Evaluation and Review of Ecology-Focused Stream Studies to Support Cooperative Monitoring, Fountain Creek Basin, Colorado
Links
- Document: Report (2.22 MB pdf) , HTML , XML
- Data Release: USGS data release - Datasets for Evaluation and Review of Ecology-Focused Stream Studies, Fountain Creek Basin, Colorado
- Download citation as: RIS | Dublin Core
Acknowledgments
The authors thank Colorado Springs Utilities and Colorado Springs Stormwater Enterprise for their continued support as well as other cooperators who supported earlier projects. Gary Dowler and the numerous technicians of Colorado Parks and Wildlife were instrumental to earlier fish-sampling efforts. Numerous U.S. Geological Survey (USGS) staff joined ecology field efforts each year as well as collected, compiled, and analyzed streamflow, water quality, and geomorphic data. The authors thank James Roberts (USGS) and Mike Meador (emeritus USGS) for providing thoughtful reviews and comments that improved earlier versions of this manuscript. The authors also thank the countless USGS hydrological technicians who collected water quality data and those who collected, compiled, and analyzed streamflow data used in this report.
Abstract
The U.S. Geological Survey, in cooperation with Colorado Springs Utilities and Colorado Springs Stormwater Enterprise, synthesized previous studies and evaluated recent monitoring data to understand the distribution of fish and invertebrates in the Fountain Creek Basin and documented response to streamflow, water temperature, and water quality. The goal was to identify opportunities for aligning data collection to help maximize information gained from additional monitoring. Fifty-two publications were compiled from the literature that were completed within the study area between 1964 and 2022. Of these publications, 19 were fish and invertebrate focused. Overall, the distribution of fish and invertebrates in the Fountain Creek Basin has changed since the early 1900s. The occurrence of several fish species has increased or decreased since 2003, and a few species have not been collected in more than 100 years. Several mayfly, stonefly, and caddisfly taxa once common at several locations before 2000 are now rarely encountered, and those that now occur more frequently are associated with warmer-water streams. Decreasing invertebrate multimetric index values were noted at six locations, and the invasive Potamopyrgus antipodarum (New Zealand mud snail) is now established at two locations and occurs at several others, but in low numbers. Various streamflow characteristics were frequently noted to affect spatial and temporal patterns in fish and invertebrate communities, including early development and recruitment of Platygobio gracilis (flathead chub). Water quality and temperature contributed to patterns in aquatic communities, but less is known about the direct effects as these data were inconsistently available. Reach-scale habitat also contributed to patterns in aquatic communities, especially measures associated with the streambank, stream channel, and composition of streambed substrate. Moving forward, aligning consistent streamflow, water temperature, water quality, and geomorphic data collection at fish-, invertebrate-, and habitat-monitoring locations could maximize information gained from monitoring efforts and potentially inform evolving management activities and interests within the basin.
Introduction
Several natural environmental factors (for example, streamflow and water quality) that affect aquatic communities have been altered throughout the Fountain Creek Basin as human population has grown, beginning as early as the mid-1800s (Zuellig, Bruce, and others, 2007). Cooperative studies by the U.S. Geological Survey (USGS) and local stakeholders began in the mid-1980s (von Guerard, 1989a, b) to understand how aquatic communities are affected by these environmental conditions. Since then, concurrent USGS cooperator-funded studies provided results to help understand temporal trends and (or) spatial patterns in surface-water hydrology (Mau and others, 2007), water quality (Bern and others, 2024), fluvial geomorphology (Hempel and others, 2021), and ecology (Zuellig, Bruce, and others, 2007; Zuellig and others, 2010; Roberts and others, 2018). Additionally, related studies completed by stakeholders (Sanderson and others, 2012), State agencies (Nesler and others, 1999), and university researchers (Haworth and Bestgen, 2016; Herrmann and others, 2016) have contributed to a better understanding of the ecology of the Fountain Creek Basin. Human populations are expected to increase in the basin in the future (Department of Local Affairs, 2023), which could further alter environmental conditions that affect aquatic communities.
Historically, cooperative surface water hydrology, water quality, fluvial geomorphology, and ecology studies in the Fountain Creek Basin were often constrained by water quality permits issued by the Colorado Department of Health and Environment (CDPHE) or other governing agencies. A distinct set of requirements and timelines associated with each permit were independently negotiated between CDPHE and each regulated entity. This structure has led to differences in what was being monitored at which locations through time (Zuellig and others, 2010; Roberts and others, 2018), which, in turn, has affected collective knowledge that may have been gained from maintaining consistent study sites and sampled constituents (Zuellig and others, 2010). Similar efforts in other basins have been termed the “data-rich but information-poor” monitoring syndrome (Ward and others, 1986). Nonetheless, ongoing permit-focused monitoring in the Fountain Creek Basin, including the efforts of other researchers and entities, has produced pertinent information, which could help align additional data collection efforts that better integrate studies of streamflow, water quality, and aquatic life to help support water resource management activities.
Purpose and Scope
The purpose of this report is to synthesize previous studies and evaluate monitoring data to understand the distribution of fish and invertebrates and the ecological response to streamflow, water temperature, and water quality within the Fountain Creek Basin, and to help identify opportunities for aligning data collection that could maximize information gained from additional cooperative studies. The report focuses on USGS ecology-based studies in the Fountain Creek Basin performed between the mid-1980s and 2022; selected studies carried out by other entities were also included. To help guide additional monitoring, selected ecological datasets from ongoing monitoring in the basin were evaluated to further support previous results or to potentially fill selected information gaps in the previously published studies.
Study Area Description
The study area was mostly encompassed by the Fountain Creek Basin, which was described in detail by Edelmann and others, 2002; Mau and others, 2007; and Zuellig, Bruce, and others, 2007, and consists of sites on the Arkansas River just upstream and downstream from the confluence with Fountain Creek (fig. 1, table 1). In general, the Fountain Creek Basin drains approximately 926 square miles (mi2) of the eastern slope of the Rocky Mountains in south-central Colorado and confluences with the Arkansas River near Pueblo, Colorado (fig. 1).
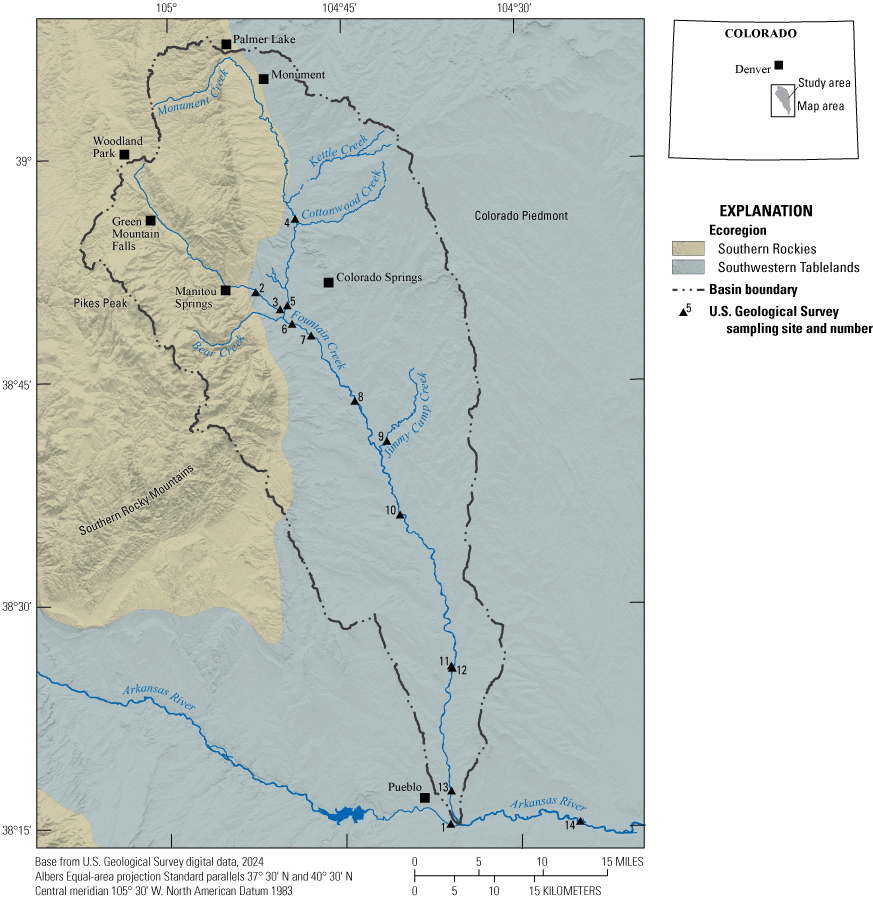
Selected U.S. Geological Survey sampling sites in the Fountain Creek Basin study area, Colorado, with ecological records for 1984–2022. Ecoregions defined in Omernik (1987).
Table 1.
Description of selected U.S. Geological Survey sampling sites in the Fountain Creek Basin study area, Colorado, with intermittent ecological records for 1984–2022.[USGS station numbers and names are from the USGS National Water Information System database (USGS, 2023). USGS, U.S. Geological Survey; NAVD 88, North American Vertical Datum of 1988; ft, feet; mi2, square miles; NA, data unavailable for drainage area]
Within the study area, elevation ranges from 4,509 feet (ft) at the lowest site on the Arkansas River to 14,109 ft at the summit of Pikes Peak. Fountain Creek and Monument Creek are the two main drainages located in the transition of two distinctive physiographic regions: the Southern Rocky Mountains and the Colorado Piedmont (Hansen and others, 1982). These landforms correspond to two ecoregions, the Southern Rockies and the Southwestern Tablelands (Omernik, 1987). The primary area of interest for this report consists of the transitional area between these main landforms, and ecoregions in the Fountain Creek Basin to just below the confluence of Fountain Creek and the Arkansas River (fig. 1).
In general, historical records documenting observations of early explorers from the 1840s indicate that several changes occurred to physical attributes of regional streams (Fausch and Bestgen, 1997). For example, plains streams, such as in the Fountain Creek Basin near the eastern edge of the Front Range of the southern Rocky Mountains, that flowed clear over cobble-gravel streambeds now consist of much finer particles with noticeable turbidity (Fausch and Bestgen, 1997). Farther east on the plains, sinuosity and pool frequency increased as riparian areas were encroached by woody vegetation in response to reductions in overbank flooding after the advent of irrigated agriculture in the mid-1800s (Fausch and Bestgen, 1997). Streamflow alteration evolved with the construction of small ditches (1840s) followed by large canals (1860–85), large main-stem reservoirs (1885–1940s), and more recently (1940s to the early 21st century) additional reservoirs, transmountain water diversions, and wastewater-treatment facility discharge (Fausch and Bestgen, 1997; Eschner and others, 1983). Changes in water quality likely also occurred concurrently with the characteristics described previously (streamflow alteration, in-stream habitat) as the landscape changed due to regional mining, industry, and pressures from urbanization. These early changes in hydrology, water quality, and the physical template of regional streams, including the Fountain Creek Basin, shaped the present-day biota, which are markedly different from those documented in historical records (Fausch and Bestgen, 1997).
Methods
Previously published studies focusing on fish and stream invertebrate communities in the study area were compiled and synthesized regarding what is known about the ecology of the Fountain Creek Basin streams and to identify possible opportunities for better aligning data collection to inform future management activities (app. 1). Studies focusing on ecological response associated with changes in environmental conditions were given the most attention compared to studies focusing on sampling methods or fish and invertebrate distribution. Topics explored were fish and invertebrate community response to streamflow, water temperature, water quality, and habitat. Selected findings within these topics were expanded where possible with augmented evaluation of more recently published data to potentially bolster results or fill data gaps of previous studies. Details of methods used for the supplemental evaluations are described in the subsequent Methods sections. Data used for supplemental analyses were selected from the 14 sites currently (2023) monitored within the Fountain Creek Basin study area variably sampled between 1985 and 2022. In general, samples were collected for three consecutive years in the 1980s and then annually since 1998. Ecological data collected after 2002 are provided by Zuellig and others (2022), and those collected before 2002 are provided by supporting publications (von Guerard, 1989a; Bruce, 2002). Refer to table 1 and figure 1 herein for site descriptions and locations. Data used for supplementary evaluation herein are provided by Wahl and others (2024).
Describing Changes in Fish and Invertebrate Communities
Changes in aquatic communities were documented by calculating the percent frequency of occurrence of selected fish species or invertebrate taxa based on observational patterns in the sampling data. Percent frequency of occurrence was calculated by compiling presence-absence data sorted into groups of 3 years for each site, where the number of times a species or taxon occurred was calculated as the percentage of years it was present within a group. Data indicating percent frequency of occurrence were then examined for changes at a site, in which a species or taxon showed obvious downward or upward patterns through time were retained for presentation and discussion. Fish data consisted of samples collected between 2003 and 2022, and invertebrate data consisted of samples collected between 1985 and 2022, dependent on site.
Additionally, invertebrate multimetric index (MMI) values were calculated for each site following Jessup and Stribling (2017) and plotted against year to detect obvious decreasing or increasing patterns in MMI values through time. In general, MMI values are calculated by selecting metrics that best discriminate between predefined reference and disturbed sites within a given basin, region, ecoregion, or other spatial stratification. The final set of metrics typically represents various aspects of community composition, richness, tolerance, abundance, function, and life history or other trait-type characteristics. Once a final set of metrics is selected, values are scored on a continuous scale, summed, and then rescaled to range between 0 and 100. The invertebrate MMI used herein was developed for the CDPHE by Tetra Tech, Inc., to evaluate the biological condition of streams in Colorado (Jessup and Stribling, 2017). Calculation of MMI values was automated using a tool packaged in a Microsoft Access database as the Ecological Data Application System (EDAS) for Colorado (Jessup and Stribling, 2017). A working version of EDAS was unavailable at the time of this publication, so MMI values were calculated by CDPHE staff in June 2023 using selected invertebrate data compiled from Zuellig and others (2022). Refer to Jessup and Stribling (2017), Zuellig and others (2014), and Bruce and others (2018) for details regarding the EDAS MMI for Colorado. After MMI values were calculated, values were plotted against year, and a loess smooth line (span=0.90) and 90-percent confidence intervals were added using the ggplot2 package (Wickham and others, 2023) in R (R Core Team, 2023) to aid interpretation. Data at sampling sites that showed obvious changes in MMI values were retained for presentation and discussion.
Corroborating the Association Between Streamflow and Age-Zero Flathead Chub
Haworth and Bestgen (2017) proposed that growth and survival of age-zero (young-of-the-year) flathead chub were greatly reduced in the Fountain Creek Basin during years when daily streamflow exceeded 20 cubic meters per second (706.29 cubic feet per second). Roberts and others (2018) used this information to suggest catch per unit effort of age-zero flathead chub consistently decreased in years with more than 25 days between May and August having streamflows at or greater than the threshold suggested by Haworth and Bestgen (2017) (fig. 6 in Roberts and others, 2018). To substantiate the proposed thresholds of these two studies, streamflow of 706.29 cubic feet per second was determined at site 10 as the 98th percentile of the average daily streamflow from 2003 to 2022. The 98th-percentile streamflow value was then calculated at site 8, which was 618.95 cubic feet per second. For each year at these two USGS streamgages (table 1; USGS, 2023), the number of daily maximum streamflow occurrences (hereafter referred to as “peak streamflow days”) were summed from May through August and represent the number of peak streamflow days above the threshold each year. The results were then plotted with the data used to create figure 6 of Roberts and others (2018) and evaluated for continuity.
Exploring Changes in Invertebrate Community Tolerance to Water Temperature
Compliance with State-regulated water temperature standards has become an area of concern among wastewater treatment facilities within the Fountain Creek Basin (Annie Berlemann, Colorado Springs Utilities, oral commun., 2023). Ideally, continuous water temperature data paired with ecological samples during the period of record of available ecological samples would be used to evaluate an ecological response to changes in water temperature. However, very little continuous water temperature data were available at ecological monitoring sites in the basin in 2023 (USGS, 2023). Therefore, a surrogate measure of ecological response to water temperature was necessary to evaluate if invertebrate community tolerance to water temperatures changed through time. Carlisle and others (2007) derived taxa-specific tolerance indicator values (TIVs) for water temperature from a nationwide dataset; values were estimated from abundance-weighted averages of each taxon in relation to concurrently collected water temperature data. Those values could then be tested with independent data to confirm the applicability of the estimated TIVs and to discern specific stressors (Yuan, 2004; Carlisle and others, 2007; Meador and others, 2008). Herein, average community tolerance to water temperature was estimated by applying taxa-specific temperature TIVs of Carlisle and others (2007) to invertebrate samples collected within the Fountain Creek Basin between 2005 and 2022 (Zuellig and others, 2022). Increasing TIVs indicate invertebrate communities are becoming more tolerant to warmer water temperatures through time, whereas decreasing TIVs indicate they are becoming less tolerant to warming temperatures. After values were calculated, average TIVs for each sample were plotted against year for each sampling site to detect obvious decreasing or increasing patterns in community tolerance through time. A loess smooth line (span=0.90) and 90-percent confidence intervals were added using the ggplot2 package (Wickham and others, 2023) in R (R Core Team, 2023) to aid interpretation. Data at sites that showed obvious changes in water temperature TIV values were retained for presentation and discussion.
Exploring the Association Between Multimetric Index Values and Documented Trends in Water Quality
Bern and others (2024) examined trends in available water quality data from 1999 to 2022 at eight sites in the Fountain Creek Basin that overlapped with sites included herein (table 1). Trends reported by Bern and others (2024) as highly likely related to ammonia, nitrate plus nitrite, unfiltered phosphorus, orthophosphate, and chloride were selected to determine if there were corresponding patterns in changes in MMI values at overlapping sites. Highly likely trends were those having the greatest statistical likelihood that they occurred in the stated direction as indicated by a two-sided attained p-value (Hirsch and others, 2015) ranging between 0.95 to 1.0. In general, increases in concentration in the selected constituents could result in decreases in MMI values if the changes in concentration were great enough to be biologically relevant. Sites with decreasing MMI values that corresponded to highly likely trends in the selected water quality data reported by Bern and others (2024) were included for discussion.
Supplemental Evaluation and Review of Ecology-Focused Stream Studies
A total of 52 publications were compiled from the literature and grouped into one of four general groups consisting of ecology, geomorphology, streamflow, and water quality (app. 1). In general, very little published information exists regarding the Fountain Creek Basin before 1980, which was well after many physical changes had taken place beginning as early as the 1840s with the implementation of irrigated agriculture (Fausch and Bestgen, 1997; Eschner and others, 1983). Except for a single groundwater study (Jenkins and Glover, 1964) and a few early museum records of fish and invertebrates mentioned in later publications (Fausch and Bestgen, 1997), the number of published studies did not increase until a decade after the passage of the Clean Water Act of 1972 (33 U.S.C. 1251 et seq.). Since then, the number of ecology, streamflow, and water quality-focused publications in the Fountain Creek Basin has steadily increased, whereas geomorphology-focused publications began more recently (since 2018; fig. 2).
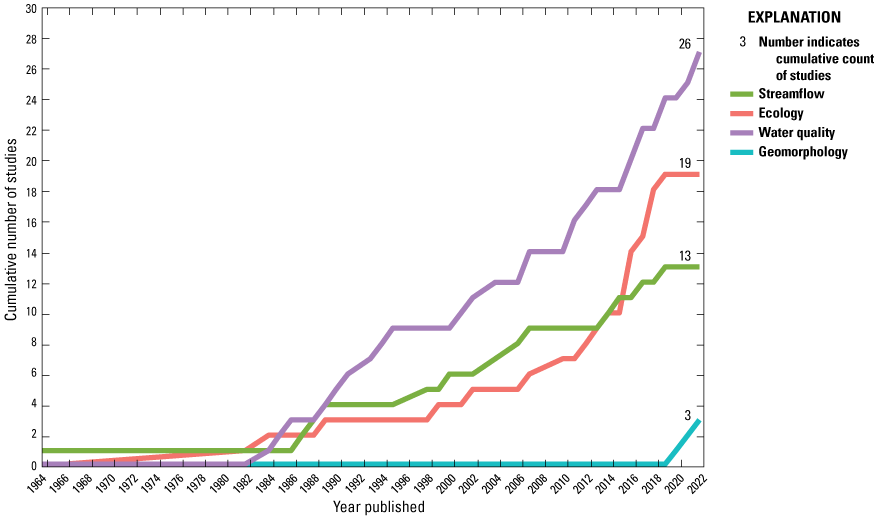
Cumulative distribution of streamflow, ecology, water quality, and geomorphology studies within the Fountain Creek Basin study area, Colorado, resulting from a general Google Scholar literature search, September 2023.
The sections “Understanding the Fish Assemblage” and “Understanding the Invertebrate Assemblage” summarize the distribution of fish and invertebrates in the Fountain Creek Basin relative to historical records. The remaining sections (“Fish and Invertebrate Response to Streamflow,” “Fish and Invertebrate Response to Water Temperature,” “Fish and Invertebrate Response to Selected Water Quality,” and “Fish and Invertebrate Response to Habitat”) provide information about the ecological response to various environmental conditions in the Fountain Creek Basin. Where possible, previously published findings were augmented with more recently published data to expand the scope of previous analyses, allowing for new inferences related to assemblage change and response.
Understanding the Fish Assemblage
Fausch and Bestgen (1997) provide a thorough history of early collections of plains stream fishes in Colorado. Before 1900, fish were collected from only seven sites within the Arkansas River Basin near the cities of Cañon City, Colorado Springs, and Pueblo (Fausch and Bestgen, 1997). Basinwide fish surveys of the Arkansas River Basin, including Fountain Creek, did not occur until after 1958 (Fausch and Bestgen, 1997). Two additional plains-fish surveys of the Arkansas River Basin were completed by the Colorado Division of Wildlife (now Colorado Parks and Wildlife) in the 1980s and 1990s (Loeffler and others, 1982; Nesler and others, 1999). Since 2008, Colorado Parks and Wildlife has extensively sampled fish in the basin, although no formal document reporting findings of this ongoing effort was available at the time of this publication. However, data associated with this more recent sampling effort are available, as is historical information, by making a special request to Colorado Parks and Wildlife.
It is difficult to determine exactly how current (2023) patterns of fish distribution compare to historical patterns, because the distribution of fishes in the Arkansas River Basin and Fountain Creek was not documented until well after major changes in hydrology, stream water quality, and habitat had already occurred. However, Fausch and Bestgen (1997) used literature collection records, museum records, and unpublished data to estimate that there were possibly 20 fish species native to the Arkansas River Basin in Colorado (refer to table 6.1 in Fausch and Bestgen, 1997, for details). Of these 20 species, three (Macrhybopsis aestivalis, speckled chub; Hybognathus placitus, plains minnow; and Carpiodes carpio, river carpsucker) have not been collected in more than 100 years; one (Notropis girardi, Arkansas river shiner) was presumed native to the Arkansas River Basin because of its occurrence in the Cimmaron River upstream and downstream from Colorado in New Mexico and Kansas (Fausch and Bestgen, 1997). Of these four species, plains minnow was collected in 1913 from the Arkansas River at Cañon City upstream from the Fountain Creek confluence and at Pueblo in 1889; speckled chub was collected in 1889 from the Arkansas River at Pueblo, suggesting that they occurred within the study area. Zuellig and others (2007) indicated that plains minnow and speckled chub were likely associated with downstream reaches of the Arkansas River and not Fountain Creek but were unaware of the additional nearby historical records, so they are included herein as historical occupants of the study area. Fausch and Bestgen (1997) also included Oncorhynchus clarkii stomias (greenback cutthroat trout) as native to the Arkansas River Basin, but this was later corrected with advanced genetic analysis by Metcalf and others (2007, 2012)67, who determined Oncorhynchus clarkii macdonaldi (yellowfin cutthroat trout) was the only salmonid native to the Arkansas River Basin.
As many as 18 native species may have historically occurred within the study area based on historical records. However, the most recent published plains-fish inventories of the Arkansas River Basin indicate nearly half of the native species are missing. For example, Loeffler and others (1982) reported 11 native species captured from the Fountain Creek Basin whereas Nesler and others (1999) reported 9. Roberts and others (2018) reported 23 species, of which 11 were considered native to the study area. Note that the results of Roberts and others (2018) were not based on inventory data as were the results of the other two studies (Loeffler and others, 1982; Nesler and others, 1999), but rather from routine monitoring that occurred between 2003 and 2016. Nonetheless, the fish fauna of Fountain Creek is markedly different from its original condition, because more than half of the 23 species reported by Zuellig and others (2022) are considered nonnative (Loeffler and others, 1982; Fausch and Bestgen, 1997; Nesler and others, 1999; Roberts and others, 2018) and because of the extirpation of several species that occurred historically.
Of the native species reported from the Fountain Creek Basin in 2023, three are State listed and occur east of the Front Range of the southern Rocky Mountains: Chrosomus erythrogaster (southern redbelly dace, State endangered), Etheostoma cragini (Arkansas darter, State threatened), and flathead chub (State species of concern); one (yellowfin cutthroat trout) has been considered extinct since 1904 (Wiltzius, 1985) but possibly historically occurred in the upper Fountain Creek Basin based on figure 4 in Metcalf and others (2012) and figure 1 in Bestgen and others (2019). Additionally, greenback cutthroat trout occurs in Bear Creek in the upper Fountain Creek Basin. Although it is not native to the Arkansas River Basin, the Bear Creek population has played a major role in the recovery of greenback cutthroat trout, which is currently (2023) State and federally listed as threatened (Metcalf and others, 2012).
It is apparent that fish distribution has changed, especially with the introduction of nonnative species compared to historical patterns, with several examples of site-specific changes in fish distribution occurring more recently (Zuellig and others, 2022). The following discussions in this paragraph represent selected examples in which a fish species either became more common or less common at a site through time. For example, despite annual sampling since 2003, Notropis stramineus (sand shiner) has not been observed at sites 5, 6, and 10 since 2005, 2003, and 2017, respectively, whereas Lepomis cyanellus (green sunfish) appears to be more common at sites 5 and 9 since 2015 and 2010 (table 2). Fundulus zebrinus (plains killifish) is a habitat specialist that prefers slow-moving backwaters and side channels (Cashner and others, 2020) and has not been collected at sites 9 and 10 since 2009 and 2012 (table 2). Cyprinella lutrensis (red shiner), Rhinichthys cataractae (longnose dace), and Arkansas darter occur at other locations but have been less frequently detected at a few sites where they were previously found (table 2). Additionally, occurrence of the State-listed flathead chub has become more common or less common through time depending on the site (table 2). Finally, nonnative Semotilus atromaculatus (creek chub) and Catostomus catostomus (longnose sucker) have occurred less frequently at sites 10 and 12, and the occurrence of Gambusia affinis (western mosquitofish) has apparently increased at site 12 (table 2).
Table 2.
Frequency of selected fish species occurring through time in collections made at locations within the Fountain Creek Basin, 2003–22.[The values represent the percentage of years, within each group of sampling years, where species were collected during sampling. Date ranges of sampling years include all years in the range. USGS station numbers and names are from the USGS National Water Information System database (USGS, 2023). Data sourced from Zuellig and others (2022). USGS, U.S. Geological Survey; --, data not collected]
Understanding the Invertebrate Assemblage
Little is known about the historical distribution of aquatic invertebrate species in streams of the eastern plains of Colorado. Only a few sporadic early collections of aquatic invertebrates from the region were made, and most of those are from the South Platte River Basin, where several species have been locally extirpated (Edmunds and McCafferty, 1984; McCafferty and others, 1993; Cordeiro, 1999; Kondratieff and Baumann, 2002; Zuellig and others, 2012). Since these early collections, there have been few organized field efforts to document species distribution of stream invertebrates from the plains part of the Arkansas River and Fountain Creek Basins. This type of effort requires compiling museum and literature records in addition to collecting new specimens often using specialized methods that differ from the typical benthic invertebrate sampling used in biomonitoring studies. This, combined with sparse historical information, makes it difficult to fully understand the likely extent of changes in species distribution relative to historical patterns before the human-induced changes that occurred in hydrology and habitat (Kondratieff and Baumann, 2002; Zuellig, Sprague, and others, 2007; Zuellig and others, 2012).
Although it is difficult to determine exact changes in the distribution of invertebrates relative to historical patterns, there is evidence that the occurrence of some invertebrate taxa has changed, or in some cases, invertebrate taxa may have been eliminated from stream locations within the Fountain Creek Basin (von Guerard, 1989a; Zuellig and others, 2022). The following discussion in this paragraph represents selected examples in which invertebrate taxa have either become more common, less common, or have been apparently eliminated. Apparent elimination of multiple invertebrate taxa in the Fountain Creek Basin occurred between the late 1990s and early- to mid-2000s, especially at upstream monitoring sites (table 3). For example, species associated with the mayfly taxa Drunella, Ephemerella, and Rhithrogena; the stonefly taxa Capniidae, Prostoia, Pteronarcella, Sweltsa, and Isoperla; and the caddisfly taxa Arctopsyche and Rhyacophila were frequently collected in various combinations at several sites (sites 2, 3, 4, 6, and 7) between 1985 and 1999 but were rarely encountered after 2000 (table 3). These results represent a substantial change in community structure, especially at the two most upstream locations (sites 2 and 3). These results are likely biased low as there is more than one species associated with each listed taxon that was possibly eliminated at these locations (Ward and others, 2002; Zuellig and others, 2012). Similar changes in invertebrate community structure have occurred in other transition-zone streams along the Front Range of the Rocky Mountains in Colorado that have been pressured by urbanization and water management (Zuellig, Sprague, and others, 2007; Zuellig and others, 2010). Theoretically, these taxa could return if local conditions improved, assuming they are still present at upstream locations. Further investigation could be beneficial in determining if these taxa still occur upstream near the affected sites and better understanding the possibility of their return under an effort to improve current (2023) environmental conditions.
Table 3.
Frequency of selected invertebrate taxa occurring through time in collections made at locations within the Fountain Creek Basin, Colorado, 1985–2022.[The values represent the percentage of years, within each group of sampling years, when taxa were collected during sampling. Date ranges of sampling years include all years in the range. USGS station numbers and names are from the USGS National Water Information System database (USGS, 2023). USGS, U.S. Geological Survey; --, data not collected]
Taxon associated with warmer water temperatures and plains stream habitat based on Zuellig and others (2012).
Taxon associated with cooler water temperatures and foothills stream habitat based on Zuellig and others (2012).
Although several taxa have been apparently eliminated from upstream locations, there are several taxa that have been detected more frequently. For example, various combinations of the mayfly taxa Acentrella, Fallceon, Paracloeodes, or Heptagenia and caddisfly taxa Cheumatopsyche have been occurring more frequently at 10 (table 3) of the 14 sampling sites (fig. 1, table 1). Historically, these taxa occurred in the transition zone between the mountains and plains but are mostly associated with locations farther out on the eastern plains of Colorado (Zuellig and others, 2012), where water temperatures are warmer, coarse substrate is often highly embedded or absent, and the presence of woody debris is more frequent than at sites closer to the plains-mountain interface. Additionally, several of these taxa are tolerant to disturbance and harsh conditions, as they frequently occur in other urbanized areas of transition-zone streams along the Front Range of the Rocky Mountains in Colorado (Sprague and others, 2006; Zuellig and others, 2012).
Occurrence of New Zealand Mud Snail
Potamopyrgus antipodarum (New Zealand mud snail) is a mollusk species native to freshwater streams and lakes in New Zealand but has globally invaded since it was first reported outside of its native range in 1889 (Smith, 1889). The success of New Zealand mud snail in naive environments is attributed to many factors, including high reproductive and feeding rates, tolerance of a wide range of environmental conditions, and little predation in introduced regions. The species is a detritivore herbivore and can affect ecological function by disrupting the flow of energy through multiple trophic levels. The New Zealand mud snail often alters community structure by directly outcompeting native mollusks and aquatic insects for food resources and living space (Lysne and Koetsier, 2008; Riley and others, 2008). The species also resists digestion from fish, passing through the gut unharmed, which disperses the snail to new locations (van Leeuwen and others, 2012) while reducing the fish’s fitness (Rakauskas and others, 2016; Butkus and Rakauskas, 2020).
The presence of New Zealand mud snail in the Fountain Creek Basin was first discovered in 2011 in very low numbers at two locations in Fountain Creek (sites 6 and 7) during routine invertebrate sampling (Zuellig and others, 2022) and later was found at a third site (site 8) in 2012 (table 4). The species remained undetected even at the same locations between 2012 and 2016 despite continued sampling until it was collected in 2017 at Jimmy Camp Creek (site 9), where it has become well established (table 4). Since 2020, the New Zealand mud snail has become well established in Sutherland Ditch (site 11) and downstream along the right bank of Fountain Creek just below the confluence with the Sutherland Ditch site, which is just upstream from the USGS streamgage at Fountain Creek near Piñon, Colo. (site 12, table 1). In 2022, the species was detected at seven sites. Five of those sites are within the Fountain Creek Basin, and one is in the Arkansas River at Moffat Street at Pueblo, Colo., USGS streamgage (site 1, table 1). Thus far, the New Zealand mud snail has only been found in low numbers at the remaining sites, which present very different habitat compared to where the mud snail is well established. For example, the Jimmy Camp Creek and Sutherland Ditch sites are small streams with lower base streamflows and with an abundance of rooted emergent vegetation, which could be important living space for mud snail. Similarly, the right bank of Fountain Creek, just below the confluence with the Sutherland Ditch site, also harbors rooted emergent vegetation. The other sampling sites where the New Zealand mud snail has not become well established as of 2023 are much larger, have much greater base flow, lack rooted emergent vegetation, and have a streambed consisting of mostly unstable substrate (sands and gravels; Zuellig and others, 2022).
Table 4.
Numbers of New Zealand mud snail individuals removed from samples collected at monitoring locations within the Fountain Creek Basin study area, Colorado, from first observation in 2011 through 2022.[USGS station numbers and names are from the USGS National Water Information System database (USGS, 2023). Data sourced from Zuellig and others (2022). USGS, U.S. Geological Survey]
The 2023 USGS monitoring program comprises 14 sites consisting mostly of main-stem locations along Fountain Creek and the Arkansas River (fig. 1, table 1). Therefore, it is unknown how widespread the distribution of mud snail is within other parts of the Fountain Creek Basin study area, especially tributary sites where the species appears to become well established after introduction. The full consequences of the introduction and continued spread of mud snail are unknown, but changes in invertebrate community structure are possible (Maret and others, 2008; Rakauskas and others, 2017) as are shifts in the distribution of benthic feeding fish (Spyra and Strzelec, 2014), such as the State-threatened Arkansas darter.
Changes in Invertebrate Multimetric Index Values
Invertebrate MMI values were calculated for Fountain Creek Basin samples collected between 2006 and 2022 and plotted against year to detect obvious changes in MMI values through time. A total of 6 locations (sites 2, 4, 5, 6, 13, and 14) revealed decreasing values through time, indicating that biological condition has decreased at these sites during the sampling period (fig. 3A–F). Some sites showed rapid decreases in values relative to other locations (sites 6 and 14 compared to sites 2, 4, 5, and 13). The remaining eight sites did not show any clear patterns and therefore are not reported herein. Invertebrate MMI values were based on the most current version of CDPHE’s index (Jessup and Stribling, 2017), which is slated for revision in 2025 (Chris Theel, CDPHE, written commun., 2023). Understanding why these changes may have occurred is outside the scope of this report but could benefit from further investigation when the revised invertebrate MMI is available.
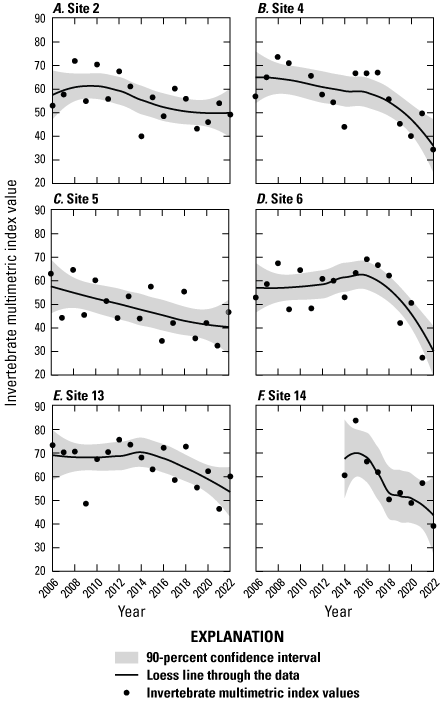
A–F, scatterplots showing invertebrate multimetric index values from selected U.S. Geological Survey sampling sites within the Fountain Creek Basin study area, Colorado, 2006–22. Refer to table 1 and figure 1 for U.S. Geological Survey sampling site descriptions and locations.
Fish and Invertebrate Response to Streamflow
The relation between streamflow characteristics and aquatic communities has received substantial attention, primarily because the association has often been modified from what it would be in natural conditions (Carlisle and others, 2019). This is an important distinction, because stream hydrology of a specific region is directly linked to the life history traits and behavioral response of the aquatic communities that evolved in the region (Lytle and Poff, 2004). For example, the rising or falling limb of a hydrograph in a snowmelt-based system is often related to spawning cues for fish (Yarnell and others, 2010) or emergence timing for invertebrates (Harper and Peckarsky, 2006). As a result, disruption of life history occurrences can prevent the persistence of native fauna (Brown and Bauer, 2010; Freeman and others, 2022) and can permanently alter communities within a stream (Gido and others, 2013; Wrona and others, 2016). Additionally, streamflow characteristics before sampling affect sampling results, interpretation of trend analysis, and often explain variability in ecological endpoints (Zuellig and Carlisle, 2019).
In the Fountain Creek Basin, numerous studies have identified various hydrograph streamflow characteristics that are important for describing variation in spatial patterns and temporal changes in invertebrate and fish community structure. Initially, von Guerard (1989a) reported a decrease in invertebrate densities in response to flooding 30 days before sampling, with flooding most prevalent in spring and early summer samples. Zuellig, Bruce, and others (2007) indicated that the number of annual high-flood pulses and average annual maximum daily streamflow contributed to explaining spatial patterns in invertebrate community similarity. Temporal invertebrate response to annual maximum mean daily streamflows were also reported by Zuellig and others (2010). Variation and magnitude in antecedent streamflow also explained temporal patterns in invertebrate and fish richness and community similarity among samples (Roberts and others, 2018).
Corroboration Between Streamflow and Age-Zero Flathead Chub
Studies examining population characteristics of early life stages of flathead chub reported lower growth and recruitment in years with more annual peak streamflow days compared to years with more stable streamflow conditions (Haworth and Bestgen, 2016, 2017). Roberts and others (2018) later validated findings of the Haworth and Bestgen studies (2016, 2017) with independent data in which they found greater catch per unit effort of age-zero flathead chub in years with fewer than 25 peak streamflow days. These patterns were corroborated with more recent data (since 2017; fig. 4) in which a similar pattern was observed between catch per unit effort of age-zero flathead chub and the number of peak streamflow days. As in Roberts and others (2018), a lower proportion of age-zero flathead chub were caught when the number of peak streamflow days was greater than 25 days (fig. 4). The extensive data on the relation between aquatic communities and streamflow documents the importance of streamflow in the Fountain Creek Basin in potentially regulating fish and invertebrate communities (von Guerard, 1989a; Zuellig, Bruce, and others, 2007, 2010; Roberts and others, 2018) and recruitment and population dynamics of individual fish species (Haworth and Bestgen, 2016, 2017; Roberts and others, 2018).
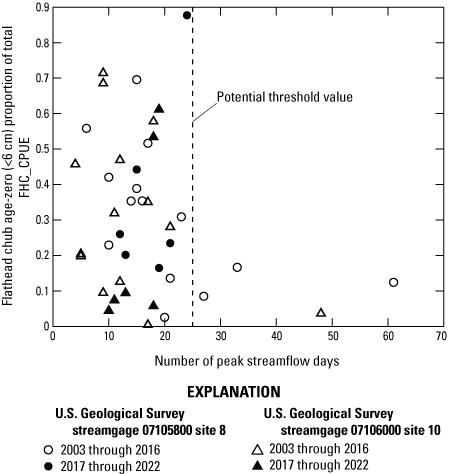
Flathead chub age-zero fish compared to the number of peak streamflow days in a year. The dashed grey line (peak streamflow days=25) is a potential threshold value above which there is little to no production of age-zero flathead chub. Modified from Roberts and others (2018) to include 2017 through 2022 data (Zuellig and others, 2022). <, less than; cm, centimeter; FHC_CPUE, the number of flathead chub captured divided by the product of surface acres sampled and number of nets.
Fish and Invertebrate Response to Water Temperature
A tremendous amount of research has been dedicated to understanding the association of water temperature with the ecology and life history traits of aquatic organisms (Connor and others, 2002; Smith and others, 2003; Pankhurst and Munday, 2011; Bonacina and others, 2023). Fish and invertebrate activity and growth are directly correlated with the ambient water temperature. Seasonal changes in water temperature can cue specific behavior, such as reproduction or migratory behavior of fish and the emergence of aquatic insects (Tyus, 1990; Finn and others, 2022). Additionally, aquatic species have unique optimal thermal preferences, such that body function is hampered in temperatures below or above each species’ threshold (Coutant, 1987). Exposure to undesirable temperatures can force individuals to vacate a location to seek refuge or even result in mortality if exposure is chronic. The elimination of species from a location because of intolerable water temperature can greatly alter local fish and invertebrate community structure (Jacobsen and others, 1997; Daufresne and others, 2004; Haase and others, 2019).
In the Fountain Creek Basin, most available water temperature data are from intermittent discrete measurements made during water quality or ecological sampling and therefore have been of limited use to explain variability in invertebrate and fish community data. Nonetheless, discrete water temperature has been noted as important for fish and invertebrates by Bruce (2002) and Roberts and others (2018). The Bruce (2002) study indicated invertebrate communities in the basin changed along a water-temperature gradient from cooler to warmer as water moved downstream away from the plains-mountain interface. Although these findings are factual, this is expected in transition zone streams in Colorado. The difficulty in these systems is separating the natural water temperature gradient (as well as other natural gradients) from human-induced changes. Additionally, Roberts and others (2018) found invertebrate community similarity and total fish catch were associated with discrete water temperature measurements at a few upstream sites (refer to table 6 in Roberts and others, 2018).
Other studies used the limited continuous water temperature data available in the Fountain Creek Basin. For example, Haworth and Bestgen (2016, 2017)40 determined spawning behavior of flathead chub was initiated after 15 degrees Celsius (°C), and individuals captured in warmer water temperatures had faster growth rates than individuals from cooler temperatures. The continuous water temperature data paired with continuous streamflow measurements at site 10 (table 1) made it possible to specify temperature-dependent early life development and growth characteristics of flathead chub in the Fountain Creek Basin (Haworth and Bestgen, 2016, 201740).
Invertebrate Community Tolerance to Water Temperature
The results of plotting average TIV values against year for each site indicate invertebrate community tolerance to warmer water temperatures increased since 2006 at 10 of 14 sites in the Fountain Creek Basin (fig. 5). The shift in communities toward tolerating warmer water could be explained by a general warming trend in water temperature, but the lack of continuous water temperature data made the explanation difficult to determine for this report. Bern and others (2024) reported upward trends in air temperature at Colorado Springs and Pueblo between 1980 and 2022. Air temperature is often correlated with water temperature (Isaak and others, 2010), but verifying this correlation in the Fountain Creek Basin could benefit from further investigation. Nonetheless, invertebrate communities in the Fountain Creek Basin are apparently shifting to tolerate warmer water temperatures since 2006 (fig. 5A–J).
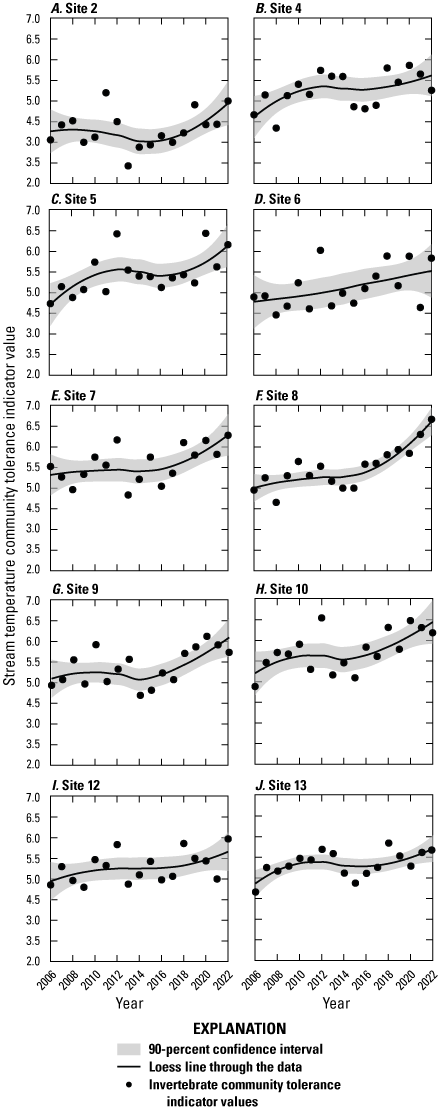
A–J, invertebrate community tolerance indicator values (Carlisle and others, 2007) for water temperature showing increasing values through time at selected sites within the Fountain Creek Basin, Colorado, 2006–22. Refer to table 1 and figure 1 for site descriptions and location.
Fish and Invertebrate Response to Selected Water Quality
Changes in water quality can be detrimental to aquatic organisms and have been linked to shifts in the structure and function of entire ecosystems (Appelberg and others, 1993; Kratina and others, 2012; Chen and others, 2019). Degradation of water quality dictates which species persist by filtering out intolerant taxa, altering species interactions, affecting ecosystem function (Sawyer and others, 2004; Bilotta and Brazier, 2008), and degrading biological condition (Violin and others, 2011).
Few studies in the Fountain Creek Basin have directly associated water quality constituents with temporal shifts in aquatic communities, because of inconsistencies in water quality monitoring at ecology locations that have restricted data analysis (Zuellig, Bruce, and others 2007, 2010108; Roberts and others, 2018). Nonetheless, there are a few examples in which water quality has explained spatial and temporal variability in aquatic communities. Zuellig, Bruce, and others (2007) observed dissolved nitrate plus nitrite explained spatial variation in invertebrate and fish community structure at a few sites in the Fountain Creek Basin between 2003 and 2005 (refer to table 7 in Zuellig, Bruce, and others, 2007). Specific conductance was correlated with temporal trends in fish communities at one site (refer to table 6 in Roberts and others, 2018). Others have explored the distribution of mercury (Hg) and selenium (Se) concentrations in fish and invertebrate tissues within the study area (Nimmo and others, 2016, 201870). In general, Hg in fish tissue was higher at upstream sites in the Fountain Creek Basin but was present at all sites studied, and it was detected in tissue even when not detected in water (Nimmo and others, 2016). Conversely, Se concentration in tissue was higher at downstream sites, where several exceedances of the Environmental Protection Agency Se standards occurred (Nimmo and others, 2016). Later, Nimmo and others (2018) investigated the effect of Se concentration in water on midge distribution in the Fountain Creek Basin and found some taxa were associated with higher and others with lower Se concentrations. Although neither study was designed to show direct body function or performance effects of Se or Hg on fish and invertebrate communities or individuals, Se and Hg are known contaminants with negative effects on aquatic life (Boening, 2000; Hamilton, 2004; Lemly, 2004).
Decreasing Multimetric Index Values and Documented Trends in Water Quality
Several highly likely trends in water quality from 1999 to 2022 were reported by Bern and others (2024) at eight sites in the Fountain Creek Basin, of which five (sites 2, 4, 5, 6, and 13, table 1) showed decreases in MMI values between 2006 and 2022 (fig. 3). Increases in concentration of the highly likely reported trends in water quality could possibly contribute to decreases in MMI values if the change in concentration was great enough to be biologically relevant (Zuellig and Carlisle, 2019, 2021111). Although a direct comparison of the trend and MMI results is limited, in part because the date ranges between the two studies differed, there are possible patterns of interest that need discussion. For example, decreases in MMI values occurred at two locations (sites 5 and 6, table 1) where upward trends in nitrate plus nitrite, orthophosphate, and chloride (site 5 only) concentrations co-occurred (table 5). Bern and others (2024) did not report trend magnitude, so it is difficult to determine if increases in concentration were large enough to indicate a possible contribution to observed decreases in MMI values. Although increased concentrations in the above constituents (nitrate plus nitrite, orthophosphate, and chloride) have been associated with the degradation of aquatic communities (Mainstone and Parr, 2002; Camargo and others, 2005; Kaushal and others, 2005), it is difficult to directly link these patterns given the previous inconsistencies. In contrast, sites 2, 4, and 13 (table 1) had various subsets of downward trends in ammonia, nitrate plus nitrite, unfiltered phosphorus, or orthophosphate (table 5). However, MMI values were also decreasing, which was counterintuitive, because MMI values would be expected to increase as concentrations decreased at these locations. There were no obvious changes in MMI values at the remaining sites (sites 7, 8, and 10), where upward and downward trends in some constituents were observed (table 5). The previous observation regarding invertebrate MMI values and water quality displays the complexity in determining the possible role water quality plays in structuring aquatic communities in the Fountain Creek Basin. Targeted studies that include paired water quality and ecological sampling could be beneficial. Nonetheless, further investigation is needed to potentially determine changes in biological condition in response to water quality constituents, especially because many co-occurring stressors are present at study sites in the Fountain Creek Basin (Zuellig, Bruce, and others, 2007). Aligning, consistent water quality data collection at ecological monitoring sites moving forward could greatly improve the ability to understand the role that water quality may play in structuring aquatic communities.
Table 5.
Selected highly likely water quality trend results from ecological monitoring locations.[Modified from Bern and others (2024, table 2). USGS streamgage numbers and names are from the USGS National Water Information System database (USGS, 2023). USGS, U.S. Geological Survey; NA, data unavailable for trend analysis; --, indicates trend result was less than highly likely]
Sites with decreasing multimetric index (MMI) values are illustrated in figure 3.
Fish and Invertebrate Response to Habitat
Instream habitat is typically described by measuring numerous characteristics of the stream channel, streambank, and streambed along a stream reach to explain spatial or temporal patterns in stream communities. In general, the presence of specific habitat types has been directly linked to fish and invertebrate community structure and population dynamics (Death and Winterbourn, 1995; Angermeier and Winston, 1998) where changes in habitat condition led to population declines or even the elimination of certain species (Rahel and others, 1996). Additionally, habitat diversity within a location has been directly related to local and regional species diversity (Boulinier and others, 1998) as the variety of habitat allowed numerous taxa to occupy a stream reach (Brown, 2003; Lepori and others, 2005). In contrast, locations with fewer habitat types often support fewer taxa (Rabení and others, 2005).
In the Fountain Creek Basin, habitat has contributed to spatial and temporal patterns in fish and invertebrate community structure. Spatial patterns in invertebrate communities are related to characteristics of the streambed (von Guerard, 1989a; Bruce, 2002) and stream wetted width (Bruce, 2002), which is expected because streambed and stream width naturally change as streams transition from mountain to plains environments. Typically, coarser particles, such as cobble, change to finer substrate, such as sand, as the stream widens and moves downstream across the plains. The proportion of substrate particle size classes (sand, gravel, or cobble) has a direct implication for the structure of aquatic communities. In the Fountain Creek Basin, substrate dominated by cobbles, with a mix of gravel and sand, contains invertebrates characterized by a higher proportion of disturbance- and contaminant-intolerant taxa (Bruce, 2002), whereas locations dominated by sand typically consist of fewer or no disturbance- and contaminant-intolerant taxa (Bruce, 2002). Although these associations are important and expected, it is difficult to separate human-induced changes in the streambed from naturally occurring gradients of transition zone streams. Zuellig, Bruce, and others (2007) attempted to lessen the effect of natural gradients and isolate human-induced effects by selecting habitat variables that were strongly related to urbanization but weakly related to elevation. In doing so, they found streambank characteristics, in part, contributed to spatial patterns in fish and invertebrate communities (Zuellig, Bruce, and others, 2007). Habitat was important in describing temporal changes in fish and invertebrate communities in the Fountain Creek Basin. For example, bank characteristics (lower bank capacity, maximum bank height) have contributed to site-specific temporal patterns in fish and invertebrate communities as well as substrate characteristics and the proportion of geomorphic units (percent of run, riffle, or pool) present at a site (Zuellig and others, 2010; Roberts and others, 2018).
Changes in substrate characteristics are often associated with natural or human-caused changes to the channel that result in the redistribution of substrate size classes along the streambed. When finer sediments (smaller particles) are redistributed along the streambed, it is often referred to as sedimentation, which is considered a primary factor affecting habitat quality, and results in decreases in fish and invertebrate production and changes in community structure (Waters, 1995). Often, finer sediments fill streambed pools and interstitial spaces between larger particles as well as directly abrade, dislodge, or bury benthic organisms and gravel spawning habitat (Jones and Clark, 1987; Wang and others, 2001; Shepherd and others, 2011). Certain families of caddisflies, mayflies, and stoneflies are highly susceptible to sedimentation and can easily be extirpated from systems that experience episodic sediment loads resulting from disturbance within the basin (McKenzie and others, 2020; Zuellig and others, 2002). High sediment loads can also alter fish behavior through a reduction in feeding success and growth rates; in addition, fine entrained sediments can damage gills, diminishing individual fitness (Kemp and others, 2011; Kjelland and others, 2015). Furthermore, high sedimentation during spawning showed delayed or decreased hatching rates in salmonids (Bowerman and others, 2014) and other fishes (Sutherland and others, 2002).
The direct effects of sedimentation on stream communities have not been well studied in the Fountain Creek Basin; however, characteristics of the streambed such as estimates of embeddedness, Wolman pebble counts (Wolman, 1954), and measures of sediment transport, have been shown to contribute to spatial and temporal changes in community structure (von Guerard, 1989a; Bruce, 2002; Zuellig and others, 2010; Roberts and others, 2018). Earlier studies in the Fountain Creek Basin related the spatial distribution of invertebrate communities to sediment transport and characteristics of the streambed (von Guerard, 1989a; Bruce, 2002). Locations with higher sediment transport and mobile benthic substrate (mostly sand) were often dominated by Oligochaetes (aquatic worms), and most insect species were less common or nonexistent. Locations with more stable substrate (mostly large gravel and cobble) had invertebrate communities with more diverse and contained higher proportions of mayflies, stoneflies, and caddisflies (von Guerard, 1989a). Several other studies found characteristics of the streambed (percentage of sand, gravel, and cobble) were also important in describing temporal patterns in fish and invertebrate community structure in the Fountain Creek Basin (Zuellig and others, 2012; Roberts and others, 2018).
Changes in Streambed Elevation
Stream ecologists often measure streambed characteristics to describe some aspect of community structure or population dynamic, whereas fluvial geomorphologists measure the streambed to describe changes in elevation and channel configuration and to document geomorphic change. In 2012, the USGS, in cooperation with Colorado Springs Utilities, began monitoring geomorphic change of Fountain Creek in response to potential changes induced by the Southern Delivery System water project that came online in 2016 to meet the demands of future human population growth in the Colorado Springs-Pueblo region (Water Technology, 2019). Since 2012, stream-channel topographic data have been collected annually at 10 study reaches located between Colorado Springs and its downstream confluence with the Arkansas River in Pueblo to generate elevation and elevation-change maps (Hempel, 2020; Hempel and others, 2021) and to assess changes in stream-channel elevation in response to the initiation and operation of the Southern Delivery System water project. These data could be used to determine if changes in channel morphology can be modeled based on existing channel characteristics and help identify infrastructure susceptible to damage during floods (Hempel, 2020; Hempel and others, 2021). This type of geomorphic change data also could be useful to help explain changes in biological communities (Paul and Meyer, 2001; Gebrekiros, 2016); however, only one ecological monitoring site (site 13) partially overlaps with the geomorphic study locations. For this reason, it is difficult to directly associate geomorphic change data (Hempel, 2020; Hempel and others, 2021) with spatial or temporal patterns in invertebrate and fish communities. Ideally, the geomorphic change data could be collected at ecological monitoring locations so they could be used to explain observed patterns in fish and invertebrate communities. In lieu of aligning ecological and geomorphic data collection, the relation between geomorphic change and communities could be explored by making use of cross-sectional streambed data collected during streamflow measurements at associated USGS streamgages in the basin. This analysis could provide a test of concept, before an effort is made to align monitoring networks, to potentially determine if changes in streambed elevation affect temporal or spatial patterns in aquatic communities in the Fountain Creek Basin.
Major Results
This study summarized information from the literature regarding what is known about changes in the distribution of fish and invertebrates within the Fountain Creek Basin and important factors that have been shown to effect spatial and temporal patterns in community structure and population dynamics. Notable changes in the distribution of fish and invertebrate taxa occurred in the Fountain Creek Basin (table 2 in Roberts and others, 2018; tables 2 and 3 herein), including complete elimination of certain mayfly, stonefly, and caddisfly taxa at several sites (table 3). Important relations among spatial and temporal patterns in aquatic communities and influential factors compiled from the literature are collectively illustrated in figure 6. Various streamflow characteristics were frequently noted to effect spatial and temporal patterns in fish and invertebrate communities, including early development and recruitment of flathead chub. Typically, streamflow metrics describing the magnitude and frequency of high streamflow before sampling were most often reported. Water quality and water temperature contributed to patterns in aquatic communities but were less commonly used as descriptors because these data were inconsistently available among and within studies. Nonetheless, there were examples in which water temperature, specific conductance, mercury, selenium, nutrients (ammonia, nitrate plus nitrite, unfiltered phosphorus, and orthophosphate), and chloride affected various aspects of aquatic community structure, including flathead chub growth and possibly biological condition based on invertebrate MMI values (fig. 6). Additionally, stream reach-scale habitat also was prominent in describing patterns in aquatic communities, especially measures associated with the streambank, stream channel, and composition of streambed substrate. Finally, several studies (Roberts and others, 2018; Zuellig and others, 2022) reported site-specific temporal changes in aquatic communities, but changes could not always be associated with water temperature, streamflow, water quality, or habitat.
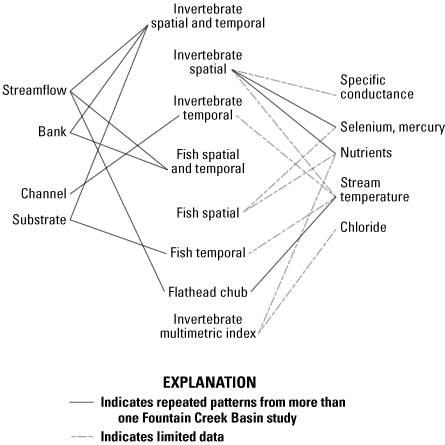
Conceptual model based on compiled literature and observed patterns in more recent data of the primary factors that could affect fish and invertebrate communities in the Fountain Creek Basin, 1984–2022.
Understanding the relations among aquatic communities and the environment is complex in transition-zone streams along the Front Range of the Rocky Mountains in Colorado. These ecosystems are affected by natural gradients and by multiple interacting stressors associated with urban development, agriculture, and intensive water management (Fausch and Bestgen, 1997). All studies included herein were observational in nature. Although these studies have revealed several connections between the environment and aquatic communities, they do not provide a direct association between human-induced environmental alterations and observed changes in spatial and temporal patterns in aquatic community structure. Concurrent observational studies along with field and laboratory experiments could be helpful in developing causal links between individual stressors and patterns in communities in the Fountain Creek Basin (Clements and others, 2002). Information generated from such integrative studies could be useful for developing causal relations and beneficial for well-targeted mitigation. Aligning consistent streamflow, water temperature, water quality, and geomorphic data collection at fish, invertebrate, and habitat monitoring locations moving forward could maximize information gained from monitoring efforts, potentially better informing evolving management activities and interests within the Fountain Creek Basin.
Summary
Various Federal and State agencies began studying how aquatic communities were affected by environmental conditions in the Fountain Creek Basin in the mid-1980s, which evolved into multifaceted monitoring by the early 2000s that was mostly constrained by water quality permit requirements regulated by the Colorado Department of Health and Environment. Focus areas of monitoring included surface-water hydrology, water quality, fluvial geomorphology, and ecology; however, monitoring locations, measured constituents, and program goals varied among regulated entities, which led to data-rich but information-poor monitoring. To identify potential opportunities for aligning monitoring components, which could maximize information gained from additional monitoring, the U.S. Geological Survey, in cooperation with Colorado Springs Utilities and Colorado Springs Stormwater Enterprise, synthesized knowledge gained in previous studies. Findings were supplemented with recent monitoring data to help understand current (2023) knowledge in the Fountain Creek Basin regarding the distribution of fish and invertebrates and the ecological response to environmental conditions such as streamflow, water temperature, and water quality.
A total of 52 publications within the study area between 1964 and 2022 were compiled from the literature and grouped into one of four general groups: ecology, geomorphology, streamflow, and water quality. Of these 52 publications, 19 were fish- and invertebrate-focused, and these were retained for synthesis and discussion. Overall, fish distribution in the Fountain Creek Basin has changed. There are several examples in which site-specific changes have occurred. For example, Notropis stramineus (sand shiner), Fundulus zebrinus (plains killifish), Cyprinella lutrensis (red shiner), Rhinichthys cataractae (longnose dace), and Etheostoma cragini (Arkansas darter) have occurred less frequently, whereas others like Lepomis cyanellus (green sunfish), Platygobio gracilis (flathead chub), and Gambusia affinis (western mosquitofish) are more frequently encountered. The distribution of some invertebrate taxa has also changed, and some invertebrate taxa may have been eliminated from certain locations. Three mayfly taxa (Drunella, Ephemerella, and Rhithrogena), five stonefly taxa (taxa Capniidae, Prostoia, Pteronarcella, Sweltsa, and Isoperla), and two caddisfly taxa (Arctopsyche and Rhyacophila) that were frequently collected in various combinations at several sites before 2000 were rarely encountered after, as of 2022. On the contrary, several invertebrate taxa have occurred with more frequency. For example, four mayfly taxa and one caddisfly taxon have occurred more frequently since 2000 at 11 of the 14 sampling sites. Additionally, Potamopyrgus antipodarum (New Zealand mud snail), an invasive mollusk, was first discovered in the Fountain Creek Basin in 2011 and is now well established in two tributary sites and has been detected at six sites at the time of this report. Finally, invertebrate multimetric index values, which measure biological condition, have declined at six locations between 2006 and 2022.
Numerous studies found various aspects of streamflow in the Fountain Creek Basin affected spatial and temporal patterns in fish and invertebrate communities and recruitment of age-zero flathead chub. Water temperature, although not extensively studied in the Fountain Creek Basin, contributed to temporal patterns in fish and invertebrates at a few locations and was shown to control flathead chub spawning and early growth and development of larvae. Tolerance indicator values of warmer water temperature showed that invertebrate communities have possibly shifted to tolerate warmer water temperatures at 10 of 14 sites since 2006. Because of its importance for aquatic biology, continuous water temperature monitoring could be beneficial for additional monitoring in the Fountain Creek Basin. On occasion, water quality, such as specific conductance, mercury, selenium, nutrients (ammonia, nitrate plus nitrite, unfiltered phosphorus, and orthophosphate), and chloride, affected various aspects of aquatic community structure and was possibly associated with biological condition based on invertebrate multimetric index values. Reach-scale habitat was also prominent in contributing to patterns in aquatic communities, especially measures associated with the streambank, stream channel, and composition of streambed substrate. Finally, several studies reported site-specific temporal changes in aquatic communities, but reported changes could not always be associated with the available environmental variables. Inconsistencies in data collection among programs have historically limited data interpretation. Aligning consistent streamflow, water temperature, water quality, and geomorphic data collection at fish, invertebrate, and habitat monitoring locations moving forward could maximize information gained from monitoring efforts and potentially better inform evolving management activities and interests within the Fountain Creek Basin.
References Cited
Angermeier, P.L., and Winston, M.R., 1998, Local vs. regional influences on local diversity in stream fish communities of Virginia: Ecology, v. 79, no. 3, p. 911–927. [Also available at https://doi.org/10.1890/0012-9658(1998)079[0911:LVRIOL]2.0.CO;2.]
Bern, C.R., Ruckhaus, M.H., and Hennessy, E., 2024, Potential climate and human water-use effects on water-quality trends in a semiarid, western U.S. watershed: Fountain Creek, Colorado, USA: Water, v. 16, no. 10, p. 1343. [Also available at https://doi.org/10.3390/w16101343.]
Bestgen, K.R., Rogers, K.B., and Granger, R., 2019, Distinct phenotypes of native cutthroat trout emerge under a molecular model of lineage distributions: Transactions of the American Fisheries Society, v. 148, no. 2, p. 442–463. [Also available at https://doi.org/10.1002/tafs.10145.]
Bilotta, G.S., and Brazier, R.E., 2008, Understanding the influence of suspended solids on water quality and aquatic biota: Water Research, v. 42, no. 12, p. 2849–2861. [Also available at https://doi.org/10.1016/j.watres.2008.03.018.]
Boening, D.W., 2000, Ecological effects, transport, and fate of mercury—A general review: Chemosphere, v. 40, no. 12, p. 1335–1351. [Also available at https://doi.org/10.1016/S0045-6535(99)00283-0.]
Bonacina, L., Fasano, F., Mezzanotte, V., and Fornaroli, R., 2023, Effects of water temperature on freshwater macroinvertebrates—A systematic review: Biological Reviews of the Cambridge Philosophical Society, v. 98, no. 1, p. 191–221. [Also available at https://doi.org/10.1111/brv.12903.]
Boulinier, T., Nichols, J.D., Sauer, J.R., Hines, J.E., and Pollock, K.H., 1998, Estimating species richness—The importance of heterogeneity in species detectability: Ecology, v. 79, no. 3, p. 1018–1028. [Also available at https://doi.org/10.1890/0012-9658(1998)079[1018:ESRTIO]2.0.CO;2.]
Bowerman, T., Neilson, B.T., and Budy, P., 2014, Effects of fine sediment, hyporheic flow, and spawning site characteristics on survival and development of bull trout embryos: Canadian Journal of Fisheries and Aquatic Sciences, v. 71, no. 7, p. 1059–1071. [Also available at https://doi.org/10.1139/cjfas-2013-0372.]
Brown, B.L., 2003, Spatial heterogeneity reduces temporal variability in stream insect communities: Ecology Letters, v. 6, no. 4, p. 316–325. [Also available at https://doi.org/10.1046/j.1461-0248.2003.00431.x.]
Brown, L.R., and Bauer, M.L., 2010, Effects of hydrologic infrastructure on flow regimes of California’s Central Valley rivers—Implications for fish populations: River Research and Applications, v. 26, no. 6, p. 751–765. [Also available at https://doi.org/10.1002/rra.1293.]
Bruce, J.F., 2002, Characterization and analysis of temporal and spatial variations in habitat and macroinvertebrate community structure, Fountain Creek Basin, Colorado Springs and vicinity, Colorado, 1998–2001: U.S. Geological Survey Water-Resources Investigations Report 2002–4093, 28 p., accessed July 6, 2021, at https://doi.org/10.3133/wri024093.
Bruce, J.F., Roberts, J., and Zuellig, R.E., 2018, Comparability among four invertebrate sampling methods and two multimetric indexes, Fountain Creek Basin, Colorado, 2010–2012: U.S. Geological Survey Scientific Investigations Report 2018–5061, 11 p. [Also available at https://doi.org/10.3133/sir20185061.]
Butkus, R., and Rakauskas, V., 2020, Experimental evidence that the invasive snail Potamopyrgus antipodarum (Gray, 1843) survives passage through the digestive tract of common riverine fish: Management of Biological Invasions: International Journal of Applied Research on Biological Invasions, v. 11, no. 1, p. 96–104. [Also available at https://doi.org/10.3391/mbi.2020.11.1.07.]
Camargo, J.A., Alonso, A., and Salamanca, A., 2005, Nitrate toxicity to aquatic animals—A review with new data for freshwater invertebrates: Chemosphere, v. 58, no. 9, p. 1255–1267. [Also available at https://doi.org/10.1016/j.chemosphere.2004.10.044.]
Carlisle, D.M., Meador, M.R., Moulton, S.R., II, and Ruhl, P.M., 2007, Estimation and application of indicator values for common macroinvertebrate genera and families of the United States: Ecological Indicators, v. 7, no. 1, p. 22–33. [Also available at https://doi.org/10.1016/j.ecolind.2005.09.005.]
Carlisle, D.M., Wolock, D.M., Konrad, C.P., McCabe, G.J., Eng, K., Grantham, T.E., and Mahler, B., 2019, Flow modification in the nation’s streams and rivers: U.S. Geological Survey Circular 1461, 75 p. [Also available at https://doi.org/10.3133/cir1461.]
Cashner, R.C., Schaefer, J., Warren, M.L., Jr., Echelle, A.A., Galvez, F., and Ghedotti, M.J., 2020, Fundulidae—Topminnows, chap. 30 of Warren, M.L., Jr., and Burr, B.M., with Echelle, A.A., Kuhajda, B.R., and Ross, S.T., eds., Characidae to Poeciliidae, v. 2 of Freshwater Fishes of North America: Baltimore, Md., Johns Hopkins University Press, p. 547–608. [Also available at https://muse.jhu.edu/book/74111.]
Chen, B., Wang, M., Duan, M., Ma, X., Hong, J., Xie, F., Zhang, R., and Li, X., 2019, In search of key—Protecting human health and the ecosystem from water pollution in China: Journal of Cleaner Production, v. 228, p. 101–111. [Also available at https://doi.org/10.1016/j.jclepro.2019.04.228.]
Clements, W.H., Carlisle, D.M., Courtney, L.A., and Harrahy, E.A., 2002, Integrating observational and experimental approaches to demonstrate causation in stream biomonitoring studies: Environmental Toxicology and Chemistry, v. 21, no. 6, p. 1138–1146. [Also available at https://doi.org/10.1002/etc.5620210605.]
Connor, W.P., Burge, H.L., Waitt, R., and Bjornn, T.C., 2002, Juvenile life history of wild fall Chinook salmon in the Snake and Clearwater Rivers: North American Journal of Fisheries Management, v. 22, no. 3, p. 703–712. [Also available at https://doi.org/10.1577/1548-8675(2002)022<0703:JLHOWF>2.0.CO;2.]
Coutant, C.C., 1987, Thermal preference—When does an asset become a liability?: Environmental Biology of Fishes, v. 18, no. 3, p. 161–172. [Also available at https://doi.org/10.1007/BF00000356.]
Daufresne, M., Roger, M.C., Capra, H., and Lamouroux, N., 2004, Long-term changes within the invertebrate and fish communities of the Upper Rhône River—Effects of climatic factors: Global Change Biology, v. 10, no. 1, p. 124–140. [Also available at https://doi.org/10.1046/j.1529-8817.2003.00720.x.]
Death, R.G., and Winterbourn, M.J., 1995, Diversity patterns in stream benthic invertebrate communities—The influence of habitat stability: Ecology, v. 76, no. 5, p. 1446–1460. [Also available at https://doi.org/10.2307/1938147.]
Department of Local Affairs, 2023, Population projections in Colorado: Colorado Information Marketplace, accessed October 13, 2023, at https://demography.dola.colorado.gov/.
Edelmann, P., Ferguson, S.A., Stogner, R.W., Sr., August, M., Payne, W.F., and Bruce, J.F., 2002, Evaluation of water quality, suspended sediment, and stream morphology with an emphasis on effects of stormflow on Fountain and Monument Creek basins, Colorado Springs and vicinity, Colorado, 1981 through 2001: U.S. Geological Survey Water-Resources Investigations Report 2002–4104, 59 p., accessed January 20, 2023, at https://doi.org/10.3133/wri024104.
Edmunds, G.F. Jr., and McCafferty, W.P., 1984, Ephemera compar—An obscure Colorado burrowing mayfly (Ephemeroptera—Ephemeridae): Entomological News, v. 95, p. 186–188. [Also available at https://www.biodiversitylibrary.org/partpdf/22608.]
Eschner, T.R., Hadley, R.F., and Crowley, K.D., 1983, Hydrologic and morphologic changes in the channels of the Platte River Basin—A historical perspective: U.S. Geological Survey Professional Paper 1277–A, 297 p. [Also available at https://doi.org/10.3133/pp1277.]
Fausch, K.D., and Bestgen, K.R., 1997, Ecology of fishes indigenous to the central and southwestern Great Plains, in Knopf, F.L., and Samson, F.B., eds., Ecology and conservation of Great Plains vertebrates: New York, Springer-Verlag, Inc., p. 131–166. [Also available at https://doi.org/10.1007/978-1-4757-2703-6_6.]
Finn, D.S., Johnson, S.L., Gerth, W.J., Arismendi, I., and Li, J.L., 2022, Spatiotemporal patterns of emergence phenology reveal complex species-specific responses to temperature in aquatic insects: Diversity & Distributions, v. 28, no. 8, p. 1524–1541. [Also available at https://doi.org/10.1111/ddi.13472.]
Freeman, M.C., Bestgen, K.R., Carlisle, D., Frimpong, E.A., Franssen, N.R., Gido, K.B., Irwin, E., Kanno, Y., Luce, C., Kyle McKay, S., Mims, M.C., Olden, J.D., LeRoy Poff, N., Propst, D.L., Rack, L., Roy, A.H., Stowe, E.S., Walters, A., and Wenger, S.J., 2022, Toward improved understanding of streamflow effects on freshwater fishes: Bethesda, Md., Fisheries, v. 47, no. 7, p. 290–298. [Also available at https://doi.org/10.1002/fsh.10731.]
Gebrekiros, S.T., 2016, Factors affecting stream fish community composition and habitat suitability: Journal of Aquaculture & Marine Biology, v. 4, no. 2. [Also available at https://doi.org/10.15406/jamb.2016.04.00076.]
Gido, K.B., Propst, D.L., Olden, J.D., and Bestgen, K.R., 2013, Multidecadal responses of native and introduced fishes to natural and altered flow regimes in the American Southwest: Canadian Journal of Fisheries and Aquatic Sciences, v. 70, no. 4, p. 554–564. [Also available at https://doi.org/10.1139/cjfas-2012-0441.]
Haase, P., Pilotto, F., Li, F., Sundermann, A., Lorenz, A.W., Tonkin, J.D., and Stoll, S., 2019, Moderate warming over the past 25 years has already reorganized stream invertebrate communities: Science of the Total Environment, v. 658, p. 1531–1538. [Also available at https://doi.org/10.1016/j.scitotenv.2018.12.234.]
Hamilton, S.J., 2004, Review of selenium toxicity in the aquatic food chain: Science of the Total Environment, v. 326, no. 1–3, p. 1–31. [Also available at https://doi.org/10.1016/j.scitotenv.2004.01.019.]
Hansen, W.R., Crosby, E.J., and Shroba, R.R., 1982, Environmental geology of the Front Range urban corridor and vicinity, Colorado, with a section on physical properties and performance characteristics of surficial deposits and rock units in the greater Denver area by R.R. Shroba: U.S. Geological Survey Professional Paper 1230, 99 p. [Also available at https://doi.org/10.3133/pp1230.]
Harper, M.P., and Peckarsky, B.L., 2006, Emergence cues of a mayfly in a high-altitude stream ecosystem—Potential response to climate change: Ecological Applications, v. 16, no. 2, p. 612–621. [Also available at https://doi.org/10.1890/1051-0761(2006)016[0612:ECOAMI]2.0.CO;2.]
Haworth, M.R., and Bestgen, K.R., 2016, Daily increment validation and effects of streamflow variability and water temperature on growth of age-0 flathead chub: North American Journal of Fisheries Management, v. 36, no. 4, p. 744–753. [Also available at https://doi.org/10.1080/02755947.2016.1165772.]
Haworth, M.R., and Bestgen, K.R., 2017, Flow and water temperature affect reproduction and recruitment of a Great Plains cyprinid: Canadian Journal of Fisheries and Aquatic Sciences, v. 74, no. 6, p. 853–863. [Also available at https://doi.org/10.1139/cjfas-2016-0238.]
Hempel, L.A., 2020, Elevation and elevation-change maps of Fountain Creek, southeastern Colorado, 2015–19: U.S. Geological Survey Scientific Investigations Map 3456, 9 p., 10 sheets, accessed January 20, 2023, at https://doi.org/10.3133/sim3456.
Hempel, L.A., Creighton, A.L., and Bock, A.R., 2021, Elevation and elevation-change maps of Fountain Creek, southeastern Colorado, 2015–20: U.S. Geological Survey Scientific Investigations Map 3481, 12 p., 10 sheets, accessed January 20, 2023, at https://doi.org/10.3133/sim3481.
Herrmann, S.J., Sublette, J.E., Helland, L.K., Nimmo, D.W.R., Carsella, J.S., Herrmann-Hoesing, L.M., and Heuvel, B.D. Vanden, 2016, Species richness, diversity, and ecology of Chironomidae (Diptera) in Fountain Creek—A Colorado Front Range sandy-bottom watershed: Western North American Naturalist, v. 76, no. 2, p. 186–252. [Also available at https://doi.org/10.3398/064.076.0206.]
Hirsch, R.M., Archfield, S.A., and De Cicco, L.A., 2015, A bootstrap method for estimating uncertainty of water quality trends: Environmental Modelling & Software, v. 73, p. 148–166. [Also available at https://doi.org/10.1016/j.envsoft.2015.07.017.]
Isaak, D.J., Luce, C.H., Rieman, B.E., Nagel, D.E., Peterson, E.E., Horan, D.L., Parkes, S., and Chandler, G.L., 2010, Effects of climate change and wildfire on stream temperatures and salmonid thermal habitat in a mountain river network: Ecological Applications, v. 20, no. 5, p. 1350–1371. [Also available at https://doi.org/10.1890/09-0822.1.]
Jacobsen, D., Schultz, R., and Encalada, A., 1997, Structure and diversity of stream invertebrate assemblages—The influence of temperature with altitude and latitude: Freshwater Biology, v. 38, no. 2, p. 247–261. [Also available at https://doi.org/10.1046/j.1365-2427.1997.00210.x.]
Jenkins, E.D., and Glover, R.E., 1964, Ground water in Fountain and Jimmy Camp Valleys, El Paso County, Colorado: U.S. Geological Survey Water Supply Paper 1583, 66 p., accessed January 20, 2023, at https://doi.org/10.3133/wsp1583.
Jessup, B.K., and Stribling, J.B., 2017, Recalibration of the Colorado benthic macroinvertebrate multimetric index, app. A of Aquatic Life Use Attainment—Methodology to determine use attainment for rivers and streams—Policy statement 10-1: Colorado Department of Public Health and Environment Water Quality Control Division—Environmental Data Unit, prepared by Tetra Tech, Inc., Center for Ecological Sciences, Montpelier, Vt., p. 1–62, 4 app. [Also available at https://qcnr.usu.edu/research/namc/files/services/colorado.pdf.]
Jones, R.C., and Clark, C.C., 1987, Impact of watershed urbanization on stream insect communities: Journal of the American Water Resources Association, v. 23, no. 6, p. 1047–1055. [Also available at https://doi.org/10.1111/j.1752-1688.1987.tb00854.x.]
Kaushal, S.S., Groffman, P.M., Likens, G.E., Belt, K.T., Stack, W.P., Kelly, V.R., Band, L.E., and Fisher, G.T., 2005, Increased salinization of fresh water in the northeastern United States: Proceedings of the National Academy of Sciences of the United States of America, v. 102, no. 38, p. 13517–13520. [Also available at https://doi.org/10.1073/pnas.0506414102.]
Kemp, P., Sear, D., Collins, A., Naden, P., and Jones, I., 2011, The impacts of fine sediment on riverine fish: Hydrological Processes, v. 25, no. 11, p. 1800–1821. [Also available at https://doi.org/10.1002/hyp.7940.]
Kjelland, M.E., Woodley, C.M., Swannack, T.M., and Smith, D.L., 2015, A review of the potential effects of suspended sediment on fishes—Potential dredging-related physiological, behavioral, and transgenerational implications: Environment Systems and Decisions, v. 35, no. 3, p. 334–350. [Also available at https://doi.org/10.1007/s10669-015-9557-2.]
Kratina, P., Greig, H.S., Thompson, P.L., Carvalho-Pereira, T.S.A., and Shurin, J.B., 2012, Warming modifies trophic cascades and eutrophication in experimental freshwater communities: Ecology, v. 93, no. 6, p. 1421–1430. [Also available at https://doi.org/10.1890/11-1595.1.]
Lemly, D.A., 2004, Aquatic selenium pollution is a global environmental safety issue: Ecotoxicology and Environmental Safety, v. 59, no. 1, p. 44–56. [Also available at https://doi.org/10.1016/S0147-6513(03)00095-2.]
Lepori, F., Palm, D., Brännäs, E., and Malmqvist, B., 2005, Does restoration of structural heterogeneity in streams enhance fish and macroinvertebrate diversity?: Ecological Applications, v. 15, no. 6, p. 2060–2071. [Also available at https://doi.org/10.1890/04-1372.]
Lysne, S., and Koetsier, P., 2008, Comparison of desert Valvata snail growth at three densities of the invasive New Zealand mudsnail: Western North American Naturalist, v. 68, no. 1, p. 103–106. [Also available at https://doi.org/10.3398/1527-0904(2008)68[103:CODVSG]2.0.CO;2.]
Lytle, D.A., and Poff, N.L., 2004, Adaptation to natural flow regimes: Trends in Ecology & Evolution, v. 19, no. 2, p. 94–100. [Also available at https://doi.org/10.1016/j.tree.2003.10.002.]
Mainstone, C.P., and Parr, W., 2002, Phosphorus in rivers—Ecology and management: Science of the Total Environment, v. 282–283, p. 25–47. [Also available at https://doi.org/10.1016/S0048-9697(01)00937-8.]
Maret, T.R., MacCoy, D.E., and Carlisle, D.M., 2008, Long-term water quality and biological responses to multiple best management practices in Rock Creek, Idaho: Journal of the American Water Resources Association, v. 44, no. 5, p. 1248–1269. [Also available at https://doi.org/10.1111/j.1752-1688.2008.00221.x.]
Mau, D.P., Stogner, R.W., Sr., and Edelmann, P., 2007, Characterization of stormflows and wastewater treatment-plant effluent discharges on water quality, suspended sediment, and stream morphology for Fountain and Monument Creek watersheds, Colorado, 1981–2006: U.S. Geological Survey Scientific Investigations Report 2007–5104, 76 p., accessed January 20, 2023, at https://doi.org/10.3133/sir20075104.
McCafferty, W.P., Durfee, R.S., and Kondratieff, B.C., 1993, Colorado mayflies (Ephemeroptera)—An annotated inventory: The Southwestern Naturalist, v. 38, no. 3, p. 252–274. [Also available at https://doi.org/10.2307/3671431.]
McKenzie, M., Mathers, K.L., Wood, P.J., England, J., Foster, I., Lawler, D., and Wilkes, M., 2020, Potential physical effects of suspended fine sediment on lotic macroinvertebrates: Hydrobiologia, v. 847, no. 3, p. 697–711. [Also available at https://doi.org/10.1007/s10750-019-04131-x.]
Meador, M.R., Carlisle, D.M., and Coles, J.F., 2008, Use of tolerance values to diagnose water-quality stressors to aquatic biota in New England streams: Ecological Indicators, v. 8, no. 5, p. 718–728. [Also available at https://doi.org/10.1016/j.ecolind.2008.01.002.]
Metcalf, J.L., Pritchard, V.L., Silvestri, S.M., Jenkins, J.B., Wood, J.S., Cowley, D.E., Evans, R.P., Shiozawa, D.K., and Martin, A.P., 2007, Across the great divide—Genetic forensics reveals misidentification of endangered cutthroat trout populations: Molecular Ecology, v. 16, no. 21, p. 4445–4454. [Also available at https://doi.org/10.1111/j.1365-294X.2007.03472.x.]
Metcalf, J.L., Stowell, S.L., Kennedy, C.M., Rogers, K.B., McDonald, D., Epp, J., Keepers, K., Cooper, A., Austin, J.J., and Martin, A.P., 2012, Historical stocking data and 19th century DNA reveal human-induced changes to native diversity and distribution of cutthroat trout: Molecular Ecology, v. 21, no. 21, p. 5194–5207. [Also available at https://doi.org/10.1111/mec.12028.]
Nimmo, D.R., Herrmann, S.J., Carsella, J.S., McGarvy, C.M., Foutz, H.P., Herrmann-Hoesing, L.M., Gregorich, J.M., Turner, J.A., and Vanden Heuvel, B.D., 2016, Mercury and selenium in fish of Fountain Creek, Colorado (USA)—Possible sources and implications: SpringerPlus, v. 5, no[437] 13 p., accessed on March 2, 2023, at https://doi.org/10.1186/s40064-016-2088-6.
Nimmo, D.W.R., Herrmann, S.J., Sublette, J.E., Melnykov, I.V., Helland, L.K., Romine, J.A., Carsella, J.S., Herrmann-Hoesing, L.M., Turner, J.A., and Vanden Heuvel, B.D., 2018, Occurrence of chironomid species (Diptera—Chironomidae) in the high Se-78 concentrations and high pH of Fountain Creek watershed, Colorado, USA: Western North American Naturalist, v. 78, no. 1, p. 39–64. [Also available at https://doi.org/10.3398/064.078.0106.]
Omernik, J.M., 1987, Ecoregions of the conterminous United States: Annals of the Association of American Geographers, v. 77, no. 1, p. 118–125. [Also available at https://doi.org/10.1111/j.1467-8306.1987.tb00149.x.]
Pankhurst, N.W., and Munday, P.L., 2011, Effects of climate change on fish reproduction and early life history stages: Marine & Freshwater Research, v. 62, no. 9, p. 1015–1026. [Also available at https://doi.org/10.1071/MF10269.]
Paul, M.J., and Meyer, J.L., 2001, Streams in the urban landscape: Annual Review of Ecology and Systematics, v. 32, p. 333–365. [Also available at https://doi.org/10.1146/annurev.ecolsys.32.081501.114040.]
R Core Team, 2023, R—A language and environment for statistical computing, version 4.4.0 (Puppy Cup): R Foundation for Statistical Computing software release, accessed October 10, 2023, at https://www.R-project.org.
Rabení, C.F., Doisy, K.E., and Zweig, L.D., 2005, Stream invertebrate community functional responses to deposited sediment: Aquatic Sciences, v. 67, no. 4, p. 395–402. [Also available at https://doi.org/10.1007/s00027-005-0793-2.]
Rahel, F.J., Keleher, C.J., and Anderson, J.L., 1996, Potential habitat loss and population fragmentation for cold water fish in the North Platte River drainage of the Rocky Mountains—Response to climate warming: Limnology and Oceanography, v. 41, no. 5, p. 1116–1123. [Also available at https://doi.org/10.4319/lo.1996.41.5.1116.]
Rakauskas, V., Butkus, R., and Merkytė, E., 2016, Consumption of the invasive New Zealand mud snail (Potamopyrgus antipodarum) by benthivorous predators in temperate lakes—A case study from Lithuania: Hydrobiologia, v. 775, no. 1, p. 213–230. [Also available at https://doi.org/10.1007/s10750-016-2733-7.]
Rakauskas, V., Šidagytė, E., Butkus, R., and Garbaras, A., 2017, Effect of the invasive New Zealand mud snail (Potamopyrgus antipodarum) on the littoral macroinvertebrate community in a temperate mesotrophic lake: Marine and Freshwater Research, v. 69, no. 1, p. 155–166. [Also available at https://doi.org/10.1071/MF17059.]
Riley, L.A., Dybdahl, M.F., and Hall, R.O., Jr., 2008, Invasive species impact—Asymmetric interactions between invasive and endemic freshwater snails: Journal of the North American Benthological Society, v. 27, no. 3, p. 509–520. [Also available at https://doi.org/10.1899/07-119.1.]
Roberts, J.J., Bruce, J.F., and Zuellig, R.E., 2018, Changes in biological communities of the Fountain Creek Basin, Colorado, 2003–2016, in relation to antecedent streamflow, water quality, and habitat: U.S. Geological Survey Scientific Investigations Report 2017–5162, 20 p., accessed January 17, 2020, at https://doi.org/10.3133/sir20175162.
Sanderson, J.S., Rowan, N., Wilding, T., Bledsoe, B.P., Miller, W.J., and Poff, N.L., 2012, Getting to scale with environmental flow assessment—The watershed flow evaluation tool: River Research and Applications, v. 28, no. 9, p. 1369–1377. [Also available at https://doi.org/10.1002/rra.1542.]
Sawyer, J.A., Stewart, P.M., Mullen, M.M., Simon, P.T., and Bennett, H.H., 2004, Influence of habitat, water quality, and land use on macro-invertebrate and fish assemblages of a southeastern coastal plain watershed, USA: Aquatic Ecosystem Health & Management, v. 7, no. 1, p. 85–99.] [Also available at https://doi.org/10.1080/14634980490281353.]
Shepherd, S.L., Dixon, J.C., Davis, R.K., and Feinstein, R., 2011, The effect of land use on channel geometry and sediment distribution in gravel mantled bedrock streams, Illinois River watershed, Arkansas: River Research and Applications, v. 27, no. 7, p. 857–866.] [Also available at https://doi.org/10.1002/rra.1401.]
Smith, H., Wood, P.J., and Gunn, J., 2003, The influence of habitat structure and flow permanence on invertebrate communities in karst spring systems: Hydrobiologia, v. 510, no. 1–3, p. 53–66.] [Also available at https://doi.org/10.1023/B:HYDR.0000008501.55798.20.]
Sprague, L.A., Zuellig, R.E., and Dupree, J.A., 2006, Effects of urbanization on stream ecosystems in the South Platte River Basin, Colorado and Wyoming, chap. A of Effects of urbanization on stream ecosystems in six metropolitan areas of the United States: U.S. Geological Survey Scientific Investigations Report 2006–5101–A, 139 p., accessed August 6, 2024, at https://doi.org/10.3133/sir20065101A.
Spyra, A., and Strzelec, M., 2014, Identifying factors linked to the occurrence of alien gastropods in isolated woodland water bodies: Naturwissenschaften, v. 101, no. 3, p. 229–239. [Also available at https://doi.org/10.1007/s00114-014-1153-7.]
Sutherland, A.B., Meyer, J.L., and Gardiner, E.P., 2002, Effects of land cover on sediment regime and fish assemblage structure in four southern Appalachian streams: Freshwater Biology, v. 47, no. 9, p. 1791–1805. [Also available at https://doi.org/10.1046/j.1365-2427.2002.00927.x.]
Tyus, H.M., 1990, Potamodromy and Reproduction of Colorado Squawfish in the Green River Basin, Colorado and Utah: Transactions of the American Fisheries Society, v. 119, no. 6, p. 1035–1047. [Also available at https://doi.org/10.1577/1548-8659(1990)119<1035:PAROCS>2.3.CO;2.]
U.S. Geological Survey [USGS], 2023, USGS water data for the Nation: U.S. Geological Survey National Water Information System database, accessed September 15, 2023, at https://doi.org/10.5066/F7P55KJN.
van Leeuwen, C.H.A.,Velde, G. van der, Groenendael, J.M. van, and Klaassen, M., 2012, Gut travelers—Internal dispersal of aquatic organisms by waterfowl: Journal of Biogeography, v. 39, no. 11, p. 2031–2040. [Also available at https://doi.org/10.1111/jbi.12004.]
Violin, C.R., Cada, P., Sudduth, E.B., Hassett, B.A., Penrose, D.L., and Bernhardt, E.S., 2011, Effects of urbanization and urban stream restoration on the physical and biological structure of stream ecosystems: Ecological Applications, v. 21, no. 6, p. 1932–1949. [Also available at https://doi.org/10.1890/10-1551.1.]
von Guerard, P., 1989a, Sediment-transport characteristics and effects of sediment transport on benthic invertebrates in the Fountain Creek drainage basin upstream from Widefield, southeastern Colorado, 1985–88: U.S. Geological Survey Water-Resources Investigations Report 89–4161, 225 p., accessed July 6, 2021, at https://doi.org/10.3133/wri894161.
von Guerard, P., 1989b, Suspended sediment and sediment-source areas in the Fountain Creek drainage basin upstream from Widefield, southeastern Colorado: U.S. Geological Survey Water-Resources Investigations Report 88–4136, 36 p., accessed July 6, 2021, at https://doi.org/10.3133/wri884136.
Wahl, C.F., Zuellig, R.E., and Hennessy, E.K., 2024, Datasets for evaluation and review of ecology-focused stream studies, Fountain Creek Basin, Colorado: U.S. Geological Survey data release, https://doi.org/10.5066/P91QQ6GT.
Wang, L., Lyons, J., Kanehl, P., and Bannerman, R., 2001, Impacts of urbanization on stream habitat and fish across multiple spatial scales: Environmental Management, v. 28, no. 2, p. 255–266. [Also available at https://doi.org/10.1007/s0026702409.]
Ward, R.C., Loftis, J.C., and McBride, G.B., 1986, The “data-rich but information-poor” syndrome in water quality monitoring: Environmental Management, v. 10, no. 3, p. 291–297. [Also available at https://doi.org/10.1007/BF01867251.]
Water Technology, 2019, Southern Delivery System (SDS) water project, Colorado: Water Technology web page, accessed September 28, 2019, at https://www.water-technology.net/projects/southern-delivery-system-water-project/.
Wickham, H., Chang, W, Henry, L., Pedersen, T.L., Takahashi, K., Wilke, C., Woo, K., Yutani, H., Dunnington, D., and van den Brand, T., 2023, ggplot2, version 3.5.1: posit, accessed October 10, 2023, at https://ggplot2.tidyverse.org.
Wolman, M.G., 1954, A method for sampling coarse river-bed material: Transactions American Geophysical Union, v. 35, no. 6, p. 951–956. [Also available at https://doi.org/10.1029/TR035i006p00951.]
Wrona, F.J., Johansson, M., Culp, J.M., Jenkins, A., Mård, J., Myers-Smith, I.H., Prowse, T.D., Vincent, W.F., and Wookey, P.A., 2016, Transitions in arctic ecosystems—Ecological implications of a changing hydrological regime: Journal of Geophysical Research, Biogeosciences, v. 121, no. 3, p. 650–674. [Also available at https://doi.org/10.1002/2015JG003133.]
Yarnell, S.M., Viers, J.H., and Mount, J.F., 2010, Ecology and management of the spring snowmelt recession: Bioscience, v. 60, no. 2, p. 114–127. [Also available at https://doi.org/10.1525/bio.2010.60.2.6.]
Yuan, L.L., 2004, Assigning macroinvertebrate tolerance classifications using generalized additive models: Freshwater Biology, v. 49, no. 5, p. 662–677. [Also available at https://doi.org/10.1111/j.1365-2427.2004.01206.x.]
Zuellig, R.E., Bruce, J.F., Evans, E.E., and Stogner, R.W., Sr., 2007, Urban-related environmental variables and their relation with patterns in biological community structure in the Fountain Creek Basin, Colorado, 2003–2005: U.S. Geological Survey Scientific Investigations Report 2007–5225, 24 p., accessed July 6, 2021, at https://doi.org/10.3133/sir20075225.
Zuellig, R.E., Bruce, J.F., and Stogner, R.W., Sr., 2010, Temporal change in biological community structure in the Fountain Creek basin, Colorado, 2001–2008: U.S. Geological Survey Scientific Investigations Report 2010–5103, 19 p., accessed July 6, 2021, at https://doi.org/10.3133/sir20105103.
Zuellig, R.E., Bruce, J.F., Stogner, R.W., Sr., and Brown, K.D., 2014, Comparability among four invertebrate sampling methods, Fountain Creek Basin, Colorado, 2010–2012: U.S. Geological Survey Scientific Investigations Report 2014–5049, 13 p. [Also available at https://doi.org/10.3133/sir20145049.]
Zuellig, R.E., and Carlisle, D.M., 2019, Effects of antecedent streamflow and sample timing on trend assessments of fish, invertebrate, and diatom communities: Journal of the American Water Resources Association, v. 55, no. 1, p. 102–115. [Also available at https://doi.org/10.1111/1752-1688.12706.]
Zuellig, R.E., and Carlisle, D.M., 2021, Estimating invertebrate response to changes in total nitrogen, total phosphorus, and specific conductance at sites where invertebrate data are unavailable: U.S. Geological Survey Scientific Investigations Report 2021–5070, 24 p. [Also available at https://doi.org/10.3133/sir20215070.]
Zuellig, R.E., Covert, S.A., and Hennessy, E.K., 2022, Invertebrate, fish, and habitat data collected within the greater Fountain Creek Basin, Colorado (ver. 3.0, March 2023): U.S. Geological Survey data release, accessed October 1, 2023, at https://doi.org/10.5066/P9PUY4FC.
Zuellig, R.E., Heinold, B.D., Kondratieff, B.C., and Ruiter, D.E., 2012, Diversity and distribution of mayflies (Ephemeroptera), stoneflies (Plecoptera), and caddisflies (Trichoptera) of the South Platte River Basin, Colorado, Nebraska, and Wyoming, 1873–2010: U.S. Geological Survey Data Series 606, 109 p. [Also available at https://doi.org/10.3133/ds606.]
Zuellig, R.E., Sprague, L.A., Collins, J.A., and Cox, O.N., 2007, Aquatic communities and selected water chemistry in St. Vrain Creek near the City of Longmont, Colorado, wastewater-treatment plant, 2005 and 2006: U.S. Geological Survey Data Series 253, 30 p. [Also available at https://doi.org/10.3133/ds253.]
Appendix 1. References from Literature Review
Ecology
Bestgen, K.R., Crockett, H.J., Haworth, M.R., and Fitzpatrick, R.M., 2016, Production of nonadhesive eggs by flathead chub and implications for downstream transport and conservation: Journal of Fish and Wildlife Management, v. 7, no. 2, p. 434–443. [Also available at https://doi.org/10.3996/022016-JFWM-018.]
Bestgen, K.R., Wilcox, C.T., Hill, A.A., and Fausch, K.D., 2017, A dynamic flow regime supports an intact Great Plains stream fish assemblage: Transactions of the American Fisheries Society, v. 146, no. 5, p. 903–916. [Also available at https://doi.org/10.1080/00028487.2017.1310137.]
Bruce, J.F., 2002, Characterization and analysis of temporal and spatial variations in habitat and macroinvertebrate community structure, Fountain Creek basin, Colorado Springs and vicinity, Colorado, 1998–2001: U.S. Geological Survey Water-Resources Investigations Report 2002–4093, 28 p., accessed July 6, 2021, at https://doi.org/10.3133/wri024093.
Bruce, J.F., Roberts, J., and Zuellig, R.E., 2018, Comparability among four invertebrate sampling methods and two multimetric indexes, Fountain Creek Basin, Colorado, 2010–2012: U.S. Geological Survey Scientific Investigations Report 2018–5061, 11 p., accessed January 17, 2020, at https://doi.org/10.3133/sir20185061.
Haworth, M.R., and Bestgen, K.R., 2016, Daily increment validation and effects of streamflow variability and water temperature on growth of age-0 flathead chub: North American Journal of Fisheries Management, v. 36, no. 4, p. 744–753. [Also available at https://doi.org/10.1080/02755947.2016.1165772.]
Haworth, M.R., and Bestgen, K.R., 2017, Flow and water temperature affect reproduction and recruitment of a Great Plains cyprinid: Canadian Journal of Fisheries and Aquatic Sciences, v. 74, no. 6, p. 853–863. [Also available at https://doi.org/10.1139/cjfas-2016-0238.]
Herrmann, S.J., Sublette, J.E., Helland, L.K., Nimmo, D.W.R., Carsella, J.S., Herrmann-Hoesing, L.M., and Heuvel, B.D. Vanden, 2016, Species richness, diversity, and ecology of Chironomidae (Diptera) in Fountain Creek—A Colorado front range sandy-bottom watershed: Western North American Naturalist, v. 76, no. 2, p. 186–252. [Also available at https://doi.org/10.3398/064.076.0206.]
Miller, D.L., 1984, Distribution, abundance, and habitat of the Arkansas Darter Etheostoma cragini (Percidae) in Colorado: The Southwestern Naturalist, v. 29, no. 4, p. 496–499, accessed January 20, 2023. [Also available at https://doi.org/10.2307/3671005.]
Nimmo, D.R., Herrmann, S.J., Carsella, J.S., McGarvy, C.M., Foutz, H.P., Herrmann-Hoesing, L.M., Gregorich, J.M., Turner, J.A., and Vanden Heuvel, B.D., 2016, Mercury and selenium in fish of Fountain Creek, Colorado (USA)—Possible sources and implications: SpringerPlus, v. 5, no. 437, 13 p., accessed March 2, 2023, at https://doi.org/10.1186/s40064-016-2088-6.
Nimmo, D.W.R., Herrmann, S.J., Sublette, J.E., Melnykov, I.V., Helland, L.K., Romine, J.A., Carsella, J.S., Herrmann-Hoesing, L.M., Turner, J.A., and Vanden Heuvel, B.D., 2018, Occurrence of Chironomid species (Diptera—Chironomidae) in the high Se-78 concentrations and high pH of Fountain Creek watershed, Colorado, USA: Western North American Naturalist, v. 78, no. 1, p. 39–64. [Also available at https://doi.org/10.3398/064.078.0106.]
Roberts, J., Bruce, J.F., and Zuellig, R.E., 2018, Changes in biological communities of the Fountain Creek Basin, Colorado, 2003–2016, in relation to antecedent streamflow, water quality, and habitat: U.S. Geological Survey Scientific Investigations Report 2017–5162, 20 p., accessed January 17, 2020, at https://doi.org/10.3133/sir20175162.
von Guerard, P., 1989a, Sediment-transport characteristics and effects of sediment transport on benthic invertebrates in the Fountain Creek drainage basin upstream from Widefield, southeastern Colorado, 1985–88: U.S. Geological Survey Water-Resources Investigations Report 89–4161, 225 p., accessed July 6, 2021, at https://doi.org/10.3133/wri894161.
Walters, D.M., Zuellig, R.E., Crockett, H.J., Bruce, J.F., Lukacs, P.M., and Fitzpatrick, R.M., 2014, Barriers impede upstream spawning migration of flathead chub: Transactions of the American Fisheries Society, v. 143, no. 1, p. 17–25. [Also available at https://doi.org/10.1080/00028487.2013.824921.]
Zuellig, R.E., Bruce, J.F., Evans, E.E., and Stogner, R.W., Sr., 2007, Urban-related environmental variables and their relation with patterns in biological community structure in the Fountain Creek Basin, Colorado, 2003–2005: U.S. Geological Survey Scientific Investigations Report 2007–5225, 24 p., accessed July 6, 2021, at https://doi.org/10.3133/sir20075225.
Zuellig, R.E., Bruce, J.F., and Stogner, R.W., Sr., 2010, Temporal change in biological community structure in the Fountain Creek Basin, Colorado, 2001–2008: U.S. Geological Survey Scientific Investigations Report 2010–5103, 19 p., accessed July 6, 2021, at https://doi.org/10.3133/sir20105103.
Zuellig, R.E., Bruce, J.F., Stogner, R.W., Sr., and Brown, K.D., 2014, Comparability among four invertebrate sampling methods, Fountain Creek Basin, Colorado, 2010–2012: U.S. Geological Survey Scientific Investigations Report 2014–5049, 13 p., accessed January 20, 2023, at https://doi.org/10.3133/sir20145049.
Geomorphology
Hempel, L.A., 2020, Elevation and elevation-change maps of Fountain Creek, southeastern Colorado, 2015–19: U.S. Geological Survey Scientific Investigations Map 3456, 9 p., 11 sheets, accessed January 20, 2023, at https://doi.org/10.3133/sim3456.
Hempel, L.A., Creighton, A.L., and Bock, A.R., 2021, Elevation and elevation-change maps of Fountain Creek, southeastern Colorado, 2015–20: U.S. Geological Survey Scientific Investigations Map 3481, 12 p., 10 sheets, accessed January 20, 2023, at https://doi.org/10.3133/sim3481.
Stogner, R.W., Sr., 2000, Trends in precipitation and streamflow and changes in stream morphology in the Fountain Creek watershed, Colorado, 1939–99: U.S. Geological Survey Water-Resources Investigations Report 2000–4130, 43 p., accessed January 20, 2023, at https://doi.org/10.3133/wri004130.
von Guerard, P., 1989b, Suspended sediment and sediment-source areas in the Fountain Creek drainage basin upstream from Widefield, southeastern Colorado: U.S. Geological Survey Water-Resources Investigations Report 88–4136, 36 p., accessed July 6, 2021, at https://doi.org/10.3133/wri884136.
Streamflow
Colarullo, S.J., and Miller, L.D., 2019, Upgrades to a Fortran program for estimating stream transit losses of reusable water, El Paso and Pueblo Counties, Colorado: U.S. Geological Survey Scientific Investigations Report 2018–5163, 21 p., 1 sheet, accessed January 20, 2023, at https://doi.org/10.3133/sir20185163.
Jenkins, E.D., and Glover, R.E., 1964, Ground water in Fountain and Jimmy Camp Valleys, El Paso County, Colorado: U.S. Geological Survey Water Supply Paper 1583, 66 p., accessed January 20, 2023, at https://doi.org/10.3133/wsp1583.
Kimbrough, R.A., and Holmes, R.R., Jr., 2015, Flooding in the South Platte River and Fountain Creek Basins in eastern Colorado, September 9–18, 2013: U.S. Geological Survey Scientific Investigations Report 2015–5119, 33 p., accessed January 20, 2023, at https://doi.org/10.3133/sir20155119.
Kohn, M.S., Fulton, J.W., Williams, C.A., and Stogner, R.W., Sr., 2014, Remediation scenarios for attenuating peak flows and reducing sediment transport in Fountain Creek, Colorado, 2013: U.S. Geological Survey Scientific Investigations Report 2014–5019, 62 p., accessed January 20, 2023, at https://doi.org/10.3133/sir20145019.
Kohn, M.S., Stevens, M.R., Mommandi, A., and Khan, A.R., 2017, Peak discharge, flood frequency, and peak stage of floods on Big Cottonwood Creek at U.S. Highway 50 near Coaldale, Colorado, and Fountain Creek below U.S. Highway 24 in Colorado Springs, Colorado, 2016: U.S. Geological Survey Scientific Investigations Report 2017–5107, 58 p., accessed January 20, 2023, at https://doi.org/10.3133/sir20175107.
Kuhn, G., 1988, Methods to determine transit losses for return flows of transmountain water in Fountain Creek between Colorado Springs and the Arkansas River, Colorado: U.S. Geological Survey Water-Resources Investigations Report 87–4119, 183 p., accessed January 20, 2023, at https://doi.org/10.3133/wri874119.
Kuhn, G., and Arnold, L.R., 2006, Application of a stream-aquifer model to Monument Creek for development of a method to estimate transit losses for reusable water, El Paso County, Colorado: U.S. Geological Survey Scientific Investigations Report 2006–5184, 111 p., accessed January 20, 2023, at https://doi.org/10.3133/sir20065184.
Kuhn, G., and Ortiz, R.F., 1989, Selected hydrologic data for Fountain Creek and Monument Creek basins, east-central Colorado: U.S. Geological Survey Open-File Report 88–705, 106 p., accessed January 20, 2023, at https://doi.org/10.3133/ofr88705.
Kuhn, G., Krammes, G.S., and Beal, V.J., 2007a, Description and user manual for a web-based interface to a transit-loss accounting program for Monument and Fountain Creeks, El Paso and Pueblo Counties, Colorado: U.S. Geological Survey Scientific Investigations Report 2007–5028, 36 p., accessed January 20, 2023, at https://doi.org/10.3133/sir20075028.
Kuhn, G., Krammes, G.S., and Beal, V.J., 2007b, Nodes and subreaches along Monument and Fountain Creeks and locations of streamflow-gaging stations, wastewater-treatment facilities, and diversions used in new Transit-Loss Accounting Program: U.S. Geological Survey Scientific Investigations Report 2007-5028 Plate 1, 1 p., accessed January 20, 2023, at https://pubs.usgs.gov/sir/2007/5028/pdf/SIR07-5028_plate1.pdf.
Kuhn, G., Samuels, E.L., Bemis, W.D., and Steger, R.D., 1998, Descriptions of the program changes (1989–97) and a user manual for a transit-loss accounting program applied to Fountain Creek between Colorado Springs and the Arkansas River, Colorado: U.S. Geological Survey Open-File Report 97–637, 39 p., accessed January 20, 2023, at https://doi.org/10.3133/ofr97637.
Leonard, G.J., 1984, Assessment of water resources at Fort Carson Military Reservation near Colorado Springs, Colorado: U.S. Geological Survey Water-Resources Investigations Report 83–4270, 78 p., accessed January 20, 2023, at https://doi.org/10.3133/wri834270.
Newman, C.P., Paschke, S.S., and Keith, G., 2021, Natural and anthropogenic geochemical tracers to investigate residence times and groundwater–surface-water interactions in an urban alluvial aquifer: Water (Basel), v. 13, no. 6, 30 p., accessed January 20, 2023, at https://doi.org/10.3390/w13060871.
Stogner, R.W., Sr., 2000, Trends in precipitation and streamflow in the Fountain Creek watershed, southeastern Colorado, 1977–99: U.S. Geological Survey Fact Sheet 136–00, 4 p., accessed January 20, 2023, at https://doi.org/10.3133/fs13600.
Stogner, R.W., Sr., Nelson, J.M., McDonald, R.R., Kinzel, P.J., and Mau, D.P., 2013, Prediction of suspended-sediment concentrations at selected sites in the Fountain Creek watershed, Colorado, 2008–09: U.S. Geological Survey Scientific Investigations Report 2012–5102, 36 p., accessed January 20, 2023, at https://doi.org/10.3133/sir20125102.
Water Quality
Arnold, L.R., Ortiz, R.F., Brown, C.R., and Watts, K.R., 2016, Groundwater and surface-water interaction, water quality, and processes affecting loads of dissolved solids, selenium, and uranium in Fountain Creek near Pueblo, Colorado, 2012–2014: U.S. Geological Survey Scientific Investigations Report 2016–5134, 78 p., accessed January 20, 2023, at https://doi.org/10.3133/sir20165134.
Battaglin, W.A., and Kisfalusi, Z.D., 2022, Characterization of and relations among precipitation, streamflow, suspended-sediment, and water-quality data at the U.S. Army Garrison Fort Carson and Piñon Canyon Maneuver Site, Colorado, water years 2016–18: U.S. Geological Survey Scientific Investigations Report 2022–5018, 94 p., accessed January 20, 2023, at https://doi.org/10.3133/sir20225018.
Bossong, C.R., 2001, Summary of water-quality data, October 1987 through September 1997, for Fountain and Monument Creeks, El Paso and Pueblo Counties, Colorado: U.S. Geological Survey Water-Resources Investigations Report 2000–4263, 69 p., accessed January 20, 2023, at https://doi.org/10.3133/wri004263.
Cain, D., and Edelmann, P., 1986, A reconnaissance water-quality appraisal of the Fountain Creek alluvial aquifer between Colorado Springs and Pueblo, Colorado, including trace elements and organic constituents: U.S. Geological Survey Water-Resources Investigations Report 86–4085, 45 p., accessed January 20, 2023, at https://doi.org/10.3133/wri864085.
Chafin, D.T., 1996, Effects of land use on water quality of the Fountain Creek alluvial aquifer, east-central Colorado: U.S. Geological Survey Water Supply Paper 2381-D, 99 p., accessed January 20, 2023, at https://doi.org/10.3133/wsp2381D.
Edelmann, P., 1990, Water quality of Fountain and Monument creeks, south-central Colorado, with emphasis on relation of water quality to stream classifications: U.S. Geological Survey Water-Resources Investigations Report 88–4132, 99 p., accessed January 20, 2023, at https://doi.org/10.3133/wri884132.
Edelmann, P., and Cain, D., 1985, Sources of water and nitrogen to the Widefield Aquifer, southwestern El Paso County, Colorado: U.S. Geological Survey Water-Resources Investigations Report 85–4162, 81 p., accessed January 20, 2023, at https://doi.org/10.3133/wri854162.
Edelmann, P., Ferguson, S.A., Stogner, R.W., Sr., August, M., Payne, W.F., and Bruce, J.F., 2002, Evaluation of water quality, suspended sediment, and stream morphology with an emphasis on effects of stormflow on Fountain and Monument Creek basins, Colorado Springs and vicinity, Colorado, 1981 through 2001: U.S. Geological Survey Water-Resources Investigations Report 2002–4104, 59 p., accessed January 20, 2023, at https://doi.org/10.3133/wri024104.
Holmberg, M.J., Stogner, R.W., Sr., and Bruce, J.F., 2016, Characterization and relation of precipitation, streamflow, and water-quality data at the U.S. Army Garrison Fort Carson and Piñon Canyon Maneuver Site, Colorado, water years 2013–14: U.S. Geological Survey Scientific Investigations Report 2016–5145, 58 p., accessed January 20, 2023, at https://doi.org/10.3133/sir20165145.
Kuhn, G., 1991, Calibration, verification, and use of a steady-state stream water-quality model for Monument and Fountain creeks, east-central Colorado: U.S. Geological Survey Water-Resources Investigations Report 91–4055, 149 p., accessed January 20, 2023, at https://doi.org/10.3133/wri914055.
Lewis, M.E., 1995, Quality of water in the alluvial aquifer and tributary alluvium of the Fountain Creek valley, southwestern El Paso County, Colorado, 1991–92: U.S. Geological Survey Water-Resources Investigations Report 94–4118, 39 p., accessed January 20, 2023, at https://doi.org/10.3133/wri944118.
Mau, D.P., Stogner, R.W., Sr., and Edelmann, P., 2007, Characterization of stormflows and wastewater treatment-plant effluent discharges on water quality, suspended sediment, and stream morphology for Fountain and Monument Creek watersheds, Colorado, 1981–2006: U.S. Geological Survey Scientific Investigations Report 2007–5104, 76 p., accessed January 20, 2023, at https://doi.org/10.3133/sir20075104.
Miller, L.D., and Stogner, R.W., Sr., 2017, Characterization of water quality and suspended sediment during cold-season flows, warm-season flows, and stormflows in the Fountain and Monument Creek watersheds, Colorado, 2007–2015: U.S. Geological Survey Scientific Investigations Report 2017–5084, 47 p., accessed January 20, 2023, at https://doi.org/10.3133/sir20175084.
Ortiz, R.F., 2004, Methods to identify changes in background water-quality conditions using dissolved-solids concentrations and loads as indicators, Arkansas River and Fountain Creek, in the vicinity of Pueblo, Colorado: U.S. Geological Survey Scientific Investigations Report 2004–5024, 20 p., accessed January 20, 2023, at https://doi.org/10.3133/sir20045024.
Ruddy, B.C., 1993, Water-quality variations and trends in Monument and Fountain Creeks, El Paso and Pueblo counties, Colorado, water years 1976–88: U.S. Geological Survey Water-Resources Investigations Report 91–4176, 66 p., accessed January 20, 2023, at https://doi.org/10.3133/wri914176.
Stoeckel, D., 2011, Evaluation of fecal contamination by human and ruminant sources in upper Fountain Creek, Colorado, 2007–2008, by using multiple lines of evidence: U.S. Geological Survey Fact Sheet 2011–3095, 4 p., accessed January 20, 2023, at https://doi.org/10.3133/fs20113095.
Stoeckel, D.M., Stelzer, E.A., Stogner, R.W., and Mau, D.P., 2011, Semi-quantitative evaluation of fecal contamination potential by human and ruminant sources using multiple lines of evidence: Water Research, v. 45, no. 10, p. 3225–3244, accessed January 20, 2023, at https://doi.org/10.1016/j.watres.2011.03.037.
Conversion Factors
Datums
Vertical coordinate information is referenced to the North American Vertical Datum of 1988 (NAVD 88).
Horizontal coordinate information is referenced to the North American Datum of 1983 (NAD 83).
Elevation, as used in this report, refers to distance above the vertical datum.
Publishing support provided by the Science Publishing Network,
Denver Publishing Service Center
For more information concerning the research in this report, contact the
Director, USGS Colorado Water Science Center
Box 25046, Mail Stop 415
Denver, CO 80225
(303) 236-4882
Or visit the Colorado Water Science Center website at
Disclaimers
Any use of trade, firm, or product names is for descriptive purposes only and does not imply endorsement by the U.S. Government.
Although this information product, for the most part, is in the public domain, it also may contain copyrighted materials as noted in the text. Permission to reproduce copyrighted items must be secured from the copyright owner.
Suggested Citation
Zuellig, R.E., Wahl, C.F., Hennessy, E.K., Jouney, A., and Foutz, P., 2024, Evaluation and review of ecology-focused stream studies to support cooperative monitoring, Fountain Creek Basin, Colorado: U.S. Geological Survey Scientific Investigations Report 2024–5074, 30 p., https://doi.org/10.3133/sir20245074.
ISSN: 2328-0328 (online)
Study Area
Publication type | Report |
---|---|
Publication Subtype | USGS Numbered Series |
Title | Evaluation and review of ecology-focused stream studies to support cooperative monitoring, Fountain Creek Basin, Colorado |
Series title | Scientific Investigations Report |
Series number | 2024-5074 |
DOI | 10.3133/sir20245074 |
Year Published | 2024 |
Language | English |
Publisher | U.S. Geological Survey |
Publisher location | Reston, VA |
Contributing office(s) | Colorado Water Science Center |
Description | Report: vii, 30 p.; Data Release |
Country | United States |
State | Colorado |
Other Geospatial | Fountain Creek Basin |
Online Only (Y/N) | Y |