Three-Dimensional Hydrogeologic Framework Model of the Russian River Watershed, California
Links
- Document: Report (8 MB pdf) , HTML , XML
- Data Release: USGS Data Release - Hydrogeologic data of the Russian River watershed, Sonoma and Mendocino Counties, California (ver. 1.1, July 2023)
- Download citation as: RIS | Dublin Core
Acknowledgments
The authors thank the Sonoma County Water Agency and the California State Water Resources Control Board for their cooperation on this project, including funding, expertise, and logistical support for field visits and data collection and analysis.
The authors thank U.S. Geological Survey colleagues for assistance in data analysis and support, including Claudia Faunt, John Engott, Ayman Alzraiee, Nick Teague, Milissa Peterson, David Dillon, Sarah Roberts, Chris Kohel, Nicole Fenton, and Christiana Rosenberg.
Abstract
The Russian River watershed is in northern Sonoma County and southern Mendocino County, California, in the northern part of the California Coast Ranges. The Russian River serves as a supply for agricultural irrigation and for municipal, domestic, and commercial uses. Through a cooperative agreement with the California State Water Resources Control Board and Sonoma County Water Agency, the U.S. Geological Survey has completed studies to better understand the hydrogeologic system and develop numerical hydrologic modeling tools to evaluate and aid in managing groundwater resources. This report focuses on the development of a digital three-dimensional hydrogeologic framework model of the Russian River watershed for use in groundwater resource assessment and numerical models.
The digital three-dimensional hydrogeologic framework model of the Russian River watershed portrays the altitude, thickness, and extent of five hydrogeologic units. These five hydrogeologic units include (1) a basement unit, (2) the Sonoma Volcanics, (3) a consolidated sedimentary rock unit, (4) an unconsolidated sediment unit, and (5) channel alluvium. Model input data were compiled from published geologic maps, interpreted well data, and a model of the top of basement derived from gravity data. These data were used to construct surfaces that represent the upper and lower subsurface boundaries of each hydrogeologic unit. Top surfaces were created for the five hydrogeologic units and then stacked in three dimensions to create a solid-volume digital model.
The digital three-dimensional hydrogeologic framework model described in this report and the corresponding data represent the generalized geometry of the subsurface geologic units; the model reproduces the input geologic data with reasonable accuracy and is consistent with previously published subsurface conceptualizations of the region. The model indicates the overall geometry of the basement within the watershed and the spatial extent, altitude, and thickness of the basin-filling units. The hydrogeologic framework model is at a scale and resolution appropriate for use as the foundation for a numerical hydrologic model of the study area.
Introduction
The Russian River watershed (fig. 1) is a diverse region of 3,885 square kilometers (km2) of urban, agricultural, and forested lands in northern Sonoma County and southern Mendocino County, California. In this report, “Russian River watershed” refers to the watershed delineated in figure 1 that excludes the Santa Rosa Creek watershed part of the Russian River watershed. The Santa Rosa Creek watershed is part of the Russian River watershed but was excluded from the study area in this report because it was previously studied and modeled (Sweetkind and others, 2010; Nishikawa, 2013; Woolfenden and Nishikawa, 2014).
The primary uses of water in the Russian River watershed include agricultural irrigation, municipal supplies, rural domestic uses that are outside of municipal systems (private wells and mutual water companies), and commercial uses (for example, wineries and recreation). Challenges to managing Russian River watershed water resources include maintaining available flows for fisheries, managing for extreme events due to climate change, flood effects, increasing water-supply demands, decreasing water availability, and streamflow and groundwater-storage depletion.
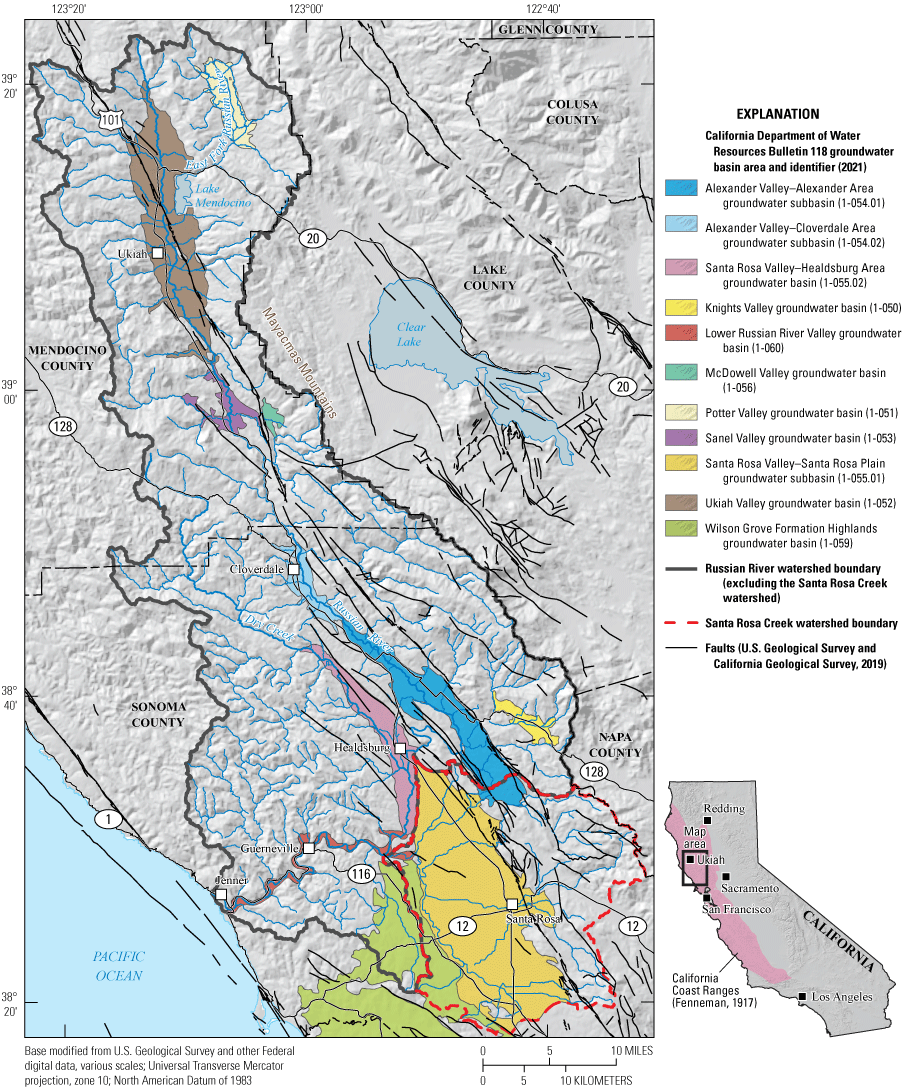
Study area and groundwater basins within the Russian River watershed as defined by the California Department of Water Resources (2021), Sonoma and Mendocino Counties, California.
The Russian River watershed contains all or parts of 10 groundwater basins or groundwater subbasins, as defined by the California Department of Water Resources (fig. 1; California Department of Water Resources, 2021). In most of these basins, water managers rely on a combination of Russian River water and groundwater to meet demand. As of 2023, the California Sustainable Groundwater Management Act (https://water.ca.gov/programs/groundwater-management/sgma-groundwater-management) identified one medium-priority basin, Ukiah Valley groundwater basin (1-052), within the Russian River watershed (fig. 1). As a result of this identification, groundwater sustainability plans were developed by local agencies (Larry Walker Associates and GEI Consultants, 2021; Sonoma County Water Agency, 2021). Management of surface water and groundwater resources in the region requires a knowledge of the groundwater system which, in turn, requires an understanding of the configuration and properties of aquifers. The development of a three-dimensional (3D) hydrogeologic framework model (HFM) for the aquifers of the Russian River watershed will help support the water management efforts.
Purpose and Scope
The U.S. Geological Survey (USGS) was invited by the California State Water Resources Control Board and Sonoma County Water Agency to cooperate on a scientific investigation to evaluate the groundwater resources of the Russian River watershed and to develop tools to better understand and manage the groundwater system. The purpose of this report is to describe the development of a digital 3D HFM of the Russian River watershed. Such a model will help fully conceptualize the 3D subsurface configuration of aquifers and also may be used as the primary geologic input to numerical simulations of the hydrologic system. The 3D HFM, and data used to construct the 3D HFM, can be found in the following data release (Fenton and others, 2022).
Previous Work
Previous geologic mapping investigations of the Russian River watershed and surrounding area include a statewide geologic map (Jennings, 2010), regional geologic maps that cover parts of the watershed (Jennings and Strand, 1960; Koenig, 1963; McNitt, 1968; Wagner and Bortugno, 1982; Blake and others, 2002), and detailed 1:24,000-scale mapping of selected quadrangles (McLaughlin and others, 2008; Delattre and McLaughlin, 2010; Delattre, 2011; Gutierrez and others, 2012).
Cardwell (1965) originally described the hydrogeology of several basins within the Russian River watershed. Using the terminology of California Department of Water Resources (2021), these basins include Lower Russian River Valley (1-060), Potter Valley (1-051), Sanel Valley (1-053), and Ukiah Valley (1-052) groundwater basins and Alexander Valley–Alexander Area (1-054.01), Alexander Valley–Cloverdale Area (1-054.02), and Santa Rosa Valley–Healdsburg Area (1-055.02) groundwater subbasins (fig. 1). The Santa Rosa Valley–Santa Rosa Plain groundwater subbasin (1-055.01), identified by the California Sustainable Groundwater Management Act as a high-priority subbasin, is adjacent to the Russian River watershed (fig. 1) and has been the subject of several previous studies (Cardwell, 1958; Sweetkind and others, 2010; Nishikawa, 2013). Sweetkind and Taylor (2010) compiled lithologic data from water wells in the Russian River watershed and surrounding region from USGS reports. Metzger and others (2006) updated the description of the geohydrology and geochemistry of the Alexander Valley. The California Department of Water Resources (2021) officially defines the boundaries and describes groundwater basins and subbasins within the Russian River watershed.
Despite previous hydrogeologic study of the Russian River watershed, a digital 3D HFM of the watershed has never been constructed. Such a model is needed to fully conceptualize the 3D subsurface configuration of aquifer materials and also may be used as the geologic input to numerical simulation of the hydrologic system.
Hydrogeology of the Russian River Watershed
The Russian River watershed is in the northern part of the California Coast Ranges (Fenneman, 1917; fig. 1, inset). The study area is drained by the Russian River (fig. 1), a principal river in the northern coastal area of California between San Francisco and Ukiah. The Russian River originates in Mendocino County about 26 kilometers (km) north of Ukiah and flows southward for about 145 km through alluvium-filled valleys and mountain gorges to the southern end of the Alexander Valley–Alexander Area groundwater basin (1-054.01) about 7 km east of Healdsburg. At the southern end of the groundwater basin, the river turns abruptly westward, transverse to the topographic and structural grain of the California Coast Ranges and flows to the Pacific Ocean at Jenner. The Russian River watershed is about 135 km long from north to south, but because of sinuosity, the river is about 175 km long. The valley surrounding the Russian River ranges in width from 20 to 50 km, and the watershed has an area of about 3,845 km2.
The Russian River watershed is bounded by two parallel mountain belts of the California Coast Ranges (fig. 1). The mountains to the east of the Russian River watershed are called the Mayacmas Mountains, which range in altitude from 915 to 1,220 meters (m) above the North American Vertical Datum of 1988 (NAVD 88) along the watershed boundary. The mountains to the west of the Russian River watershed range in altitude from about 610 to 1,825 m above NAVD 88 along the watershed boundary. The altitude of the mountains bordering the Russian River increases slightly from south to north. The altitude of the divide between the Russian River and the Santa Rosa Valley–Healdsburg Area groundwater subbasin (1-055.02) to the west (fig. 1) ranges from 425 to 915 m above NAVD 88.
Cardwell (1965) subdivided the watershed into the “upper Russian River” and “lower Russian River.” The upper Russian River of Cardwell (1965) included Potter Valley (1-051), Ukiah Valley (1-052), and Sanel Valley (1-053) groundwater basins (fig. 1). The lower Russian River of Cardwell (1965) included the Alexander Valley–Alexander Area (1-054.01) and Alexander Valley–Cloverdale Area (1-054.02) groundwater subbasins, the Santa Rosa Valley–Healdsburg Area groundwater subbasin (1-055.02), and the Lower Russian River Valley groundwater basin (1-060; fig. 1). The Wilson Grove Formation Highlands groundwater basin (1-059) was not included in the hydrogeologic characterization of Cardwell (1965).
Geologic Setting
The generally northwest-trending mountain ranges in the northern part of the Coast Ranges in California are underlain by thick, highly deformed, and variably metamorphosed Jurassic and Cretaceous rocks. The principal accumulations of Cenozoic sedimentary rocks, volcanic rocks, and alluvial deposits are in the Potter Valley (1-051) and Ukiah Valley (1-052) groundwater basins and Alexander Valley–Alexander Area (1-054.01) and Santa Rosa Valley–Healdsburg Area (1-055.02) groundwater subbasins (figs. 1, 2). These sedimentary basins are in part the result of transtensional opening related to oblique extension associated with slip along major right-lateral faults, including the Healdsburg Fault and Maacama Fault Zone (McLaughlin and Nilsen, 1982; McLaughlin and others, 2012; fig. 2). These major faults are part of distributed right-lateral offset within the San Andreas Fault system along the tectonic transform boundary in northern California (McLaughlin and others, 2012). The Russian River flows southward through these northwest-trending, alluvial valleys that are separated by basement highs. In the southern part of the watershed, the river turns west and flows through a generally incised canyon to its outlet at the Pacific Ocean (Cardwell, 1965; fig. 1). The correlation between the stratigraphic units identified in the Russian River watershed (described later) and the generalized hydrogeologic units subsequently used to develop the 3D HFM are shown on figure 3.

Geology of the Russian River watershed and vicinity, Sonoma and Mendocino Counties, California. Map modified from Jennings (2010).
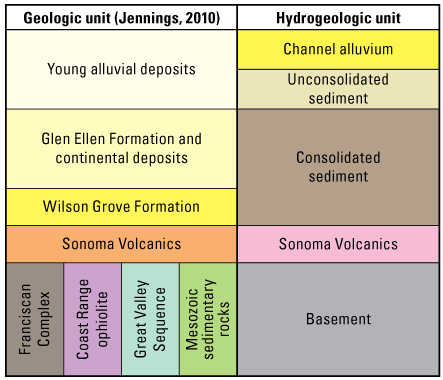
Generalized groupings of mapped geologic units in the Russian River watershed (generalized from Jennings, 2010) and interpreted correlative hydrogeologic units used in this study, Sonoma and Mendocino Counties, California.
Stratigraphic Units
Mesozoic basement rocks are the oldest in the study area and include Jurassic to Upper Cretaceous accretionary rocks of the Franciscan Complex, Jurassic mafic igneous rocks of the Coast Ranges ophiolite, Jurassic to Upper Cretaceous forearc or marginal basin strata of the Great Valley Sequence, and other Mesozoic sedimentary rocks (Wagner and Bortugno, 1982; Blake and others, 2002; McLaughlin and others, 2012; fig. 2). These rock units constitute the basement throughout most of the northern Coast Ranges; they are exposed over approximately 60 percent of the Russian River watershed and are at depth beneath the younger rocks in the study area. Rocks of the Franciscan Complex are a heterogeneous assemblage of highly sheared, folded, and faulted sandstone, graywacke, shale, mélange, conglomerate, chert, greenstone, and serpentinite (Blake and others, 1988; Wakabayashi, 2015). The Coast Ranges ophiolite is comprised of mafic-composition igneous rocks such as gabbro and basalt and ultramafic rocks (Hopson and others, 2008). The Great Valley Sequence is primarily comprised of consolidated mudstone, shale, and sandstone (Ingersoll, 1979). Great Valley Sequence rocks in the southern part of the Russian River watershed include a thick massive conglomerate that provides adequate water for domestic use (Cardwell, 1965; Gutierrez and others, 2012). The unit mapped as Mesozoic sedimentary rocks (fig. 2) is near Sanel Valley groundwater basin (1-053) and is comprised of marine sandstone, shale, and conglomerate (Wagner and Bortugno, 1982; Jennings, 2010).
Neogene to Quaternary sedimentary materials, Neogene volcanic rocks, and young alluvial deposits are the primary basin-fill materials in the elongate northwest-trending basins of the Russian River watershed (Nilsen and Clarke, 1989; Lock and others, 2006; Jennings, 2010; figs. 1, 2). Neogene to Quaternary sedimentary materials consist of non-marine basin-filling sedimentary rocks of the Pliocene-Pleistocene Glen Ellen Formation and “continental deposits” of Cardwell (1965), the Pliocene marine Wilson Grove Formation, and Neogene sedimentary rocks (fig. 2). Neogene volcanic rocks consist of the Sonoma Volcanics (fig. 2). Volcanic rocks mapped as young volcanics, including the Clear Lake volcanics (Wagner and Bortugno, 1982) are outside of the Russian River watershed and are not discussed (fig. 2).
The Glen Ellen Formation and continental deposits are the primary basin-fill materials in the Russian River watershed. In the northern part of the Russian River watershed, the materials are identified as the “continental deposits” of Cardwell (1965). In the southern part of the Russian River watershed, the materials are identified as the Glen Ellen Formation (Lock and others, 2006; Sweetkind and others, 2010; McLaughlin and others, 2012; figs. 2, 3). The Glen Ellen Formation and continental deposits both generally consist of mixtures of clayey gravel and silty sand as poorly consolidated coarse alluvial-fan and fluvial Pliocene to Pleistocene deposits (Cardwell, 1965; Lock and others, 2006; McLaughlin and others, 2012) that crop out locally along the margins of the alluvial valleys. Based on well records, these deposits are tens to hundreds of meters thick (Cardwell, 1965; California Department of Water Resources, 1983). An exploration borehole for a dam site near the City of Ukiah penetrated as much as 450 m of gravel deposits (Treasher, 1955). These basin materials generally rest unconformably on or are in fault contact with basement rocks.
The Wilson Grove Formation crops out in the Wilson Grove Formation Highlands groundwater basin (1-059) in the southern part of the Russian River watershed (figs. 1, 2; Cardwell, 1958; Sweetkind and others, 2010; Jennings, 2010). The unit is late Miocene and Pliocene and consists of consolidated to weakly consolidated marine deposits of massive or thick-bedded, fine-grained to very fine-grained, fossiliferous sand or sandstone (Sweetkind and others, 2010). Well logs from the Santa Rosa Valley–Santa Rosa Plain groundwater subbasin (1-055.01; fig. 1) indicate that the Wilson Grove Formation is as thick as 300 m (Sweetkind and others, 2010). In the Russian River watershed, the unit likely rests unconformably on basement rocks.
The Sonoma Volcanics crop out along the margins of Alexander Valley–Alexander Area groundwater subbasin (1-054.01) and in the southeastern part of the Russian River watershed adjacent to Knights Valley groundwater basin (1-050; figs. 1, 2). The Sonoma Volcanics are a heterogeneous suite of rocks (Wagner and Bortugno, 1982; Blake and others, 2002; McLaughlin and others, 2008) and include a variety of volcanic rock types, such as lava flows that range in composition from basalt to dacite to rhyolite, pyroclastic flow deposits, nonwelded tuff and tephra, and brecciated volcanic rocks that may be flow breccias and avalanche breccias. Volcanic rocks and deposits of the Sonoma Volcanics are interbedded with sedimentary formations, such as the Glen Ellen Formation.
Neogene sedimentary rocks in the Russian River watershed not associated with the continental deposits, Glen Ellen Formation, or Sonoma Volcanics crop out east of the City of Cloverdale and are not in a designated groundwater basin (figs. 1, 2). These rocks are Pliocene marine sedimentary rocks, mostly moderately consolidated, and consist of sandstone, siltstone, shale, and conglomerate (Jennings, 2010).
Young alluvial deposits in the Russian River watershed (figs. 2, 3) include Holocene unconsolidated alluvial channel deposits along the active channel of the Russian River and its tributaries, Pleistocene terrace deposits, and Pleistocene to Holocene dissected alluvium. The deposits consist of stream-channel, flood plain, and alluvial-fan deposits, consisting largely of poorly sorted silty gravel and sand (Cardwell, 1965). The deposits vary from 6 to 60 m in depth and are the main water-bearing strata in the Russian River watershed (Cardwell, 1965; California Department of Water Resources, 1983).
Faults
Mountain uplifts and alluvial valleys in the northern Coast Ranges trend northwestward, which is parallel to the major structural features of the region (Cardwell, 1965; Page, 1966). Major faults in the Russian River watershed that are part of the San Andreas Fault system include the Healdsburg Fault and Maacama Fault Zone (fig. 2). The right-lateral Healdsburg Fault, and associated faults, may be traced to the southeast to San Pablo Bay (the northeastern part of the San Francisco Bay; not shown), where it steps southwest and is linked to the right-lateral Hayward Fault in the eastern San Francisco Bay area (not shown; McLaughlin and others, 2012; Watt and others, 2016). Jurassic and Cretaceous basement uplifted on the east side of the Healdsburg Fault separates the Alexander Valley groundwater subbasins (1-054.01 and 1-054.02) from the Santa Rosa Valley–Healdsburg Area groundwater subbasin (1-055.02; Gutierrez and others, 2012).
The Maacama Fault Zone is a north-northwest trending, right-lateral fault that extends from Sonoma County northward into Mendocino County (Upp, 1989; McLaughlin and others, 2012; Prentice and others, 2014). The Maacama Fault Zone is mapped as a series of en echelon segments that bound the east side of Alexander Valley groundwater subbasins (1-054.01 and 1-054.02), cross the Sanel Valley groundwater basin (1-053), and transect the Ukiah Valley groundwater basin (1-052; figs. 1, 2). Sedimentary basins are aligned broadly subparallel to the Maacama Fault Zone (fig. 2) and may be generally related to oblique pull-apart extension between en echelon and branching splays of this overall dextral-slip fault zone. Although in some basins, such as in Ukiah Valley groundwater basin (1-052), the connection between sedimentation and faulting is more complex (McLaughlin and Nilsen, 1982; Nilsen and Clarke, 1989).
Gravity-Derived Basin Depth and Geometry
Basin depth and geometry were examined using inversion of gravity data controlled by surface geology (Jachens and Moring, 1990) that were modified to include borehole constraints. Gravity data and methods and the resulting basin depth and geometry are discussed in this section.
Gravity Data and Methods
Gravity data were previously used to model the thickness of basin deposits in the Santa Rosa Valley–Santa Rosa Plain groundwater subbasin (1-055.01) and part of the Alexander Valley–Alexander Area groundwater subbasin (1-054.01) in the southern part of the Russian River watershed (McPhee and others, 2007; Langenheim and others, 2010) and in Potter Valley groundwater basin (1-051) in the northeast part of the Russian River watershed (Langenheim and others, 2007). This study expands and builds on those previous efforts by incorporating new gravity measurements that augment sparse, regional gravity data and subsurface depth constraints. The gravity data were inverted to create a basin thickness map for the study area.
Existing data (Chapman and Bishop, 1974, 1978; Langenheim and others, 2006, 2007) were combined with more than 450 new gravity measurements that were collected near the Sanel Valley (1-053) and Ukiah Valley (1-052) groundwater basins and the Alexander Valley–Cloverdale Area groundwater subbasin (1-054.02). Gravity stations were non-uniformly distributed in the Russian River watershed. Station spacing was, on average, one station per 4 km2, although the station spacing was as low as one station per 10 km2 within parts of the upland areas outside of the basins. Although there were several detailed gravity profiles collected previously in the area near the Ukiah Valley groundwater basin (1-052; Erickson, 2008), most of the measurements have poor elevation control, resulting in correspondingly inaccurate gravity anomalies and were not used in this study.
New gravity data were tied to base station CH66 in the City of Ukiah (Chapman, 1966), with an observed gravity value of 980,018.55 milligals (mGals). The datum of observed gravity is the International Gravity Standardization Net as described by Morelli (1974). Elevations were obtained using either a Trimble Real Time Kinematic Model 4400 or Trimble Geo 7 Global Positioning System receivers, with a vertical precision of 0.03–0.3 m.
Gravity data were reduced to free-air anomalies using standard formulas (Telford and others, 1990). Bouguer, curvature, and terrain adjustments to a radial distance of 166.7 km were applied to the free-air anomaly at each station to determine the complete Bouguer anomalies at a standard crustal reduction density of 2,670 kilograms per cubic meter (kg/m3; Plouff, 1977). An isostatic adjustment was then applied to remove the long-wavelength effects of masses in the deep crust or upper mantle that isostatically support regional topography. The isostatic adjustment assumes an Airy-Heiskanen model (Heiskanen and Vening-Meinesz, 1958) of isostatic compensation. Compensation is achieved by varying the depth of the model crust-mantle interface, using the following parameters: a sea-level crustal thickness of 25 km, a crust-mantle density contrast of 400 kg/m3, and a crustal density of 2,670 kg/m3 for the topographic load. The resulting isostatic gravity anomalies should indicate density variations in the upper to middle crust (fig. 4). In general, the upland areas are characterized by higher gravity values than in the lowland areas.
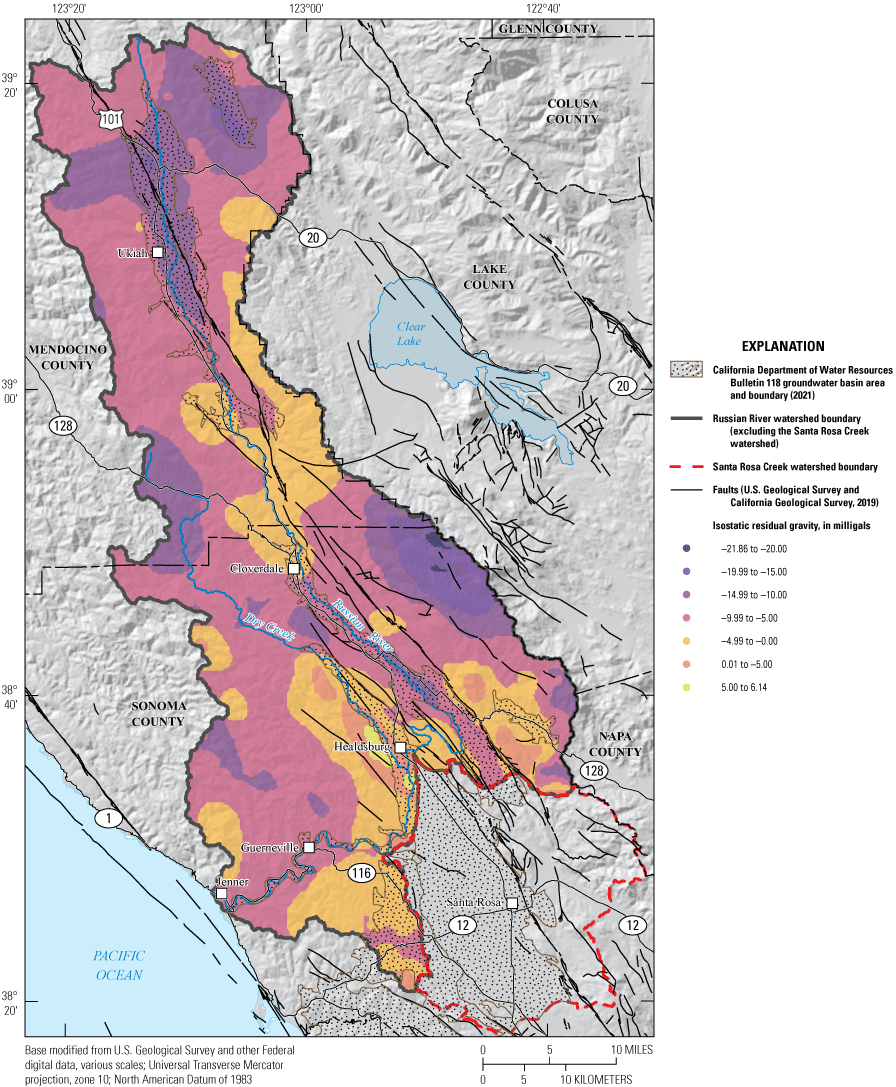
Isostatic residual gravity anomalies (Fenton and others, 2022) of the Russian River watershed, Sonoma and Mendocino Counties, California.
Basin Depth and Geometry
The gravity inversion takes advantage of the large density contrast between dense Mesozoic rocks (primarily the Franciscan Complex and Coast Ranges ophiolite) and lighter Cenozoic sedimentary rocks, volcanic rocks, and alluvial deposits, as measured in a handful of density logs in oil wells in the southern part of the study area (Brocher, 2005). However, variations in the lithology and density of basement rocks resulted in gravity values measured on basement that vary by as much as 20 mGals throughout the Russian River watershed, in places exceeding the amplitude of gravity lows in the valley areas. Such a large variation required removal of a regional field that accounts for variations in basement density, which was done by gridding only those measurements on basement to make an initial estimate of the basement gravity field. That initial basement gravity field was then subtracted from the observed gravity field to produce that component of the gravity field caused by variations in basin thickness (basin gravity). An estimate of the thickness of Cenozoic sedimentary rocks, volcanic rocks, and alluvial deposits was then calculated by application of a density-depth relation to the basin gravity field. The density-depth relation used in Langenheim and others (2010) was used for this study.
The calculated thicknesses of the basin fill deposits, where drill holes were interpreted to have penetrated the basin-fill thickness, equaled the observed thicknesses within an average of about 10 m, which was expected because the model solution was constrained to these values. Basic uncertainties in the gravity data indicate that 15–30 m is likely the best resolution that can be expected in areas of good gravity coverage. Resolution is likely to be less accurate in areas of poor gravity coverage or in areas far from either basement outcrops or wells that drilled into basement. In addition, the calculations were done for grid cells that were 300 by 300 m; therefore, the results represent average thickness of basin fill for each cell, and variations of the basin-fill thickness over distances less than 300 m were not resolved. Furthermore, gravity data indicate the average shape of the basin, with averaging becoming more pronounced where the basin is deeper and farther from the gravity measurement at the land surface. As a result, places where basin fill is thickest are subject to averaging a greater volume of materials; thus, contours appear smoother in these areas than in areas where the basin fill is thinner. Finally, the model assumes no lateral variations in density of the basin fill, which may not be representative if the deposits coarsen toward the uplands.
The model illustrates that the Russian River watershed is underlain by three main structural basins (fig. 5): (1) an elongate basin underlying Ukiah Valley groundwater basin (1-052), (2) another elongate basin underlying the Alexander Valley groundwater subbasins (1-054.01 and 1-054.02), and (3) a deep basin underlying the southern end of the Santa Rosa Valley–Healdsburg Area groundwater subbasin (1-055.02). Smaller structural basins underlie the smaller groundwater basins in the Russian River watershed. Excluding the smaller basin underlying Potter Valley groundwater basin (1-051), all smaller underlying basins are separated by shallow basement ridges that are in part associated with major right-lateral strike-slip faults.
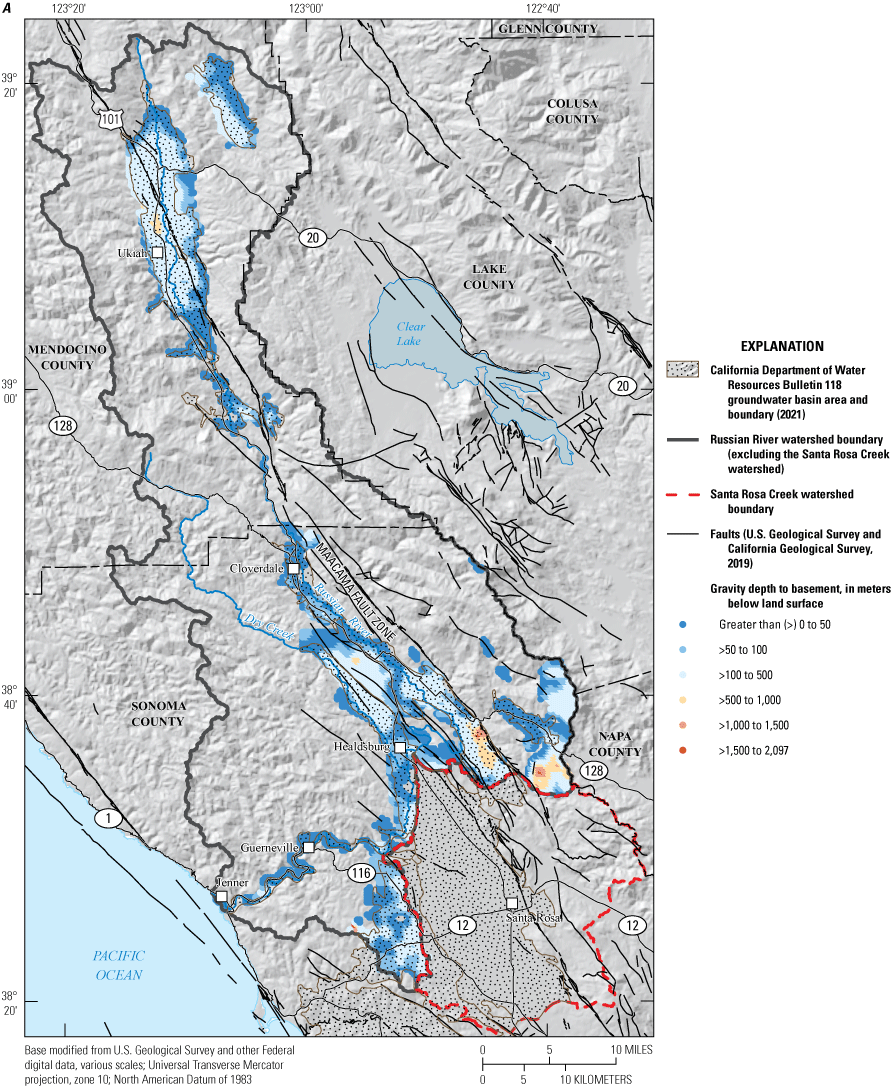
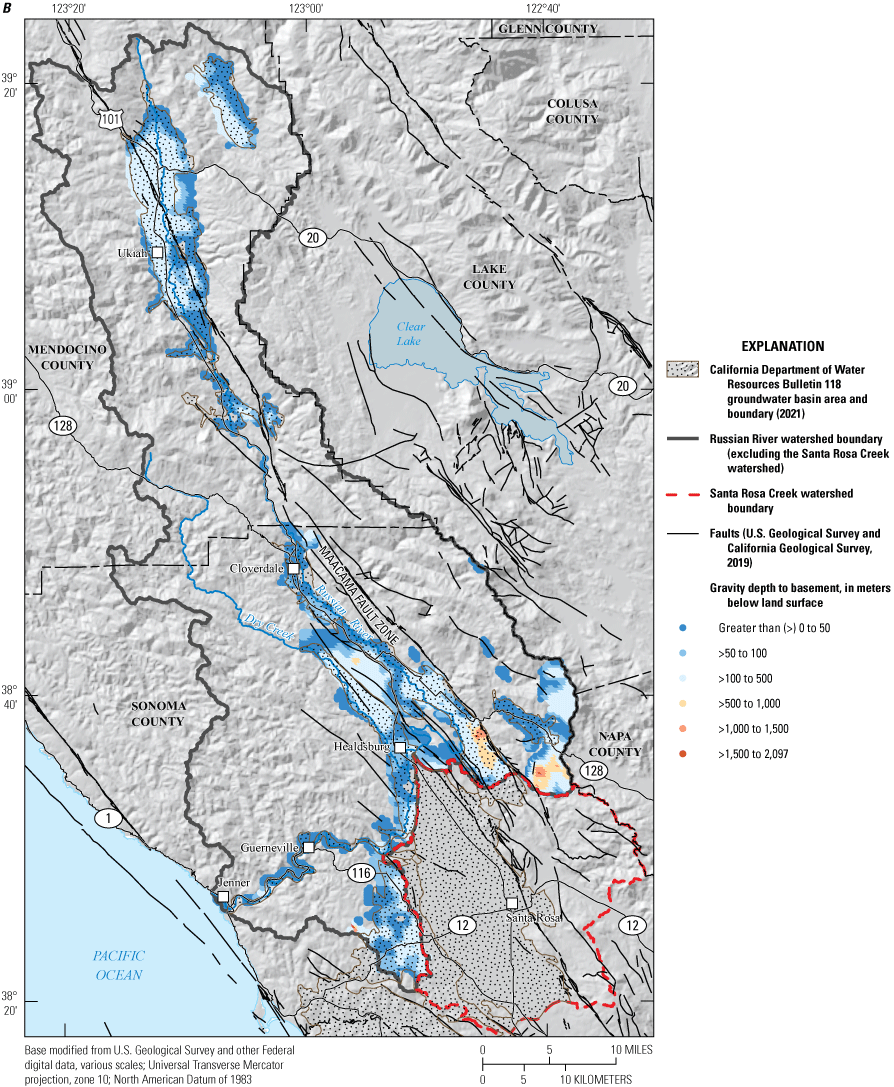
Modeled depth to basement derived from inversion of gravity data (Fenton and others, 2022) of the Russian River watershed, Sonoma and Mendocino Counties, California. The two inversions are identical except in the Ukiah Valley groundwater basin (1-052), where a small, isolated basement outcrop is modeled as A, not rooted in low-density basement; and B, rooted in low-density basement.
Ukiah Valley groundwater basin (1-052) coincides with an elongate 10-mGal gravity minimum, representing thicker basin fill that is bisected by the Maacama Fault Zone (figs. 2, 5). The Maacama Fault Zone transects the gravity minimum, and the thickest fill forms a linear trough on the western side of the groundwater basin that coincides with the Maacama Fault Zone (only on its southeastern margin; fig. 5). This geometry indicates that the Ukiah Valley groundwater basin (1-052) is only partly related to the active fault traces that are superimposed on a previously formed basin of uncertain origin. The basin fill beneath the Ukiah Valley groundwater basin (1-052) is modeled to be as thick as 577 m if a small, isolated basement outcrop that lies within the Maacama Fault Zone is not rooted into underlying low-density basement (fig. 5A). The basin fill is modeled to be as thick as 454 m if it is rooted into underlying low-density basement (fig. 5B).
The area around the McDowell Valley (1-056) and Sanel Valley (1-053) groundwater basins coincides with a small rhomboid gravity minimum (figs. 2, 5). The gravity minimum is modeled as a small northwest-trending basin, with sedimentary fill locally as much as 250 m in thickness that is transected by a slight gravity ridge coincident with the Maacama Fault Zone (fig. 5). To the south, valley fill in the Alexander Valley–Cloverdale Area groundwater subbasin (1-054.02) is no more than 100–150 m thick. Gravity variations in the Alexander Valley–Cloverdale Area groundwater subbasin (1-054.02) mainly coincide with variations in the density of the Franciscan Complex basement. The Alexander Valley–Alexander Area groundwater subbasin (1-054.01) is underlain by an elongate 15-mGal gravity minimum that is proximal to the Maacama Fault Zone and represents a combination of thicker basin fill and less dense basement (figs. 2, 5). The margins of these gravity minimums do not always coincide with the active traces of the Maacama Fault Zone. The Alexander Valley–Alexander Area groundwater subbasin (1-054.01) is as much as 1,500 m thick, and the northeastern margin of the area is parallel to the Maacama Fault Zone (fig. 5).
Hydrogeologic Conceptualization of the Russian River Watershed
For the purposes of the 3D HFM, the mapped stratigraphic units were simplified into five hydrogeologic units (HGUs) based on provenance and hydrologic characteristics (figs. 2, 3, 6): (1) basement—grouping all deformed, consolidated Jurassic and Cretaceous rocks, including the Franciscan Complex, the Great Valley Sequence, the Coast Ranges ophiolite, and undifferentiated Mesozoic sedimentary rocks; (2) Sonoma Volcanics—volcanic and volcaniclastic rocks of the Sonoma Volcanics; (3) consolidated sediment—all consolidated continental and marine sedimentary rocks, including the marine sandstones of the Wilson Grove Formation, the Glen Ellen Formation, and the continental deposits of Cardwell (1965); (4) unconsolidated sediment—Quaternary alluvial and terrace deposits; and (5) channel alluvium—Holocene unconsolidated alluvium along the Russian River and its tributaries. Geologic units from the state geologic map (Jennings, 2010) were grouped to represent the HGUs and were used to determine the surface extent of each unit (fig. 6).
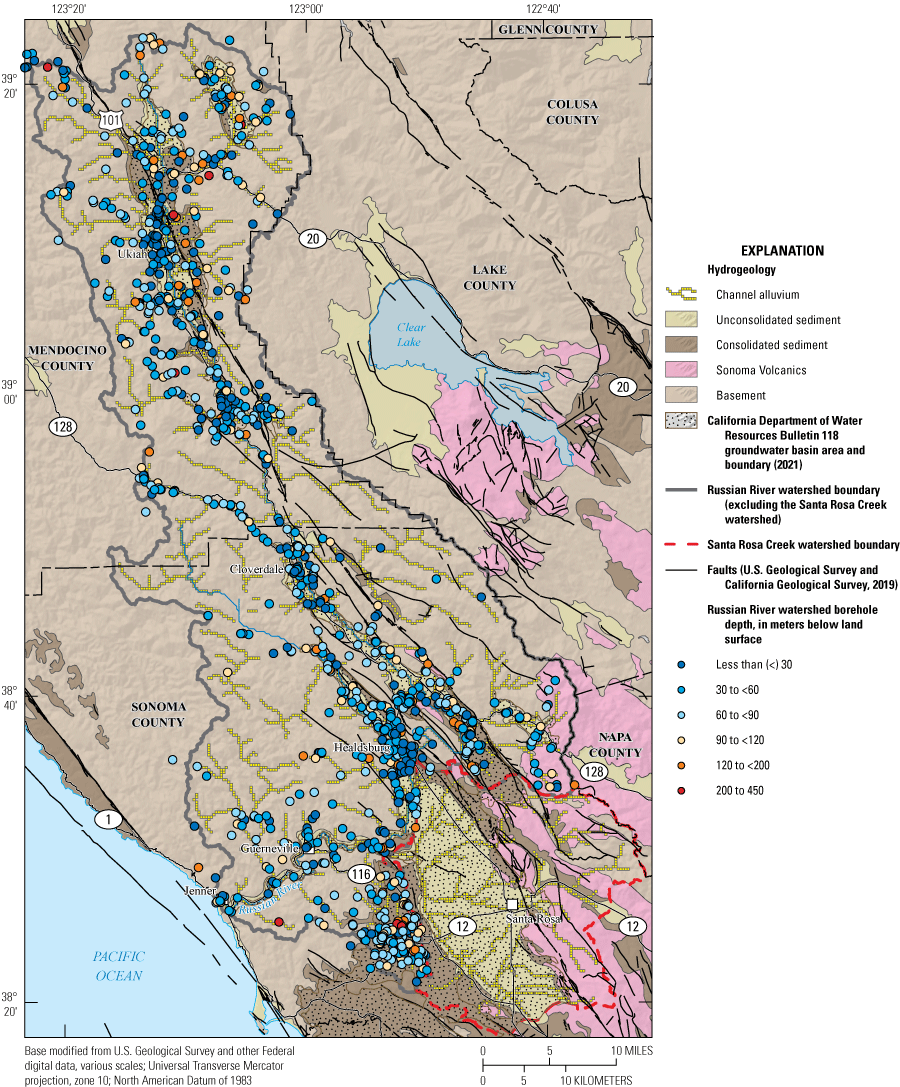
Hydrogeology of the Russian River watershed and vicinity showing borehole depths (Sweetkind and others, 2010; Fenton and others, 2022), Sonoma and Mendocino Counties, California. Map modified from Jennings (2010).
The basement HGU forms the basal hydrogeologic structure on which the overlying basin-fill materials are deposited. This unit bounds and divides each of the groundwater basins and, outside of the basins, is incised by the Russian River and its tributaries. The Sonoma Volcanics HGU primarily crops out east of Alexander Valley–Alexander Area groundwater subbasin (1-054.01) and is likely present at depth in both Alexander Valley–Alexander Area and Cloverdale Area groundwater subbasins (1-054.01 and 1-054.02) and in the Santa Rosa Valley–Healdsburg Area groundwater subbasin (1-055.02). In these groundwater basins, the HGU interfingers with consolidated sediments at depth, as observed in outcrops. The boundary between the Sonoma Volcanics HGU and the consolidated sediment HGU in the HFM is arbitrary by necessity because of the interfingering nature of these two units in a geologic sense and the contribution of volcanic detritus shed from the volcanic rocks to the basin fill. Volcanic materials are not expected to be in the Russian River watershed outside of these areas, except as thin ash beds. The consolidated sediment HGU occurs in outcrop or at depth in all groundwater basins, except for the Lower Russian River Valley groundwater basin (1-060). The consolidated sediment HGU also occurs in the adjacent Wilson Grove Formation Highlands groundwater basin (1-059). These sediments are the deepest basin-filling HGU in each groundwater basin, except where the sediments interfinger with the Sonoma Volcanics HGU in the Alexander Valley groundwater subbasins (1-054.01 and 1-054.02) and Santa Rosa Valley–Healdsburg Area groundwater subbasin (1-055.02). The unconsolidated sediment HGU is widespread across the Russian River watershed and may directly overlie each of the lower HGUs, depending on location. The channel alluvium HGU is limited to the active Russian River mainstem and its tributaries and is incised into the underlying HGUs.
Hydrogeologic Framework Model
The 3D HFM of the Russian River watershed was developed to define the thickness and subsurface extent of the HGUs and to better evaluate the effect of local geology and structures on the hydrologic system. The 3D HFM uses geologic and hydrologic data to inform a quantitative representation of the surface and subsurface extent of consolidated and unconsolidated rocks and structures within each of the 10 groundwater basins. The 3D HFM is a digital model where the hydrogeologic data can be visualized and queried within the 3D volume at any chosen location and orientation. Surface and subsurface data from multiple sources were used as input to the interpolation and modeling process that resulted in the 3D HFM. Input data define the altitude of the top and base, thickness, and spatial extent of each modeled HGU at specific points.
Data Compilation
Digital elevation data for the study area consist of USGS seamless 1:24,000-scale National Elevation Data digital elevation models (DEMs; U.S. Geological Survey, 2019). Data were assigned a projected coordinate system of Universal Transverse Mercator, zone 10, North American Datum of 1983, with a grid cell spacing of about 10 m. DEM data were used to define the upper surface of the HGUs and add elevations to points generated from surface-geological data. Elevation data were referenced to NAVD 88.
Geologic map data were compiled from the digital 1:750,000 scale Geologic Map of California (Jennings, 2010). Supporting geologic map data were collected from Cardwell (1965), Blake and others (2002), and McLaughlin and others (2012). Surface fault traces were compiled from these geologic maps and the U.S. Geological Survey and California Geological Survey (2019). In a geographic information system (GIS; Esri ArcGIS version 10.7.1), points were digitized from the geologic map representing the stratigraphic top of HGUs mapped at land surface. The x and y coordinate of each point on a geologic contact representing the stratigraphic top of HGUs was assigned in GIS using the projected coordinates on the map. The z coordinate elevation was obtained from the 10-m DEM data. A series of files were exported that contained x, y, and z coordinates for mapped contacts of the top of each HGU to be modeled.
Geologic and hydrologic information were collected from 900 wells (Fenton and others, 2022), including driller’s lithologic descriptions, well-construction details, geophysical logs, and specific capacity data. Driller’s lithologic descriptions and well-construction details from 215 wells (Sweetkind and others, 2010) were also compiled from the Knights Valley (1-050) and Lower Russian River (1-060) groundwater basins, and the Alexander Valley–Alexander Area (1-054.01) and Santa Rosa Valley–Healdsburg Area (1-055.02) groundwater subbasins. All boreholes were assumed to be vertical. All HGU contacts from well information were assigned the x and y coordinates of the well’s surface location. Hydrogeologic unit contacts, originally compiled as depth below land surface from the source data, were converted to altitude values by subtracting measured depth from the land-surface altitude at the well using the 10-m DEM data. This calculation using land-surface altitude introduces a small amount of uncertainty into the altitudes of the subsurface unit contacts, because downhole data in a drill hole are usually referenced to a datum above land surface. Tabulating the elevation of the actual measuring point for each well was beyond the scope of this study, and no attempt was made to tabulate the original elevation datums used in depth measurements for each well.
Well driller’s lithologic descriptions of downhole intervals were regularized to a small number of internally consistent lithologic classes using methodology developed for other studies in northern and central California (Sweetkind and others, 2010; Cromwell and others, 2022). Lithology descriptions were interpreted from well drillers’ logs by determining a dominant lithology and then adding additional modifying descriptors for grain size, qualifiers, and color. When numerous drillers’ lithologic logs were viewed and interpreted together, the HGUs were confidently identified and mapped in the subsurface. Mappable HGU tops were identified in well data by stratigraphic interpretations based on rock type, bedding and sorting characteristics, stratigraphic succession, and an understanding of the relations among mapped geologic units and their lithologic characteristics. Well data are published in Sweetkind and others (2010) and Fenton and others (2022).
Input Data and Hydrogeologic Unit Interpretation
Borehole geologic information was used in conjunction with geologic map data to evaluate the subsurface thickness and extent of the sedimentary HGUs, except for the top of the basement unit, which was defined by the depth to basement model from gravity data (fig. 5). Geologic maps were used to define the surface exposure of each HGU (figs. 2, 6), except for the channel alluvium unit. The surface extent of the channel alluvium is defined by the Russian River watershed active stream network, where young, unconsolidated channel materials are likely to be present. Subsurface HGU tops and thicknesses for all sedimentary units were interpreted from well drillers' lithology logs. Boreholes spudded in mapped hydrogeologic outcrops were interpreted first to evaluate the range of lithologies certain to be found in each unit. After this initial work, HGU tops and thicknesses were interpreted from all other boreholes based on general unit characteristics and the conceptual understanding of Russian River watershed geology and structure. The resulting effort produced a collection of HGU-top altitude and thickness points that were formatted as input data for the 3D HFM. Typical lithologic descriptions from well drillers’ logs that were classified as each of the basin-fill HGUs:
-
● Sonoma Volcanics: ash and pumiceous tuff, other volcanic rocks
-
● Consolidated sediment:
-
○ Alexander Valley–Alexander Area groundwater subbasin (1-054.01), Alexander Valley–Cloverdale Area groundwater subbasin (1-054.02), and Santa Rosa Valley–Healdsburg Area groundwater subbasin (1-055.02)
-
○ Wilson Grove Formation Highlands groundwater basin (1-059)
-
○ Ukiah Valley groundwater basin (1-052) and Potter Valley groundwater basins (1-051)
-
-
● Unconsolidated sediment: clay, clay and gravel, gravel, and cobbles
-
● Channel alluvium: clay, clay and gravel, clay sand and gravel, sand and gravel, gravel, and cobbles
An example cross section through the Alexander Valley–Alexander Area groundwater subbasin (1-054.01; fig. 7) is illustrated with regularized lithology types and HGU interpretations. The unconsolidated sediment HGU is relatively thin and corresponds to mapped outcrops of this unit with a mixture of clay and gravel lithologies. Underlying the unconsolidated sediment HGU in this cross section is the consolidated sediment HGU with interbedded horizons of Sonoma Volcanics. The consolidated sediment HGU is a heterogeneous combination of clays, gravels, and sandstones, with intervals of volcanic rock, and ash and pumiceous tuff that are representative of the Sonoma Volcanics HGU. Interbedding of consolidated sediment and Sonoma Volcanics HGUs observed in borehole data is consistent with the conceptual understanding of the southern Russian River watershed, in particular, Alexander Valley–Area groundwater basin (1-054.01) and Santa Rosa Valley–Healdsburg Area groundwater subbasin (1-055.02).
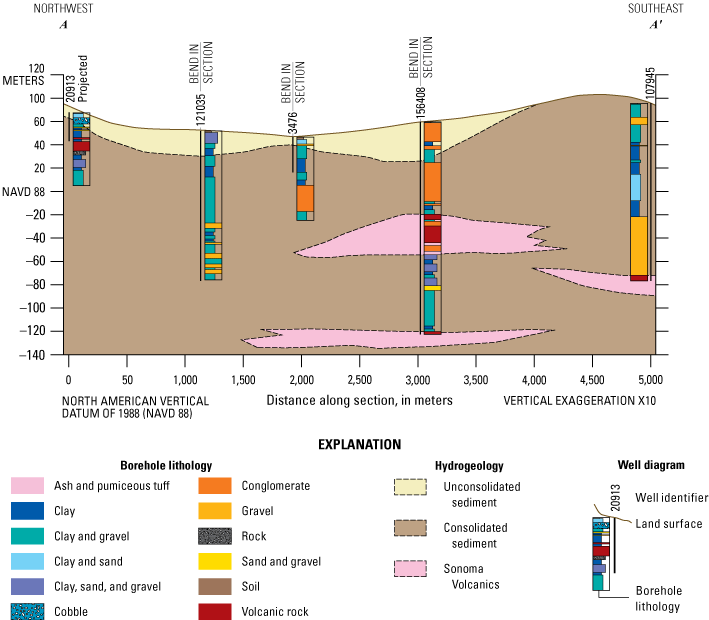
Example cross section through the Alexander Valley–Alexander Area groundwater subbasin (1-1-054.01; shown on fig. 2) showing standardized lithology types and conceptual hydrogeologic interpretations between select boreholes (Sweetkind and others, 2010; Fenton and others, 2022), Russian River watershed, Sonoma and Mendocino Counties, California.
Inversion of gravity data produced two depth-to-basement models that are equivalent in all respects, except in the Ukiah Valley groundwater basin (1-052) where they differ in their treatment of a small, isolated basement outcrop adjacent to the Maacama Fault Zone (fig. 5). The rooted and shallower version of the two depth-to-basement inversions (fig. 5B) was incorporated for use in the 3D HFM to define the basin depth and geometry. The rooted model provides a geologically conservative estimate of basin depth with respect to the emplacement and structure of basement rocks in the Ukiah Valley groundwater basin (1-052). Basin depth estimates from the rooted model were modified to conform to the geologic map of Jennings (2010). Basement outcrops in Jennings (2010) were assigned a basin thickness value of 0 m, and all other depth-to-basement estimates within the sedimentary basins were maintained from the original gravity inversion.
Hydrogeologic Framework Model Construction
To construct the 3D HFM, surface and subsurface hydrogeologic data were compiled as point data from HGU contacts illustrated on geologic maps and from HGU tops interpreted from drillers’ lithologic descriptions. These data were interpolated to generate 3D surface horizons and volumes of each HGU. The 3D HFM used the unrooted, gravity-derived depth-to-basement surface as a lower boundary on the shape and extent of the various groundwater basins and basin-filling HGUs.
Fault data were not included as structural inputs during the digital 3D HFM construction. The Maacama Fault Zone and Healdsburg Fault are major right-lateral strike-slip faults that dissect the Russian River watershed; however, all fault traces except for the Maacama Fault Zone in the Ukiah Valley groundwater basin (1-052) are in basement rocks and do not intersect the shallower HGUs within the groundwater basins. In the Ukiah Valley groundwater basin (1-052), the Maacama Fault Zone was assumed to be a vertical feature, and lateral and vertical offsets of HGUs were assumed to be accounted for in the 3D HFM by the x and y input data. Vertical traces of the Maacama Fault Zone and Healdsburg Fault can be visualized with the digital 3D HFM horizons and can be added as post-process vertical inputs to the 3D HFM in conjunction with numerical groundwater flow arrays.
The 3D HFM was constructed using EarthVision v. 9.0, which is a 3D geologic-modeling software package (Dynamic Graphics, Inc., 2021) that uses a biharmonic, cubic-spline algorithm to produce minimum-tension (minimum-curvature) horizon grids from x, y, z point data. The geologic-modeling software was used to interpolate horizons of the five HGUs determined in this study. Horizontal grid spacing of each unit horizon is 300 m in the x and y horizontal directions. Control points were added to the x, y, z point data of the Sonoma Volcanics unit to enforce conceptual geologic structures. The software package constructs each HGU horizon in stratigraphic order, from oldest (deepest) to youngest (most shallow), with subsequently younger units added on top of older, previously constructed horizons. In this manner, the unit top horizon and unit thickness for each HGU were obtained, with the total thickness of a given HGU defined as the difference between that unit top horizon and the upper surface of the unit below. Any HGUs present within the model volume were given a minimum thickness of 6 m to meet the anticipated computational requirements of a subsequent numerical model. The correlation between geologic units and the HGUs used in the 3D HFM and the stratigraphic stacking order used in the 3D HFM is shown on figure 3. All horizon grids are clipped at the land surface using a discretized DEM grid.
After gridding each modeled HGU, altitude and thickness attributes were mapped to the cell centroid nodes of a polygonal array of grid cells (x and y coordinates) in GIS. The array of nodes for the study area represents the centroids of 35,303 square grid cells that are 300 m in x and y dimensions (Fenton and others, 2022), corresponding to the anticipated computational array of a subsequent numerical hydrologic model. Altitude values were reassigned by sampling the gridded input data at the x and y coordinate locations of each grid node; elevation of the land surface was defined at each grid node by sampling a DEM. Use of the polygonal array within a GIS allowed for manual review and adjustment of HGU altitude and thickness values to ensure consistency of model output with the hydrogeologic map (fig. 6) and with geologic rules for stacking the HGUs. In general, the modeled HGU hydrogeologic horizons appropriately represented input data and provided reasonable interpolations where data were minimal. Some adjustments were required at unit margins where a younger unit pinches out on an existing older unit and other areas where input data were sparse. The gridding algorithm sometimes extrapolated unreasonably high or low values where data are absent or where data indicate abrupt changes in altitude. These unrealistic horizon altitudes were discovered through visual inspection of the data and were edited manually.
Only a limited number of boreholes were spudded in the channel alluvium HGU allowing unit thickness to be explicitly defined by data. As a result, horizon altitudes were manually adjusted within the 3D HFM to assign thickness values to the channel alluvium HGU. In most of the main valley areas, the average thickness of channel alluvium penetrated by wells was 20 m, so this thickness was applied to the channel alluvium HGU in the 3D HFM. Several boreholes in the Lower Russian River Valley groundwater basin (1-060) were interpreted to have penetrated channel alluvium to more than 40 m in thickness. In these areas of the 3D HFM, channel alluvium HGU was assigned this larger thickness value. Few wells provided data on alluvial thickness in small tributary drainages that are incised into basement rocks throughout the study area; therefore, thickness values for these drainages were manually assigned based on regional average unit thickness and location within the Russian River watershed. A digital geospatial database of the final 3D HFM contains the data used to construct the 3D HFM, interpolated altitudes, and interpolated thicknesses of the HGUs as a cellular array (Fenton and others, 2022).
Hydrogeologic Framework Model Results
The 3D HFM quantifies the surface and subsurface extent, altitude, and thickness of five HGUs in the Russian River watershed. The resulting framework can be visualized and queried at any chosen depth or orientation and is appropriate for regional and subregional geologic and hydrogeologic applications. The 3D HFM is visualized as perspective views of the Ukiah Valley groundwater basin (1-052; fig. 8) and the Alexander Valley–Alexander Area (1-054.01) and Santa Rosa Valley–Healdsburg Area (1-055.02) groundwater subbasins (fig. 9).
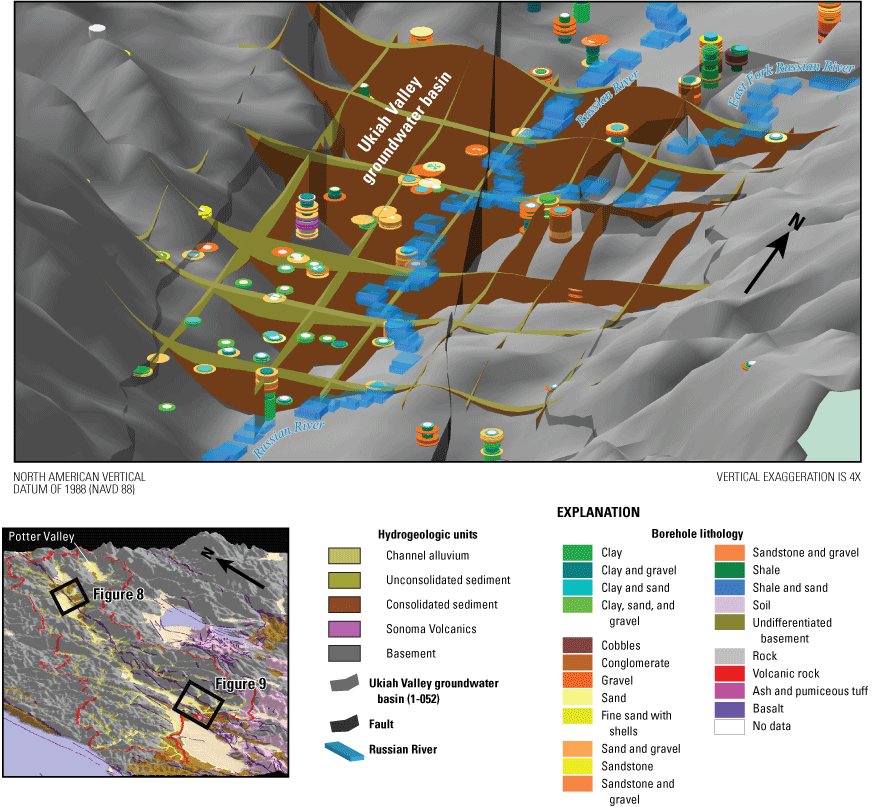
Perspective view of borehole data and vertical sections from the three-dimensional hydrogeologic framework model (Fenton and others, 2022) in the Ukiah Valley groundwater basin (1-052), Sonoma and Mendocino Counties, California. Vertical exaggeration is 4X. Horizontal and vertical scale is variable because of the perspective view.
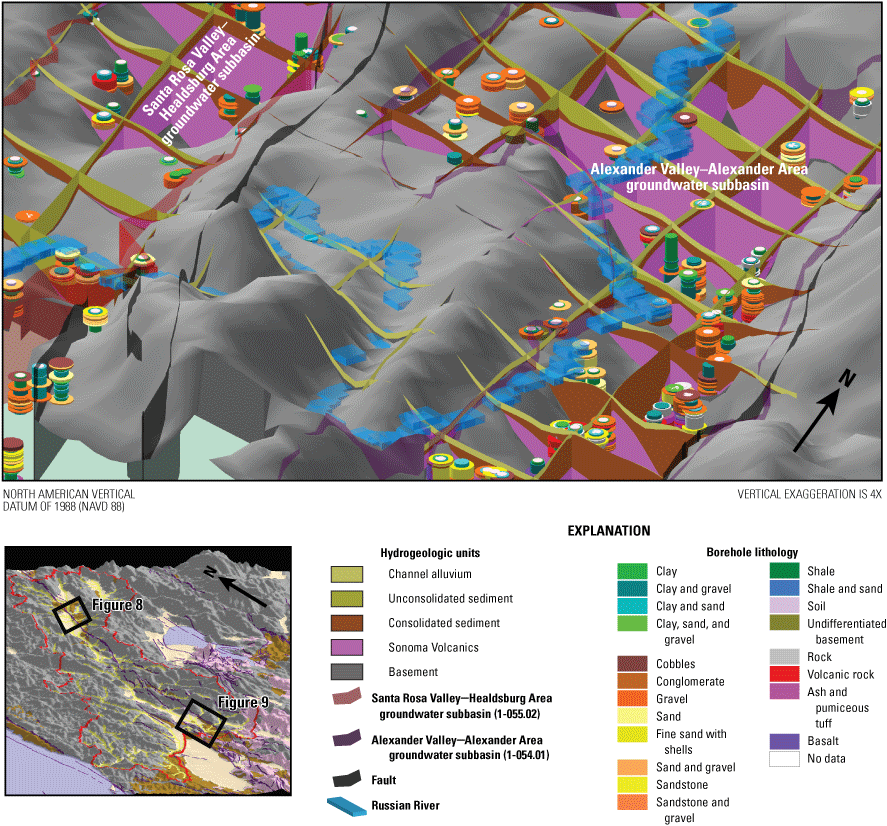
Perspective view of borehole data and vertical sections from the three-dimensional hydrogeologic framework model (Sweetkind and others, 2010; Fenton and others, 2022) in the Alexander Valley–Alexander Area (1-054.01) and Santa Rosa Valley–Healdsburg Area (1-055.02) groundwater subbasins, Sonoma and Mendocino Counties, California. Vertical exaggeration is 4X. Horizontal and vertical scale is variable because of the perspective view.
Due to the method of assignment of channel alluvial thickness, the channel alluvium HGU appears in an unvarying way across the Russian River watershed, largely independent of geography and underlying geology. The HGU is modeled as being present along the active stream network throughout the Russian River watershed and varying in thickness from 20 m to more than 40 m in valley centers (thickest in the northeastern part of the Lower Russian River Valley groundwater basin [1-060] and from 7.2 to 32 m at valley margins). The channel alluvium HGU is modeled to represent active downcutting of streams into underlying HGUs and is accompanied by deposition of unconsolidated channel materials.
In Potter Valley (1-051) and Ukiah Valley (1-052) groundwater basins (fig. 8), a thick interval of the consolidated sediments HGU (as much as 430 m) underlies a relatively thin layer of the unconsolidated sediment’s HGU (less than 100 m), and there are no apparent structural offsets that affect these two units. The thickness of the consolidated sediment HGU is dependent largely on the depth of the basin; the greatest thickness in the Ukiah Valley groundwater basin (1-052) is along the western margin and central part of the basin (fig. 8), coincident with the greatest basin depth (fig. 5A). A similar relation between HGU thickness and basin depth observed in Potter Valley groundwater basin (1-051) indicates that deposition of consolidated sediment was likely coincident with downdrop of both basins. The unconsolidated sediment HGU is relatively thicker in these same deep-basin areas, perhaps indicating syn-deposition with down dropping of the Potter Valley (1-051) and Ukiah Valley (1-052) groundwater basins. The true thickness of the consolidated sediment HGU in the deepest parts of the valley is unknown because borehole data that penetrate through the unit down to the basement HGU are not available. In the Potter Valley (1-051) and Ukiah Valley (1-052) groundwater basins, the channel alluvium HGU is present in the main-stem Russian River (figs. 6, 8) and in adjoining tributaries.
Knights Valley (1-050), Lower Russian River Valley (1-060), McDowell Valley (1-056), and Sanel Valley (1-053) groundwater basins and Alexander Valley–Cloverdale Area groundwater subbasin (1-054.02) are relatively shallow, with basin-fill sediment typically ranging in thickness from 10 to 100 m. The consolidated sediment HGU is present but discontinuous in the Sanel Valley groundwater basin (1-053) and Alexander Valley–Cloverdale Area groundwater subbasin (1-054.02) and is absent in the Knights Valley (1-050), Lower Russian River Valley (1-060), and McDowell Valley (1-056) groundwater basins. Most of the basin fill in these basins is composed of the unconsolidated sediment and channel alluvium HGUs. Along the Russian River and its tributaries, in this central part of the 3D HFM, the channel alluvium HGU commonly directly overlies the basement HGU (figs. 2, 6).
Alexander Valley–Alexander Area (1-054.01) and Santa Rosa Valley–Healdsburg Area (1-055.02) groundwater subbasins are elongated, narrow, deep (as much as 1,500 m thick) basins composed of all four basin-fill HGUs (fig. 9). The two groundwater subbasins are separated from each other by a basement-rock high that is incised by the Russian River (figs. 6, 9). The Sonoma Volcanics HGU is modeled as the basal basin-fill HGU in both basins (10–1,300 m thick) but is not continuous across either basin. The total thickness of the Sonoma Volcanics HGU is unknown because available borehole data do not extend through the deepest part of the basin. The Sonoma Volcanics HGU is modeled as a continuous unit (fig. 9), but some of this unit volume represents an interlayered sequence of volcanic rocks and consolidated sedimentary materials, as shown on figure 7. Stratigraphically above the Sonoma Volcanics, in the 3D HFM, is the consolidated sediment HGU (10–970 m thick), which is laterally extensive across the Alexander Valley–Alexander Area (1-054.01) and Santa Rosa Valley–Healdsburg Area (1-055.02) groundwater subbasins and is unconformable with the basement HGU where the Sonoma Volcanics are absent. The unconsolidated sediment HGU (6–200 m thick) is generally discontinuous across the two basins, and the unit unconformably overlies either the Sonoma Volcanics HGU or the basement HGU in some places. The channel alluvium HGU in this southern part of the 3D HFM is present along the active stream network and cuts through and dissects (especially in shallow basin areas) the underlying HGUs.
The Wilson Grove Formation Highlands groundwater basin (1-059) is delineated by continuous outcrops of the Wilson Grove Formation in the southern part of the Russian River watershed and areas to the south and west of the watershed. In the 3D HFM, the Wilson Grove Formation Highlands groundwater basin (1-059) is modeled with the consolidated sediment HGU overlying the basement HGU, and with the channel alluvium HGU present along active stream channels. The consolidated sediment HGU ranges in thickness from 6 to about 500 m and follows the structural trend of the basement HGU.
Outcrops of Sonoma Volcanics east of the Alexander Valley–Alexander Area groundwater subbasin (1-054.01) are topographically elevated relative to the groundwater basin and extend to the southeast beyond the Russian River watershed boundary. In the 3D HFM, these outcrops consist of the Sonoma Volcanics HGU overlying the basement HGU, with the channel alluvium HGU present along active stream channels. The Sonoma Volcanics HGU ranges in thickness from 6 to about 1,500 m, following the structural trend of the basement HGU.
Aquifer Properties
The primary water-bearing units in the Russian River watershed are the four basin-fill HGUs: channel alluvium, unconsolidated sediment, consolidated sediment, and Sonoma Volcanics. These basin-fill units are underlain by the basement HGU, which serves as the basal unit of the 3D HFM. However, the basement HGU should not be considered hydrologically impermeable. The geologic units composited as the basement HGU include a wide range of lithologic types, not all of which are impermeable. For example, sandstones within the Great Valley Sequence, included in the basement HGU, can supply adequate water for domestic use (Cardwell, 1965). In less permeable lithologies within the basement HGU, small-scale fractures, joints, and faults can provide conduits for transmitting precipitation and runoff into the basement rocks in the subsurface and then laterally into the basin-fill materials.
In the interest of characterizing aquifer properties of the HGUs, specific capacity data from borehole records were compiled and analyzed. Of the 900 boreholes compiled for this study, 513 contained aquifer test information suitable for estimating specific capacity (Fenton and others, 2022). These boreholes were distributed across the Russian River watershed and were spudded in each of the four basin-fill HGUs and the basement HGU. Specific capacity data for each borehole were assigned to the HGUs of the 3D HFM by comparing the reported downhole screened interval in each well to the altitude of HGU tops. Specific capacity values were assigned to a given HGU if at least 60 percent of the screened interval was in that unit, and 448 wells out of 513 met that characteristic (table 1). The distribution of estimated specific capacity data in the Russian River watershed is shown on figure 10, and summary statistics for each HGU are listed in table 1.
Table 1.
Specific capacity estimates for hydrogeologic units in the three-dimensional hydrogeologic framework model (Fenton and others, 2022) of the Russian River watershed, Sonoma and Mendocino Counties, California.[MAD, median absolute deviation]
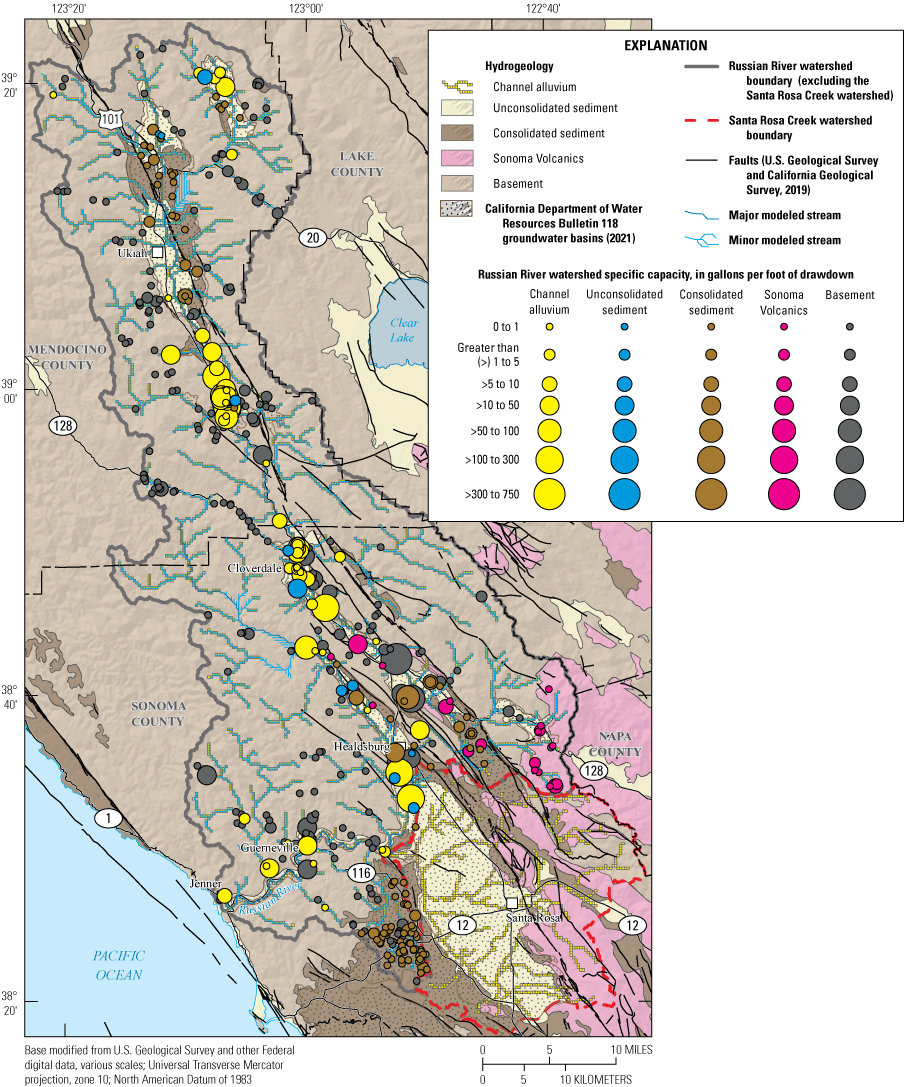
Specific capacity estimates from wells for hydrogeologic units in the three-dimensional hydrogeologic framework model (Fenton and others, 2022) of the Russian River watershed, Sonoma and Mendocino Counties, California.
The channel alluvium and unconsolidated sediment HGUs have the highest specific capacity values of all HGUs. The channel alluvium HGU has a median specific capacity estimate of 5.0 gallons per minute per foot (gpm/ft) of drawdown with a median absolute deviation (MAD) of 4.72 gpm/ft. In general, specific capacity estimates in the channel alluvium HGU are greater than estimates from proximal boreholes in the other HGUs (fig. 10). A cluster of high specific capacity estimates in the Sanel Valley groundwater basin (1-053) and in the Alexander Valley–Cloverdale Area groundwater subbasin (1-054.02) may be correlated to the relatively shallow basin depths and proximity to the main-stem Russian River. Similar high specific capacity values are found along the Russian River in the Santa Rosa Valley–Healdsburg Area groundwater subbasin (1-055.02) and the Lower Russian River Valley groundwater basin (1-060; fig. 10). The unconsolidated sediment HGU has a median specific capacity of 1.34 with a MAD of 1.16 gpm/ft, but there are a limited number of records for this HGU (table 1).
The consolidated sediment, Sonoma Volcanics, and basement HGUs have median specific capacity estimates much less than 1.0 gpm/ft with MADs less than 1.0 (table 1). Some boreholes screened in the basement HGU have substantially larger specific capacity values, but these are generally limited to a few locations in in the upland area between the northwestern parts of Alexander Valley–Alexander Area (1-054.01) and Santa Rosa Valley–Healdsburg Area (1-055.02) groundwater subbasins (fig. 10). Cardwell (1965) reported productive wells drilled in a thick, unnamed, possibly Late Cretaceous, conglomerate in this upland area. Other relatively high specific capacity values for the basement HGU occur in the Lower Russian River Valley groundwater basin (1-060; fig. 10).
Summary
The Russian River watershed is in the northern part of the California Coast Ranges in northern California. The study area is drained by the Russian River and contains all or parts of 10 groundwater basins or subbasins. The Russian River originates in Mendocino County and flows southward for about 145 kilometers through alluvium-filled valleys and mountain gorges to the southern end of the study area in Sonoma County; the river turns abruptly westward, transverse to the topographic and structural grain of the Coast Ranges and flows to the Pacific Ocean. Groundwater basins in the Russian River watershed are northwest-trending, alluvial valleys that are separated by basement highs and are bounded or transected by the northwest-trending Maacama Fault Zone and Healdsburg Fault.
The depths and geometries of groundwater basins were estimated from gravity-derived depth-to-basement inversion models. The models indicate the Russian River watershed is underlain by three main structural basins: (1) an elongate basin underlying the Ukiah Valley groundwater basin (1-052), (2) another elongate basin underlying the Alexander Valley–Alexander Area (1-054.01) and Alexander Valley–Cloverdale Area (1-054.02) groundwater subbasins, and (3) a deep basin underlying the southern end of the Healdsburg Area of Santa Rosa Valley–Healdsburg Area groundwater basin (1-055.02). Smaller structural basins are beneath the other groundwater basins in the Russian River watershed.
Two gravity-derived depth-to-basement inversion models were developed for the Ukiah Valley groundwater basin (1-052). In the first model, a small, isolated basement outcrop within the Maacama Fault Zone is not rooted (unrooted) into underlying low-density basement—the basin fill beneath the groundwater basin is as much as 577 meters (m) thick. In the second model, the basement outcrop is rooted into underlying low-density basement—the basin fill beneath the groundwater basin is as much as 454 m thick. The rooted and shallower version of the two depth-to-basement inversions was incorporated for use in the three-dimensional hydrogeologic framework model to define the basin depth and geometry.
A digital three-dimensional hydrogeologic framework model of the primary hydrogeologic units (HGUs) of the Russian River watershed was developed from geologic, geophysical, and hydrologic data. The primary HGUs from oldest (deepest) to youngest (most shallow) are (1) basement—grouping all deformed, consolidated Jurassic and Cretaceous rocks, including the Franciscan Complex, the Great Valley Sequence, the Coast Ranges ophiolite, and undifferentiated Mesozoic sedimentary rocks; (2) Sonoma Volcanics—volcanic and volcaniclastic rocks of the Sonoma Volcanics; (3) consolidated sediment—all consolidated continental and marine sedimentary rocks, including the marine sandstones of the Wilson Grove Formation, the Glen Ellen Formation, and continental deposits of Cardwell (1965); (4) unconsolidated sediment—Quaternary alluvial and terrace deposits; and (5) channel alluvium—Holocene unconsolidated alluvium along the Russian River and its tributaries.
Top surfaces were created for each HGU and then stacked in three dimensions to create a solid-volume digital model using geologic modeling software. The solid-volume model is internally consistent in that the calculated base of each unit is coincident with the top of the underlying unit, and the thickness of each unit is the difference between the unit top and unit bottom. Major structures and HGU outcrop patterns of this model were generally consistent with published geologic maps and subsurface interpretations.
The hydrogeologic framework model indicates the overall geometry of the basement within the watershed and the spatial extent, altitude, and thickness of basin-filling HGUs in each of the groundwater basins and subbasins. Each of the basin-filling HGUs form important groundwater aquifer units in these groundwater basins. The model is at a scale and resolution appropriate for use as the foundation for a numerical hydrologic model of the study area.
Aquifer properties of each HGU were evaluated from well drillers’ lithologic logs and from specific capacity data. The unconsolidated sediment and channel alluvium HGUs were 1.34 and 5.0 gallons per minute per foot (gpm/ft) of drawdown, respectively. The consolidated sediment, Sonoma Volcanics, and basement HGUs have median specific capacity estimates much less than 1.0 gpm/ft of drawdown.
References Cited
Blake, M.C., Jr., Graymer, R.W., and Stamski, R.E., 2002, Geologic map and map database of western Sonoma, northernmost Marin, and southernmost Mendocino Counties, California: U.S. Geological Survey Miscellaneous Field Studies Map, MF-2402, 43 p., accessed August 4, 2023, at https://pubs.usgs.gov/mf/2002/2402/.
Blake, M.C., Jr., Jayko, A.S., McLaughlin, R.J., and Underwood, M.B., 1988, Metamorphic and tectonic evolution of the Franciscan Complex, northern California, chap. 38 in Ernst, W.G., ed., Metamorphism and crustal evolution of the Western United States: Englewood Cliffs, N.J., Prentice-Hall, p. 1035–1060.
Brocher, T.M., 2005, A regional view of urban sedimentary basins in northern California based on oil industry compressional-wave velocity and density logs: Bulletin of the Seismological Society of America, v. 95, no. 6, p. 2093–2114. [Available at https://doi.org/10.1785/0120050025.]
California Department of Water Resources, 2021, California’s Groundwater Update 2020: California Department of Water Resources Bulletin 118, 485 p., accessed August 4, 2023, at https://data.cnra.ca.gov/dataset/calgw_update2020.
Cardwell, G.T., 1958, Geology and ground water in the Santa Rosa and Petaluma Valley areas, Sonoma County, California: U.S. Geological Survey Water Supply Paper 1427, 273 p. [Available at https://doi.org/10.3133/wsp1427.]
Cardwell, G.T., 1965, Geology and ground water in Russian River valley areas and in Round, Laytonville, and Little Lake Valleys, Sonoma and Mendocino Counties, California: U.S. Geological Survey Water Supply Paper 1548, 154 p., 6 pl. [Available at https://doi.org/10.3133/wsp1548.]
Cromwell, G., Sweetkind, D.S., Densmore, J.N., Engott, J.A., Seymour, W.A., Larsen, J.D., Ely, C.P., Stamos, C.L., and Faunt, C.C., 2022, Hydrogeologic characterization of the San Antonio Creek Valley watershed, Santa Barbara County, California: U.S. Geological Survey Scientific Investigations Report 2022–5001, 124 p., accessed July 23, 2023, at https://doi.org/10.3133/sir20225001.
Delattre, M.P., 2011, Preliminary geologic map of the Healdsburg 7.5’ quadrangle, Sonoma County, California: California Geological Survey, scale 1:24,000, accessed August 4, 2023, at https://www.conservation.ca.gov/cgs/rgm/preliminary.
Delattre, M.P., and McLaughlin, R.J., 2010, Geologic map of the Jimtown 7.5’ quadrangle, Sonoma County, California: California Geological Survey, scale 1:24,000, accessed August 4, 2023, at https://www.conservation.ca.gov/cgs/rgm/preliminary.
Dynamic Graphics, Inc., 2021, Software for 3D modeling and visualization: Dynamic Graphics, Inc. website, accessed November 18, 2021, at https://www.dgi.com/earthvision-software-for-3d-modeling-and-visualization/.
Erickson, G., 2008, Evolution of an intermontane basin along the Maacama Fault, Little Lake Valley, northern California: Arcata, Calif., Humboldt State University, Master’s thesis, 109 p., accessed August 8, 2023, at https://scholarworks.calstate.edu/concern/theses/7d278w28p.
Fenneman N.M., 1917, Physiographic subdivisions of the United States: Processing of the National Academy of Sciences, v. 3, no. 1, p. 17–22, accessed July 15, 2023, at https://doi.org/10.1073/pnas.3.1.17.
Fenton, N.C., Cromwell, G., Sweetkind, D.S., Langenheim, V.E., Ely, C.P., Kohel, C.A., Martin, A.J., Morita, A.Y., Peterson, M.F., and Roberts, M.A., 2022, Hydrogeologic data of the Russian River watershed, Sonoma and Mendocino Counties, California (ver. 1.1, July 2023): U.S. Geological Survey data release, accessed August 4, 2023, at https://doi.org/10.5066/P9GPH5FW.
Gutierrez, C.I., Holland, P.J., and Delattre, M.P., 2012, Preliminary geologic map of the Geyserville 7.5’ quadrangle, Sonoma County, California—A digital database: California Geological Survey, scale 1:24,000, accessed August 4, 2023, at https://www.conservation.ca.gov/cgs/rgm/preliminary.
Hopson, C.A., Mattinson, J.M., Pessagno, E.A., Jr., and Luyendyk, B.P., 2008, California Coast Range ophiolite—Composite Middle and Late Jurassic oceanic lithosphere, in Wright, J.E., and Shervais, J.W., eds., Ophiolites, arcs, and batholiths—A tribute to Cliff Hopson: Geological Society of America Special Paper, v. 438, p. 1–101. [Available at https://doi.org/10.1130/2008.2438(01).]
Ingersoll, R.V., 1979, Evolution of the Late Cretaceous forearc basin, northern and central California: Geological Society of America Bulletin, v. 90, no. 9, p. 813–826, accessed March 5, 2024, at https://doi.org/10.1130/0016-7606(1979)90<813:EOTLCF>2.0.CO;2.
Jachens, R.C., and Moring, B.C., 1990, Maps of the thickness of Cenozoic deposits and the isostatic residual gravity over basement for Nevada: U.S. Geological Survey Open-File Report 90–404, 15 p., 2 pl. [Available at https://doi.org/10.3133/ofr90404.]
Jennings, C.W., with modifications by Gutierrez, C., Bryant, W., Saucedo, G., and Wills, C., 2010, 2010 Geologic map of California: California Geological Survey Geologic Data Map 2, scale 1:750,000, 13 p., 1 pl., accessed July 29, 2019, at https://www.conservation.ca.gov/cgs/publications/gmc.
Jennings, C.W., and Strand, R.G., 1960, Geologic map of California—Ukiah Sheet: California Division of Mines and Geology, scale 1:250,000, accessed August 5, 2023, at https://www.conservation.ca.gov/cgs/Documents/Publications/Geologic-Atlas-Maps/GAM_24-Ukiah-1960-Map.pdf.
Koenig, J.B., 1963, Geologic map of California: Santa Rosa Sheet: California Division of Mines and Geology, scale 1:250,000, accessed August 5, 2023, at https://www.conservation.ca.gov/cgs/Documents/Publications/Geologic-Atlas-Maps/GAM_22-SantaRosa-1963-Map.pdf.
Langenheim, V.E., Graymer, R.W., Jachens, R.C., McLaughlin, R.J., Wagner, D.L., and Sweetkind, D.S., 2010, Geophysical framework of the northern San Francisco Bay region, California: Geosphere, v. 6, no. 5, p. 594–620. [Available at https://doi.org/10.1130/GES00510.1.]
Langenheim, V.E., Jachens, R.C., Morin, R.L., and McCabe, C.A., 2007, Preliminary gravity and magnetic data of the Lake Pillsbury region, northern Coast Ranges, California: U.S. Geological Survey Open-File Report 2007–1368, 24 p. [Available at https://doi.org/10.3133/ofr20071368.]
Langenheim, V.E., Roberts, C.W., McCabe, C.A., McPhee, D.K., Tilden, J.E., and Jachens, R.C., 2006, Preliminary isostatic gravity map of the Sonoma volcanic field and vicinity, Sonoma and Napa Counties, California: U.S. Geological Survey Open-File Report 2006–1056, 1 pl. [Available at https://doi.org/10.3133/ofr20061056.]
Larry Walker Associates and GEI Consultants, 2021, Groundwater Sustainability Plan: Ukiah Valley Basin Groundwater Sustainability Agency, accessed September 29, 2023, at https://ukiahvalleygroundwater.org/managing-our-groundwater/groundwater-sustainability-plan/.
Lock, J., Kelsey, H., Furlong, K. and Woolace, A., 2006, Late Neogene and Quaternary landscape evolution of the northern California Coast Ranges—Evidence for Mendocino triple junction tectonics: Geological Society of America Bulletin, v. 118, nos., 9–10, p. 1232–1246. [Available at https://doi.org/10.1130/B25885.1.]
McLaughlin, R.J., and Nilsen, T.H., 1982, Neogene non-marine sedimentation and tectonics in small pull-apart basins of the San Andreas Fault system, Sonoma County, California: Sedimentology, v. 29, no. 6, p. 865–876. [Available at https://doi.org/10.1111/j.1365-3091.1982.tb00089.x.]
McLaughlin, R.J., Langenheim, V.E., Sarna-Wojcicki, A.M., Fleck, R.J., McPhee, D.K., Roberts, C.W., McCabe, C.A., and Wan, E., 2008, Geologic and geophysical framework of the Santa Rosa 7.5′ quadrangle, Sonoma County, California: U.S. Geological Survey Open-File Report 2008–1009, p. 7–33, 3 pl., scale 1:24,000, accessed August 5, 2023, at https://pubs.usgs.gov/of/2008/1009/.
McLaughlin, R.J., Sarna-Wojcicki, A.M., Wagner, D.L., Fleck, R.J., Langenheim, V.E., Jachens, R.C., Clahan, K., and Allen, J.R., 2012, Evolution of the Rodgers Creek—Maacama right-lateral fault system and associated basins east of the northward-migrating Mendocino Triple Junction, northern California: Geosphere, v. 8, no. 2, p. 342–373. [Available at https://doi.org/10.1130/GES00682.1.]
McNitt, J.R., 1968, Geology of the Kelseyville quadrangle, Sonoma, Lake and Mendocino Counties, California: California Division of Mines and Geology, map sheet 9, scale, 1:62,500, accessed August 5, 2023, at https://ngmdb.usgs.gov/Prodesc/proddesc_391.htm.
McPhee, D.K., Langenheim, V.E., Hartzell, S., McLaughlin, R.J., Aagaard, B.T., Jachens, R.C., and McCabe, C., 2007, Basin structure beneath the Santa Rosa Plain, northern California—Implications for damage caused by the 1969 Santa Rosa and 1906 San Francisco earthquakes: Bulletin of the Seismological Society of America, v. 97, no. 5, p. 1449–1457. [Available at https://doi.org/10.1785/0120060269.]
Metzger, L.F., Farrar, C.D., Koczot, K.M., and Reichard, E.G., 2006, Geohydrology and water- chemistry of the Alexander Valley, Sonoma County, California: U.S. Geological Survey Scientific Investigations Report 2006–5115, 83 p., accessed August 4, 2023, at https://pubs.usgs.gov/sir/2006/5115/.
Morelli, C., ed., 1974, The international gravity standardization net 1971: International Association of Geodesy Special Publication, no. 4, 194 p., accessed August 5, 2023, at https://apps.dtic.mil/sti/citations/ADA006203.
Nilsen, T.H., and Clarke, S.H., Jr., 1989, Late Cenozoic basins of northern California: Tectonics, v. 8, no. 6, p. 1137–1158. [Available at https://doi.org/10.1029/TC008i006p01137.]
Nishikawa, T., ed., 2013, Hydrologic and geochemical characterization of the Santa Rosa Plain watershed, Sonoma County, California: U.S. Geological Survey Scientific Investigations Report 2013–5118, 178 p. [Available at https://doi.org/10.3133/sir20135118.]
Page, B.M., 1966, Geology of the Coast Ranges of California, chap. 6 in Bailey, E.H., ed., Geology of Northern California: California Division of Mines and Geology, Bulletin 190, p. 253–323, accessed August 5, 2023, at http://ia802904.us.archive.org/16/items/northecalifornia00bailrich/northecalifornia00bailrich.pdf.
Plouff, D., 1977, Preliminary documentation for a FORTRAN program to compute gravity terrain corrections based on topography digitized on a geographic grid: U.S. Geological Survey Open-File Report 77–535, 45 p. [Available at https://doi.org/10.3133/ofr77535.]
Prentice, C.S., Larsen, M.C., Kelsey, H.M., and Zachariasen, J., 2014, Late Holocene slip rate and ages of prehistoric earthquakes along the Maacama Fault near Willits, Mendocino County, northern California: Bulletin of the Seismological Society of America, v. 104, no. 6, p. 2966–2984. [Available at https://doi.org/10.1785/0120140003.]
Sonoma County Water Agency, 2021, Groundwater sustainability plan: Santa Rosa Plain Groundwater Sustainability Agency, accessed September 29, 2023, at https://santarosaplaingroundwater.org/gsp/.
Sweetkind, D.S., and Taylor, E.M., 2010, Digital tabulation of geologic and hydrologic data from wells in the northern San Francisco Bay region, northern California: U.S. Geological Survey Open-File Report 2010–1063, 125 p. [Available at https://doi.org/10.3133/ofr20101063.]
Sweetkind, D.S., Taylor, E.M., McCabe, C.A., Langenheim, V.E., and McLaughlin, R.J., 2010, Three-dimensional geologic modeling of the Santa Rosa Plain, California: Geosphere, v. 6, no. 3, p. 237–274. [Available at https://doi.org/10.1130/GES00513.1.]
Telford, W.M., Geldart, L.P., and Sheriff, R.E., 1990, Applied geophysics (2d ed.): New York, Cambridge University Press, 770 p., accessed August 5, 2023, at https://www.cambridge.org/highereducation/books/applied-geophysics/FA576F8F33FCA4D56975BC58CE07851E#overview.
Upp, R.R., 1989, Holocene activity and tectonic setting of the Maacama Fault Zone, Mendocino County, California: Engineering Geology, v. 27, nos. 1–4, p. 375–412. [Available at https://doi.org/10.1016/0013-7952(89)90038-0.]
U.S. Geological Survey, 2019, 3D Elevation program 10-meter resolution digital elevation model: U.S. Geological Survey web page, accessed October 23, 2019, at https://www.usgs.gov/3d-elevation-program.
U.S. Geological Survey and California Geological Survey, 2019, Quaternary fault and fold database for the United States: U.S. Geological Survey website, accessed October 21, 2019, at https://www.usgs.gov/programs/earthquake-hazards/faults.
Wagner, D.L., and Bortugno, E.J., 1982, Geologic map of the Santa Rosa quadrangle: California Division of Mines and Geology, Regional Geologic Map RGM-2A, map scale 1:250,000. [Available at https://ngmdb.usgs.gov/Prodesc/proddesc_518.htm.]
Wakabayashi, J., 2015, Anatomy of a subduction complex—Architecture of the Franciscan Complex, California, at multiple length and time scales: International Geology Review, v. 57, nos. 5–8, p. 669–746. [Available at https://doi.org/10.1080/00206814.2014.998728.]
Watt, J., Ponce, D., Parsons, T., and Hart, P., 2016, Missing link between the Hayward and Rodgers Creek Faults: Science Advances, v. 2, no. 10, 8 p., accessed August 4, 2023, at https://doi.org/10.1126/sciadv.1601441.
Woolfenden, L.R., and Nishikawa, T., eds., 2014, Simulation of groundwater and surface-water resources of the Santa Rosa Plain watershed, Sonoma County, California: U.S. Geological Survey Scientific Investigations Report 2014–5052, 258 p., accessed August 4, 2023, at https://doi.org/10.3133/sir20145052.
Datums
Vertical coordinate information is referenced to the North American Vertical Datum of 1988 (NAVD 88).
Horizontal coordinate information is referenced to the North American Datum of 1983 (NAD 83).
Altitude, as used in this report, refers to distance above the vertical datum. The datum of observed gravity is the International Gravity Standardization Net as described by Morelli (1974).
Supplemental Information
Specific capacity is expressed as well yield per unit depth of drawdown, in gallons per minute per foot (of drawdown; gpm/ft).
Measurements of gravity are expressed in milligals (mGals).
For more information concerning the research in this report, contact the
Director, California Water Science Center
U.S. Geological Survey
6000 J Street, Placer Hall
Sacramento, California 95819
https://www.usgs.gov/centers/california-water-science-center
Publishing support provided by the Science Publishing Network,
Sacramento Publishing Service Center
Disclaimers
Any use of trade, firm, or product names is for descriptive purposes only and does not imply endorsement by the U.S. Government.
Although this information product, for the most part, is in the public domain, it also may contain copyrighted materials as noted in the text. Permission to reproduce copyrighted items must be secured from the copyright owner.
Suggested Citation
Cromwell, G., Sweetkind, D.S., Langenheim, V.E., and Ely, C.P., 2024, Three-dimensional hydrogeologic framework model of the Russian River watershed, California: U.S. Geological Survey Scientific Investigations Report 2024–5083, 25 p., https://doi.org/10.3133/sir20245083.
ISSN: 2328-0328 (online)
Study Area
Publication type | Report |
---|---|
Publication Subtype | USGS Numbered Series |
Title | Three-dimensional hydrogeologic framework model of the Russian River watershed, California |
Series title | Scientific Investigations Report |
Series number | 2024-5083 |
DOI | 10.3133/sir20245083 |
Year Published | 2024 |
Language | English |
Publisher | U.S. Geological Survey |
Publisher location | Reston, VA |
Contributing office(s) | California Water Science Center |
Description | Report: viii, 25 p.; Data Release |
Country | United States |
State | California |
Other Geospatial | Russian River watershed |
Online Only (Y/N) | Y |
Google Analytic Metrics | Metrics page |