External Quality-Assurance Project Report for the National Atmospheric Deposition Program National Trends Network and Mercury Deposition Network, 2021–22
Links
- Document: Report (2.14 MB pdf) , HTML , XML
- Data Releases:
- USGS data release - Data for the U.S. Geological Survey Precipitation Chemistry Quality Assurance Project for the National Atmospheric Deposition Program, 1978–2017
- USGS data release - U.S. Geological Survey Precipitation Chemistry Quality Assurance Project data 2017–2018
- USGS data release - U.S. Geological Survey Precipitation Chemistry Quality Assurance Project data 2019–2020
- USGS data release - U.S. Geological Survey Precipitation Chemistry Quality Assurance Project data 2021–2022
- Download citation as: RIS | Dublin Core
Acknowledgments
Assistance from the National Atmospheric Deposition Program office and laboratory staff was crucial and greatly appreciated. Work was completed by Alexander Liethen under contract to the U.S. Geological Survey.
Abstract
The U.S. Geological Survey Precipitation Chemistry Quality Assurance project (PCQA) operated five distinct programs to provide external quality-assurance monitoring for the National Atmospheric Deposition Program (NADP) National Trends Network (NTN) and Mercury Deposition Network (MDN) during 2021–22. The NTN programs included (1) a field audit program to evaluate sample contamination and stability, (2) an interlaboratory-comparison program to evaluate analytical laboratory performance, and (3) a colocated sampler program to estimate overall variability of NTN wet-deposition measurements, and the MDN programs included the (4) system blank program to evaluate sample contamination and stability and (5) an interlaboratory-comparison program. The results indicated increased levels of sample contamination compared to previous years for NTN samples and decreased contamination in MDN samples. Strong analytical laboratory performance with low overall variability and bias in concentration data was indicated for the NTN Central Analytical Laboratory. Slight perturbations in contamination levels in NTN samples and in analytical performance for MDN are considered inconsequential. The colocated sampler program results indicated overall variability in NTN data to range from 0 to 30.3 percent for cations, 1.6 to 11.4 percent for ammonium, 0.8 to 20.2 percent for anions, 10.3 to 17.2 percent for hydrogen-ion concentration, and 1.5 to 12.2 percent for specific conductance. The PCQA results indicate that NADP data continue to be of sufficient quality for applications in independent research and NADP data products, including spatial interpolations and time trends for chemical constituents in wet deposition. Small shifts in data quality indicated by the 2021–22 PCQA results are included to be used for interpretation of the NADP data products.
Introduction
The U.S. Geological Survey (USGS) Precipitation Chemistry Quality Assurance project (PCQA; https://nadp.slh.wisc.edu/quality-assurance/) ensures that the National Atmospheric Deposition Program (NADP) provides data users with long-term (since 1978), known-quality atmospheric wet-deposition information to evaluate the ecological and health effects of air contaminants that are deposited to the Earth’s surface. Interactive site location maps for both networks are available on the NADP website, the Mercury Deposition Network (MDN) network map is available at nadp.slh.wisc.edu/maps-data/mdn-interactive-map/, and the National Trends Network (NTN) map is available at nadp.slh.wisc.edu/maps-data/ntn-interactive-map/ (NADP, 2023a,c). During the study period, there were 260 NTN sites in 2021 and 259 sites in 2022, which is similar to what has been reported in the past several years (Zac Najacht, Wisconsin State Laboratory of Hygiene [WSLH], written commun., 2023). The number of active MDN sites in 2021–22 remained steady at 80 sites. The PCQA project is administered on behalf of more than 100 Federal, State, Tribal, and other agencies and stakeholders that participate in the NADP by the USGS Observing Systems Division, Hydrologic Networks Branch (Denver, Colorado), and the USGS New York Water Science Center (Troy, New York). Results of the PCQA project allow investigators to account for inherent variability and bias in NADP data that are potentially introduced during the collection, processing, and laboratory analysis of samples. Results of the PCQA project allow investigators to identify and quantify true environmental signals. Reporting of the 2021–22 results in this report follows the general template for objectives, methods and wording established in Wetherbee and others (2023).
Purpose and Scope
The NADP incorporated two wet-deposition monitoring networks in 2021–22: (1) the NTN and (2) the MDN. This report updates the independent assessment of NADP data quality using PCQA results obtained for calendar years 2021–22 (study period) for the NTN and MDN. Results from previous years are used for comparison.
The field audit program and the system blank program assessed the effects of onsite exposure, sample handling, and shipping on the chemistry of NTN and MDN samples, respectively. Two interlaboratory-comparison programs assessed the bias and variability of chemical analysis data from the Central Analytical Laboratory (CAL) and the Mercury (Hg) Analytical Laboratory (HAL), both of which are at the WSLH at the University of Wisconsin-Madison. The CAL moved from the Illinois State Water Survey, Prairie Research Institute (PRI), to the WSLH during 2017. Shortly thereafter, the HAL moved from its former location at Eurofins Frontier Global Sciences, Inc. (Bothell, Washington), to the WSLH during 2018. The NTN colocated program resumed in 2021, with two sites participating in both years. The colocated sites for 2021 were OK00 and 00OK and TX56 and 56TX, and the 2022 study sites were NE99 and 99NE and OH09 and 09OH. Detailed information on USGS quality-assurance procedures and analytical methods for the NTN and MDN are available in Latysh and Wetherbee (2005, 2007) and Wetherbee and Martin (2017). Data used to support the conclusions presented in this report are publicly available through three USGS data releases (Wetherbee, 2020a, b, 2022).
Statistical Methods
In this report, nonparametric, rank-based statistical methods are used in place of traditional statistical analyses and hypothesis testing. The sign test (Kanji, 2006) was used to evaluate if the median of differences between two groups is significantly different from zero. Network maximum contamination levels (NMCLs) were evaluated at the 90-percent significance level (α [maximum probability of incorrect rejection of the null hypothesis] =0.10). Other statistical tests were evaluated at the 95-percent significance level (α=0.05), unless otherwise noted. Statistical analyses were completed using R, version 4.2.1 (R Core Team, 2022).
Bias was quantified on the basis of relative and absolute differences and percentage differences (Wetherbee and others, 2023). These parameters are calculated for each program as follows:
whereCn
is the sample concentration, in milligrams per liter, microequivalents per liter, or nanograms per liter, for the test sample or precipitation depth in centimeters;
Cc
is the sample concentration, in milligrams per liter, microequivalents per liter, or nanograms per liter, for the control sample or precipitation depth in centimeters; and
Ct
is either Cc (field audit and system blank programs), a most probable target value (interlaboratory-comparison programs), or the mean of Cn and Cc for replicate measurements using identical precipitation gages (colocated sampler program).
Variability was quantified in this report using f-pseudosigma (f-psig), a nonparametric analog of the standard deviation of a statistical sample (Hoaglin and others, 1983):
whereThe f-psig ratio was also used to compare the variability of an entire dataset with the variability of a subset:
wheref-psigsubset
is the f-pseudosigma of the subset and
f-psigo
is the overall f-pseudosigma of the entire dataset.
The mass of mercury contamination in each system blank sample was calculated as follows:
where[HgSB]
is the total mercury concentration in system blank sample, in nanograms per liter;
VolumeSB
is the volume of system blank sample, in liters;
[HgBot]
is the total mercury concentration in the bottle sample, in nanograms per liter; and
VolumeBot
is the volume of the bottle sample, in liters.
Maximum contamination levels and precipitation-sample stability were determined by a calculation of upper confidence limits (UCLs) on percentiles of concentration data using a bootstrap method in the “rcompanion” package in R to determine distribution-free UCLs for percentiles, which is appropriate for skewed data (version 2.4.26; Mangiafico, 2023). The UCLs for the percentiles are also known as tolerance intervals. This calculation method is different from that used for previous PCQA reports (Wetherbee and Martin, 2016), which applied the binomial probability distribution to perform computations with SAS software (Hahn and Meeker, 1991; SAS Institute, Inc., 2016). Comparing the results of both calculation methods indicated negligible differences in the UCL.
Before determining contamination levels, concentrations of solutes in the samples that were less than the method detection limit (MDL) were assigned a value of one-half the MDL. Helsel (2012) determined how such substitution leads to bias in hypothesis tests and calculation of statistical locations, but for this report, the substitution of one-half the detection limit had a minor effect on calculated percentiles because the percentage of censored values was always less than 25 percent and was determined to have no discernable effect on quantification of the medians and interquartile ranges. In the 2020–22 study period, only 3 of 173 samples had concentrations that were less than analytical detection. The total number of samples was similar to what was noted in 2019–21, which was 185 samples; therefore, one-half the MDL was a convenient substitution for purposes of capturing reasonable estimates of bias and variability using the nonparametric methods described by Gibbons and Coleman (2001) for the field audit and system blank data. This method is also applicable for the colocated sampler program.
For the interlaboratory-comparison program data, most probable values (MPVs) for concentrations of solutes in split samples of natural and synthetic rainwater solutions were calculated as the median concentrations for each analyte in each unique solution. The MPVs were calculated using the Nondetects and Data Analysis for Environmental Data (more commonly known as NADA) package in R to incorporate values less than analytical detection limits (version 1.6–1.1; Lee, 2023). The NADA package uses survival-analysis techniques, such as the Regression on Ordered Statistics method, to properly include censored values reported as less than analytical detection limits. The Regression on Ordered Statistics method uses the empirical distribution functions of the positively skewed datasets, which are flipped end to end to plot the probabilities of exceedance of the observations. The method calculates the survival function probability of “surviving” to the next lowest uncensored concentration, given the amount of data at or less than that concentration. A complete explanation of these calculation and analysis methods is provided by Helsel (2012).
National Trends Network Quality-Assurance Programs
Programs operated for the NTN during 2021–22 include the field audit program and an interlaboratory-comparison program. The field audit program uses equipment-rinse samples (bucket samples) paired with corresponding de-ionized water or synthetic precipitation solutions (bottle samples) to identify changes to chemical concentrations in NTN wet-deposition samples resulting from field exposure of the sample-collection apparatus (Wetherbee and others, 2010; Wetherbee and Martin, 2017). The standing objectives of the NTN interlaboratory-comparison program are to (1) estimate the variability and bias in data reported by CAL and other participating laboratories and (2) facilitate integration of data from various wet-deposition monitoring networks.
Field Audit Program
Contamination can be introduced to NADP samples by dissolution of materials residing on the bucket walls. Sources of such materials include bucket and bag handling during deployment and sample collection and dry deposition into the exposed bucket while it is deployed. In contrast, loss of dissolved constituents from the solution is possible through adsorption into the bucket walls. Dissolved constituents from the solution can also be lost through other chemical or biological processes.
During 2021, site operators received field audit samples from the USGS and then waited for a week without wet deposition to process them in the deployed buckets. Site operators poured 75 percent of the volume of their field audit solution into the sample bucket and sealed the bucket with a lid for 24 hours before decanting the solution to a clean sample bottle (bucket sample). During 2022, the CAL prepared and shipped the field audit samples to the field for the first time in PCQA history. For 2021, the processing protocol specified that 25 percent of the field audit sample volume remain in the original sample bottle (bottle sample) without contacting any field sampling materials. The protocol was changed by the CAL to retain 50 percent of the bottle sample in 2022. Contamination and sample stability are evaluated for network data by statistical analysis of paired “bucket-minus-bottle” concentration differences for the processed field audit samples.
An NADP site operator who either processed and submitted a field audit sample to CAL or notified the USGS that an attempt was made to process the field audit sample during the year was considered to have participated in the field audit program. Field audit samples were shipped to 100 sites each year during 2020, 2021, and 2022. During 2020, 65 field audit samples were processed; during 2021, 67 field audit samples were processed; and during 2022, 57 field audit samples were processed (Nichole Miller, WSLH, written commun., 2023). During previous years, the number of field audit samples ranged from 9 (in 2018 when the CAL moved locations) to 180 in 2007 when samples were sent to all sites (Wetherbee, 2022). Since 2019, the number of samples has remained constant with return rates of approximately 60 percent.
Network Maximum Contamination Levels
The NMCLs in NTN samples were estimated by the 90-percent UCL for the 90th percentiles of paired sample-concentration differences calculated for each analyte. The NMCLs serve as practical lower limits of quantitation for network-measured wet deposition of chemical constituents (Wetherbee and others, 2010, 2014). The NMCLs are calculated for 3-year moving periods of time and were highest for hydrogen ion (1.41 microequivalents per liter [μeq/L], from pH), calcium (0.088 milligram per liter [mg/L]), nitrate (0.083 mg/L), and sulfate (0.058 mg/L) during the 2020–22 period (table 1). The NMCL for calcium is equivalent to the 39th percentile for all NTN samples during the 2020–22 period, which suggests that concentrations lower than this percentile may be indistinguishable from contamination.
Table 1.
National Atmospheric Deposition Program National Trends Network method detection limits, network maximum contamination levels, and analyte losses estimated from field audit samples in addition to calculated concentration quartiles for all valid monitoring data, 2019–22.[Data are available in Wetherbee (2022, 2023). All analyte concentrations are in milligrams per liter except hydrogen ion (microequivalents per liter); NTN, National Trends Network; MDL, method detection limit; NMCL, network maximum contamination level; p>|M|], sign test p-value, probability of rejecting the null hypothesis: “The true median of the differences is zero,” when true; NADP NTN, National Atmospheric Deposition Program National Trends Network; Q1, 25th percentile; Q3, 75th percentile; n.d., no data]
Calculated as the 90-percent upper confidence limits for the 90th percentiles of 2019–22 and 2020–22 paired sample-concentration differences using the bootstrap confidence limit function in R, where differences are calculated as bucket minus bottle.
The NMCL can be defined in three ways: (1) the NMCL is the maximum contamination expected in 90 percent of the samples with 90-percent confidence, (2) there is a 10-percent chance that contamination in NTN samples has been underestimated at the NMCL, or (3) there is 90-percent confidence that the contamination would exceed the NMCL in 10 percent of the NTN samples.
The 25th and 75th percentile values for all 2020–22 NTN monitoring data (Mark Kuether, NADP Program Office, WSLH, written commun., 2023) are compared to estimated annual NMCLs in table 1. Trends in the NMCLs are illustrated for each analyte in figure 1. The NMCLs for cations decreased slightly during 2020–22 compared to 2019–21. The MDL for ammonium increased from 0.01 mg/L to 0.014 mg/L between 2020 and 2022. This change in sensitivity for low-level ammonium concentrations could mask small changes in ammonium contamination, which was lower in 2020–22 compared to 2019–21. The NMCL for chloride, nitrate, and sulfate increased slightly compared to those levels for the 2019–21 period. This increase indicates a rise in contamination of NTN samples during 2021–22 for anions. When compared to the 2020–22 NTN percentile data, it is evident that values lower than the 23d percentile could be due to contamination (Wetherbee, 2022). For chloride, nitrate, and sulfate, this value is 0.041 mg/L, 0.307 mg/L, and 0.188 mg/L, respectively. The NMCLs for nitrate (0.083 mg/L) and sulfate (0.058 mg/L) were at the 10th and 3d percentiles for NADP measurements for these analytes, respectively; therefore, although the NMCL has risen in the most recent 3-year increment, the 2020–22 increases are not concerning.
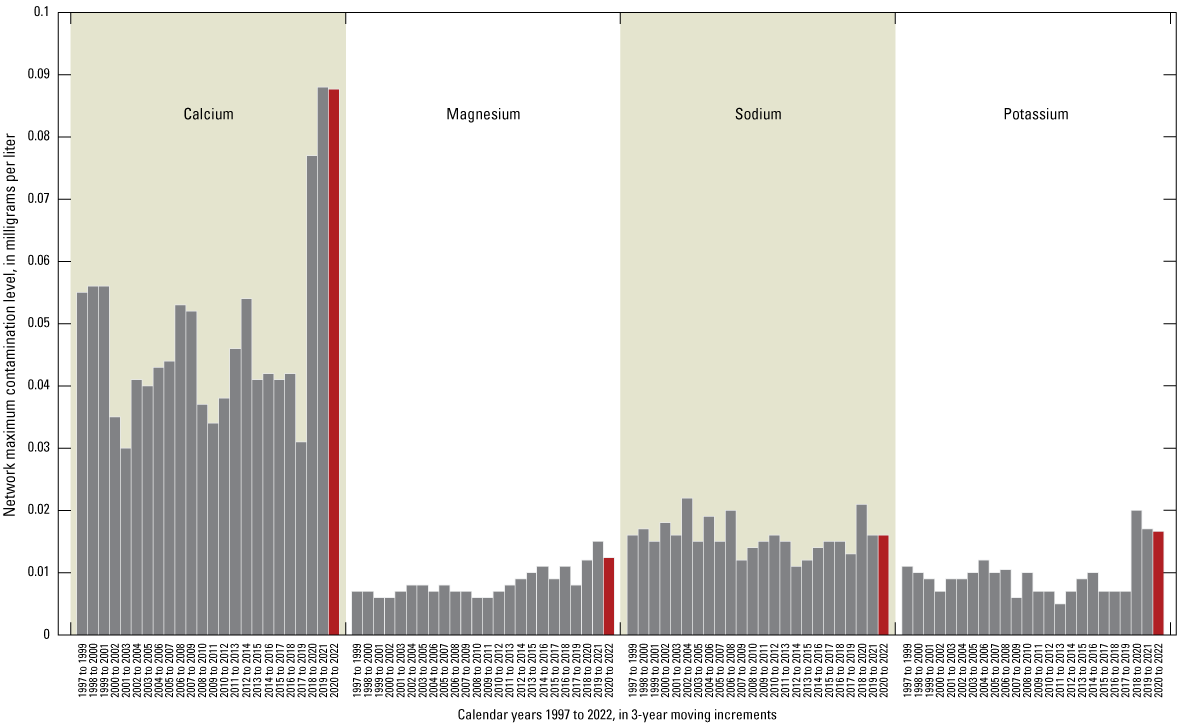
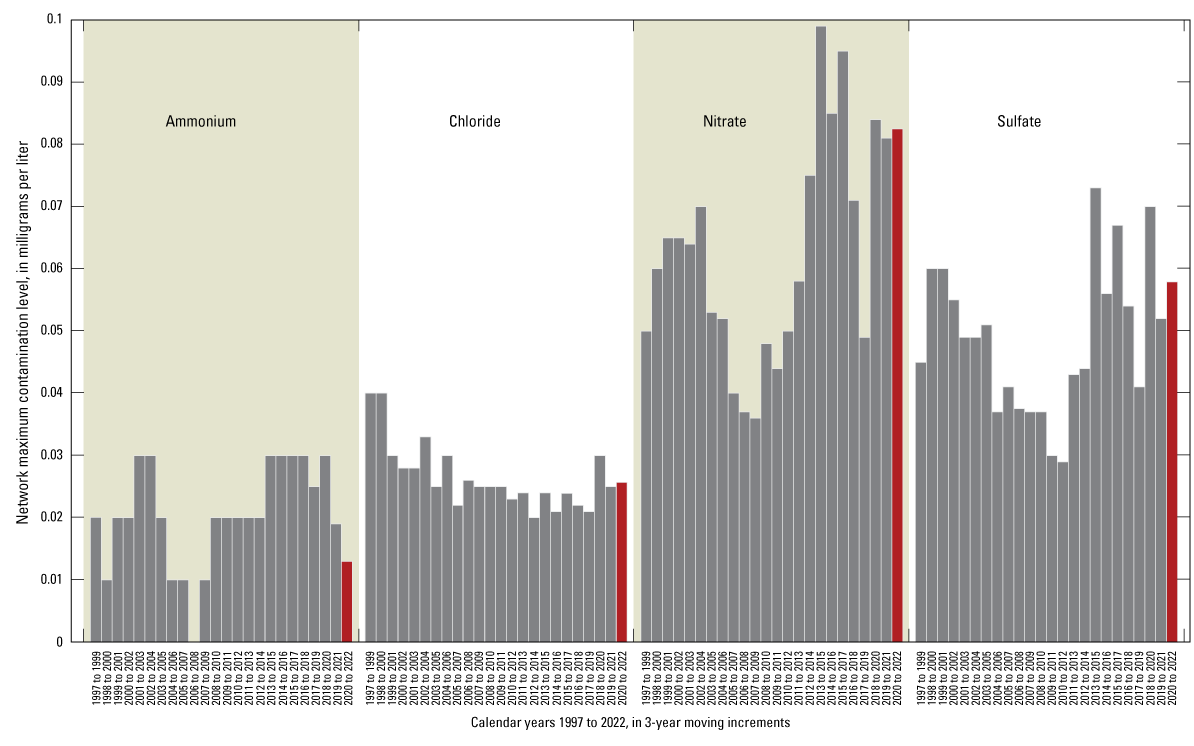
Network maximum contamination levels for National Trends Network analytes calculated using 3-year moving increments, 1997–2022. The 3-year increment for 2020–22 is shown with a red bar.
The NADP changed its sampling protocol during 2020 by lining the buckets with clean-room grade, disposable plastic bags to decrease shipping, washing, and packaging costs associated with the reusable buckets. Extensive laboratory testing of the bags by the CAL did not reveal contamination issues (Camille Danielson, WSLH, oral and written commun., 2020). Therefore, any contamination might be introduced by field handling of the bags during the installation procedure or when the bag-lined buckets are switched out at the time of sample collection when the bag-lined bucket containing the previous week’s sample and the fresh bag-lined bucket are briefly exposed to the atmosphere. Analyses completed by the Illinois State Water Survey in 2015 did not reveal a significant difference between analyte concentrations in samples collected using unlined and bag-lined buckets, indicating that the effect that the contamination has on the samples was negligible (Mark Rhodes, Illinois State Water Survey, written commun., 2016). However, the NMCL increases determined in 2018–20 indicate a substantial introduction of contamination during this period, wherein the WSLH began to analyze field audit samples and bag liners were introduced to the sampling protocol (fig. 1) but no other changes were introduced. In early 2023, the NADP transitioned to a newly constructed bag, primarily to improve field-handling protocols.
Analyte Losses
Maximum values for analyte losses in NTN samples were higher in 2020–22 than for the 2019–21 periods for all analytes except calcium, sodium, and potassium (table 1). Maximum values for nitrate and ammonium losses during 2020–22 were higher than those for the previous 3 years (fig. 2A) . The MDL for ammonium is the second highest at the CAL at 0.014 mg/L for 2021–22. The CAL detected losses of nitrate and ammonium (~50–65 percent) from low-concentration solutions that were allowed to dwell in bag liners in laboratory studies (NADP, 2019). Increased ammonium loss could also be due to the switch to bag-lined bucket sampling protocols mentioned previously. Hydrogen-ion maximum loss decreased from 4.6 μeq/L during 2019–21 to 4.1 μeq/L during 2020–22 (fig. 2B). Note that the hydrogen ion was biased high in 2019. Therefore, the increased calcium contamination and concurrent loss of hydrogen ion in NTN samples might be related to changing environmental conditions, such as a shift to a drier climate and dustier troposphere (Hedin and Likens, 1996; Brahney and others, 2015). Finally, the CAL identified a pH calibration error in early 2020 that caused some hydrogen-ion concentrations to have a slightly high bias, which might have accentuated the bucket-minus-bottle hydrogen-ion concentration differences during the 2018–20 period. In 2022, the positive analytical bias for the hydrogen-ion concentrations was back once again. It is possible that this bias can still be attributed to bag contamination or mishandling of the bags now used in the buckets or other environmental related contamination sources. The maximum hydrogen-ion loss of 4.1 μeq/L is less than the median valid NTN hydrogen-ion concentration (4.6 μeq/L) measured during 2019–21. Calcium and magnesium NMCLs decreased by 2 and 4 percent, respectively, during this same period, indicating less buffering of hydrogen ion with dust contamination. The 3-year median calcium and magnesium concentrations remained nearly unchanged, and the upper quartile calcium and magnesium concentrations in NTN samples decreased by 6 and 15 percent, respectively, between 2018 and 2020 (Wetherbee and others, 2023) and between 2020 and 2022 (table 1). The hydrogen-ion data indicate a slight decrease in NTN concentration loss between 2019 and 2021 (4.6 μeq/L) and between 2020 and 2022 (4.1 μeq/L) (fig. 2B).
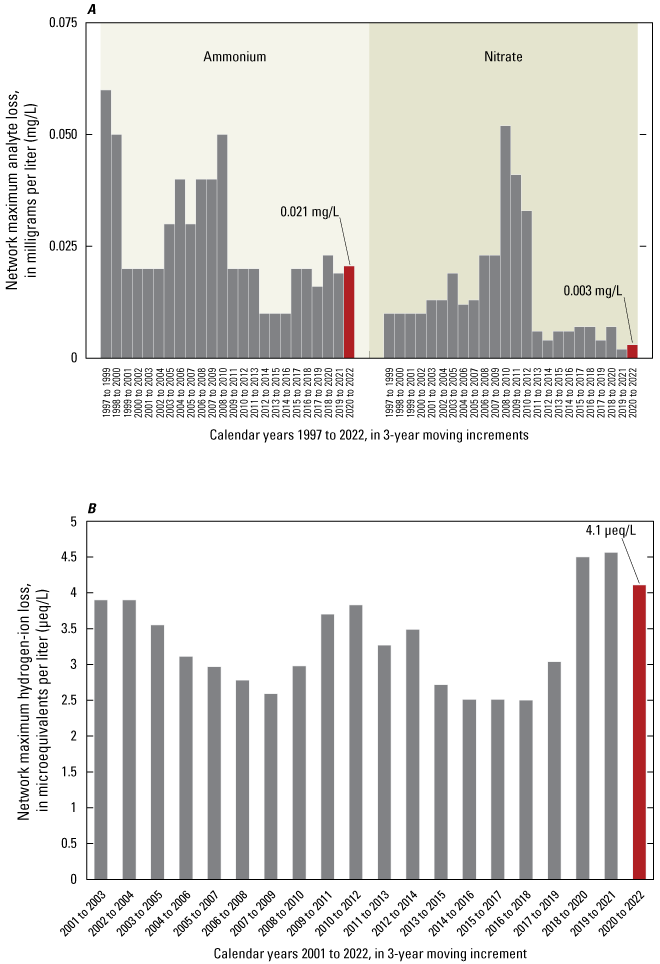
Maximum loss of (A) ammonium and nitrate and (B) hydrogen ion from weekly National Trends Network samples calculated using 3-year moving increments, 1997–2022. The 3-year increment for 2020–22 is shown with a red bar.
National Trends Network Interlaboratory-Comparison Program
The CAL’s analytical variability and bias were evaluated with respect to other international monitoring networks in the Northern Hemisphere. No attempt was made to account for the different onsite sampling protocols used by different monitoring networks. During 2021–22, 11 laboratories participated in the interlaboratory-comparison program:
-
1. Asia Center for Air Pollution Research (ACAP), in Niigata-shi, Japan;
-
2. Illinois State Water Survey PRI (formerly NADP Central Analytical Laboratory during 2017–18), in Champaign, Illinois;
-
3. Wood Group, Inc. (WOOD), in Gainesville, Florida;
-
4. Ministry of the Environment and Climate Change (MOECC), Dorset Chemistry Laboratory in Dorset, Ontario, Canada;
-
5. Environment and Climate Change Canada, Science and Technology Branch (ECST), in Downsview, Ontario, Canada;
-
6. Norwegian Institute for Air Research (NILU) in Kjeller, Norway;
-
7. Cary Institute of Ecosystem Studies (CIES) in Millbrook, N.Y.;
-
8. U.S. Department of Agriculture, Forest Service, Northern Research Station (NRS) in Durham, New Hampshire;
-
9. RTI International (RTI) in Research Triangle Park, North Carolina;
-
10. Universidad Nacional Autónoma de México (UNAM), Centro de Ciencias de la Atmósfera, in Mexico City, Mexico; and
-
11. NADP CAL, WSLH, in Madison, Wis. (formerly known as WCAL during 2017–18).
Each of the participating laboratories received four samples from PCQA every month for chemical analysis. Three types of samples were used in the interlaboratory-comparison program: (1) synthetic standard reference samples prepared by PCQA, which are traceable to National Institute of Standards and Technology reference materials (National Institute of Standards and Technology-traceable materials); (2) de-ionized water blank samples prepared by PCQA; and (3) natural wet-deposition samples collected at NTN sites, blended by CAL, and sent to PCQA for shipping to the laboratories as blind samples (Wetherbee and Martin, 2017). Synthetic precipitation samples used in the interlaboratory-comparison program were made from stock solutions prepared by High Purity Standards (Charleston, South Carolina). Natural samples were filtered through 0.45-micrometer filters; contained in 60-, 125-, and 250-milliliter polyethylene bottles by CAL; and shipped in chilled, insulated containers to the PCQA to enhance stability of nutrient analytes—ammonium, nitrate, and sulfate—in the samples (Tchobanoglous and Schroeder, 1987; U.S. Geological Survey, 2002).
Median concentrations of calcium, magnesium, sodium, potassium, ammonium, chloride, nitrate, sulfate, and hydrogen ion and median specific conductance were computed by solution from the data submitted by all participating laboratories. The median values were considered to be equal to the MPV. Censored concentration values reported as less than the MDL were included in the estimation of MPV for each solution using the ROS method (Helsel, 2012). The largest percentages of censored concentration values in the samples analyzed during 2021–22 were those for magnesium and potassium (7.2 and 5.2 percent, respectively), most commonly in natural wet-deposition samples. For 2021–22, the number of samples analyzed for each solution ranged from 10 to 22, and the mean was 20 samples analyzed per solution (table 2). The MPVs for the synthetic precipitation solutions and the number of samples analyzed per solution are listed by solution identifier in table 2.
Table 2.
Most probable values for analytes in natural precipitation solutions used in the 2021 and 2022 National Trends Network interlaboratory-comparison program.[Data are available in Wetherbee (2023). Ca2+, calcium; Mg2+, magnesium; Na+, sodium; K+, potassium; NH4+, ammonium; Cl−, chloride; NO3−, nitrate; SO42−, sulfate; H+, hydrogen-ion concentration from pH; all analyte concentrations are in milligrams per liter except hydrogen ion (microequivalents per liter); specific conductance is given in microsiemens per centimeter at 25 degrees Celsius]
Data from each laboratory were compared against these MPVs to evaluate bias. The RTI laboratory routinely analyzed the samples only for chloride, nitrate, sulfate, sodium, potassium, and ammonium. The ECST, NRS, and RTI laboratories did not analyze the samples for specific conductance. The NRS and RTI laboratories did not measure pH for any of the samples. Data are missing for many NRS samples for 2022.
The CAL participates in the USGS Standard Reference Sample (SRS) Project (https://bqs.usgs.gov/srs/, accessed August 2, 2023). Data for the 2021 USGS SRS Project results are available in the CAL’s 2021 Quality Assurance Report (University of Wisconsin-Madison, Wisconsin State Laboratory of Hygiene, 2023). The 2022 SRS Project results for the CAL and HAL were ranked as “very good” by the SRS Project, with sulfate being the only analyte with a percentage error greater than 10 percent (16 percent) in the spring of 2022 (University of Wisconsin-Madison, Wisconsin State Laboratory of Hygiene, 2024).
Bias and Variability Within the Interlaboratory-Comparison Program
Interlaboratory bias for the participating laboratories was evaluated using the following methods: (1) comparison of the medians of the differences between laboratory results and MPV, (2) hypothesis testing using the sign test, and (3) comparison of laboratory results for de-ionized water samples. The arithmetic signs of the median differences indicate whether the reported results for each constituent are positively or negatively biased. The sign test null hypothesis states the true median of the reported results minus MPV differences is zero. Test results were evaluated at the α=0.05 significance level for a two-tailed test.
Calculated variation between laboratories was compared using the f-psig ratios (eq. 6). Analytical detection limits are reported for each laboratory in table 3. Results for variability and bias within the analytical data reported by each of the participating laboratories are presented in tables 4 and 5. For this program, statistically significant bias was determined to be of practical importance only when the absolute value of the median relative concentration difference was greater than the participating laboratory’s analytical MDL.
Table 3.
Analytical detection limits for laboratories participating in the U.S. Geological Survey interlaboratory-comparison program for the National Atmospheric Deposition Program, 2021–22.[Data are available in Wetherbee (2023). Participating laboratories: ACAP, Asia Center for Air Pollution Research; CAL, Central Analytical Laboratory, Wisconsin State Laboratory of Hygiene, University of Wisconsin-Madison; CIES, Cary Institute of Ecosystem Studies; ECST, Environment and Climate Change Canada, Science and Technology Branch; MOECC, Ministry of the Environment and Climate Change; NILU, Norwegian Institute for Air Research; NRS, U.S. Department of Agriculture, Forest Service, Northern Research Station; PRI, Illinois State Water Survey, Prairie Research Institute; RTI, RTI International; UNAM, Universidad Nacional Autónoma de México; WOOD, Wood Group, Inc.; mg/L, milligram per liter; —, no data]
Table 4.
Median differences between reported constituent concentrations and most probable values for synthetic wet-deposition samples, 2021 interlaboratory-comparison program.[Data are available in Wetherbee (2023). Overall f-psig, f-pseudosigma for all participating laboratories; median diff., median of differences between each laboratory’s individual results and the most probable value during 2021, in analyte units; sign test p-value, probability of rejecting the null hypothesis: “The true median of the differences between laboratory results and the most probable value is zero,” when true; f-psig ratio, ratio of each individual laboratory’s f-pseudosigma to the overall f-pseudosigma; ACAP, Asia Center for Air Pollution Research; <, less than; CAL, Central Analytical Laboratory, Wisconsin State Laboratory of Hygiene, University of Wisconsin-Madison; CIES, Cary Institute of Ecosystem Studies; ECST, Environment and Climate Change Canada, Science and Technology Branch; —, not calculated; MOECC, Ministry of the Environment and Climate Change; NILU, Norwegian Institute for Air Research; NRS, U.S. Department of Agriculture, Forest Service, Northern Research Station; PRI, Illinois State Water Survey, Prairie Research Institute; RTI, RTI International; UNAM, Universidad Nacional Autónoma de México; WOOD, Wood Group, Inc.; all analyte concentrations are in milligrams per liter, except hydrogen ion (microequivalents per liter); specific conductance is given in microsiemens per centimeter at 25 degrees Celsius; spec. cond., specific conductance]
Denotes values for which the median bias is greater than the method detection limit (table 3) and statistically significant (α=0.05; Kanji, 2006).
Table 5.
Median differences between reported constituent concentrations and most probable values for synthetic wet-deposition samples, 2022 interlaboratory-comparison program.[Data are available in Wetherbee (2023). Overall f-psig, f-pseudosigma for all participating laboratories; median diff., median of differences between each laboratory’s individual results and the most probable value during 2021, in analyte units; sign test p-value, probability of rejecting the null hypothesis: “The true median of the differences between laboratory results and the most probable value is zero,” when true; f-psig ratio, ratio of each individual laboratory’s f-pseudosigma to the overall f-pseudosigma; ACAP, Asia Center for Air Pollution Research; <, less than; CAL, Central Analytical Laboratory, Wisconsin State Laboratory of Hygiene, University of Wisconsin-Madison; CIES, Cary Institute of Ecosystem Studies; ECST, Environment and Climate Change Canada, Science and Technology Branch; —, not calculated; MOECC, Ministry of the Environment and Climate Change; NILU, Norwegian Institute for Air Research; NRS, U.S. Department of Agriculture, Forest Service, Northern Research Station; PRI, Illinois State Water Survey, Prairie Research Institute; RTI, RTI International; UNAM, Universidad Nacional Autónoma de México; WOOD, Wood Group, Inc.; all analyte concentrations are in milligrams per liter, except hydrogen ion (microequivalents per liter); specific conductance is given in microsiemens per centimeter at 25 degrees Celsius; spec. cond., specific conductance]
Denotes values for which the median bias is greater than the method detection limit (table 3) and statistically significant (α=0.05) (Kanji, 2006).
During 2021, the interlaboratory-comparison results for ECST, RTI, and WOOD indicated no practically significant bias (in other words, greater than the absolute values of the detection limits) for all analytes. In 2022, ECST was the only participating laboratory that indicated no practically important bias. For the other participating laboratories, the results indicated practically significant bias for the following analytes by year:
-
• ACAP—nitrate and sulfate in 2021 and sulfate and hydrogen ion in 2022.
-
• CAL—nitrate and hydrogen ion in 2021 and chloride, sulfate, and hydrogen ion in 2022, noting that the hydrogen-ion bias was even higher in 2022.
-
• CIES—sodium and nitrate in 2021–22, chloride in 2021, and calcium in 2022.
-
• MOECC—calcium and nitrate in 2021–22 and sulfate, hydrogen ion, and specific conductivity in 2022.
-
• NILU—hydrogen ion in 2021 and ammonium and sulfate in 2022.
-
• NRS—chloride and sulfate in 2021–22 and sodium and sulfate in 2021 only.
-
• PRI—calcium in 2021 and sulfate in 2021–22.
-
• RTI—ammonium and nitrate in 2022.
-
• WOOD—calcium, nitrate, and specific conductance in 2022.
The CAL results demonstrated moderately high variability (f-psig ratio greater than 1.0) for magnesium, chloride, nitrate, and hydrogen ion in 2021 but slightly higher relative variability only for hydrogen ion in 2022 (tables 4 and 5). Results for the ACAP, ECST, PRI, RTI, and WOOD laboratories indicated generally low variability for most analytes except hydrogen ion during 2021–22. Meanwhile, higher variability than overall was indicated for several analytes for MOECC, NILU, NRS, and UNAM (tables 4 and 5). Variability for MOECC, UNAM, and NRS was generally higher than overall during 2022. The median differences between constituent concentrations and MPVs for synthetic wet-deposition samples measured at NRS were incomplete in 2022, which affected the measured performance of the laboratory.
Blank samples were not shipped to laboratories during 2021 because of the COVID-19 pandemic. The interlaboratory-comparison program shipped four blank samples to participating laboratories each year during 2022. MOECC and NRS only analyzed three blank samples and UNAM analyzed only one (table 6). The ACAP laboratory reported two detections for calcium, potassium, and ammonium, and four detections for sodium in 2022. The CAL did not report any concentrations greater than analytical detection limits during 2022. The CIES laboratory reported two detections for chloride, and sulfate, four detections for nitrate, and three detections for potassium during 2022. The ECST laboratory reported detections for potassium in all blank samples during 2022 and detections for calcium in three of the four blank samples. The MOECC laboratory reported detections for ammonium and nitrate in all three samples; detections for magnesium, potassium, and sulfate in one of the samples; and detections for sodium in two of the 2022 samples. The NILU laboratory reported four detections for ammonium, two for potassium, and one for calcium and sodium. The NRS laboratory reported detections for all three of its blanks for sulfate, chloride, and potassium, and two detections were reported for sodium, with magnesium, and calcium having one detection. The PRI laboratory reported two detections for sulfate and chloride. RTI had one result greater than detection for ammonium. WOOD and UNAM did not have any results greater than the detection limit (note that UNAM only had processed one blank sample). The UNAM laboratory data were also incomplete because of sample shipping difficulties in 2022.
Table 6.
Number of analyte determinations greater than the method detection limits for de-ionized water samples, 2022.[Data are available in Wetherbee (2023). No blanks were shipped to the laboratories during 2021 because of the COVID-19 pandemic. Participating laboratories: ACAP, Asia Center for Air Pollution Research; CAL, Central Analytical Laboratory, Wisconsin State Laboratory of Hygiene, University of Wisconsin-Madison; CIES, Cary Institute of Ecosystem Studies; ECST, Environment and Climate Change Canada, Science and Technology Branch; MOECC, Ministry of the Environment and Climate Change; NILU, Norwegian Institute for Air Research; NRS, U.S. Department of Agriculture, Forest Service, Northern Research Service; PRI, Illinois State Water Survey, Prairie Research Institute; RTI, RTI International; UNAM, Universidad Nacional Autónoma de México; WOOD, Wood Group, Inc.; —, no data]
Interlaboratory-Comparison Control Charts
Each participating laboratory’s results were compared to the MPV over time in control charts. Control charts were generated for each laboratory and served to facilitate the visual identification of bias, and the difference of individual concentration results from that of the MPV falling outside of the control limit was an indicator of potential laboratory bias. For values that are far outside of the control limit, laboratories can use the data to determine if there are any analytical or environmental considerations for that period as to why the samples were so far out of range. Analyte determinations that exceeded the control limits (plus or minus [±] 3 f-psig) during 2021–22 are summarized in table 7. Each laboratory was provided with its own sets of control charts. The CAL values were mostly within the ±3 f-psig statistical control limits for all analytes, with the exception of the hydrogen ion, for which values were reported at about ±4 f-psig in 2021 and ±6 f-psig during 2022. The laboratory once again has issues with stable pH calibration, which was seen previously in 2020 (University of Wisconsin-Madison, Wisconsin State Laboratory of Hygiene, 2021b). All the CALNAT samples plotted within statistical control for the CAL, and all values other than chloride were reported within ±2 f-psig. The laboratory is looking into an automated system that would replace the current manual pH method, which is expected to reduce the variability of results.
Table 7.
Number of analyte determinations outside ±3 f-pseudosigma statistical control limits, by participating laboratory, 2021–22.[Data are available in Wetherbee (2023). n, number of samples analyzed; Ca2+, calcium; Mg2+, magnesium; Na+, sodium; K+, potassium; NH4+, ammonium; Cl−, chloride; NO3−, nitrate; SO42−, sulfate; Sc, specific conductance ; H+, hydrogen-ion concentration from pH; ACAP, Asia Center for Air Pollution Research; CAL, Central Analytical Laboratory, Wisconsin State Laboratory of Hygiene, University of Wisconsin-Madison; CIES, Cary Institute of Ecosystem Studies; ECST, Environment and Climate Change Canada, Science and Technology Branch; —, no data; MOECC, Ministry of the Environment and Climate Change; NILU, Norwegian Institute for Air Research; NRS, U.S. Department of Agriculture, Forest Service, Northern Research Service; PRI, Illinois State Water Survey, Prairie Research Institute; RTI, RTI International; UNAM, Universidad Nacional Autónoma de México; WOOD, Wood Group, Inc.]
Control charts for the CAL for 2021–22 are shown in figure 3A and B. Points in the control charts are color coded by solution type to provide a visual indication of potential solution-specific bias. During 2021, bias trended from slightly negative in early 2021 to slightly positive in late 2022 for ammonium (fig. 3A). Hydrogen-ion concentrations trended from slightly positive in 2021 to strongly positive in 2022 (fig. 3B). During 2021–22, no significant bias was observed in the control chart for nitrate. In 2022, no analytical bias was observed for sodium, magnesium and calcium. The CAL specific conductance results indicated a positive documented bias during 2021 but no bias during 2022 (fig. 3B). Compared to the 2019–20 control charts, calcium, sodium, and magnesium decreased, nitrate increased slightly, and sulfate was higher in 2022 than the previous period (Wetherbee and others, 2023). Note that ammonium seems to be within better control in the 2021–22 period, even with tighter control limits. A positive documented bias for hydrogen-ion results was detected in 2021 and 2022, with the highest values reported in 2022 (fig. 3B).
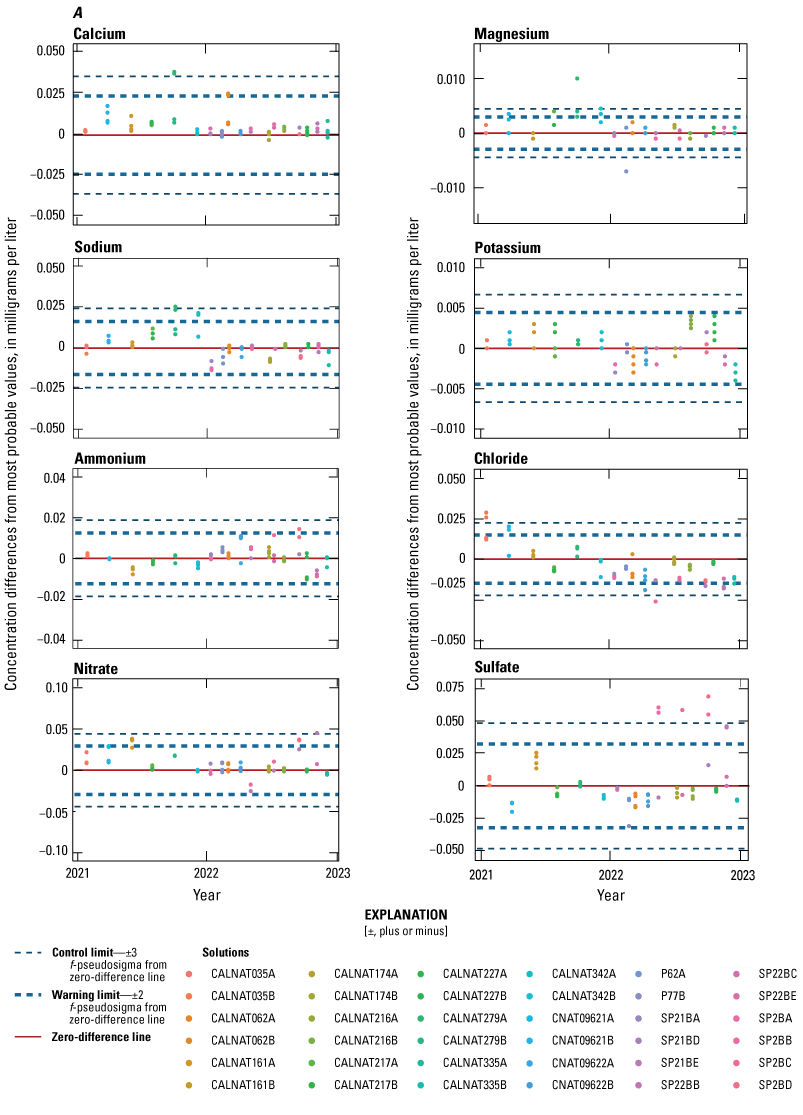
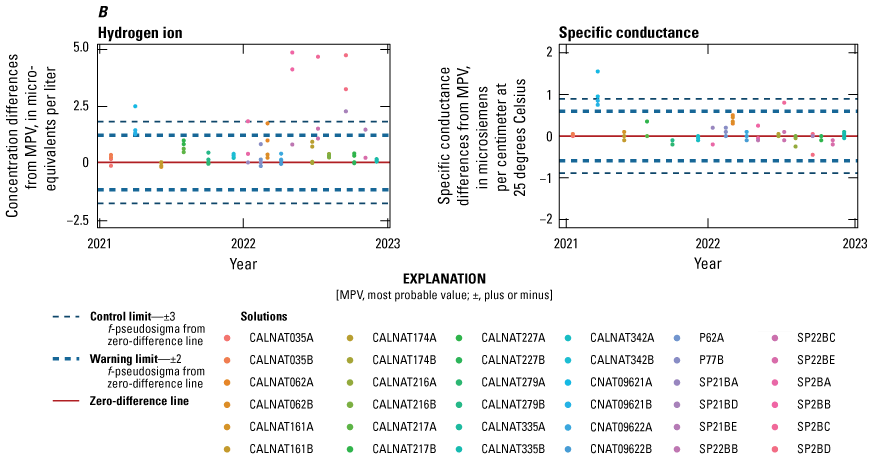
Differences between concentration values reported by the Central Analytical Laboratory at the Wisconsin State Laboratory of Hygiene, University of Wisconsin-Madison, and the most probable values for all participating laboratories in the interlaboratory-comparison program for the National Trends Network, 2021–22, for (A) calcium, magnesium, sodium, potassium, ammonium, chloride, nitrate, and sulfate and (B) hydrogen-ion concentrations and specific conductance. Data are available in Wetherbee (2023).
Colocated Program
The USGS resumed the NTN colocated program in 2021, after not operating the program in 2019 and 2020. In 2021 colocated N-CON Systems Company, Inc. (N-CON), collectors were operated at NTN sites OK00 and 00OK at Salt Plains National Wildlife Refuge, Oklahoma, and TX56 and 56TX at Lyndon B. Johnson National Grasslands, Texas. In 2022, the colocated N-CON collectors were at NE99 and 99NE at the North Platte Agricultural Experiment Station in Nebraska and OH09 and 09OH at Oxford, Ohio. Sites were selected based on operator willingness to participate in the program and the ability to represent a wide variety of geographical and meteorological conditions. A single OTT Pluvio-2 precipitation gage measured and recorded precipitation depth and the operation of the N-CON collectors. Colocated sites were operated using identical field and laboratory sample-collection and sample-analysis procedures (University of Wisconsin-Madison, Wisconsin State Laboratory of Hygiene, 2019).
In both years, weekly precipitation chemistry data from colocated sites were analyzed for differences in analyte concentrations in samples obtained from the original NTN collector and the colocated collector. Only valid data (quality rating codes “A” and “B”) identified in the NADP by a laboratory-type code “W” (sufficient volume for complete analysis) and “WI” (volume for incomplete analysis) were used (NADP, 2023b). Samples flagged as contaminated by the NADP were considered prone to a greater error component. For this reason, valid contaminated samples were eliminated from statistical analysis, which is consistent with previous reports (Wetherbee and Martin, 2020). Median absolute percentage differences for replicate samples from the colocated N-CON samplers are in table 8. Although many of the analytes had similar concentrations for both samplers, higher median absolute percentage differences in weekly colocated measurements were detected for potassium and hydrogen ion in both years and calcium, magnesium, and sodium as well in 2022. Concentration differences were higher at both sites in 2022 compared to the 2021 data. The data for OH09 and 09OH indicate lower overall variability than the data for NE99 and 99NE, which can be at least partially attributed to the total number of replicate samples being substantially lower for NE99 and 99NE. This variability can partially be attributed to sample volume and catch efficiency discrepancies, which were most pronounced at the NE99 and 99NE sites, as well as evaporation as a result of differences in the lid pad seal tightness on the sampling bucket. Overall, the 2021–22 colocated data indicated overall variability in NTN measurements ranging from about ±2.0 to 12 percent. This range of variability is comparable to results from the previous colocated studies in 2017 and 2018, where the variability ranged from ±3.2 to 11 percent, with potassium and hydrogen ion being exceptions (22 and 32 percent in 2018, respectively; Wetherbee and Martin, 2020). Variability among samplers and sites is likely attributed to differences in the sensitivities in the sensors opening and closing the buckets, age and tightness of the lid pad seals, and temperature and precipitation type differences. This results in varying amounts of precipitation collected or remaining after some of the sample has evaporated, thus changing the concentration of the constituents. These results are intended to be used as error terms in NADP concentration data.
Table 8.
Median absolute percentage differences for replicate samples from identical colocated N-CON Systems Company, Inc., samplers at National Atmospheric Deposition Program sites OK00, TX56, NE99, and OH09, 2021–22.[Data are available in Wetherbee (2023). n, number of samples; Ca+2, calcium; Mg+2, magnesium; Na+, sodium; K+, potassium; NH4+, ammonium; Cl−, chloride; NO3−, nitrate; SO4−2, sulfate; H+, hydrogen-ion concentration from pH; OK00/00OK, Salt Plains National Wildlife Refuge, near Jet, Oklahoma; TX56/56TX, Lyndon B. Johnson National Grasslands, near Alvord, Texas; NE99/99NE, North Platte Agricultural Experiment Station, near North Platte, Nebraska; OH09/09OH, Miami University-Ohio, near Oxford, Ohio]
Mercury Deposition Network Quality-Assurance Programs
The USGS operated a system blank program and an interlaboratory-comparison program for the MDN during 2021–22. The MDN system blank program is similar to the NTN field audit program, whereby the effects of onsite environmental exposure, handling, and shipping on sample contamination are evaluated. The MDN interlaboratory-comparison program quantified variability and bias of MDN analytical data provided by the HAL for 2021–22. Protocols for the PCQA external quality-assurance programs for MDN are described in detail by Wetherbee and Martin (2017).
System Blank Program
The MDN site operators received de-ionized water system blank samples from PCQA for processing during 2021–22. The HAL prepared and shipped the 2022 system blank samples to the field for the first time in PCQA history. After a week without wet deposition at a site, operators poured one-half of the volume of the system blank solution through the glass sample train. The glass sample train consists of a funnel, which collects the precipitation, and a thistle tube, which drains the precipitation into the sample bottle. The solution that washed through the sample train is called the system blank sample, and the solution remaining in the original sample bottle is called the bottle sample. System blank and bottle samples were sent together to HAL for total mercury analysis. During 2021, 37 system blank samples were processed, and during 2022, 49 system blank samples were processed (Nichole Miller, WSLH, written commun., 2023). In all, 86 system blank samples were processed with accompanying bottle samples for chemical analysis at the HAL, and data for these system blank sample pairs were received by PCQA for assessment of sample contamination and stability.
Network Maximum Contamination Levels for Mercury
The NMCLs for total mercury were calculated from the system blank data using a 3-year moving window, starting with 2004–6. The NMCLs during the 2019–21 and 2020–22 periods were not greater than 0.758 nanogram per liter (ng/L) and 0.790 ng/L, respectively. The most recent NMCLs were lower than during the 2017–19 and 2018–20 periods, when the NMCLs were not greater than 1.00 ng/L and 0.926 ng/L, respectively (Wetherbee and others, 2023). These concentrations are less than the first percentile of all MDN sample data collected during 2020–22, which is 1.65 ng/L. Therefore, contamination in MDN samples during 2017–20 was less than the first percentile of all MDN concentrations with 90-percent confidence, and no more than 10 percent of the MDN samples had contamination concentrations in excess of about 1.0 ng/L with 90-percent confidence (Mark Kuether, NADP Program Office, WSLH, written commun., 2023). Although there have been slight changes in the NMCL in the last few years, the changes are not substantial and the degree of accuracy of the samples remains quite good for comparison purposes (Wetherbee and others, 2023).
Mass of Mercury Contamination
The mass of mercury contamination in each system blank sample was calculated using equation 7 listed in the “Statistical Methods” section. The mass of mercury contamination in each system blank sample and the UCLs of the percentiles of the system blank sample minus bottle sample mercury mass differences were calculated. The maximum estimated contaminant mass per sample remained the same at 0.090 ng of mercury during 2019–21 and 2020–22. The MDN NMCL for total mercury contamination mass per sample has been stable at about 0.1 ng per sample over the past 8 years (table 9).
Table 9.
The 3-year moving network maximum contamination levels and 90-percent upper confidence limits at the 50th, 75th, and 90th percentiles of total mercury contamination mass in system blank samples, 2004–22.[Data are available in Wetherbee (2020a, b, 2022, 2023). ng total Hg/L, total nanograms of mercury per liter; %, percent; UCL, upper confidence limit; Hg, total mercury; ng Hg, nanogram of mercury]
Mercury Deposition Network Interlaboratory-Comparison Program
The objective of the MDN interlaboratory-comparison program is to compare the variability and bias of HAL analytical results with results from laboratories supporting various monitoring networks, while not accounting for the different onsite protocols used by different networks. Nine laboratories participated in the program during the study period:
-
1. HAL at WSLH in Madison, Wis.;
-
2. Department of Atmospheric Science, National Central University, in Jhong-Li District, Taoyuan City, Taiwan;
-
3. Flett Research, Ltd., in Winnipeg, Manitoba, Canada;
-
4. Swedish Environmental Institute in Göteborg, Sweden;
-
5. Quebec Laboratory for Environmental Testing (commonly referred to as “LEEQ” or “QLET”) in Montreal, Quebec, Canada;
-
6. North Shore Analytical, Inc. (commonly referred to as “NSA”), in Duluth, Minnesota;
-
7. USGS Wisconsin Mercury Research Laboratory (commonly referred to as “WML”) in Middleton, Wis.;
-
8. National Institute for Minamata Disease (commonly referred to as “NIMD”), Minamata, Kumamoto, Japan; and
-
9. Environmental Research and Training Center (commonly referred to as “ERTC”), Amphoe Klong Luang, Pathumthani, Thailand.
During 2021, each participating laboratory received six samples per quarter consisting of 1-percent (volume:volume) hydrochloric acid blanks and mercuric nitrate spiked at four concentrations in a 1-percent hydrochloric acid matrix. The laboratories were instructed to analyze the samples as soon as they were received to promote accurate time representation of the data. All samples were single-blind samples, whereby the chemical analyst knew that the sample was a quality-control sample but did not know the total mercury concentrations in the samples. The stock solutions used to make the samples are used for several years, so it is expected that the concentrations may decrease over time, which is acceptable because the project is primarily concerned with the medians of the results by all participating laboratories. The medians of all the concentration values obtained from the participating laboratories were considered to be MPVs, which are listed in table 10. The MPVs are similar to the values in 2019–20 (Wetherbee and others, 2023), indicating consistent preparation of these solutions for this project.
Table 10.
Most probable values for total mercury in spiked solutions and hydrochloric acid blank samples used for the U.S. Geological Survey Mercury Deposition Network interlaboratory-comparison program, 2021–22.[Data are available in Wetherbee (2023). Blank MPV estimated by Regression on Ordered Statistics method in R–NADA package because of large number of censored values. Hg, total mercury; MPV, most probable value; ng/L, nanogram per liter; %, percent; HCl, hydrochloric acid; BLANK, mercury-free de-ionized water with 1% HCl by volume; <d.l., less than analytical detection limit, number of samples equals 9 for 2021 and 2022; MP1–MP4, mercuric nitrate standard diluted to target concentrations in 1% HCl]
Control Charts
Total mercury analysis data submitted by each laboratory were compared to MPVs for each of the solutions. Differences between reported results and MPVs were plotted on annual control charts, which were delivered to each laboratory by PCQA. Control charts include warning limits placed at ±2 f-psig and control limits at ±3 f-psig from the zero-difference line. Values outside the control limits represent periods when a laboratory’s analyses were considered to be out of statistical control. The HAL control chart is shown in figure 4.
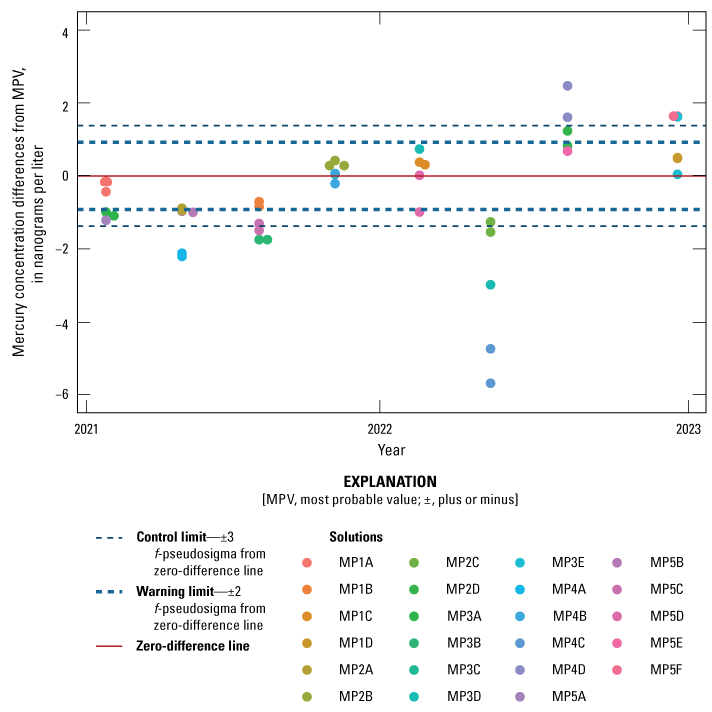
Differences between total mercury concentrations reported by the Mercury Analytical Laboratory at the Wisconsin State Laboratory of Hygiene, University of Wisconsin-Madison, and the median concentration values for all participating laboratories in the interlaboratory-comparison program for the Mercury Deposition Network, 2021–22. Data are available in Wetherbee (2023).
The HAL control chart shows that its results had a slight negative bias during 2021 and a slight positive bias in 2022 (fig. 4). It should be noted that the second quarter of 2022 had a set of six samples for which four results were outside of the ±3 f-psig control limits (fig. 4). A positive analytical bias in the HAL MDN 2022 results was observed (about 0.38 ng/L), where the f-psig was 1.51 ng/L and the f-psig ratio was 220 percent higher than overall among participating laboratories. During the previous study period in 2019, the HAL’s analytical variability was lower than the overall interlaboratory variability (f-psig ratio of 0.80; Wetherbee and others, 2023). In 2020, the HAL results indicated higher analytical variability (f-psig ratio of 1.94) and a median difference from the MPV of −1.120 ng/L, which was significantly different from zero (probability less than 0.0001). Although this variability was statistically significant in 2020, the HAL was successful on other performance testing samples.
Interlaboratory Variability and Bias
Each laboratory’s results for variability and bias are summarized in table 11. Methods for evaluation of the data are analogous to those for the NTN interlaboratory-comparison program. The f-psig ratio was computed as documented in equation 6 and expressed as a decimal for each laboratory, whereby an f-psig ratio larger than 1.00 indicates that results provided by a laboratory demonstrated higher variability than the overall variability among the participating laboratories; a ratio smaller than 1.00 indicates less variability than overall. Annual overall f-psig values were 0.380 ng/L and 0.546 ng/L for 2021 and 2022, respectively (table 11). The arithmetic signs of the median differences indicate whether the results of analyses for total mercury were positively or negatively biased. Interlaboratory bias was evaluated for statistical significance using the sign test for location of a median (Kanji, 2006; Wetherbee and others, 2014).
Table 11.
Differences between reported concentrations and most probable values for total mercury determinations, Mercury Deposition Network interlaboratory-comparison program, 2021–22.[Data are available in Wetherbee (2023). Overall f-psig, f-pseudosigma for all participating laboratories; ng/L, nanogram per liter; median diff., median of differences between each laboratory’s individual results and the most probable values for each solution; sign test p-value, probability of rejecting the null hypothesis: “The true median of the differences between laboratory results and the most probable value is zero,” when true; f-psig ratio, ratio of each individual laboratory’s f-pseudosigma to the overall f-pseudosigma; HAL, Mercury Analytical Laboratory at Wisconsin State Laboratory of Hygiene, University of Wisconsin-Madison; DASNCU, Department of Atmospheric Sciences, National Central University (Taiwan); ERTC, Environmental Research and Training Center (Thailand); FRL, Flett Research, Ltd. (Canada); IVL, Swedish Environmental Research Institute; <, less than; NIMD, National Institute for Minamata Disease (Japan); NSA, North Shore Analytical, Inc.; WML(A/M), U.S. Geological Survey Mercury Research Laboratory (A, automated method/M, manual method); statistical warning limits are +2 overall f-psig, statistical control limits are +3 overall f-psig]
Results in table 11 indicate that during 2021–22, the HAL analytical variability was more than two times higher than the overall interlaboratory variability (f-psig ratios of 2.03 and 2.70) and greater than that of all other participating laboratories except the Environmental Research Training Center. The HAL results for 2021 indicated a negative analytical bias of −0.92 ng/L mercury with a significant sign test probability value of 0.012. For 2022, the variability for the HAL bias was positive (0.43 ng/L mercury) but not statistically significant (probability=0.115).
The results imply that the HAL analytical performance declined during 2021–22, characterized by high variability and bias compared to the other participating laboratories. However, the HAL participated in a performance testing program completed by Environment and Climate Change Canada, and the HAL results for this program were ranked very good according to the z-score (denotes the reported values position within the distribution of all reported values) in 2021 and 2022 (University of Wisconsin-Madison, Wisconsin State Laboratory of Hygiene, 2024). The HAL also performed well (102-percent recovery) in the 2021–22 USGS Standard Reference Sample Project (University of Wisconsin-Madison, Wisconsin State Laboratory of Hygiene, 2024). This information is provided for consideration of potential corrective actions for the HAL.
Analytical Results for Blank Samples
Interlaboratory-comparison results for the analyses of blank samples for mercury in 2021–22 are listed in table 12. Four blind blank samples were shipped to each laboratory annually. Method reporting limits differ among the laboratories, but they were less than or equal to the HAL detection limit of 0.20 ng/L during 2021. The HAL reported zero values greater than the detection limit of 0.20 ng/L for blank samples during 2021. The HAL detection limit decreased by a factor of 10 in 2022, and the HAL reported one value greater than detection (0.02 ng/L) during 2022. As determined for results in previous years, blank analyses indicate that mercury contamination at HAL during the study period was low (Wetherbee and Martin, 2016, 2020); therefore, most mercury contamination in MDN samples, which was estimated using the system blanks, was likely introduced in the field.
Table 12.
Number of total mercury determinations greater than the method detection limits for blank samples, and range of detection limits for each laboratory in the Mercury Deposition Network interlaboratory-comparison program, 2021–22.[Data are available in Wetherbee (2023). Four determinations were completed per year per laboratory. HAL, Mercury Analytical Laboratory, Wisconsin State Laboratory of Hygiene, University of Wisconsin-Madison; DASNCU, Department of Atmospheric Sciences, National Central University (Taiwan); ERTC, Environmental Research and Training Center (Thailand); FRL, Flett Research, Ltd. (Canada); IVL, Swedish Environmental Research Institute; NIMD, National Institute for Minamata Disease (Japan); NSA, North Shore Analytical, Inc.; WML(A/M), U.S. Geological Survey Mercury Research Laboratory (A, automated method/M, manual method); nd, no data; na, not applicable]
Data Quality Assessment
The biased hydrogen-ion concentration measurements in 2022 and the variability and bias in the total mercury concentration data should be taken into account by NADP data users for evaluation of spatial variation and temporal trends in atmospheric deposition. This bias in hydrogen-ion concentrations differs from the previous study period, where it was determined in 2019 that the hydrogen ion changed because of calibration changes, and was detected again in 2022. Calcium contamination, bias, and variability of NTN samples was seen again in 2022 (Wetherbee and others, 2023). However, the results presented herein should be considered in combination with information provided in NADP Quality Assurance Reports available on the NADP website (University of Wisconsin-Madison, Wisconsin State Laboratory of Hygiene, 2020a, b, c, 2021a, b, 2023). Other external analytical performance programs indicated generally acceptable performance by the CAL during the same period, specifically the Environment and Climate Change Canada laboratory performance studies, the USGS Standard Reference Sample Project, and the World Meteorological Organization performance testing program (University of Wisconsin-Madison, Wisconsin State Laboratory of Hygiene, 2024). However, these studies also indicated bias in the CAL data for pH (hydrogen ion), nitrate, and sulfate.
Summary
The U.S. Geological Survey Precipitation Chemistry Quality Assurance project implemented three programs to provide external quality-assurance monitoring for the National Atmospheric Deposition Program (NADP) National Trends Network (NTN) and two additional programs to provide external quality-assurance monitoring for the NADP Mercury Deposition Network (MDN) during 2021–22. The field audit program assessed the effects of sample-collection-site exposure, sample handling, and sample shipping protocols on the results of laboratory chemical analyses of NTN samples; the system blank program assessed the same effects for MDN samples. Two interlaboratory-comparison programs assessed the bias and variability of the chemical analysis data from the Central Analytical Laboratory (CAL), Mercury Analytical Laboratory (HAL; both at the Wisconsin State Laboratory of Hygiene, University of Wisconsin-Madison), and other participating laboratories that analyze precipitation samples for major ions, nutrients, and trace levels of mercury. The colocated sampler program was used to estimate overall variability of the NTN wet-deposition samples.
Interruptions in laboratory operations owing to the COVID-19 pandemic and changes in Precipitation Chemistry Quality Assurance sample preparation and shipping protocols during 2021 may have affected the results presented in this report, and those factors should be considered when using this information to evaluate the quality of NADP data for the NTN and MDN samples that were collected during 2021. The results indicate to data users to be aware of bias and variability of hydrogen ion (from pH) and total mercury values when evaluating spatial and temporal trends in the NADP data.
National Trends Network
Field audit results for 2021–22 indicate that network maximum contamination levels (NMCLs) for NTN samples remained similar to recent values for all analytes. Notable increases in the NMCLs for NTN from 2021 to 2022 were 0.009–0.020 milligram per liter (mg/L) for sulfate, 0.004–0.020 mg/L for nitrate, and 0.008–0.020 mg/L for chloride. Method detection limits were not determined for hydrogen-ion during this period. Variable levels of sample contamination over the past 10 years are small in terms of absolute concentrations; however, the 2020–22 NMCLs for sodium, ammonium, chloride, nitrogen, and hydrogen ion were greater than the 25th percentile concentrations, respectively, in NTN samples during the same period. The NMCLs for nitrate (0.083 mg/L) and sulfate (0.058 mg/L) were at the 10th and 3d percentiles for NADP measurements for these analytes, respectively. This program also estimated the maximum loss of ammonium, nitrate, and hydrogen ion in weekly NTN samples. Ammonium maximum loss increased from 0.019 mg/L (2019–21) to 0.021 mg/L (2020–22). Nitrate maximum loss was negligible at 0.002–0.003 mg/L, and hydrogen-ion maximum loss decreased from 4.6 to 4.1 microequivalents per liter (μeq/L) during the study period. Hydrogen-ion maximum loss decreased from 1.46 μeq/L during 2019–21 to 1.41 μeq/L during 2020–22. Ongoing changes in the environment and laboratory variations can account for these differences.
For samples collected and analyzed in 2021, statistically significant, positive bias was observed for CAL laboratory analyses for calcium, magnesium, sodium, potassium, nitrate, and hydrogen ion. For samples collected and analyzed in 2022, statistically significant bias was observed for CAL analyses for calcium, sodium, potassium, ammonium, chloride, sulfate, and hydrogen ion. The magnitudes of the biases were greater than the detection limits and of practical importance for nitrate and hydrogen ion in 2021 and for chloride, sulfate, and hydrogen ion in 2022. The CAL results generally indicated low variability relative to that of the other laboratories participating in the intercomparison program except for magnesium and nitrate in 2021 and hydrogen ion in 2021 and 2022. The Asia Center for Air Pollution Research (ACAP); Environment and Climate Change Canada, Science and Technology Branch; Illinois State Water Survey, Prairie Research Institute (PRI); Research Triangle International (RTI); and Wood Group, Inc. (WOOD), laboratories demonstrated generally low variability for all constituents except hydrogen ion (for ACAP and WOOD in 2021), ammonium (for PRI in 2021), and chloride (for PRI in 2021 and 2022).
The participating laboratories did not receive de-ionized water blanks for analysis in 2021, but they received four blanks in 2022, with the exception of the Ministry of the Environment and Climate Change (MOECC) and U.S. Department of Agriculture, Forest Service, Northern Research Service (NRS), which received three blank samples each, and Universidad Nacional Autónoma de México (UNAM), which received one sample because of shipping difficulties. The CAL, UNAM, and WOOD laboratories reported no analytical determinations greater than the method detection limits for blank samples in 2022, and few concentrations greater than analytical detection limits for blank samples were reported for the RTI and PRI laboratories during 2022. Note that the NILU; UNAM; RTI; Environment and Climate Change Canada, Science and Technology Branch; MOECC; and NRS laboratories use reporting limits that are unchanging from year to year instead of actual detection limits, which likely vary less than the listed reporting limits. Participation by all laboratories was limited during 2021 because of the COVID-19 pandemic. Laboratories that reported a moderately higher number of concentrations greater than their analytical detection limits were Cary Institute of Ecosystem Studies, NRS, MOECC, and ACAP.
Analyte determinations that exceeded statistical control limits (plus or minus [±] 3 f-pseudosigma [f-psig]) for CAL during 2021–22 include calcium (2), magnesium (5), chloride (4), sulfate (5), specific conductance (3), and hydrogen ion (10). The interlaboratory-comparison program results indicated comparatively greater numbers of analyses exceeding the control limits for the MOECC, NRS, NILU, Cary Institute of Ecosystem Studies, and UNAM laboratories.
Weekly wet-deposition data from the colocated sampler program indicated overall variability ranging from 1.5 to 12.2 percent for specific conductance, 10.3 to 17.2 percent for hydrogen-ion concentration, 0.8 to 20.2 percent for anions, 1.6 to 11.4 percent for ammonium and 0 to 30.3 percent for cations. These values refer to percentage differences at all four of the sites used in the study over the 2021–22 period, which included OK00 and 00OK and TX56 and 56TX in 2021, and in 2022 the study sites were NE99 and 99NE and OH09 and 09OH.
Mercury Deposition Network
The MDN NMCL for total mercury decreased from 0.926 nanogram per liter (ng/L) during 2018–20 to 0.758 ng/L during 2019–21 and then increased slightly to 0.790 ng/L during 2020–22. The maximum contamination in MDN samples during 2020–22 was not greater than 0.790 ng/L with 90-percent confidence, and no more than 10 percent of the MDN samples had contamination concentrations exceeding 0.790 ng/L with 90-percent confidence. This concentration is less than the first percentile of all MDN weekly total mercury concentrations measured during 2020–22 (1.65 ng/L). Median differences from the MPV were −0.92 and 0.43 ng/L for 2021 and 2022, respectively, which were also less than the first percentile of all weekly MDN total mercury concentrations. The HAL bias was statistically significant for 2021 but not 2022 results.
The HAL control chart for 2021–22 for the interlaboratory-comparison program indicates a negative bias of about 1 ng/L mercury for total mercury concentration analyses during 2021, trending to a positive bias of about 1 ng/L mercury during 2022. Six results were outside the ±3 f-psig control limits and ±2 f-psig warning limits during 2021 and five were out of the ±3 f-psig control limits and ±2 f-psig warning limits during 2022. In comparison with the participating laboratories, the HAL total mercury analyses were characterized by more variability than overall (all laboratories) during 2021 with an f-psig ratio of 2.03. The HAL results indicated variability about 270 percent higher than overall during 2022 with an f-psig ratio of 2.70. Participating laboratories analyzed four blank samples containing no mercury each year. The HAL reported no values greater than the detection limit of 0.20 ng/L during 2021 and one value greater than the detection limit of 0.02 ng/L during 2022 for blank samples.
References Cited
Brahney, J., Mahowald, N., Ward, D.S., Ballantyne, A.P., and Neff, J.C., 2015, Is atmospheric phosphorus pollution altering global alpine Lake stoichiometry?: Global Biogeochemical Cycles, v. 29, no. 9, p. 1369–1383, accessed January 24, 2023, at https://doi.org/10.1002/2015GB005137.
Latysh, N.E., and Wetherbee, G.A., 2005, External quality assurance programs managed by the U.S. Geological Survey in support of the National Atmospheric Deposition Program/National Trends Network: U.S. Geological Survey Open-File Report 2005–1024, 66 p., accessed January 31, 2018, at https://doi.org/10.3133/ofr20051024.
Latysh, N.E., and Wetherbee, G.A., 2007, External quality assurance programs managed by the U.S. Geological Survey in support of the National Atmospheric Deposition Program/Mercury Deposition Network: U.S. Geological Survey Open-File Report 2007–1170, 33 p., accessed January 24, 2023, at https://doi.org/10.3133/ofr20071170.
Lee, L., 2023, NADA v1.6–1.1: Quantargo software release, accessed November 12, 2023, at https://www.quantargo.com/help/r/latest/packages/NADA/1.6-1.1/plot-methods.
Mangiafico, S., 2023, rcompanion package for statistical functions, version 2.4.26: R Foundation for Statistical Computing software release, accessed August 2023 at https://cran.r-project.org/src/contrib/Archive/rcompanion/.
National Atmospheric Deposition Program [NADP], 2019, Network Operation Subcommittee (NOS) meeting minutes: NADP, 14 p., accessed May 22, 2024, at https://nadp.slh.wisc.edu/wp-content/uploads/2021/04/nos2019fall.pdf.
National Atmospheric Deposition Program [NADP], 2023a, MDN interactive map: NADP digital data, accessed November 13, 2023, at https://nadp.slh.wisc.edu/maps-data/mdn-interactive-map/.
National Atmospheric Deposition Program [NADP], 2023b, National Atmospheric Deposition Program National Trends Network (NTN) weekly metadata (ver. 2.1): NADP, 2 p., accessed July 21, 2023, at https://nadp.slh.wisc.edu/wp-content/uploads/ntn-weekly-metadata_v2_1.pdf.
National Atmospheric Deposition Program [NADP], 2023c, NTN site information: NADP digital data, accessed November 13, 2023, at https://nadp.slh.wisc.edu/maps-data/ntn-interactive-map/.
R Core Team, 2022, R—A language and environment for statistical computing, version 4.2.1: R Foundation for Statistical Computing software release, accessed September 26, 2022, at https://www.R-project.org/.
SAS Institute, Inc., 2016, SAS software, version 9.4: Cary, N.C., SAS Institute, Inc., software release, accessed January 26, 2023, at https://support.sas.com/software/94/.
University of Wisconsin-Madison, Wisconsin State Laboratory of Hygiene, 2019, National Trends Network site operations manual (ver. 3.0): National Atmospheric Deposition Program, 21 p., accessed July 20, 2023, at https://nadp.slh.wisc.edu/wp-content/uploads/2021/04/NTN-2000_Operations_Manual_v_3-0.pdf.
University of Wisconsin-Madison, Wisconsin State Laboratory of Hygiene, 2020a, Laboratory quality assurance plan: Madison, Wis., National Atmospheric Deposition Program, 78 p., accessed January 25, 2022, at https://nadp.slh.wisc.edu/wp-content/uploads/2021/04/QAPNADPLab2020.pdf.
University of Wisconsin-Madison, Wisconsin State Laboratory of Hygiene, 2020b, 2019 quality assurance report: Madison, Wis., National Atmospheric Deposition Program, 33 p., accessed January 25, 2022, at https://nadp.slh.wisc.edu/wp-content/uploads/2021/04/cal_2019_QAR.pdf.
University of Wisconsin-Madison, Wisconsin State Laboratory of Hygiene, 2020c, 2019 quality assurance report: Madison, Wis., National Atmospheric Deposition Program, 17 p., accessed January 25, 2022, at https://nadp.slh.wisc.edu/wp-content/uploads/2021/04/HAL_QAR_2019.pdf.
University of Wisconsin-Madison, Wisconsin State Laboratory of Hygiene, 2021a, 2020 quality assurance report: Madison, Wis., National Atmospheric Deposition Program, 47 p., accessed January 25, 2022, at https://nadp.slh.wisc.edu/wp-content/uploads/2021/10/cal_2020_QAR.pdf.
University of Wisconsin-Madison, Wisconsin State Laboratory of Hygiene, 2021b, 2020 quality assurance report: Madison, Wis., National Atmospheric Deposition Program, 26 p., accessed January 25, 2022, at https://nadp.slh.wisc.edu/wp-content/uploads/2021/10/hal_2020_QAR.pdf.
University of Wisconsin-Madison, Wisconsin State Laboratory of Hygiene, 2023, 2021 quality assurance report: Madison, Wis., National Atmospheric Deposition Program, 66 p, accessed February 20, 2024, at https://nadp.slh.wisc.edu/wp-content/uploads/2023/11/CAL_HAL_2021_QAR.pdf.
University of Wisconsin-Madison, Wisconsin State Laboratory of Hygiene, 2024, 2022 quality assurance report: Madison, Wis., National Atmospheric Deposition Program, 56 p, accessed June 13, 2024, at https://nadp.slh.wisc.edu/wp-content/uploads/2024/06/NAL_2022_QAR.pdf.
U.S. Environmental Protection Agency, 2002, Method 1631, revision E—Mercury in water by oxidation, purge and trap, and cold vapor atomic fluorescence spectrometry: Washington, D.C., U.S. Environmental Protection Agency, Office of Water, EPA–821–R–02–019, 38 p., accessed May 6, 2014, at https://www.epa.gov/sites/default/files/2015-08/documents/method_1631e_2002.pdf.
U.S. Geological Survey, 2002, Processing of water samples (ver. 2, April 2002; edited by Wilde, F.D.): U.S. Geological Survey Techniques of Water-Resources Investigations, book 9, chap. A5, 90 p., accessed April 15, 2020, at https://doi.org/10.3133/twri09A5.
Wetherbee, G.A., 2020a, Data for the U.S. Geological Survey Precipitation Chemistry Quality Assurance Project for the National Atmospheric Deposition Program, 1978–2017: U.S. Geological Survey data release, accessed January 10, 2022, at https://doi.org/10.5066/P94RC4GD.
Wetherbee, G.A., 2020b, U.S. Geological Survey Precipitation Chemistry Quality Assurance Project data 2017–2018: U.S. Geological Survey data release, accessed May 1, 2021, at https://doi.org/10.5066/P9ZKXD8N.
Wetherbee, G.A., 2022, U.S. Geological Survey Precipitation Chemistry Quality Assurance Project data 2019–2020: U.S. Geological Survey data release, accessed December 1, 2022, at https://doi.org/10.5066/P9B119QP.
Wetherbee, G.A., 2023, U.S. Geological Survey Precipitation Chemistry Quality Assurance Project data 2021–2022: U.S. Geological Survey data release, https://doi.org/10.5066/P9OZ5GL1.
Wetherbee, G.A., Latysh, N.E., Greene, S.M., and Chesney, T.A., 2010, U.S. Geological Survey external quality-assurance project report to the National Atmospheric Deposition Program/National Trends Network and Mercury Deposition Network, 2007–08: Champaign, Ill., National Atmospheric Deposition Program Quality Assurance Report 2010–01 and Illinois State Water Survey Miscellaneous Publication 190, 82 p., accessed April 15, 2020, at https://www.isws.illinois.edu/pubdoc/MP/ISWSMP-190.pdf.
Wetherbee, G.A., and Martin, R., 2016, External quality assurance project report for the National Atmospheric Deposition Program’s National Trends Network and Mercury Deposition Network, 2013–14: U.S. Geological Survey Scientific Investigations Report 2016–5069, 22 p., accessed April 15, 2020, at https://doi.org/10.3133/sir20165069.
Wetherbee, G.A., and Martin, R., 2017, Updated operational protocols for the U.S. Geological Survey Precipitation Chemistry Quality Assurance Project in support of the National Atmospheric Deposition Program: U.S. Geological Survey Open-File Report 2016–1213, 18 p., accessed January 31, 2018, at https://doi.org/10.3133/ofr20161213.
Wetherbee, G.A., and Martin, R., 2020, External quality assurance project report for the National Atmospheric Deposition Program’s National Trends Network and Mercury Deposition Network, 2017–18: U.S. Geological Survey Scientific Investigations Report 2020–5084, 31 p., accessed August 5, 2021, at https://doi.org/10.3133/sir20205084.
Wetherbee, G.A., Martin, R., and Liethen, A., 2023, External quality-assurance project report for the National Atmospheric Deposition Program’s National Trends Network and Mercury Deposition Network, 2019–20: U.S. Geological Survey Scientific Investigations Report 2023–5045, 29 p., accessed July 21, 2023, at https://doi.org/10.3133/sir20235045.
Wetherbee, G.A., Martin, R., Rhodes, M.F., and Chesney, T.A., 2014, External quality-assurance project report for the National Atmospheric Deposition Program/National Trends Network and Mercury Deposition Network for 2009–2010: U.S. Geological Survey Scientific Investigations Report 2013–5147, 53 p., accessed April 15, 2020, at https://doi.org/10.3133/sir20135147.
Conversion Factors
International System of Units to U.S. customary units
Supplemental Information
Specific conductance is given in microsiemens per centimeter at 25 degrees Celsius (µS/cm at 25 °C).
Concentrations of chemical constituents in water are given in either milligrams per liter (mg/L), microequivalents per liter (µeq/L), or nanograms per liter (ng/L).
100(p)th is the percentile equal to 100 times a value of p, for example, 100×(0.9)=90th percentile.
Absolute value of x=|x|, where x takes the form of numerical values or algebraic expressions.
Study period refers to calendar years 2021–22.
Abbreviations
α
maximum probability of incorrect rejection of the null hypothesis.
ACAP
Asia Center for Air Pollution Research
CAL
Central Analytical Laboratory [Wisconsin State Laboratory of Hygiene, University of Wisconsin-Madison]
CIES
Cary Institute of Ecosystem Studies
ECST
Environment and Climate Change Canada (formerly [2015–16] Environment Canada), Science and Technology Branch
f-psig
f-pseudosigma
HAL
Mercury Analytical Laboratory [Wisconsin State Laboratory of Hygiene, University of Wisconsin-Madison]
MDL
method detection limit
MDN
Mercury Deposition Network
MOECC
Ministry of the Environment and Climate Change
MPV
most probable value
NADP
National Atmospheric Deposition Program
N-CON
N-CON Systems Company, Inc.
NILU
Norwegian Institute for Air Research
NMCL
network maximum contamination level
NRS
U.S. Department of Agriculture, Forest Service, Northern Research Station
NTN
National Trends Network
PCQA
Precipitation Chemistry Quality Assurance project
PRI
Illinois State Water Survey, Prairie Research Institute
RTI
Research Triangle Institute, International
SRS
Standard Reference Sample
UCL
upper confidence limit
UNAM
Universidad Nacional Autónoma de México
USGS
U.S. Geological Survey
WOOD
Wood Group, Inc.
WSLH
Wisconsin State Laboratory of Hygiene [University of Wisconsin-Madison]
±
plus or minus
For more information about this publication, contact:
Director, USGS Water Resources Mission Area Headquarters
12201 Sunrise Valley Drive
Reston, VA 20192
For additional information, visit: https://www.usgs.gov/mission-areas/water-resources
Publishing support provided by the
Pembroke and Rolla Publishing Service Centers
Disclaimers
Any use of trade, firm, or product names is for descriptive purposes only and does not imply endorsement by the U.S. Government.
Although this information product, for the most part, is in the public domain, it also may contain copyrighted materials as noted in the text. Permission to reproduce copyrighted items must be secured from the copyright owner.
Suggested Citation
Deyette, N.A., Wetherbee, G.A., and Martin, R., 2024, External quality-assurance project report for the National Atmospheric Deposition Program National Trends Network and Mercury Deposition Network, 2021–22: U.S. Geological Survey Scientific Investigations Report 2024–5054, 34 p., https://doi.org/10.3133/sir20245054.
ISSN: 2328-0328 (online)
Publication type | Report |
---|---|
Publication Subtype | USGS Numbered Series |
Title | External quality-assurance project report for the National Atmospheric Deposition Program National Trends Network and Mercury Deposition Network, 2021–22 |
Series title | Scientific Investigations Report |
Series number | 2024-5054 |
DOI | 10.3133/sir20245054 |
Year Published | 2024 |
Language | English |
Publisher | U.S. Geological Survey |
Publisher location | Reston, VA |
Contributing office(s) | WMA - Observing Systems Division |
Description | Report: viii, 34 p.; 4 Data Releases |
Online Only (Y/N) | Y |
Additional Online Files (Y/N) | N |
Google Analytic Metrics | Metrics page |