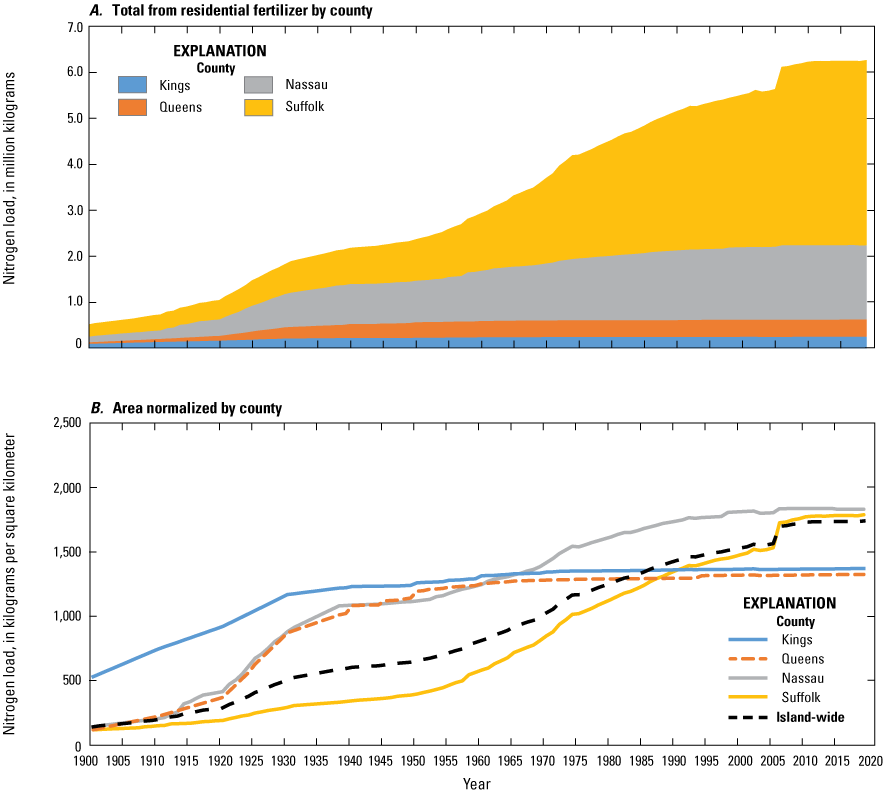
Graphs showing nonpoint, island-wide nitrogen loads from residential fertilizer as A, totals from each county and B, area-normalized loads per square kilometer for each county on Long Island, New York,1900–2019.
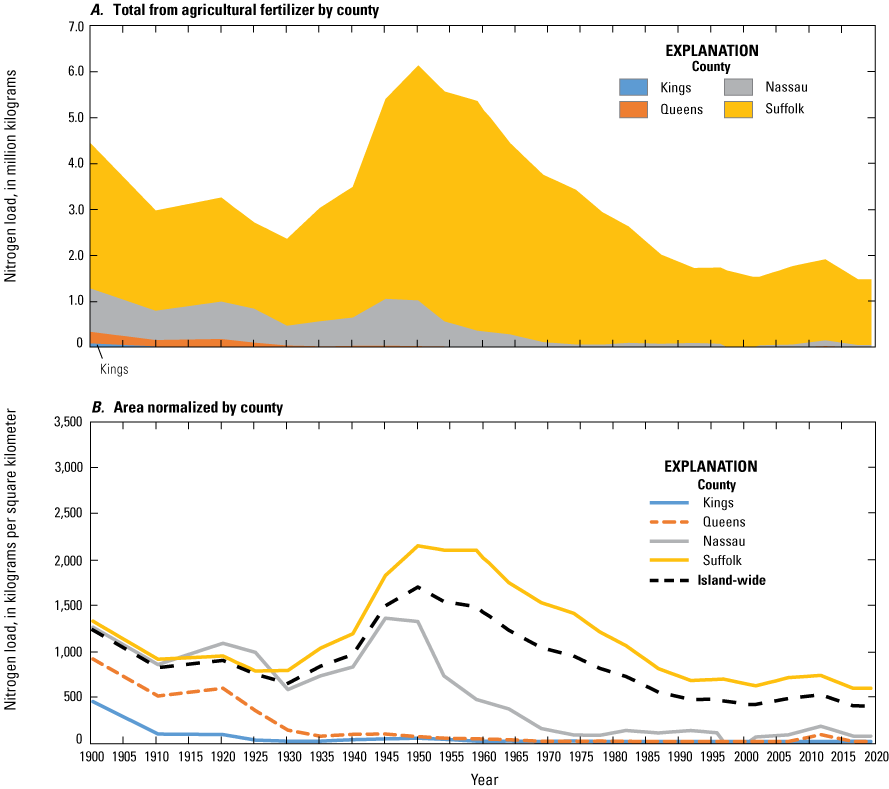
Graphs showing nonpoint, island-wide nitrogen loads from agricultural fertilizer as A, totals from each county and B, area-normalized loads per square kilometer for each county on Long Island, New York, 1900–2019.
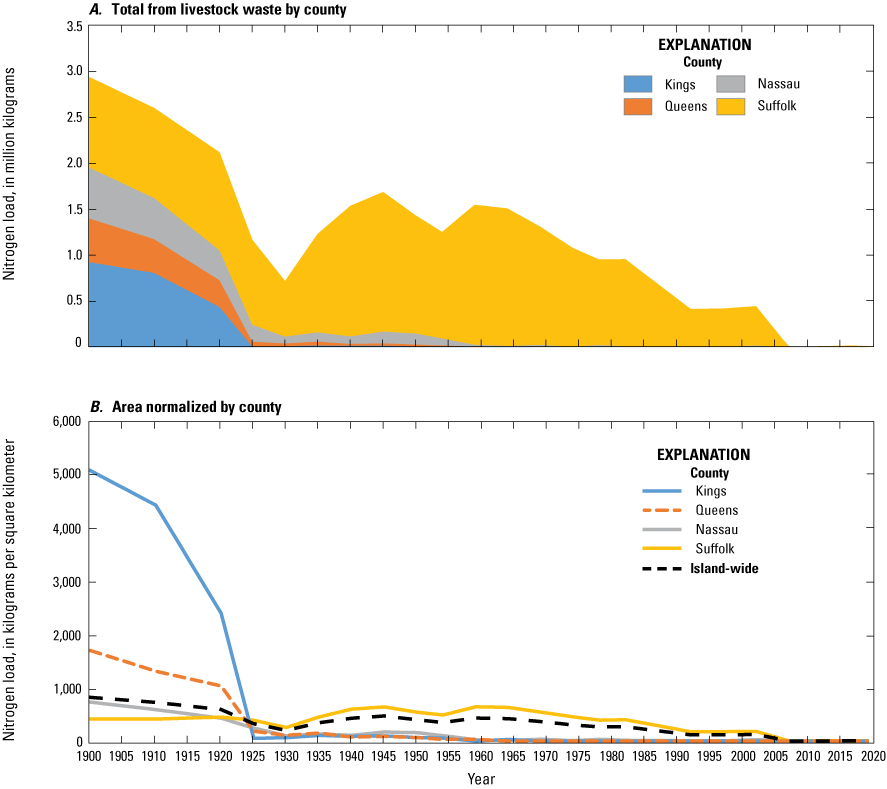
Graphs showing nonpoint, island-wide nitrogen loads from livestock waste as A, totals from each county and B, area-normalized loads per square kilometer for each county on Long Island, New York, 1900–2019.
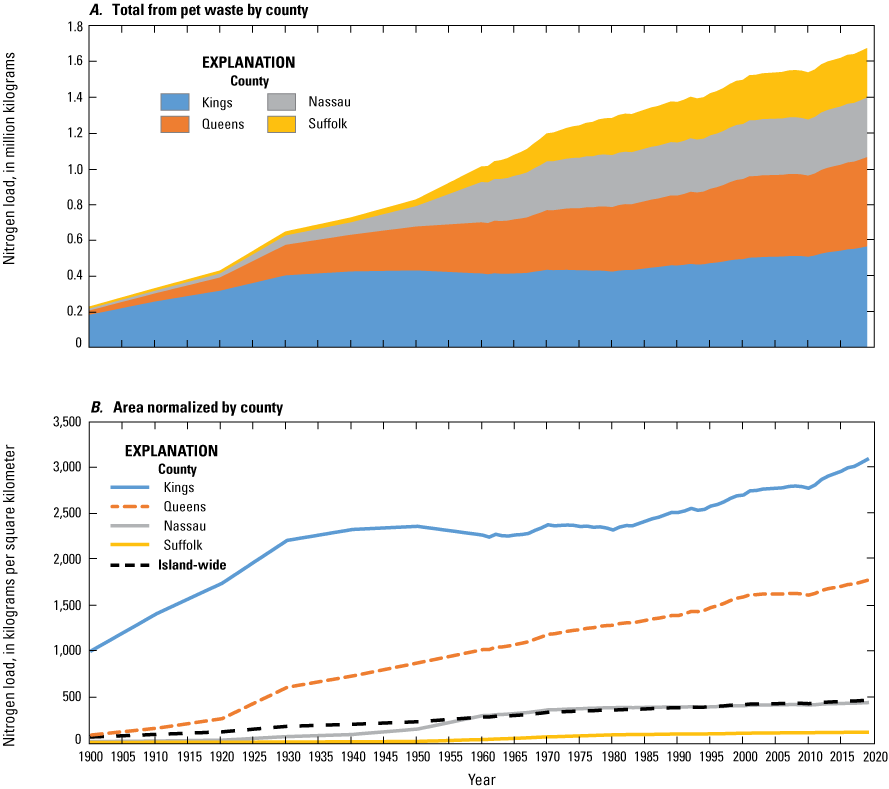
Graphs showing nonpoint, island-wide nitrogen loads from pet waste as A, totals from each county and B, area-normalized loads per square kilometer for each county on Long Island, New York, 1900–2019.
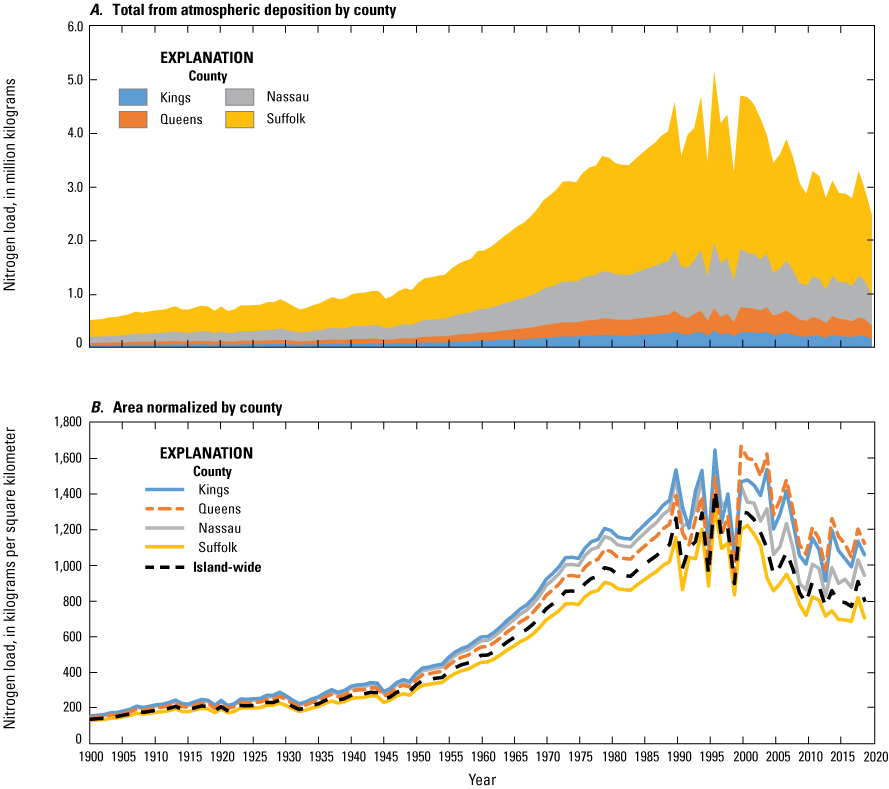
Graphs showing nonpoint, island-wide nitrogen loads from atmospheric deposition as A, totals from each county and B, area-normalized loads per square kilometer for each county on Long Island, New York, 1900–2019.
Nitrogen Loads, Island Wide
For the period 1900 to 2019, the average annual island-wide nitrogen load from the six nonpoint source components was about 14.92 million kilograms (Mkg N; table 4). This load is an aggregate of 4.15 Mkg N from septic systems, 3.28 Mkg N from residential fertilizer, 3.19 Mkg N from agricultural fertilizer, 1.22 Mkg N from livestock waste, 0.98 Mkg N from pet waste, and 2.10 Mkg N from atmospheric deposition (fig. 8A; table 4).
Area-normalizing the average annual island-wide nitrogen load for the 1900–2019 period results in an average annual nitrogen load of about 4,100 kilograms of nitrogen per square kilometer (kg N/km2; table 5). The average annual area-normalized nitrogen load for septic systems, residential fertilizer, agricultural fertilizer, livestock waste, pet waste, and atmospheric deposition are 1,100, 910, 880, 340, and 270, and 580 kg N/km2, respectively (table 5).
All six nonpoint source contributions (fig. 8B) varied over time (fig. 8A). The three largest island-wide average annual nitrogen loads are septic systems, residential fertilizer, and agricultural fertilizer, which contributed 28, 22, and 21 percent, respectively, of the island-wide total. The peak annual loads for these three sources were at different times because of land use changes between 1900 and 2019. Island-wide, agricultural fertilizer was the largest contributing source of nitrogen from 1900 to 1958, at which time septic systems became the largest contributing source. The load of nitrogen from agricultural fertilizer peaked in 1950 with 6.13 Mkg N, about 39 percent of the island-wide total for that year; Suffolk County accounted for 83 percent of this amount. The island-wide septic system nitrogen load peaked in 1971 at 7.78 Mkg N, or about 38 percent of the island-wide total for that year. About 67 percent of the total load from septic systems was in Suffolk County, and 33 percent was in Nassau County. In 2006, residential fertilizer surpassed septic systems as the largest nitrogen source, island wide. The conversion from agricultural, pastural, and undeveloped land into developed residential and industrial communities from 1900 to 2019 and large-scale sewer projects starting in the early 1970s are reflected in these changes in contributions from individual sources.
Agricultural Fertilizer and Livestock Waste Nitrogen Loads
More than 40 percent of the land on Long Island was reported as being used for agriculture in 1900, when more than 391,000 acres were farmed (NASS, 2020) (fig. 5A). The estimated load of nitrogen from agricultural fertilizer in 1900 was more than 4.44 Mkg N (table 6; fig. 12A), or about 48 percent of the nitrogen from all sources (fig. 8A). The second largest source of nitrogen in 1900 was from livestock at 2.94 Mkg N (table 6; fig. 13A), when the enumerated counts for horses and cattle were largest. The livestock load in 1900 accounted for 32 percent of the total load, with Kings, Queens, Nassau, and Suffolk Counties contributing 32, 16, 19, and 33 percent, respectively. This combination of agricultural fertilizer and livestock accounted for 80 percent of the nitrogen load in 1900. The remaining nonpoint sources—septic systems, residential fertilizer, pets, and atmospheric deposition—contributed only 7, 6, 2, and 5 percent, respectively, of the total nitrogen load of 9.26 Mkg N (table 6).
Table 6.
Nitrogen load and area-normalized nitrogen loads for Long Island, New York, 1900–2019.[Because of rounding, sums of the values may not equal the totals shown. Mkg N, million kilograms of nitrogen; kg N/km2, kilogram of nitrogen per square kilometer]
Agricultural nitrogen loads, which include both agricultural fertilizer and livestock waste, decreased over the analysis period, whereas loads associated with development (septic systems, residential fertilizer, pet waste, and atmospheric deposition) increased over time. The reason for these diverging trends was the population of the island increased and migrated eastward, resulting in agricultural land being converted to residential or commercial land during the latter half of the 20th century (fig. 8B).
Some of the variability in estimated nitrogen loads from livestock waste may be due in part to differences in USDA census collection techniques, the types of livestock counted, and how the numbers were used in this report (fig. 8B). Horses and cattle were the primary livestock influencing the nitrogen load from 1900 to 1925. However, duck farming along some coastal areas of Long Island increased throughout this period and well into the 1960s. Following a steady decline after 1900, livestock waste production increased between 1930 and 1945 (fig. 8B). This increase can be attributed to the emergence of duck farms in central and eastern Long Island, which had 5 million ducks in 1945 (USDA, 2019).
Septic System, Residential Fertilizer, and Pet Waste Nitrogen Loads
Nitrogen loads from septic systems, residential fertilization, and pet waste—all associated with residential development— gradually increased between 1900 and 2019 as a result of the increase in developed land and the corresponding increase in population. The developed areas of Long Island increased from 27 percent of the total island land area in 1900 to about 68 percent in 2019. The population of Long Island in 1900 was 1.4 million (with a majority of 1.1 million residing in Kings County) (fig. 4) and grew to more than 8 million people by 2020. Nitrogen load from septic systems in 1958 accounted for about 32 percent of total nonpoint sources analyzed. The island-wide estimate of nitrogen load from septic systems was highest in 1971 at about 7.78 Mkg N and then decreased (fig. 8B) as sewer systems became more available in densely populated areas (fig. 6). The eastward transition from onsite domestic septic to sewered areas resulted in several sharp decreases in nitrogen loads from this source over the following decade (fig. 8B).
Corresponding reductions in the residential fertilizer estimates were not observed because residential fertilization remained constant regardless of sewer availability. Nitrogen loads from residential fertilizer loads equaled septic system loads from 1987 to 2005 (fig. 8A) and then exceeded septic system loads after 2006. One reason for this apparent increase in loads in 2006 may be the use of a more detailed land use data source, which increased the resolution from 250 to 30 m (table 1).
Nitrogen load from pet waste increased since 1900 from 0.23 Mkg N to 1.68 Mkg N by 2019 (table 6). This steady increase is based solely on the rate of change in human population; the proportion of dogs and cats to human population was assumed to be unchanged from 1900 to 2019.
Atmospheric Deposition Nitrogen Load
Nitrogen loads from atmospheric deposition showed a steady increase after 1900 until they peaked in 1996 at 5.11 Mkg N (fig. 8B). Oscillations after 1990 are likely an artifact of the transition from the NACID to the NADP data source described in the Atmospheric Deposition Nitrogen Source section (fig. 8B). The Clean Air Act of 1970 (Public Law 91–604, 84 Stat. 1676) authorized the EPA to establish national ambient air quality standards to protect public health and welfare and to regulate emissions (EPA, 1996). The Clean Air Act Amendments of 1990 (Public Law 101–549, 104 Stat. 2399) resulted in the reduction of nitrogen load from atmospheric deposition after 1995.
By 2019, island-wide nitrogen load from all six nonpoint sources was about 18.2 Mkg (table 6); septic systems, residential fertilizer, agricultural fertilizer, livestock waste, pet waste, and atmospheric deposition contributed 32, 34, 8, less than 1, 9, and 16 percent of the island-wide total nitrogen, respectively.
Nitrogen Loads, by County
The temporal and spatial patterns of nitrogen loading from 1900 to 2019 varies by county and is a function of historical changes in land use and in changing human and animal populations within each county. The nitrogen loads at the island-wide scale were compared at the county scale to understand the specific changes that occurred within each county. The nitrogen loads from the nonpoint sources from each county were compared by normalizing the nitrogen load by the county land area. The county and area normalized loads were compared to the island-wide loads and graphed to provide a visual of the comparison.
Septic System Nitrogen Load
Nitrogen loads from septic systems in 1900 generally were limited to unsewered, developed areas in southwestern parts of Nassau County (fig. 9B) (fig. 6). By 1970, nitrogen loads from septic systems were widespread in most of Nassau County and western Suffolk County (fig. 9D) because of the urban and suburban sprawl that followed the end of World War II. By 2019, nitrogen loads from septic systems were widely distributed throughout central and eastern Suffolk County (figs. 6 and 9F) because extensive sewer systems did not exist, whereas nitrogen loads had decreased in Nassau County and western Suffolk County from their peak in the late 1960s because of extensive sewering (fig. 6). Area-normalized annual septic system nitrogen loads for each county were computed to provide a basis for comparison between each county from 1900 to 2019 (fig. 10B).
The nitrogen load from septic systems in Kings and Queens Counties was zero throughout the study period because active sewer systems have been in place since about 1850. The average annual nitrogen loads from septic systems in Nassau and Suffolk Counties were approximately 1.27 and 2.87 Mkg N, respectively (table 4). Septic system nitrogen load from Nassau County contributed more than 50 percent of the island-wide load from 1914 to 1960 (fig. 10A) and about 68 percent of the load in 1948. The maximum septic system nitrogen load and area-normalized load from Nassau County exceeded 3.16 Mkg N and 4,200 kg N/km2, respectively, in 1966 and then declined steadily before leveling off by 1985 (fig. 10B).
The septic system nitrogen load in Suffolk County accounted for more than 50 percent of the island-wide load from 1900 to 1913 and in 1961 to 2019. That percentage increased to more than 89 percent by 1978 and generally remained constant through 2019 (fig. 10A). The maximum nitrogen load and area-normalized load from septic systems in Suffolk County exceeded 5.74 Mkg N and 2,400 kg N/km2, respectively, in 1980.
The 1900 to 2019 area-normalized average annual septic system nitrogen loads in Nassau and Suffolk Counties are approximately 1,700 and 1,200 kg N/km2 (table 5), respectively. In 2019, the nitrogen loads from septic systems in Nassau and Suffolk Counties were 0.63 and 5.26 Mkg N, and the area-normalized nitrogen loads were about 820 and 2,200 kg N/km2 in Nassau and Suffolk Counties, respectively (table 6).
The island-wide nitrogen load from septic systems decreased by about 20 percent after 1980 (fig. 8A), which can be attributed to large-scale sewering in western Long Island. For example, in Nassau County, the continued expansion of sewer system capacities and areas of connections covered about 80 percent of the county area by 2019 (fig. 6), which resulted in substantial reductions in septic system nitrogen load (State of New York, 2020). The effects of sewer system expansion in parts of Nassau County were reflected as decreases in the septic system nitrogen load (fig. 10A); these effects were also evident in the island-wide septic system load (fig. 8B).
Residential Fertilizer Nitrogen Load
The estimated residential fertilization nitrogen load substantially increased as developed areas expanded between 1900 and 2019 (fig. 11). The average annual nitrogen loads from residential fertilizer in Kings, Queens, Nassau, and Suffolk Counties were approximately 0.22, 0.28, 0.91, and 1.86 Mkg N, respectively (table 4). The average annual area-normalized nitrogen load from residential fertilizer for both Kings and Nassau County was 1,200 kg N/km2, which was greater than the loads in Queens, and Suffolk Counties, which were 1,000, and 780 kg N/km2, respectively (table 5). In 1966, the Nassau County area-normalized residential fertilizer nitrogen load surpassed those of the other counties at about 1,300 kg N/km2 (fig. 11B), eventually leveling off at about 2,100 kg N/km2 around 2005 (fig. 11B). This pattern also occurred in Kings and Queens Counties after about 1960 and might represent a maximum developed threshold for each county. Although the average annual area-normalized nitrogen load from residential fertilizer is highest in Nassau County and lowest in Suffolk County, the nitrogen load in Suffolk County (fig. 11A) was about 61 percent of the island-wide load from 1985 to 2019 (fig. 11A).
The resolution of the land use and land cover data improved from 250 m in 2005 to 30 m in 2006. This improvement allowed for a more detailed representation of land use conditions after 2005 (table 1; fig. 3). The finer resolution resulted in a shift in land use category for some areas and an overall increase in the area categorized as developed land. This shift led to an apparent increase in the residential fertilizer load in 2006, mostly in Suffolk County, of about 460,000 kg N (figs. 11A and 8B). Nitrogen loads from residential fertilizer in Kings, Queens, Nassau, and Suffolk Counties in 2019 were 0.25, 0.37, 1.39, and 4.25 Mkg N, respectively, and the area-normalized nitrogen loads in Kings, Queens, Nassau, and Suffolk Counties were about 1,400, 1,300, 1,800 and 1,800 kg N/km2, respectively (table 6).
Agricultural Fertilizer Nitrogen Load
The nitrogen load from agricultural fertilizer in 1900 was distributed across all counties (fig. 9A); 2, 6, 21, and 71 percent of 4.44 Mkg N came from Kings, Queens, Nassau, and Suffolk Counties, respectively (table 6). Loads decreased after 1900 in Kings, and Queens Counties (fig. 12A). An overall decrease is also observed in Nassau and Suffolk Counties, however between 1943 and 1963 there was a notable increase that was most likely attributable to a period of intense potato farming (fig. 5B). By 1970, loads from agricultural fertilizer had decreased substantially in Nassau County and in central Suffolk County (figs. 9C and 12A) and, by 2019, were generally limited to eastern parts of Suffolk County (fig. 9E). In 2019, Nassau and Suffolk County agricultural fertilizer load estimates were 0.05 and 1.42 Mkg N, representing a combined 65 percent decrease from that of 1900 (table 6).
Agricultural fertilizer nitrogen load accounted for 48 percent of the island-wide load in 1900 and steadily decreased to about 8 percent by 2019 (fig. 8A). The average annual nitrogen loads from agricultural fertilizer in Kings, Queens, Nassau, and Suffolk Counties were approximately 0.01, 0.04, 0.40, and 2.74 Mkg N, respectively (table 4). The agricultural fertilizer nitrogen load for Long Island had an overall decrease from 4.44 Mkg N in 1900 to 1.47 Mkg N in 2019 (table 6, fig. 12A); however, the annual load peaked in 1950 at 6.13 Mkg N (about 39 percent of the total Long Island nitrogen load). The nitrogen load from agricultural fertilizer in Kings and Queens Counties decreased between 1900 and 1930; Kings County, the smallest and most urbanized county, contributed less than 1 percent (fig. 12A) and Queens County contributed 5 percent of the total agricultural fertilizer nitrogen load for Long Island during this period. Although there were farming activities in Kings and Queens Counties after 1930, their combined nitrogen contributions from agricultural fertilizer decreased to less than 1 percent of the island-wide total (fig. 12A). Nassau and Suffolk Counties contributed about 23 and 71 percent of the island-wide agricultural fertilizer nitrogen load from 1900 to 1930. In 1927, Nassau County nitrogen load from agricultural fertilizer was 27 percent of the island-wide agricultural fertilizer load, and this percentage steadily decreased to less than 5 percent after 1965. Nearly all farming activity on Long Island after 1930 was in Suffolk County, where farming contributed more than 80 percent of the total agricultural fertilizer nitrogen load on Long Island. By 2019, agricultural fertilizer nitrogen load estimates in Nassau and Suffolk Counties had dropped to about 0.05 and 1.42 Mkg N, respectively (table 6). Agricultural fertilizer nitrogen loads for those counties, normalized to county land area, were about 58 and 600 kg N/km2 (table 6, fig. 12B), respectively, which is substantially less than their respective average annual values.
The maximum agricultural fertilizer nitrogen load and area-normalized load from Kings and Queens Counties were in 1900, when these values were 0.08 Mkg N and 430 kg N/km2 for Kings County and 0.25 Mkg N and 900 kg N/km2 for Queens County (table 6). The maximum agricultural fertilizer nitrogen load and area-normalized nitrogen load in Nassau County peaked in 1945 at 1.02 Mkg N and 1,300 kg N/km2, respectively. The maximum agricultural fertilizer nitrogen load and area-normalized nitrogen load from Suffolk County exceeded 5.11 Mkg N and 2,100 kg N/km2, respectively, in 1950 (fig. 12B).
Livestock Waste Nitrogen Load
The island-wide average annual livestock waste nitrogen load between 1900 and 2019 was about 1.22 Mkg N (table 4), and the area-normalized load was 340 kg N/km2 (table 5). From 1900 to 1925, the agricultural census (NASS, 2020) reported horses and cows as the most abundant livestock. More than 126,000 horses were estimated to be on Long Island in 1900, with more than 89,000 in Kings County alone. The number of horses on Long Island decreased to about 3,200 horses in 1930, of which only 7 horses were reported in Kings County. The decrease in horses likely reflects a growing reliance on motor vehicles in the early 20th century. Between 1900 and 1925, the estimated nitrogen load from livestock waste within Kings County decreased from 0.93 Mkg to 0.09 Mkg (fig. 13A) mostly because of the declining number of horses in the county during that period.
The first census of duck populations in 1930 estimated that there were 1.1 million ducks cultivated on Long Island, mostly in Suffolk County (NASS, 2020). The census of agriculture enumerated duck populations until 1955, when 4.8 million ducks were counted. The nitrogen and area-normalized load from livestock waste in Suffolk County peaked in 1960 at about 1.52 Mkg N and 640 kg N/km2, owing primarily to duck farming (fig. 13A and 13B). The peak area-normalized nitrogen loads from livestock waste for Kings, Queens, and Nassau Counties were in 1900 at 5,000, 1,700, and 730 (kg N/km2), respectively (table 6, fig. 13B).
Pet Waste Nitrogen Load
Nitrogen load from pet waste gradually increased from 1900 to 2019. The average annual load for Long Island was 0.98 Mkg N (table 4), with Kings, Queens, Nassau, and Suffolk Counties contributing 0.41, 0.27, 0.18 and 0.12 Mkg N, respectively (table 4). The pet waste nitrogen load increased from 0.23 Mkg N in 1900 to 1.68 Mkg N by 2019 (table 6). This increase (fig. 14A; table 6) is a function of the increase in the human population, which was assumed to indicate a proportional increase in households and pets. Therefore, the area-normalized loads of 3,100, 1,800, 440, and 120 kg N/km2 in Kings, Queens, Nassau, and Suffolk Counties, respectively (table 6; fig. 14B), reflect population densities in each county, which peaked in 2019.
Atmospheric Deposition Nitrogen Load
Estimates of atmospheric deposition of nitrogen on Long Island varied over space and time from 1900 to 2019. The average annual nitrogen loads from atmospheric deposition were approximately 0.13, 0.19, 0.51, and 1.27 Mkg N (table 4) for Kings, Queens, Nassau, and Suffolk Counties, respectively, with corresponding average annual area-normalized nitrogen loads of 710, 670, 670, and 530 kg N/km2 (table 5).
The atmospheric deposition nitrogen load increased gradually until about 1940 and then more rapidly until around 2000. Changes in atmospheric deposition of nitrogen after 1940 were largest and most noticeable in the western counties, close to urban areas around New York City (fig. 15A). Nitrogen loads from atmospheric deposition in Kings, Queens, Nassau, and Suffolk Counties in 1900 were 0.03, 0.04, 0.12, and 0.31 Mkg N, respectively, and by 2019 were 0.19, 0.32, 0.72, and 1.67 Mkg N, respectively (table 6). In 1900, the area-normalized nitrogen loads from atmospheric deposition in Kings, Queens, Nassau, and Suffolk Counties were 160, 150, 150, and 130 kg N/km2 (table 6; fig. 15B), respectively. The large fluctuations between 1990 and 2019 are an artifact of the transition from the NACID to the NADP data (figs. 15A, B). By 2019, these area-normalized nitrogen loads from atmospheric deposition increased to 1,000, 1,100, 940, and 700 kg N/km2 (table 6; fig. 15B) for the same counties. The area-normalized nitrogen loads from atmospheric deposition in all counties were 2.3 to 3 times greater in 2019 than those in 1940 (fig. 15B). Although the westernmost counties had the highest area-normalized nitrogen loads from atmospheric deposition (fig. 15B), they only contributed about 40 percent of the island-wide nitrogen load from atmospheric deposition (fig. 15A), because Suffolk County constitutes 66 percent of Long Island.
Uncertainties and Limitations
The loads calculated in this report are unattenuated and do not represent the actual loads entering the water table and into the groundwater system. Loads from atmospheric deposition, agricultural fertilizer, and residential fertilizer are very likely overestimates of what enters the groundwater system, because of various processes including interception, runoff, and transformation or accumulation in soils.
There are several potential uncertainties and limitations in this analysis. The largest arise from the underlying spatial and tabular data, the methodology used to estimate the nitrogen loads, and the geoprocessing techniques used to analyze the data at various scales.
This analysis did not account for any interdependencies between nitrogen sources, and this limitation may affect the nitrogen input estimates from a given source. For instance, the livestock waste nitrogen source may include atmospheric deposition on pastures, and the agricultural nitrogen input also may include livestock waste as fertilizer. Data on the transfer of feed for livestock and agricultural fertilizer across county lines are not available; however, the livestock load could occur in a nongrowing season and was included in the analysis. Accounting for these complicated nitrogen source interdependencies was beyond the scope of this analysis, and it would require detailed, site-specific nitrogen-load budgets of pasture and agricultural lands to better evaluate the net nitrogen load for each of these interrelated sources.
The method used to estimate nitrogen loads from livestock may result in an incorrect estimate of that component of the load for the reasons previously discussed in the “Estimating Nitrogen Loads” section. However, as the proportion of nitrogen from livestock in 2019 was only about 0.1 percent of the total load and, between 1980 and 2019, averaged only 2.0 percent, this suggests that any error in the estimate from this source is small in comparison to recent (since 1980) nitrogen loads on Long Island (fig. 13A, table 6). Furthermore, the livestock contributions were calculated on the basis of data that were available in censuses; in cases where livestock existed but were not included in the census at that time, the livestock nitrogen load would likely be underestimated. Additionally, the enumerations of livestock were inconsistent across census collections, and some species, such as ducks, were not enumerated in the USDA census collection. It is possible that the methods or criteria for enumerating species of livestock were different for each census collection.
Agricultural fertilizer use varied over the analysis period, and many of the assumptions used in this report were structured to represent general changes in fertilizer sources. There are many variations in application rates for each crop, and the details of where, when, and how fertilizer was applied are poorly understood. Data gaps include the location of specific crops, the fertilizer methods used, how many times fertilizer was applied, and the specific grade of fertilizer (either organic or inorganic). The methods used to enumerate crop types changed between each census, and certain crop types were not always included or represented in all census collections. Crop types not represented in a census collection were assigned to the “unreported” crop category when the sum of the total acres of individual crops was less than the total farmed acres. When this occurred, an average application rate was applied to the unreported crop acreage. Because many of these details were not available, the estimates used in this report should be represented as regional estimates with a potential uncertainty larger during the earlier years of the analysis period.
The spatial data used, such as land use and land cover, are categorical and are useful for regional analysis; however, these data are generalized, and fine details, such as crop types, crop location, or populations within a developed area, are not represented. Land use between 1900 and 1938 was represented as static (and equivalent to 1938 conditions), which may introduce another level of uncertainty in nonpoint sources, except for the atmospheric deposition source, which is independent of land use. The spatial resolution of land use was represented in finer detail for 2006–19 than for 1900–2005 because of the increase in resolution in the 2006 NLCD from 250 m to 30 m (Fry and others, 2011). The finer land use representation introduced an offset in the number of developed land use cells between 2005 and 2006, which results in a corresponding change in residential fertilizer load estimates.
The methods for creating a continuous annual dataset of the census collections involved linear interpolation between each collection. This linear calculation—used for population, farm and individual crop acreage, and livestock amounts—represents the trends between each census collection and is a simplification of actual changes in population over time. An additional and likely more significant limitation is that transient residents, such as seasonal tourists, also contribute to nitrogen loads but were not included in the resident population enumerated in the census data. In certain townships, large amounts of nitrogen could be contributed by tourism, and in those areas, nitrogen estimates from septic systems could be undercounted.
Residential and golf course fertilizer estimates were each calculated by multiplying their respective areas by a constant application rate to compute an annual value. These rates can have a wide range of potential values and multiple variables; however, a simpler approach was used in this study so that scalar adjustments could be performed if more detailed data become available. As a result of this simplified approach, the residential and golf course fertilizer (fig. 11) primarily reflects general land use changes.
The temporal differences of the data used in the analysis were not always consistent, and a snapshot representation of 1 year was sometimes used for multiple years. For example, impervious surfaces were used to reduce the area of the residential fertilizer application. Because impervious surface information was not available before 1974, that year’s data were used to represent a large temporal extent within the analysis. It is likely that the imperviousness from 1900 to 1973 was considerably less than the imperviousness from the 1974 information, especially between 1900 and 1950; however, this difference is not represented.
Summary
Long-term estimates of nitrogen loading from nonpoint sources at or just below the land surface are essential for assessing the current and future effects of nitrogen on drinking water and on nitrogen loading to surface receiving waters. The annual load of nitrogen for Long Island, New York, for a 120-year period (1900–2019) was estimated by using a geographic information system to analyze, visualize, and process data sources, and format output data. The results from this analysis provided spatial and temporal estimates of nitrogen loading derived from six nonpoint sources (septic systems, residential fertilizer, agricultural fertilizer, livestock waste, pet waste, and atmospheric deposition). These load estimates represent the annual mass of nitrogen that could potentially enter the aquifer system; these estimates do not account for processes that may attenuate loading in the soil and unsaturated zones before entering the aquifer at the water table. The annual nitrogen load is gridded at a 500- by 500-foot resolution. Island-wide and countywide summaries of the annual nitrogen load and area-normalized load were analyzed to evaluate the average annual nitrogen load and area-normalized nitrogen load from the six nonpoint sources.
The island-wide average annual nitrogen load was estimated to be 14.92 million kilograms of nitrogen (Mkg N); of this, septic systems, residential fertilizer, agricultural fertilizer, livestock waste, pet waste, and atmospheric deposition accounted for 4.15, 3.28, 3.19, 1.22, 0.98, and 2.10 Mkg N, respectively. The island-wide average annual area-normalized nitrogen loads from septic systems, residential fertilizer, agricultural fertilizer, livestock waste, pet waste, and atmospheric deposition were about 1,100, 910, 880, 340, 270, and 580 kilograms of nitrogen per square kilometer (kg N/km2) with an island-wide, area-normalized nitrogen load of 4,100 kg N/km2.
The maximum septic system nitrogen load and area-normalized load in Nassau County exceeded 3.16 Mkg N and 4,200 kg N/km2, respectively, in 1966. In Suffolk County, the septic system nitrogen load peaked in 1980, exceeding 5.74 Mkg N, with an area-normalized load of about 2,400 kg N/km2. The nitrogen load from septic systems decreased as sewers became more available. By 2019, the nitrogen loads from septic systems in Nassau and Suffolk Counties were 0.63 and 5.26 Mkg N, respectively, and the area-normalized nitrogen loads in Nassau and Suffolk Counties were about 820 and 2,200 kg N/km2, respectively.
Suffolk County was the largest source of nitrogen from agricultural fertilizer, accounting for about 71 percent of the total agricultural nitrogen load from 1900 to 1930. After 1970, approximately 96 percent of all farming activity on Long Island was in Suffolk County. In 2019, the area-normalized nitrogen loads from agricultural fertilizer in Nassau and Suffolk Counties were about 58 and 600 kg N/km2, respectively, substantially less than the loads of 1,200 and 1,300 kg N/km2 estimated in 1900. The agricultural fertilizer nitrogen load for Long Island ranged from 4.44 Mkg N in 1900 to 1.47 Mkg N in 2019 and peaked in 1950 with an annual load of 6.13 Mkg N, which was 39 percent of the total Long Island nitrogen load for that year.
The spatial (land use) changes driven by human activities on Long Island are the foundation for the distribution of the nitrogen load from anthropogenic sources—septic systems, residential fertilization, agricultural fertilization, and livestock and pet waste. Land use changes reflect the transition from largely agricultural activity in 1900 (when agricultural fertilizer was the greatest source of nitrogen) to the start of increased development in about 1958. As the population and developed areas in Nassau and Suffolk Counties increased, the island-wide nitrogen load from septic systems increased to 7.78 Mkg N in 1971, or 38 percent of the island-wide load for that year. The nitrogen load from septic systems was greater than all other nonpoint sources from 1958 to 2006, when it was surpassed by the load from residential fertilizer. The land use transition reduced the agricultural area from 40 percent in 1900 to 3 percent of Long Island in 2019, whereas the developed areas increased from less than 27 percent in 1900 to about 68 percent in 2019. This land use change is noticeable in the decrease of the agricultural fertilizer load and increases in the septic system and residential fertilizer loads throughout the analysis period.
Atmospheric deposition of nitrogen varied geographically and temporally. The countywide, area-normalized nitrogen loads from atmospheric deposition were practically equal before 1940, after which the countywide load differences among counties increased. By 2019, the area-normalized nitrogen load from atmospheric deposition in each county was 2.3 to 3 times greater than in 1940. The increase in countywide load was largest in Kings County; the increase was not as large to the east, in Queens, Nassau, and Suffolk Counties. Although the westernmost counties had the highest area-normalized nitrogen loads from atmospheric deposition, they only received about 40 percent of the total island-wide atmospheric nitrogen deposition.
References Cited
Alexander, R.B., and Smith, R.A., 1990, County-level estimates of nitrogen and phosphorus fertilizer use in the United States, 1945 to 1985: U.S. Geological Survey Open-File Report 90–130, 12 p., accessed August 11, 2022, at https://doi.org/10.3133/ofr90130.
American Society of Agricultural Engineers, 2003, Manure production and characteristics: American Society of Agricultural Engineers Standard D384.1 FEB03, 4 p. [Also available at https://elibrary.asabe.org/abstract.asp?aid=32018.]
American Veterinary Medical Association, 2018, U.S. pet ownership statistics: American Veterinary Association web page, accessed April 22, 2022, at https://www.avma.org/resources-tools/reports-statistics/us-pet-ownership-statistics.
Bachman, L.J., Lindsey, B.D., Brakebill, J.W., and Powars, D.S., 1998, Ground-water discharge and base-flow nitrate loads of nontidal streams, and their relation to a hydrogeomorphic classification of the Chesapeake Bay watershed, middle Atlantic coast: U.S. Geological Survey Water-Resources Investigations Report 98–4059, 71 p. [Also available at https://doi.org/10.3133/wri984059.]
Bachman, L.J., and Phillips, P.J., 1996, Hydrologic landscapes on the Delmarva Peninsula part 2—Estimates of base-flow nitrogen load to Chesapeake Bay: Journal of the American Water Resources Association, v. 32, no. 4, p. 779–791. [Also available at https://doi.org/10.1111/j.1752-1688.1996.tb03475.x.]
Bleifuss, P.S., Hanson, G.N., and Schoonen, M.A.A., 2012, Tracing sources of nitrate in the Long Island aquifer system: State University of New York at Stony Brook web page, accessed June 23, 2020, at https://www.geo.sunysb.edu/reports/bleifuss/.
Boyer, E.W., Goodale, C.L., Jaworski, N.A., and Howarth, R.W., 2002, Anthropogenic nitrogen sources and relationships to riverine nitrogen export in the northeastern U.S.A.: Biogeochemistry, v. 57, no. 1, p. 137–169. [Also available at https://doi.org/10.1023/A:1015709302073.]
Brakebill, J.W., and Gronberg, J.M., 2017, County-level estimates of nitrogen and phosphorus from commercial fertilizer for the conterminous United States, 1987–2012: U.S. Geological Survey data release, accessed November 5, 2018, at https://doi.org/10.5066/F7H41PKX.
Busciolano, R.J., 2005, Statistical analysis of long-term hydrologic records for selection of drought-monitoring sites on Long Island, New York: U.S. Geological Survey Scientific Investigations Report 2004–5152, 47 p. [Also available at https://doi.org/10.3133/sir20045152.]
Cao, P., Lu, C., and Yu, Z., 2018, Historical nitrogen fertilizer use in agricultural ecosystems of the contiguous United States during 1850–2015—Application rate, timing, and fertilizer types: Earth System Science Data, v. 10, no. 2, p. 969–984, accessed February 8, 2022, at https://doi.org/10.5194/essd-10-969-2018.
CDM Smith, 2020, Suffolk County subwatersheds wastewater plan: Suffolk County Department of Health Services, prepared by CDM Smith, 842 p., accessed February 23, 2022, at https://suffolkcountyny.gov/Departments/Health-Services/Environmental-Quality#SubWWPlan.
Correll, D.L., Jordan, T.E., and Weller, D.E., 1992, Nutrient flux in a landscape—Effects of coastal land use and terrestrial community mosaic on nutrient transport to coastal waters: Estuaries, v. 15, no. 4, p. 431–442. [Also available at https://doi.org/10.2307/1352388.]
Eckhardt, D.A.V., Flipse, W.J., Jr., and Oaksford, E.T., 1989, Relation between land use and ground-water quality in the upper glacial aquifer in Nassau and Suffolk Counties, Long Island, New York: U.S. Geological Survey Water-Resources Investigations Report 86–4142, 35 p., 1 pl. in pocket. [Also available at https://doi.org/10.3133/wri864142.]
Eckhardt, D.A.V., and Stackelberg, P.E., 1995, Relation of ground-water quality to land use on Long Island, New York: Ground Water, v. 33, no. 6, p. 1019–1033. [Also available at https://doi.org/10.1111/j.1745-6584.1995.tb00047.x.]
Esri, 2013, Imagery hybrid: Esri map data, accessed June 24, 2020, at https://www.arcgis.com/home/item.html?id=86265e5a4bbb4187a59719cf134e0018.
Esri, 2015, Streets: Esri map data, accessed June 24, 2020, at https://esri.maps.arcgis.com/home/item.html?id=55ebf90799fa4a3fa57562700a68c405.
Falcone, J.A., 2015, U.S. conterminous wall-to-wall anthropogenic land use trends (NWALT), 1974–2012: U.S. Geological Survey Data Series 948, 33 p., plus appendixes 3–6 as separate files, accessed June 23, 2020, at https://doi.org/10.3133/ds948.
Falcone, J.A., 2017, Coefficient-based consistent mapping of imperviousness in the conterminous U.S. at 60-m resolution for 1974, 1982, 1992, 2002, and 2012: U.S. Geological Survey data release, accessed June 23, 2020, at https://doi.org/10.5066/F72F7KMG.
Falcone, J.A., 2021, Estimates of county-level nitrogen and phosphorus from fertilizer and manure from 1950 through 2017 in the conterminous United States: U.S. Geological Survey Open-File Report 2020–1153, 20 p., accessed September 14, 2018, at https://doi.org/10.3133/ofr20201153.
Fry, J.A., Xian, G.Z., Jin, S., Dewitz, J., Homer, C.G., Yang, L., Barnes, C.A., Herold, N.D., and Wickham, J.D., 2011, Completion of the 2006 national land cover database for the conterminous United States: Photogrammetric Engineering & Remote Sensing, v. 77, no. 9, p. 858–864, accessed May 2, 2020, at https://pubs.usgs.gov/publication/70034549.
Georgas, N., Rangarajan, S., Farley, K.J., and Jagupilla, S.C.K., 2009, AVGWLF‐based estimation of nonpoint source nitrogen loads generated within Long Island sound subwatersheds: Journal of the American Water Resources Association, v. 45, no. 3, p. 715–733. [Also available at https://doi.org/10.1111/j.1752-1688.2009.00318.x.]
Giblin, A.E., and Gaines, A.G., 1990, Nitrogen inputs to a marine embayment—The importance of groundwater: Biogeochemistry, v. 10, no. 3, p. 309–328. [Also available at https://doi.org/10.1007/BF00003150.]
Goldewijk, K.K., Hall, F.G., Collatz, G.J., Meeson, B.W., Los, S.O., Brown De Colstoun, E., and Landis, D.R., 2007. ISLSCP II historical land cover and land use, 1700–1990: Oak Ridge National Laboratory database, accessed February 14, 2022, at https://doi.org/10.3334/ORNLDAAC/967.
Gronberg, J.M., and Spahr, N.E., 2012, County-level estimates of nitrogen and phosphorus from commercial fertilizer for the conterminous United States, 1987–2006: U.S. Geological Survey Scientific Investigations Report 2012–5207, 20 p., accessed November 5, 2018, at https://doi.org/10.3133/sir20125207.
Hayes, S., Newland, L., Morgan, K., and Dean, K., 1990, Septic tank and agricultural non-point source pollution within a rural watershed: Toxicological and Environmental Chemistry, v. 26, nos. 1–4, p. 137–155. [Also available at https://doi.org/10.1080/02772249009357541.]
Hember, R.A., 2018a, North American Climate Integration and Diagnostics [NACID]—Nitrogen deposition 1 [NDEP1]: Mendeley Data database, accessed June 23, 2020, at https://doi.org/10.17632/ktmg7xtt4j.1.
Hember, R.A., 2018b, Spatially and temporally continuous estimates of annual total nitrogen deposition over North America, 1860–2013: Data in Brief, v. 17, p. 134–140, accessed May 2, 2020, at https://doi.org/10.1016/j.dib.2017.12.052.
Katz, B.G., Lindner, J.B., and Ragone, S.E., 1980, A comparison of nitrogen in shallow ground water from sewered and unsewered areas, Nassau County, New York, from 1952 through 1976: Ground Water, v. 18, no. 6, p. 607–616. [Also available at https://doi.org/10.1111/j.1745-6584.1980.tb03655.x.]
LeaMond, C.E., Haefner, R.J., Cauller, S.J., and Stackelberg, P.E., 1992, Ground-water quality in five areas of differing land use in Nassau and Suffolk Counties, Long Island, New York, 1987–88: U.S. Geological Survey Open-File Report 91–180, 67 p. [Also available at https://doi.org/10.3133/ofr91180.]
Lloyd, S., Mollod, G., LoBue, C., and Lindberg, M., 2016, Modeling nitrogen source loads on the north shore of Long Island: The Nature Conservancy, 32 p., accessed August 7, 2020, at https://www.conservationgateway.org/ConservationByGeography/NorthAmerica/UnitedStates/edc/Documents/TNC%20REPORT%20-%20Modeling%20nitrogen%20source%20 loads%20on%20the%20north%20shore%20of%20LI%2003-01-2016.pdf.
Loehr, R.C., 1974, Characteristics and comparative magnitude of non-point sources: Water Pollution Control Federation, v. 46, no. 8, p. 1849–1872, accessed June 2018 at https://www.jstor.org/stable/25038207.
Long Island Index, 2020, Long Island index map [sewer map outlines]: Long Island Index data, accessed June 1, 2017, at http://www.longislandindexmaps.org/.
Mehring, A.L., Adams, J.R., and Jacob, K.D., 1957, Statistics on fertilizers and liming materials: U.S. Department of Agriculture Statistical Bulletin No. 191, 182 p., accessed April 28, 2022, at https://usda.library.cornell.edu/concern/publications/k930bx03n.
Monti, J., Jr., 2024, Annual nitrogen load estimates from six nonpoint sources on Long Island, New York, from 1900 to 2019: U.S. Geological Survey data release, https://doi.org/10.5066/P9MC2LAJ.
Monti, J., Jr., and Scorca, M.P., 2003, Trends in nitrogen concentration and nitrogen loads entering the South Shore Estuary Reserve from streams and ground-water discharge in Nassau and Suffolk counties, Long Island, New York, 1952–97: U.S. Geological Survey Water-Resources Investigations Report 2002–4255, 36 p., [Also available at https://doi.org/10.3133/wri024255.]
National Agricultural Statistics Service [NASS], 2019, CropScape—Cropland data layer: U.S. Department of Agriculture, National Agricultural Statistics Service database, accessed June 30, 2020, at https://nassgeodata.gmu.edu/CropScape.
National Agricultural Statistics Service [NASS], 2020, Census of agriculture: U.S. Department of Agriculture web page, accessed June 23, 2020, at https://www.nass.usda.gov/AgCensus/.
National Atmospheric Deposition Program [NADP], 2021, Total deposition maps v. 2014.01: National Atmospheric Deposition Program data, accessed July 15, 2022, at https://nadp.slh.wisc.edu/committees/tdep/#tdep-maps.
Pearsall, K.A., 1996, Comparison of native ground-water quality with water quality in agricultural and residential areas of Long Island, New York: U.S. Geological Survey Open-File Report 95–401, 11 p. [Also available at https://doi.org/10.3133/ofr95401.]
Phillips, S.W., Focazio, M.J., and Bachman, J.L., 1999, Discharge, nitrate load, and residence time of ground water in the Chesapeake Bay watershed: U.S. Geological Survey Fact Sheet 150–99, 6 p. [Also available at https://doi.org/10.3133/fs15099.]
Ragone, S.E., Katz, B.G., Kimmel, G.E., and Lindner, J.B., 1980, Nitrogen in ground water and surface water from sewered and unsewered areas, Nassau County, Long Island, New York: U.S. Geological Survey Water-Resources Investigations Report 80–21, 64 p. [Also available at https://doi.org/10.3133/wri8021.]
Reilly, T.E., Buxton, H.T., Franke, O.L., and Wait, R.L., 1983, Geohydrology, modeling strategy, and regional evaluation, pt. 1 of Effects of sanitary sewers on ground-water levels and streams in Nassau and Suffolk Counties, New York: U.S. Geological Water-Resources Investigations Report 82–4045, 45 p. [Also available at https://doi.org/10.3133/wri824045.]
Reiners, S., Ketterings, Q.M., and Czymmek, K.J., 2019, Nutrient guidelines for vegetables: Cornell University Department of Animal Science Publication Series No. 249, accessed June 8, 2020, at http://nmsp.cals.cornell.edu/publications/files/VegetableGuidelines2019.pdf.
Ross, W.H., and Mehring, A.L., 1938, Soils & men—Yearbook of agriculture 1938: U.S. Department of Agriculture, p. 522–545, accessed April 28, 2022, at https://archive.org/details/yoa1938.
Schwede, D.B., and Lear, G.G., 2014, A novel hybrid approach for estimating total deposition in the United States: Atmospheric Environment, v. 92, p. 207–220, accessed May 5, 2020, at https://doi.org/10.1016/j.atmosenv.2014.04.008.
Scorca, M.P., and Monti, J., Jr., 2001, Estimates of nitrogen loads entering Long Island Sound from ground water and streams on Long Island, New York, 1985–96: U.S. Geological Survey Water-Resources Investigations Report 00–4196, 29 p. [Also available at https://doi.org/10.3133/wri004196.]
Sohl, T.L., Reker, R.R., Bouchard, M.A., Sayler, K.L., Dornbierer, J., Wika, S., Quenzer, R., and Friesz, A.M., 2016, Modeled historical land use and land cover for the conterminous United States: Journal of Land Use Science, v. 11, no. 4, p. 476–499, accessed June 22, 2020, at https://doi.org/10.1080/1747423X.2016.1147619.
Sohl, T.L., Reker, R., Bouchard, M., Sayler, K., Dornbierer, J., Wika, S., Quenzer, R., and Friesz, A., 2018a, Modeled historical land use and land cover for the conterminous United States—1938–1992: U.S. Geological Survey data release, accessed March 23, 2020, at https://doi.org/10.5066/F7KK99RR.
Sohl, T.L., Sayler, K.L., Bouchard, M.A., Reker, R.R., Friesz, A.M., Bennett, S.L., Sleeter, B.M., Sleeter, R.R., Wilson, T.S., Soulard, C., Knuppe, M., and Van Hofwegen, T., 2014, Spatially explicit modeling of 1992—2100 land cover and forest stand age for the conterminous United States: Ecological Applications, v. 24, no. 5, p. 1015–1036. [Also available at https://doi.org/10.1890/13-1245.1.]
Sohl, T.L., Sayler, K.L., Bouchard, M.A., Reker, R.R., Friesz, A.M., Bennett, S.L., Sleeter, B.M., Sleeter, R.R., Wilson, T., Soulard, C., Knuppe, M., and Van Hofwegen, T., 2018b, Conterminous United States land cover projections—1992 to 2100: U.S. Geological Survey data release, accessed March 23, 2020, at https://doi.org/10.5066/P95AK9HP.
Stackelberg, P.E., 1995, Relation between land use and quality of shallow, intermediate, and deep ground water in Nassau and Suffolk Counties, Long Island, New York: U.S. Geological Survey Water-Resources Investigations Report 94–4080, 82 p. [Also available at https://doi.org/10.3133/wri944080.]
Stanley, D.W., 1988, Historical trends in nutrient loading to the Neuse River estuary, North Carolina, in Lyke, W.L., and Hoban, T.J., eds., Proceedings of the AWRA symposium on coastal water resources, Wilmington, N.C., May 22–25, 1988: Bethesda, Md., American Water Resources Association, p. 155–164.
State of New York, 2020, Current descriptive data of municipal wastewater treatment plants: New York State Office of Information Technology Services data, accessed August 3, 2020, at https://data.ny.gov/Energy-Environment/Current-Descriptive-Data-of-Municipal-Wastewater-T/g5a2-qa6a.
State of New York, 2023, New York State Geographic Information Systems Clearinghouse—New York State building footprint county overview: New York State Office of Information Technology Services database, accessed January 30, 2023, at https://data.gis.ny.gov/datasets/sharegisny::nys-building-footprints/explore.
Steyaert, L.T., and Knox, R.G., 2008, Reconstructed historical land cover and biophysical parameters for studies of land-atmosphere interactions within the eastern United States: Journal of Geophysical Research, v. 113, no. D2, 27 p., accessed February 14, 2022, at https://doi.org/10.1029/2006JD008277.
University of Illinois at Urbana-Champaign (Department of Atmospheric Sciences), 2012, Historical land-cover change and land-use conversions global dataset: National Oceanic and Atmospheric Administration dataset, accessed February 14, 2022, at https://www.ncei.noaa.gov/access/metadata/landing-page/bin/iso?id=gov.noaa.ncdc:C00814.
U.S. Census Bureau, 2002, Measuring America—The decennial censuses from 1790 to 2000: U.S. Census Bureau report POL/02–MA(RV), 140 p, 4 app., accessed December 17, 2020, at https://www2.census.gov/library/publications/2002/dec/pol_02-ma.pdf.
U.S. Census Bureau, 2016, Population distribution and change—2010 [data tables]: U.S. Census Bureau data, accessed November 9, 2020, at https://www.census.gov/data/tables/time-series/dec/c2010br-01.html.
U.S. Census Bureau, 2021a, 2020 Census redistricting data summary file—2020 Census of population and housing: U.S. Census Bureau technical documentation, [variously paged; 201 p.], accessed October 1, 2021, at https://data.census.gov.
U.S. Census Bureau, 2021b, Decennial census of population and housing by decades: U.S. Census Bureau data, accessed October 4, 2022, at https://www.census.gov/programs-surveys/decennial-census/decade.html.
U.S. Census Bureau, 2022, Historical households tables—Table HH-4—Households by size: 1960 to present: U.S. Census Bureau dataset, accessed October 4, 2022, at https://www.census.gov/data/tables/time-series/demo/families/households.html.
U.S. Department of Agriculture [USDA], 2019, Census of agriculture historical archive: U.S. Department of Agriculture data, accessed June 23, 2020, at http://agcensus.mannlib.cornell.edu/AgCensus/homepage.do.
U.S. Environmental Protection Agency [EPA], 1996, National ambient air quality standards for nitrogen dioxide [final decision]: Federal Register, v. 61, no. 196, p. 52852–52856, accessed June 3, 2020, https://www3.epa.gov/ttn/naaqs/standards/nox/s_nox_pr.html.
Valiela, I., Collins, G., Kremer, J., Lajtha, K., Geist, M., Seely, B., Brawley, J., and Sham, C.H., 1997, Nitrogen loading from coastal watersheds to receiving estuaries—New method and application: Ecological Applications, v. 7, no. 2, p. 358–380, accessed May 16, 2018, at https://doi.org/10.1890/1051-0761(1997)007[0358:NLFCWT]2.0.CO;2.
Veatch, A.C., Slichter, C.S., Bowman, I., Crosby, W.O., and Horton, R.E., 1906, Underground water resources of Long Island, New York: U.S. Geological Survey Professional Paper 44, 394 p., 3 pl. [Also available at https://doi.org/10.3133/pp44.]
Walter, D.A., Masterson, J.P., Finkelstein, J.S., Monti, J., Jr., Misut, P.E., and Fienen, M.N., 2020, Simulation of groundwater flow in the regional aquifer system on Long Island, New York, for pumping and recharge conditions in 2005–15: U.S. Geological Survey Scientific Investigations Report 2020–5091, 75 p., accessed March 3, 2022, at https://doi.org/10.3133/sir20205091.
Watson, K.S., Farrell, R.P., and Anderson, J.S., 1967, The contribution from the individual home to the sewer system: Water Pollution Control Federation, v. 39, no. 12, p. 2039–2054. [Also available at https://www.jstor.org/stable/25035887.]
Zhu, Z., and Reed, B.C., eds., 2014, Baseline and projected future carbon storage and greenhouse-gas fluxes in ecosystems of the eastern United States: U.S. Geological Survey Professional Paper 1804, 204 p., accessed June 20, 2020, at https://doi.org/10.3133/pp1804.
Conversion Factors
Supplemental Information
Nitrogen loads are given in millions of kilograms (Mkg). Area-normalized loads are given in kilograms nitrogen per square kilometer (kg N/km2).
Nitrogen loading is given in kilograms per hectare per year, kilograms per person per year, kilograms per household per year, or kilograms per animal per year.
Concentrations of nitrogen are given in milligrams per liter (mg/L).
One square kilometer is equal to 100 hectares.
Abbreviations
EPA
U.S. Environmental Protection Agency
GIS
geographic information system
N
nitrogen
NACID
North American Climate Integration and Diagnostics
NADP
National Atmospheric Deposition Program
NASS
National Agricultural Statistics Service
NDEP1
Nitrogen Deposition Version 1
NLCD
National Land Cover Database
USDA
U.S. Department of Agriculture
USGS
U.S. Geological Survey
For more information, contact
Director, New York Water Science Center
U.S. Geological Survey
425 Jordan Road
Troy, NY 12180–8349
dc_ny@usgs.gov
or visit our website at
https://www.usgs.gov/centers/new-york-water-science-center
Publishing support provided by the Pembroke and Lafayette Publishing Service Centers
Disclaimers
Any use of trade, firm, or product names is for descriptive purposes only and does not imply endorsement by the U.S. Government.
Although this information product, for the most part, is in the public domain, it also may contain copyrighted materials as noted in the text. Permission to reproduce copyrighted items must be secured from the copyright owner.
Suggested Citation
Monti, J., Jr., Walter, D.A., and Jahn, K.L., 2024, Nitrogen load estimates from six nonpoint sources on Long Island, New York, from 1900 to 2019: U.S. Geological Survey Scientific Investigations Report 2024–5047, 40 p., https://doi.org/10.3133/sir20245047.
ISSN: 2328-0328 (online)
Study Area
Publication type | Report |
---|---|
Publication Subtype | USGS Numbered Series |
Title | Nitrogen load estimates from six nonpoint sources on Long Island, New York, from 1900 to 2019 |
Series title | Scientific Investigations Report |
Series number | 2024-5047 |
DOI | 10.3133/sir20245047 |
Year Published | 2024 |
Language | English |
Publisher | U.S. Geological Survey |
Publisher location | Reston, VA |
Contributing office(s) | New York Water Science Center |
Description | Report: vi, 40 p.; Data Release |
Country | United States |
State | New York |
Other Geospatial | Long Island |
Online Only (Y/N) | Y |
Additional Online Files (Y/N) | N |
Google Analytic Metrics | Metrics page |