Real-Time Pier Scour Monitoring and Observations at Three Scour-Critical Sites in Idaho, Water Years 2020–22
Links
- Document: Report (3.7 MB pdf) , HTML , XML
- Data Release: USGS data release - Hydraulic assessment summary at selected real-time pier scour monitoring sites in Idaho, 2020–2022
- Download citation as: RIS | Dublin Core
Acknowledgments
The authors would like to thank Idaho Transportation Department personnel for their assistance with the pier sonar installation. The use of the “UBIT” (Under Bridge Inspection Truck) allowed U.S. Geological Survey (USGS) personnel to install each of the pier sonar enclosures safely and efficiently, ultimately leading to the success of the monitoring. The authors also would like to thank USGS personnel Pete Elliott, John Carricaburu, Connor Molloy, and Michael Allen for their assistance with pier sonar installation, operation, and quality assurance procedures. Taylor Dudunake and William (Will) Nuckoles collected the geomorphic data at each site. Finally, the authors thank USGS colleagues Mark Henneberg, Steve Holnbeck, Greg Lind, and Marc Stewart for their input regarding the study design, equipment usage, and technical expertise.
Abstract
To observe real-time pier scour at three scour-critical sites in Idaho, the U.S. Geological Survey, in cooperation with Idaho Transportation Department, installed and operated fixed real-time (15-minute interval) bed elevation scour sonar sensors at three bridge locations associated with U.S. Geological Survey streamflow gaging stations for water years 2020 through 2022. Daily mean and peak streamflow conditions during the 3-year study were at or below average except for the peak flow in 2022. Each of the three sites included in the study had a coarse bed with an armored channel. Observed pier scour at each of the three sites was less than 20 percent than the stated minimum depth to the pier pile tip. The below average daily mean and peak streamflow during the study period may have resulted in below average scour.
Observed pier scour data during spring runoff (water years 2020–22) were compared to both Coarse Bed and Hydraulic Engineering Circular 18 (HEC-18) general pier scour design equation estimates to better understand how the observed pier scour data compared to design pier scour equation estimates during the same observational periods. For the 3-year study period, the Coarse Bed design equation generally overpredicted scour by about 2.5 times less than the HEC-18 general pier scour equation. The risk associated with each design equation was summarized using a reliability index to describe how each prediction might be expected to reliably overestimate scour depth. Overall, the Coarse Bed design scour equation provided more reasonable scour depth estimates than the HEC-18 general pier scour equation but with more risk to underestimating scour depth. Because these data are limited (3 sites, 3 years, and during average streamflow conditions), further research is needed to compare observed scour data to estimates predicted by the Coarse Bed design equation and other design equations.
This study demonstrated that real-time pier scour monitoring is a useful method and countermeasure at critical bridge sites. A recently developed rapid deployment real-time pier scour monitoring method may be a useful method to consider for future studies. Real-time monitoring at scour critical sites may be a useful tool to confirm previous scour evaluation estimates where site inspection scour observations conflict with the scour evaluation estimates. Considering alternative scour monitoring and evaluation methods, including the rapid estimation method, and updating pier scour calculations using the most recent coarse-bed pier scour equation may offer a more cost-effective solution to identifying and updating scour critical coding for bridges in Idaho. For scour critical bridge sites, the real-time pier scour monitoring methods used for this study provided an effective real-time local pier scour monitoring countermeasure.
Introduction
Scour is defined as, “Erosion of streambed or bank material due to flowing water” (Federal Highway Administration, 2012a, p. xxxviii). Scour occurring at bridge sites is the leading cause of bridge failures (Federal Highway Administration, 2012a). Total bridge scour is the sum of long-term degradation, contraction, and local scour. Long-term degradation describes the lowering of the streambed elevation over a long-term period. Contraction scour describes the lowering of the streambed elevation resulting from the contraction (or flow constriction) near the bridge site. Local scour is defined as the “removal of material from around piers [also commonly referred to as “bents” or “pile bents”], abutments, spurs, and embankments” (Federal Highway Administration, 2012a, p. 3.2) and is caused by an acceleration of flow and resulting vortices caused by the localized obstruction impeding the flow (Federal Highway Administration, 2012a). The data collected and the focus of this study were limited to local pier scour. Long-term degradation, contraction, or local abutment scour were not monitored or considered in this study.
The Federal Highway Administration (FHWA) requires a scour and stream stability analysis and evaluation (Federal Highway Administration, 2012a) for all bridges reported on the National Bridge Inventory classified as “scour critical” (Federal Highway Administration, 1995, 2012b). A Plan of Action and bridge scour countermeasure are required for each scour critical bridge. A bridge scour countermeasure is, “a measure intended to prevent, delay, or reduce the severity of hydraulic problems” (Federal Highway Administration, 2012a, p. xxvii). Scour critical designations are further categorized into the following four coded groups (Federal Highway Administration, 1995, 2012b).
Code 3: Bridge foundations (abutment, pier, or a combination of each) have been determined to be unstable for calculated scour conditions where scour is within limits of footing or piles, below spread-footing base or pile tips, or a combination of each.
Code 2: Field review indicates that extensive scour has occurred at bridge foundations, which are determined to be unstable by (1) a comparison of calculated scour and observed scour during the bridge inspection, or (2) an engineering evaluation of the observed scour condition reported by the bridge inspection.
Code 1: Field review indicates that failure of piers, abutments, or a combination of each is imminent. Bridge is closed to traffic. Failure is imminent based on (1) a comparison of calculated scour and observed scour during the bridge inspection, or (2) an engineering evaluation of the observed scour condition reported by the bridge inspection.
Code 0: Bridge has failed and closed to traffic.
As of 2020, the National Bridge Inventory (Federal Highway Administration, 2019) listed 265 of Idaho's nearly 4,500 bridges (about 6 percent) as “scour critical” (Federal Highway Administration, 2019). Of these bridge sites, 190 sites were coded “3,” 25 sites were coded “2,” zero sites were coded “1” or “0.” Each Idaho bridge identified as scour critical (National Bridge Inventory item 113 coded 3,2,1, or 0) has a monitoring and closure plan that addresses the public safety hazard (Ayres Associates, 2004a). Direct streambed elevation monitoring during flood flow scenarios is the most accurate method to determine if a bridge pier streambed elevation is approaching a scour critical bed elevation (Ayres Associates, 2004b). The Plan of Action requires monitoring and inspection at each scour critical bridge during and after any identified flood events (Ayres Associates, 2004a, 2004b). Bridges are often located on waterways that have short duration peak flow events. Streamflow can ascend from normal to peak conditions in a matter of hours, leaving too little time for bridge inspection crews to mobilize and monitor scour conditions. Conversely, a peak flow event may be forecasted in advance for a scour critical bridge site, and inspection crews may be placed on high alert or even sent to the bridge site to wait for the flood flow to arrive.
The U.S. Geological Survey (USGS), in cooperation with Idaho Transportation Department (ITD), installed and operated fixed real-time bed elevation scour sonar sensors (sonars) at three scour critical bridge sites in Idaho for water years 2020 through 2022. Using the real-time streambed elevation data, an observed peak-to-peak local pier scour depth was estimated for the peak flow season (March–June). Observed real-time pier scour data were compared to estimated scour depths using the most recent pier scour equations (Federal Highway Administration, 2012a).
Purpose and Scope
This report presents the methods and results of a study that directly monitored the streambed elevation at three selected scour critical bridge sites in Idaho (fig. 1). This report also discusses the project goals and provides a description of the three selected sites. Methods used to install, operate, and disseminate the sonar data are described. Site-specific geomorphic survey data are summarized, and we explain how those data were used to estimate scour using empirically derived pier scour equations. Results are presented for geomorphic and scour estimates; the real-time data served to the USGS National Water Information System (NWIS) database (NWIS; U.S. Geological Survey, 2024a, 2024b, 2024c), and the USGS ScienceBase data release (Fosness and Dudunake, 2024). A summary comparing the observed scour depths to the most recent pier scour estimates during peak flows at each site for each of the 3 years of the study is described, including factors which may cause variability between observed scour and pier scour estimates. Finally, considerations for future real-time monitoring and research are presented.
Methods For Site Selection and Real-Time Pier Scour Monitoring
Site selection and monitoring included (1) selecting three scour critical bridge sites in Idaho, and (2) installing real-time bed elevation scour sonar sensors at the most scour susceptible pier and monitoring the streambed elevation in real-time at each selected site.
Site Selection and Summary
The three sites were selected based on the following three factors: (1) The site is currently listed by ITD as scour critical, (2) the site is co-located with an existing USGS streamflow gaging station, and (3) the site has diverse hydraulic and geomorphic conditions (fig. 1). The selected bridges provided a combination of varying characteristics including channel substrate, pier style, and flow regulation. All references to elevation have been updated, where necessary, to the North American Vertical Datum of 1988 (NAVD 88) for consistency.
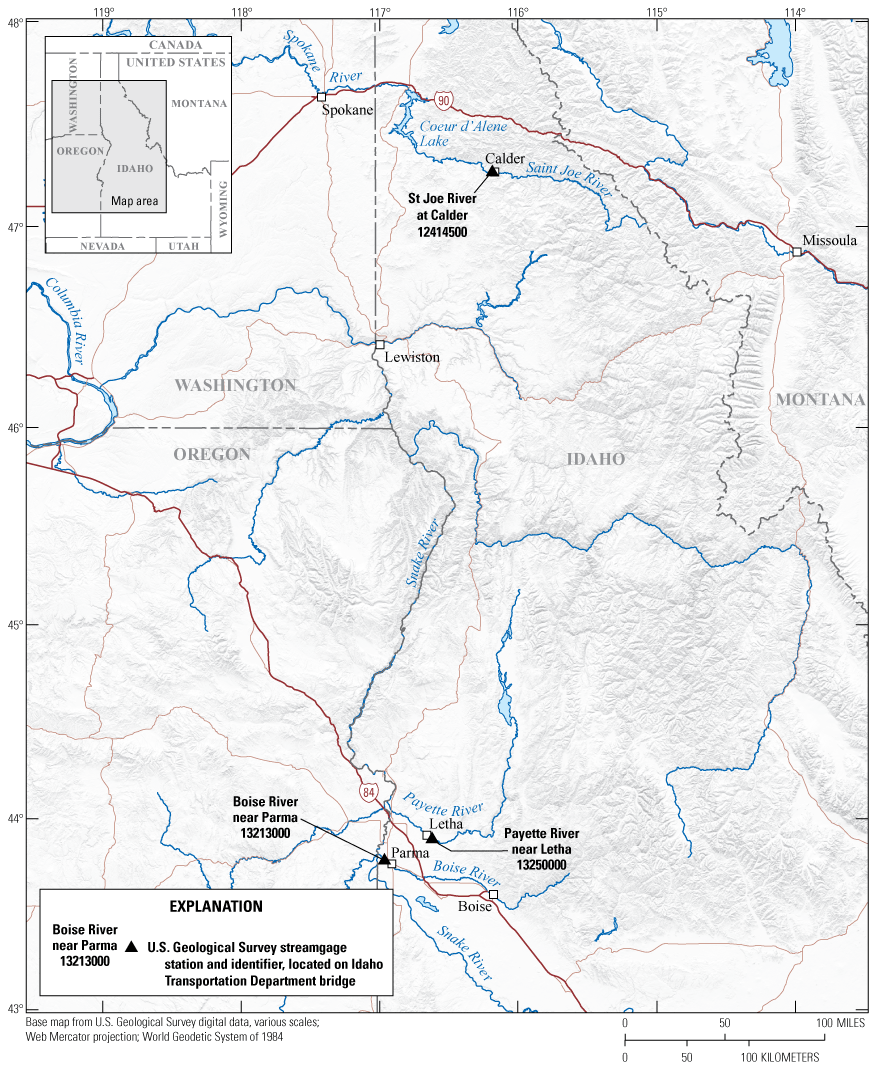
Selected real-time pier scour monitoring locations in Idaho, water years 2020–22 (U.S. Geological Survey, 2024a, 2024b, 2024c).
Site Description of ITD Bridge Structure Number 19935—Payette River Near Letha, Idaho (USGS Station Number 13250000)
Idaho Transportation Department bridge structure number 19935, constructed in 1960, carries Idaho Boulevard (County Road 3830) over the Payette River and is located 1.0 mile (mi) east of Letha, Idaho (fig. 1; table 1). The bridge is co-located with USGS streamflow gaging station Payette River near Letha, Idaho (USGS station number 13250000). Idaho Transportation Department bridge structure number 19935 is currently listed as scour critical with a National Bridge Inventory Code (item 113) value of 3 (Federal Highway Administration, 2019).
The bridge has 9 spans (360 feet [ft] total length) with 2 steel shell pile abutments and 8 bents. Each bent includes 5 cylindrical (1.3-ft diameter) steel shell pile piers with concrete caps. The piers selected for this study were located at bent two (second pier from south abutment). Bent two had the highest observed depths and velocities based on direct observation by USGS. At the time of the initial site inspection, the angle the flow velocity intersects the piers (angle of attack) during low flow was nearly 90 degrees to the piers. Because of the high angle of attack, the sonars at bent two would be protected from debris by bents three through eight. Because the angle of attack varies with stream stage, and because bent two is in the thalweg at low to medium flows, bent two was still prone to collecting debris on the upstream face, although less than bents three to eight.
Two sonars were installed on January 15, 2020 at the upstream and downstream piers at bent two (fig. 2). Two sonars were installed because of the high angle of attack and the possibility of signal interference due to debris. The upstream sonar elevation was 2,283.27 ft and the downstream sonar elevation was 2,283.26 ft. The streambed elevation at the upstream and downstream sonar at the time of installation was 2,279.90 ft and 2,278.00 ft., respectively. The streambed elevation at the upstream side of bent two was 2,279.56 ft. The bridge design specified that the “steel piling shall have a penetration of not less than 15 ft below the original ground surface,” resulting in a minimum estimated pile tip elevation of about 2,264.56 ft (Fosness and Dudunake, 2024). The as-built pile tip elevation is unknown. Five test holes were cored and sampled at the bridge site and included in the design plans. Test hole number two was located at bent two. From test hole number 2: a layer of large gravel extended from the design surface (2,279.90 ft) down about 4.5 ft, a sand layer extended another 3.25 ft, and a sandy clay layer extended another 4.5 ft. The remaining test hole was sand.
Table 1.
Selected real-time pier scour monitoring sites in Idaho, water years 2020–22 (U.S. Geological Survey, 2024a, 2024b, 2024c)[Latitude and longitude referenced to North American Datum of 1983. Gage datum elevation referenced to North American Vertical Datum of 1988. Dates shown as month/day/year. USGS, U.S. Geological Survey; ft, foot]
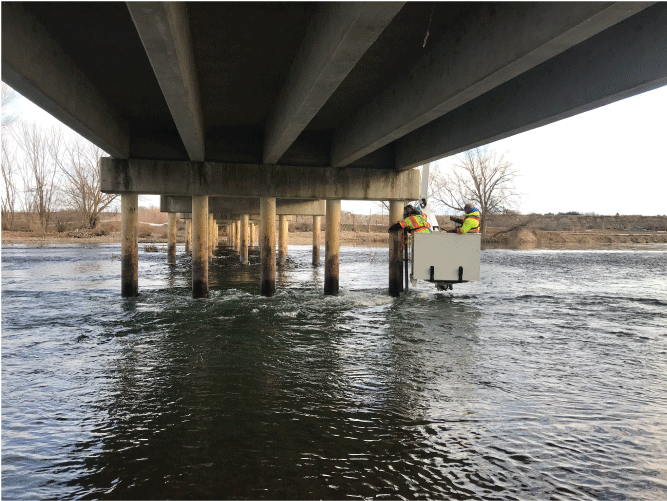
Installation of a sonar device at the upstream pier of bent two, Idaho Transportation Department bridge structure 19935, January 15, 2020. Site is co-located with U.S. Geological Survey (USGS) station Payette River near Letha, Idaho (USGS station number 13250000). [Photograph by Rene Leon, Idaho Transportation Department.]
The bridge design maximum water-surface elevation is 2,288.63 ft. This elevation correlates to a current gage height of 12.47 ft (local USGS streamflow gaging station datum) and a streamflow of about 7,230 cubic feet per second (ft3/s; U.S. Geological Survey, 2024a).
The Payette River is regulated by several upstream dams including Black Canyon Diversion Dam, Deadwood Dam, Cascade Dam, and Lardo Dam. As a result, traditional peak streamflow statistical methods do not provide reliable peak flood frequency flow estimates. The Bureau of Reclamation provided regulated flood frequency statistics for the reach below Black Canyon Diversion Dam, located 13.4 mi upstream of the bridge. The 1-percent annual exceedance probability (AEP; commonly referred to as the 100-year recurrence interval) regulated flow estimate is about 22,800 ft3/s, and the 0.2-percent AEP (commonly referred to as the 500-year recurrence interval) flow estimate is about 43,100 ft3/s (Jonathan Rocha, Bureau of Reclamation, written commun., 2023). Overbank flow occurs at a gage height of about 17.85 ft (local USGS streamflow gaging station datum; elevation 2,294 ft). The corresponding flow is estimated at 29,600 ft3/s. Low chord (lowest elevation of the bridge superstructure) is at an elevation of 2,294.71 ft; pressure flow (submerged low chord) at the bridge is unlikely because overbank flow will exceed the elevation of the road to the south, flanking the bridge. The flow at a water surface elevation immediately below the low chord elevation on the bridge (2,294.71 ft) is about 33,900 ft3/s (U.S. Geological Survey, 2024a).
The streambed at the bridge is composed of sand, gravel, and some silt, and is generally free of vegetation. The main channel is straight near the bridge. As noted in the USGS streamflow gaging station description, the downstream bed elevation gradually changes, even at base flow, and requires regular stage-discharge relationship shifts (Fosness and Dudunake, 2024).
Site Description of ITD Bridge Structure Number 27415—Boise River Near Parma, Idaho (USGS Station Number 13213000)
Idaho Transportation Department bridge structure number 27415, constructed in 1954, carries Hexon Road over the Boise River and is located 0.4 mi south and 1.2 mi west of Parma, Idaho (fig. 1; table 1). The bridge is co-located with USGS streamflow gaging station Boise River near Parma, Idaho (USGS station number 13213000). Idaho Transportation Department bridge structure number 27415 is currently listed as scour critical with a National Bridge Inventory Code (item 113) value of 3 (Federal Highway Administration, 2019).
The bridge length is 331 ft and has 11 spans with 10 bents. Each bent includes 6 timber piles enclosed by wooden planks. In November 2017, bent 2 was rehabilitated with piles 1 (downstream) and 6 (upstream) replaced with hollow structural pipe (12.75-inch wide by 0.5-inch thick). Additionally, bents 1, 3, 4, and 5 had 10-gauge galvanized corrugated metal pipe nose armor installed on the upstream side. Bents 1–4 (from the west abutment) are in the main Boise River channel with bents 5–9 (from the west abutment) in a secondary channel inundated only during higher flows. The piers selected for this study were located at bent two (from the west abutment). Personnel from the USGS surveyed the channel along the upstream face of the bridge prior to installing the sonar. The sonar soundings and surveys indicated that the streambed was uneven near bent two (the location of the sonar).
The sonar was installed on February 24, 2020, on the upstream side of bent two at an elevation of 2,205.41 ft (fig. 3). The streambed elevation at the time of sonar install was about 2,201.8 ft. The streambed elevation near bent two from the design notes was about 2,206.76 ft., which is about 5 ft higher than the current streambed elevation. The bridge design noted, “all [timber] piling shall be driven to a minimum penetration of 15 feet below stream bed elevation,” which results in a designed highest pile tip elevation of 2,191.76 ft (Fosness and Dudunake, 2024). The as-built pile tip elevation is unknown, and no test hole data were available noting the particle size by depth.
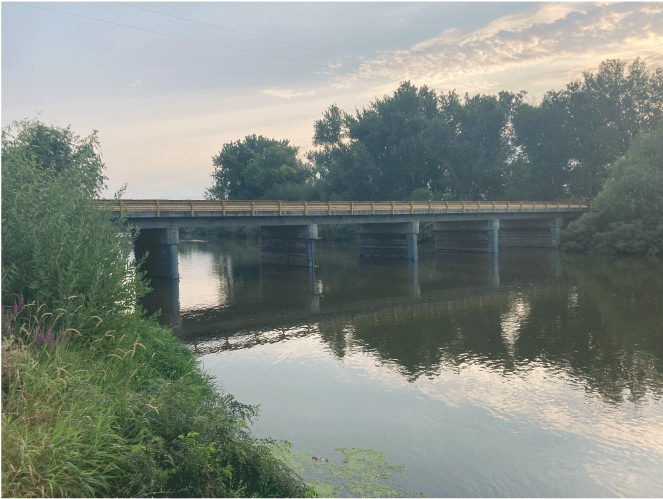
Sonar device at bent two, Idaho Transportation Department bridge structure 27415, July 28, 2021. Site is co-located with U.S. Geological Survey (USGS) station Boise River near Parma, Idaho (USGS station number 13213000). [Photograph by William Nuckoles, formerly U.S. Geological Survey.]
The bridge design maximum water-surface elevation is noted in the design documents as 2,215.76 ft., which is the high-water elevation from a flood that occurred in 1943. Streamflow in the lower Boise River is regulated by Lucky Peak Dam 60 mi upstream of the bridge, along with numerous irrigation diversions and returns. Regulated peak streamflow statistics were provided by the U.S. Army Corps of Engineers for the 10-percent, 1-percent, and 0.2-percent AEPs. The regulated peak streamflow statistics were calculated for the USGS streamflow gaging station Boise River at Glenwood Bridge near Boise, Idaho (USGS station number 13206000; 43.7 mi upstream of the bridge), but the peak flow is expected to be about the same with only minor gains and losses during peak flows. Regulated peak streamflow statistics are 7,500 ft3/s for the 10-percent AEP, 16,600 ft3/s for the 1-percent AEP, and 34,800 ft3/s for the 0.2-percent AEP (U.S. Army Corps of Engineers, 2015). Streamflow less than 3,800 ft3/s remains within the main (low flow) channel, while bank-to-bank flows occur at a streamflow exceeding 7,100 ft3/s.
Low chord on the bridge is at an elevation of 2,216.95 ft; pressure flow could occur at any water-surface elevation above the low chord elevation due to the main channel containing the flow. The maximum computed flow at the USGS streamflow gaging station (from the stage-discharge rating curve) is 11,500 ft3/s occurring at a water surface elevation of 2,215.51 ft. The maximum computed flow with rating extension to the low chord bridge elevation is 14,900 ft3/s. Channel banks are vegetated rip-rap dikes with varied amounts of growth and contain all streamflow up to 11,500 ft3/s (the maximum discharge estimated at the USGS streamflow gaging station). Both the 1-percent AEP (38,400 ft3/s) and the 0.2-percent AEP (16,600 ft3/s) would likely cause pressure flow conditions at the bridge. The channel bed is composed of sand, gravel, and cobble and is generally straight for several hundred feet upstream and downstream from the bridge (gage).
Site Description of ITD Bridge Structure Number 30730—Saint Joe River at Calder, Idaho (USGS Station Number 12414500)
Idaho Transportation Department bridge structure number 30730, constructed in 1990, carries Elk Prairie Road over the Saint Joe River and is located 0.1 mi south of Calder, Idaho (fig 1; table 1). The bridge is co-located with USGS streamflow gaging station Saint Joe River at Calder, Idaho (USGS station number 12414500). Idaho Transportation Department bridge structure number 30730 is currently listed as scour critical with a National Bridge Inventory Code (item 113) value of 3 (Federal Highway Administration, 2019).
The bridge has 3 spans (296 ft total length) with 2 steel pile abutments and 2 bents. Each bent includes 10 steel shell pile piers with concrete caps measuring 29.2 ft long by 3.5 ft wide. The pier selected for this study was located at bent two (northernmost bent). The sonar was installed on January 30, 2020, at an elevation of 2,180.9 ft. (fig. 4). The streambed elevation at the time of installation was 2,177.7 ft. The streambed elevation at bent two, as noted in the design notes, was about 2,177.6 ft with the concrete extending below the ground surface to an elevation of 2,173.35 ft. Rip-rap, an angular rock erosion countermeasure (unknown size) was added around the bents (5 ft along the sides and extending 10 ft upstream and downstream). The bridge design noted that pier 2 minimum pile tip elevation shall be driven to 2,097.56 ft. The as-built pile tip elevation is unknown. Four test holes were cored and sampled at the bridge site and included in the design plans. Test drill hole number one was located about 37 ft south of bent two and described as “Silty, sand and gravel with cobbles” to a bed elevation of 2,160.56 ft (Fosness and Dudunake, 2024). The remaining test hole was sand.
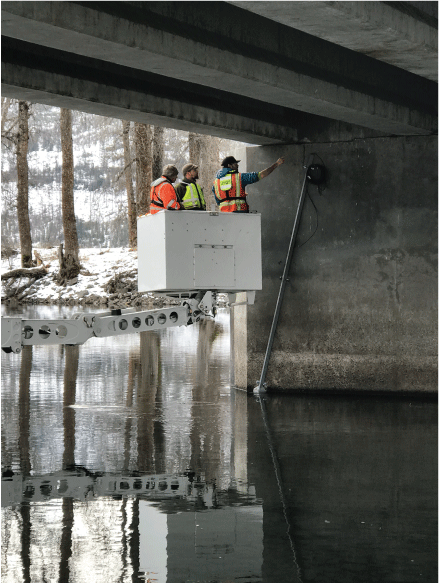
U.S. Geological Survey (USGS) and Idaho Transportation Department (ITD) personnel inside the Under Bridge Inspection Truck (UBIT) during installation of a sonar device at bent two, ITD bridge structure 30730, January 30, 2020. Site is co-located with USGS station Saint Joe River at Calder, Idaho (USGS station number 12414500). [Photograph taken by Rene Leon, Idaho Transportation Department.]
The bridge design maximum water-surface elevation (for the designed 1-percent AEP) was 2,191.50 ft, almost 2 ft below the bridge low chord elevation of 2,193.6 ft. Flow upstream of the bridge site is unregulated, and peak flow was estimated for the 10-percent, 1-percent, and 0.2-percent AEP using the USGS StreamStats program (U.S. Geological Survey, 2019). The 10-percent AEP flow estimate is 26,100 ft3/s; 1-percent AEP flow estimate is 39,900 ft3/s; and the 0.2-percent AEP flow estimate is 49,700 ft3/s. The 1-percent AEP design elevation correlates to a streamflow of about 40,000 ft3/s. Overbank flow, as noted by the USGS station description, occurs at about 14,100 ft3/s. Overbank flow combined with the bridge being elevated above the surrounding floodplain results in minor contraction of the flow under the bridge. Additionally, the overbank flow prevents pressure flow at the bridge (low chord elevation is 2,193.6 ft). The main channel surface substrate is gravel and cobble, and the channel is generally straight in the vicinity of the bridge and streamflow gaging station.
Real-Time Pier Scour Monitoring
Real-time (15-minute interval) streambed elevation monitoring provided a bridge scour countermeasure by remotely observing bridge scour conditions and recording the streambed elevation data. Directly monitoring streambed elevation is the most accurate method to determine if a bridge is approaching a scour critical depth (Ayres Associates, 2004a). Real-time instrumentation and data collection methods used for this study paralleled those described at length in Henneberg (2018). Airmar EchoRange Single Frequency Smart Transducers (sonars; Airmar Technology Corporation, 2019), were mounted within enclosed and custom fabricated steel housings and directly mounted to the piers. The sonars were mounted on the piers so that they would measure the lowest streambed elevation at the nose of the piers. The elevation of the measuring point on each sonar was derived using survey leveling from the sonar measurement points to USGS streamflow gaging station reference marks (Kenney, 2010). A global navigation satellite system (GNSS) survey determined the elevation of each reference mark referenced to the NAVD 88. The USGS reference mark was surveyed following the techniques and methods in Rydlund and Densmore (2012). The elevation of the sonar measuring point (zero depth) was computed by subtracting the vertical distance from the reference mark to the measuring point on the sonar. The sonar power and data transmission lines were run back to the USGS streamflow gaging station along utility corridors or the outside of bridge railings. At the gaging station shelter the National Marine Electronics Association 0183 (NMEA 0183) interface standard data string was converted to serial digital interface at 1,200-baud (SDI–12) protocol data format using a NMEA 0183 to SDI-12 protocol converter (Henneberg, 2018). The protocol converter was connected to the data collection platform (DCP) using standard SDI-12 wiring conventions. The protocol converter was queried by the DCP for raw depth below transducer values using standard SDI-12 commands. Every 15 minutes after the hour the DCP queried the protocol converter for the instantaneous depth below transducer value from the sonar and computed the streambed elevation by subtracting the depth below transducer from the surveyed elevation of the sonar. The 15-minute streambed elevation values were logged to the DCP and transmitted hourly via Geostationary Operational Environmental Satellite (GOES) network to the National Water Information System (NWIS) data repository (https://doi.org/10.5066/F7P55KJN; Henneberg, 2018). The telemetered 15-minute streambed elevation was then published to U.S. Geological Survey National Water Information System (U.S. Geological Survey, 2024a, 2024b, 2024c).
Geomorphic Site Survey and Hydraulic Assessment
Geomorphic site surveys provided site specific parameters required for the hydraulic assessment. The real-time bed elevation provided measurable observations, but the hydraulic assessment was a useful method to compare to the (1) observed scour data and (2) selected flood frequency flows. This section describes the at-site geomorphic data collection along with the methods used to develop the hydraulic assessment.
Geomorphic Site Survey
Site-specific geomorphic data and other observations were collected during a single visit to each bridge. Geomorphic data collected during each visit included a Wolman pebble count (Wolman, 1954) to define the median diameter of the streambed material, an estimate of the flow angle of attack (during peak flow conditions), an assessment of pier shape and dimensions, observations of the floodplain, and observations of bridge scour countermeasures (table 2; Fosness and Dudunake, 2024). In addition, a GNSS site survey was completed to determine the elevation of each bridge structure (road deck and low chord elevations). Real-time (15-minute) hydrologic data were available at each USGS streamflow gaging station (both prior to and during this study) and included real-time discharge and water-surface elevation data (U.S. Geological Survey, 2024a, 2024b, 2024c). The historic USGS discharge measurement data were used to develop peak flow velocity estimates at each site. For each peak flow, the velocity was linearly interpolated using observed measurement data collected from each bridge (table 2). Depth for each peak flow condition was computed using the difference between the water surface elevation and the computed channel bed elevation at each site (table 2).
Table 2.
Hydraulic assessment parameter summary at selected real-time pier scour monitoring sites in Idaho, water years 2020–22 (Fosness and Dudunake, 2024).[Dates shown as month/day/year. ft3/s, cubic foot per second; ft, foot; in, inch; ft/s, foot per second; USGS, U.S. Geological Survey; —, not applicable
Hydraulic Assessment
The FHWA Hydraulic Toolbox (Federal Highway Administration, 2022) provided an efficient and simple approach to estimating pier scour using the bridge scour analysis tool. The results from this tool were compared with the observed streambed elevation changes at each pier instrumented for this study. Pier scour calculations required select parameters including: (1) pier shape and dimensions, (2) angle of attack, (3) flow depth, (4) average velocity, and (5) sediment gradation analysis (diameter of the bed material where 50 percent (D50) and 84 percent (D84) of the sizes are smaller; table 2). Pier shape and dimensions, angle of attack, and sediment gradation data were collected during the geomorphic site surveys (Fosness and Dudunake, 2024). The D50 and D84 particle sizes were computed using the sediment gradation analysis tool within the Hydraulic Toolbox (Federal Highway Administration, 2022; Fosness and Dudunake, 2024). For each selected flow, the upstream flow depth and average velocity were computed using historic discharge measurement data at the bridge for each selected flow condition (table 2; Fosness and Dudunake, 2024).
The bridge scour analysis tool offers five pier scour computation methods: Hydraulic Engineering Circular 18 (HEC-18), Florida Department of Transportation, Complex Pier, Coarse Bed, and Cohesive Materials (Federal Highway Administration, 2012a, 2022). Streambed armoring may limit pier scour depths if the flow condition is clear-water, non-cohesive with a bed material (D50) greater than or equal to 0.787 inches (in), and a gradation coefficient (ratio of D84 divided by D50) greater than or equal to 1.5 (Federal Highway Administration, 2022). Each of the study sites met the preceding conditions; therefore, the Coarse Bed pier scour equation is the most suitable (Holnbeck, 2011; Federal Highway Administration 2012a, 2022). An additional analysis was completed comparing the calculated Coarse Bed equation scour depths to the HEC-18 pier scour equation scour depths (Federal Highway Administration 2012a, 2022; Fosness and Dudunake, 2024).
Real-time Streambed Elevation Monitoring Data Quality Assurance
Quality assurance methods were established prior to the study to ensure consistent and accurate techniques and methods were applied to the real-time streambed elevation monitoring at each of the study sites. Gage datum surveys established the vertical reference to NAVD 88 at each site and the uncertainty associated with the GNSS derived elevation was documented (Rydlund and Densmore, 2012). Elevations at the sonars were established using standard leveling techniques from the gage reference marks (with NAVD 88 elevation; Kenney, 2010). Computed streambed elevations were periodically verified on site by USGS hydrographers by sounding from a known reference point. Sounding quality assurance methods included comparisons with measured depths from an acoustic Doppler current profiler and physical depth measurements (using a Columbus weight and reel, weighted tape, or survey rod; fig. 5).
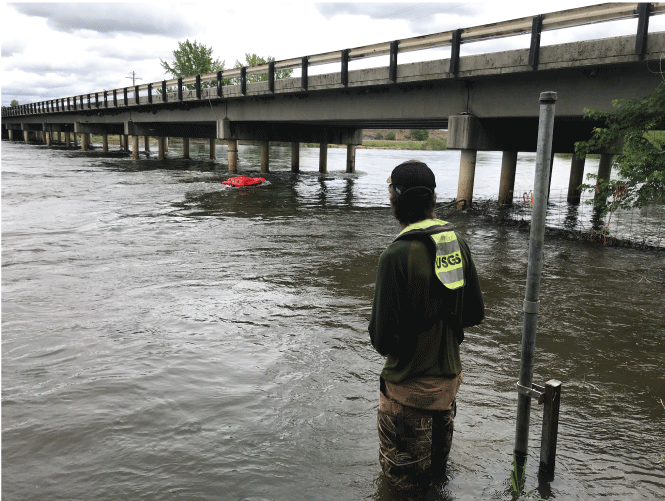
U.S. Geological Survey (USGS) hydrologic technician maneuvering a remote-controlled boat with an acoustic Doppler current profiler into position to verify the accuracy of two sonar devices at Idaho Transportation Department bridge structure number 19935, May 21, 2020. Site is co-located with USGS station Payette River near Letha, Idaho (USGS station number 13250000). [Photograph by Paul V. Schauer, U.S. Geological Survey.]
Data gaps existed within the real-time data record largely due to a combination of debris, ice, power malfunctions, and the sonars coming out of the water at low flows. Debris accumulation generally occurred as large woody debris entangled on the pier and blocked the sonar signal, floating vegetation caught on the pier or sonar, or a combination of each. Every effort was made to remove debris when it was observed during routine site visits or when viewed in the real-time data. Occasionally, large woody debris could not be removed from the pier. Ice accumulation was not as prolific as debris but did occur occasionally. Unexpected loss of power occurred occasionally and resulted in a loss of data during the outage. Power was restored at the gaging station as soon as possible. The sonars were installed as low as reasonably possible, although they did come out of the water occasionally at low flows.
In an effort to filter out erroneous data collected from the sonar data, minimum and maximum elevation values, or thresholds, were established at each site. In general, high thresholds were used to filter data affected by debris (large woody and floating vegetation) and periods when the sonar was out of water or affected by ice; low thresholds were used to filter reflected sonar data resulting in extreme low values.
The sonars used for this study applied the default and constant speed of sound underwater (4,921 ft per second; Airmar Technology Corporation, 2019) to measure the depth. The speed of sound underwater is known to change based on water temperature, possibly resulting in erroneous depth measurements (Xylem, 2018). Water temperature was measured and recorded from the sonar and transmitted with the streambed elevation data. The effect of water temperature on depth was analyzed at each site to assess the need for a water temperature correction. We determined that no corrections were needed to the sonar measurements due to (1) shallow depths and (2) low variability in water temperatures. These factors resulted in a depth uncertainty of less than 0.1 ft., within the accepted accuracy of the sonar (Airmar Technology Corporation, 2019). The quality-assurance depth checks also confirmed water temperature corrections were not necessary for the study sites.
Results of Real-Time Pier Scour Monitoring and Hydraulic Assessment
A summary of observed real-time streambed elevation results and calculated bridge scour estimates at each bridge monitoring site are presented. Peak-to-peak real-time streambed elevation differences represent pier scour at the streambed below the sonar and are presented for each of the 3 water years. The peak-to-peak streambed elevation change represents the maximum change in elevation within the peak runoff period (March–June) and is compared to calculated pier scour estimates from the FHWA Hydraulic Toolbox bridge scour analysis tool (Federal Highway Administration, 2022). It is assumed that the maximum peak-to-peak streambed elevation occurs during a non-scour condition (no pier scour present) and is equal to the streambed elevation at the approach directly upstream of the pier. The minimum peak-to-peak streambed elevation occurs during a maximum scour condition and is the lowest streambed elevation of a local pier scour hole. A positive observed streambed elevation change represents the local pier scour depth as computed from the HEC-18 and Coarse Bed equations (Federal Highway Administration, 2012a).
Real-Time Streambed Elevation and Scour Observations for ITD Bridge Structure Number 19935—Payette River Near Letha, Idaho (USGS Station Number 13250000)
Real-time streambed elevation was monitored at the upstream and downstream portion of bent two. Real-time streambed elevation and streamflow data are available at NWIS (U.S. Geological Survey, 2024a) for the study duration. Data gaps in the streambed elevation values at the Payette River near Letha, Idaho occurred due to miscellaneous gage malfunctions, erroneously high and low values, and the sonar coming out of the water during low flow periods. NWIS defines a data gap any time values are missing for more than 2 hours. Many erroneously high values attributed to aeration in the beam path of the sonar were screened and caused data gaps in the record. Periodically, reflected sonar data caused erroneously low values, which were also screened from the record. Data gaps due to aeration or reflected sonar data do not exceed 5 hours. Quality assurance depth checks collected periodically at the site provide an uncertainty estimate for the observed scour data. The observed pier scour uncertainty for the upstream and downstream piers ranged from 0.1 to 0.2 ft (table 3). Daily mean streamflow during the study period (water years 2020–22) was generally at or below the median daily statistic for the site. Instantaneous peak streamflow ranged from a low of 4,600 ft3/s (April 5, 2021) in water year 2021 (ranked 36 within the 38-year peak flow record) to a high of 12,800 ft3/s (June 13, 2022) in water year 2022 (ranked 18 within the 38-year peak flow record). The water year 2022 peak flow was larger than the average and median peak flows at the site of 12,200 ft3/s and 11,900 ft3/s, respectively. In general, the daily mean and instantaneous peak flows were below or near average for the duration of the study period (U.S. Geological Survey, 2024a; fig. 6).
Streambed elevation data collected during the water year 2020 peak runoff period (March–June) did not indicate significant scour at the upstream or downstream sonar (fig. 6). Observed peak-to-peak scour for water year 2020 at the upstream and downstream sonars were 1.5 ft and 0.9 ft (±0.1 ft), respectively. Water year 2021 flow was well below average resulting in an aggrading (increasing) streambed elevation within the March–June runoff period. The channel bed elevation aggraded from March to June resulting in a negative peak-to-peak scour value (fill) at the upstream and downstream sonar (−0.6 ft and −0.7 ft [±0.2 ft], respectively). While the peak flows were largest during the runoff period in water year 2022, the resulting observed peak-to-peak scour depths were similar to water year 2020 at 1.4 ft (±0.2 ft; upstream) and 0.8 ft (±0.2 ft; downstream) (fig. 5; table 3). The minimum pile tip elevations given in the design plans suggest that the scour depth to the pile tips would need to be about 16 ft for the upstream pier and about 14 ft for the downstream pier. The subsurface particle gradation (from test hole number 2 in the bridge design), while not measured as part of this study, suggests a coarse bed to an elevation of 2,274.9 ft, or a depth of about 5.1 ft (upstream) and 3.3 ft (downstream).
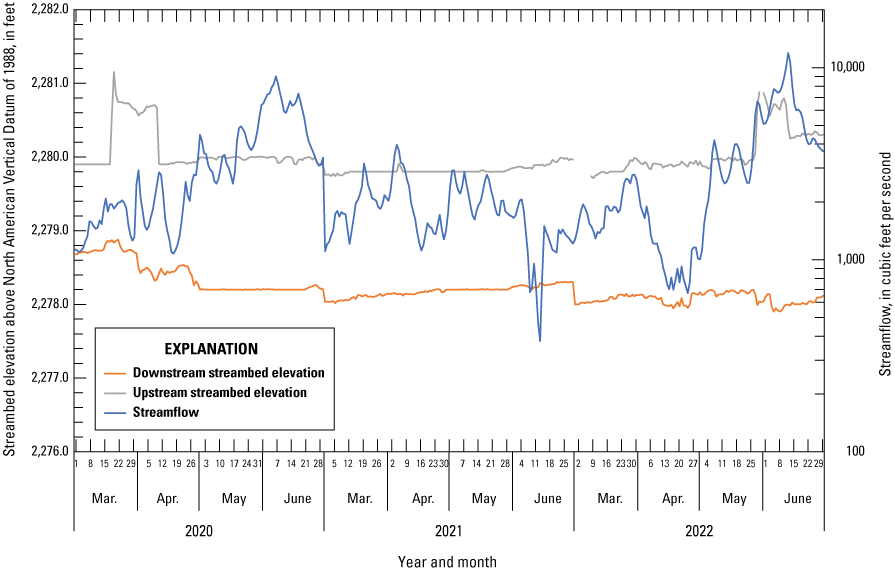
Peak runoff streamflow and streambed elevation (upstream and downstream sensors) at Idaho Transportation Department bridge structure number 19935, March–June, 2020–22. Site is co-located with U.S. Geological Survey (USGS) station Payette River near Letha, Idaho (USGS station number 13250000). [Streamflow data are from U.S. Geological Survey, 2024a. Streambed elevation data are from Fosness and Dudunake, 2024.]
Coarse Bed equation calculated scour values generally overestimated scour when compared to the observed streambed elevation data. Pier scour was calculated at a single location at the upstream side of the bridge, and the same pier scour value was used to compare against the upstream and downstream observed scour values. For water year 2020 the calculated scour overestimated the scour depth by 2.1 ft at the upstream sonar and 2.7 ft at the downstream sonar. Calculated scour for water year 2021 was 1.6 ft and overestimated the observed scour by 2.2 ft at the upstream pile and 2.3 ft at the downstream pile. The largest calculated scour occurred in water year 2022 at 4.5 ft; again, overestimating scour by 3.1 ft (upstream) and 3.7 ft (downstream). In general, the calculated scour values produced comparable scour estimates to the observed peak-to-peak streambed elevation change (table 3). Both the observed streambed elevation changes and calculated scour values were less than the scour depth to the minimum pile tip elevation at the upstream and downstream piers.
Table 3.
Observed real-time bed elevations for the peak runoff season (March–June, 2020–22) and calculated pier scour depths (Fosness and Dudunake, 2024).[Runoff period is March–June of the year specified. bed, streambed; ft, foot; Min, minimum; Max, maximum; peak-to-peak, the difference between the minimum and maximum bed elevation within the runoff period, USGS, U.S. Geological Survey; —, not applicable; Designed scour depth to min pile tip, depth of scour required to reach the lowest elevation of the pile tip based on bridge design drawing information; Calculated pier scour depth, calculated scour using Coarse Bed equation]
Real-Time Streambed Elevation and Scour Observations for ITD Bridge Structure Number 27415—Boise River Near Parma, Idaho (USGS Station Number 13213000)
Real-time streambed elevation was monitored at the upstream portion of bent two. Real-time streambed elevation and streamflow data are available at NWIS (U.S. Geological Survey, 2024b) for the study duration. There are many data gaps in the streambed elevation data at the Boise River near Parma due to heavy aquatic vegetation growth in the channel and drifting aquatic vegetation that collected on the pier and sonar. Aquatic vegetation was cleared during site visits; however, data gaps generally increased throughout the growing season. The observed pier scour uncertainty varied by up to 0.5 ft due to an uneven streambed at the pier (table 3). Soundings and a channel cross section survey prior to sonar installation indicated that the streambed is 1.1 to 2.3 ft higher immediately upstream of the bridge than directly underneath the sonar. Prior to sonar installation, soundings were made on both sides of the pier. The streambed elevation on the left bank side of the pier was 2,203.0 ft and the streambed elevation on the right bank side of the pier was 2,201.8 ft. Soundings on March 16, 2020, also revealed differences from the left to right bank sides of the pier. Due to the highly turbid nature of the river, it is unclear if the difference in the soundings from either side of the pier were due to debris on the streambed, erroneous soundings on the bridge structure, or an actual difference in the streambed elevation. Approach flow angle of attack does not suggest there would be a scour hole on one side of the pier or the other (table 2). Daily mean streamflow during the study period was generally at or below the median daily statistic for the site. Annual instantaneous peak streamflow ranged from a low of 2,090 ft3/s (May 20, 2020; ranked 34 within the 47-year peak flow record) to a high of 3,270 ft3/s (June 13, 2022; ranked 29 within the 47-year peak flow record). The average and median peak flows of 4,960 ft3/s and 5,780 ft3/s, respectively, are larger than each of the peak flows during the study period. In general, both the daily mean and instantaneous peak flows were below or near average for the duration of the study period (U.S. Geological Survey, 2024b).
Streambed elevation data for water year 2020 showed a scour pattern in late May through mid-June resulting in a peak-to-peak observed scour of 1.8 ft (±0.5 ft; fig. 7). water year 2021 resulted in the channel aggrading until the peak flow, scouring through mid-June, then rapidly filling through late June. While there was some observed scour during runoff in 2021, the net observed peak-to-peak change in streambed elevation resulted in 2.2 ft (±0.2 ft) of fill. Water year 2022 experienced the largest peak flow, and the streambed elevation generally remained stable until the peak flow in June resulting in 1.3 ft (±0.5 ft) of peak-to-peak scour (table 3). Throughout the study period the streambed elevation at bent 2 aggraded. The average streambed elevations in 2020, 2021, and 2022 are 2,202.5, 2,202.9, and 2,203.3 ft, respectively. The minimum design pile tip depth given in the design plans suggest a scour depth to the minimum pile tips of about 11 ft (table 3).
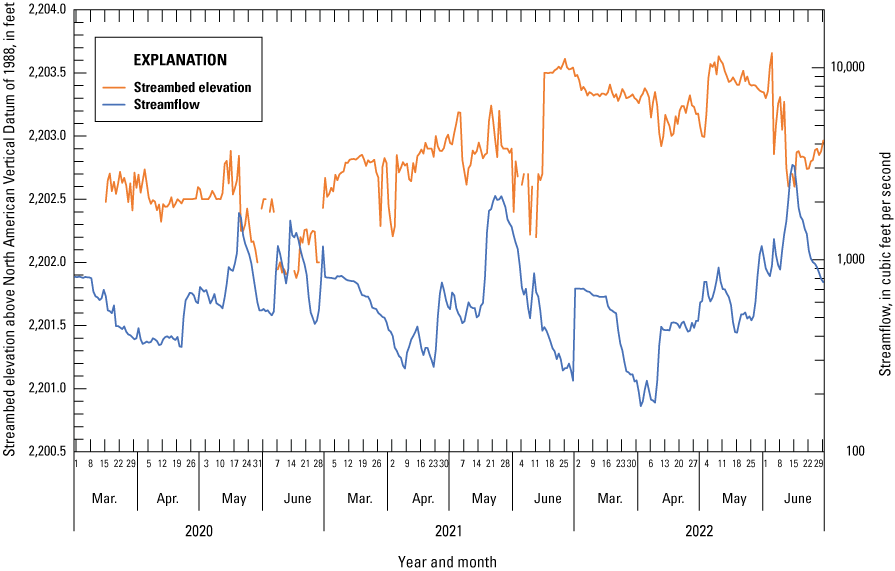
Peak runoff streamflow and streambed elevation at Idaho Transportation Department bridge structure number 27415, March–June, 2020–22. Site is co-located with U.S. Geological Survey (USGS) station Boise River near Parma, Idaho (USGS station number 13213000). [Streamflow data are from U.S. Geological Survey, 2024b. Streambed elevation data are from Fosness and Dudunake, 2024.]
Coarse Bed-calculated scour values generally increased for each year following the same trend as the peak flow. The calculated scour for water year 2020 was 1.4 ft.; underestimating the observed scour by 0.4 ft. Similarly, water year 2021 produced a calculated scour estimate of 1.5 ft; overestimating by 3.7 ft compared to the observed scour. Likely because of the increased peak flow in 2022, the calculated scour in water year 2022 was 1.6 ft, an overestimate of 0.3 ft compared to the observed scour (table 3). While the water year 2021 scour results overestimated scour by over 3 ft due to aggradation at the pier that year, the calculated scour estimates for water years 2020 and 2022 were within 0.5 ft. of the observed scour. Both the observed and calculated scour values were less than the scour depth to the minimum pile tip elevation.
Real-Time Streambed Elevation and Scour Observations for ITD Bridge Structure Number 30730—Saint Joe River at Calder, Idaho (USGS Station Number 12414500)
Real-time streambed elevation was monitored at the upstream portion of bent number 2. Real-time streambed elevation and streamflow data are available at NWIS (U.S. Geological Survey, 2024c) for the study duration. There are periodic data gaps of less than 24 hours throughout the streambed elevation record at the Saint Joe River at Calder, Idaho due to erroneously high and low values caused by reflected sonar data off the angular rip rap armoring the pier. Longer duration data gaps, up to 46 days, during high flow and late spring, are caused by the filtering of erroneously high values caused by debris that collected on the nose of the pier. Special visits were made to clear debris during peak flows; however, debris-affected values were not urgent during the flow recession so special visits were not prioritized. Small debris during the flow recession would generally clear the nose of the pier during the next peak flow event. There are periodic data gaps in the late summer due to the sonar coming out of the water and during the winter due to ice on the sonar. Occasional equipment malfunctions also caused short duration data gaps. Daily mean streamflow during the study period was generally at or below the median daily statistic for the site. Annual instantaneous peak streamflow ranged from a low of 9,210 ft3/s (May 2, 2021; ranked 93 within the 105-year peak flow record) to a high of 17,000 ft3/s (May 7, 2022; ranked 40 within the 93-year peak flow record). Only the peak flow in water year 2022 exceeded the average and median peak flows of 16,600 ft3/s and 15,400 ft3/s, respectively. Except for water year 2022, the mean daily and peak flows were generally below average (U.S. Geological Survey, 2024b).
Water year 2020 produced an observed peak-to-peak scour of 0.9 ft (±0.5 ft; fig. 8). Water year 2021 resulted in the channel generally aggrading until the peak flow in May. Overall, the channel largely aggraded, with a peak-to-peak scour of 0.8 ft (±0.2 ft). Water year 2022 produced an average peak flow of 17,000 ft3/s but the streambed elevation experienced only minor changes with a peak-to-peak scour result of 1.0 ft (±0.2 ft; table 3). The observed pier scour uncertainty varied from 0.2 to 0.5 ft; this was likely because the streambed elevation at the pier was uneven due to a scour hole on the upstream right bank side of the pier and large angular rip-rap at the pier. Quality-assurance soundings collected directly upstream of the pier nose were less than soundings directly at the sonar and may have resulted in increased uncertainties due to measuring away from the sonar sounding location. Additionally, erroneous sonar soundings were attributed to beam scattering caused by the angular rip-rap at the pier and an uneven streambed surface. The streambed elevation data obtained from this study (about 2,178 ft) suggests that a scour depth of about 80 ft would be required to reach the minimum pile tip elevation (2,097.56 ft).
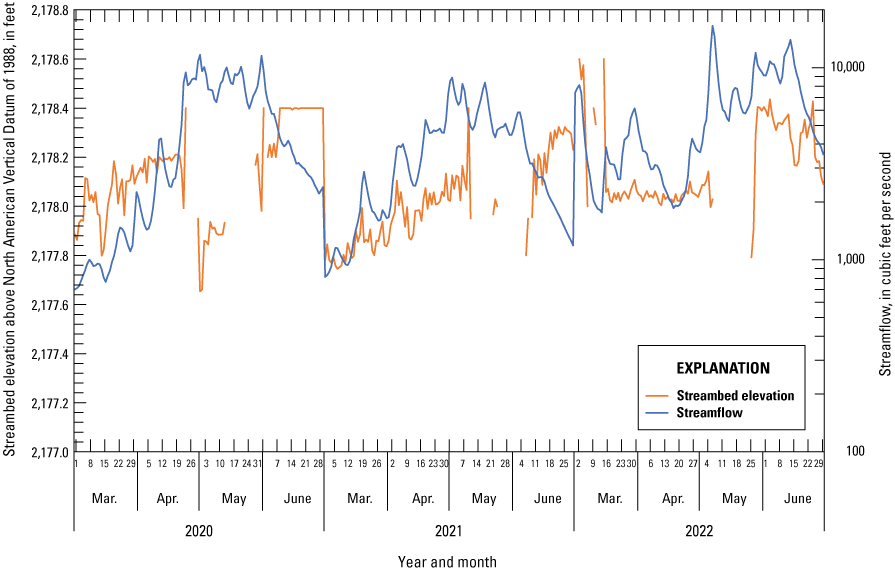
Peak runoff streamflow and streambed elevation at Idaho Transportation Department bridge structure number 30730, March–June, 2020–22. Site is co-located with U.S. Geological Survey (USGS) station Saint Joe River at Calder, Idaho (USGS station number 12414500). [Streamflow data are from U.S. Geological Survey, 2024c. Streambed elevation data are from Fosness and Dudunake, 2024.]
Coarse Bed calculated scour values ranged from 1.7 ft (water year 2021) to 2.9 ft (water year 2022; table 3). The calculated values for water years 2020 and 2021 were within about 1.4 foot of the observed scour. Water year 2022 calculated pier scour overestimated by 1.9 ft compared to the observed scour. The water year 2022 peak flow (17,000 ft3/s) was above average for the site.
Risk and Accuracy Assessment
HEC-18 design pier scour equations were not developed to predict scour depth but rather predict the maximum probable scour depth for design applications. While conservative scour estimates are desirable for safety reasons, overly conservative scour estimates may lead to (1) over-designed bridge foundations and (2) overestimation of predicted scour at existing bridges. For each case, overly conservative scour estimates can lead to an increase in design, construction, and operating cost that is not economically acceptable. The observed scour data from this study were compared to two HEC-18 design equations (Coarse Bed and HEC-18 general pier scour; fig. 9) and summarized in terms of risk (reliability index) and accuracy (relative root mean square error).
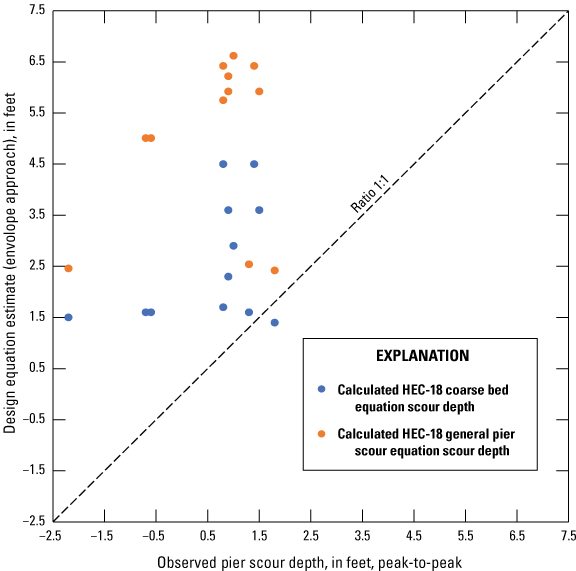
Coarse Bed and Hydraulic Engineering Circular 18 general design equation pier scour calculated depths to observed pier scour depths for selected real-time pier scour monitoring locations in Idaho, March–June, 2020–22 (Federal Highway Administration, 2012a).
The observed pier scour depth was compared to each depth calculated by the Coarse Bed and HEC-18 general pier scour equations (Federal Highway Administration, 2012a, 2012c, 2016). A comparison of the observations and calculated results for each HEC-18 design equation provided a (1) reliability index and (2) relative root mean square error for the dataset (table 4).
Table 4.
Comparison of the performance between the calculated Hydraulic Engineering Circular 18 (HEC-18) Coarse Bed and HEC-18 Pier Scour Equations showing the resulting reliability index and relative root mean square error (Fosness and Dudunake, 2024).[Runoff period is March–June of the year specified. ft, foot; peak-to-peak, the difference between the minimum and maximum observed pier scour within the runoff period; HEC-18, Hydraulic Engineering Circular 18; x, scour depth ratio; dim., dimensionless; RI, reliability index; RE, relative error; RRMSE; relative root mean square error; USGS, U.S. Geological Survey; —, not applicable]
The reliability index (RI) describes the risk of underpredicting the pier scour depth (Federal Highway Administration, 2012a, 2012c, 2016). The Federal Highway Administration described a target reliability index equal to 2.0 based on, “an assessment of the reliability of current practice” (Federal Highway Administration, 2016, p. 22). An RI greater than 2.0 indicates a lower risk of underpredicting scour, whereas an RI less than 2.0 indicates a higher risk of underpredicting scour (Federal Highway Administration, 2012c, 2016). The RI was calculated using the same approach as FHWA to compare the RI for the Coarse Bed and HEC-18 general pier scour equations. The results from this study were an RI of 1.2 (higher risk of underpredicting scour) for the Coarse Bed design equation and 2.2 (lower risk of underpredicting scour) for the HEC-18 general scour design equation (table 4).
The relative root mean square error (RRMSE; table 4) describes the overall error associated with each method compared to the observation to evaluate scour design equation prediction performance. The Coarse Bed design equation overpredicted scour depths (RRMSE =2.2) as intended by the design equation. The Coarse Bed design equation generally overpredicted scour by about 2.5 times less than the HEC-18 general pier scour equation for data collected during the 3-year study period.
Considering the risk (RI) and the accuracy (RRMSE), the Coarse Bed design equation provided a more accurate result than the HEC-18 general pier scour equation but less reliable at overpredicting the scour depth compared to the general scour equation. The risk and accuracy assessments are based on 12 observations collected from this study. Additional field data collected over a large range of flow observations are needed to perform a more thorough assessment to compare the field observations with the predictions from the HEC-18 pier scour design equations (Coarse Bed and HEC-18 general).
Discussion and Considerations for Further Research
Pier scour at bridges is largely a function of pier width, pier length, angle of attack, depth, velocity, substrate, and pier shape (Ayres Associates, 2004a). The selected sites had exposed pier widths ranging from 1.1 to 3.5 ft. The largest observed peak-to-peak scour depth occurred at the pier with the smallest width (ITD bridge structure number 27415) suggesting that pier width alone did not correlate to scour depth. Pier length is not a function of scour depth if the pier is aligned with the flow (Ayres Associates, 2004a). Only ITD bridge structure number 19935 had an estimated flow angle of attack exceeding 0 degrees on the piers at peak flow that may contribute to scour (table 2). It is important to note that flow angle of attack at each site was estimated from field observations during peak flow conditions to visually observe the angle of attack values. Observed depths and velocities were generally similar at the three sites during the study period (table 2). Like pier width, the site with the smallest observed depths and velocities (ITD bridge structure number 27415) resulted in the largest peak-to-peak observed streambed elevation change for the study period suggesting that the observed depths and velocities were not correlated to scour depth. All sites included in this study had coarse bed channels with a surface substrate (D50 greater than [>] 0.787 in) that may have armored the streambed (gradation coefficient of 1.5 to 2.2) during the peak flows observed during water years 2020–22 (table 2). The largest observed scour was associated with bridge structure number 27415, a site that had the smallest D50 (0.85 in), suggesting that smaller substrate resulted in increased scour depths. Peak-to-peak streambed elevation changes were used to explain the calculated scour depths at each site based on observed field data collected during the peak runoff period (March–June) for water years 2020–22. Scour was not observed from any single flow event, so the peak-to-peak was used as an alternative method to quantify pier scour during the runoff period. Observed peak-to-peak streambed elevation changes at each of the study sites within the study period ranged from a minimum of −2.2 ft (aggrading) to a maximum of 1.8 ft (degrading; table 3). The negative value (−2.2 ft ±0.2 ft) occurred at ITD bridge structure number 27415: Boise River near Parma, Idaho during water year 2021, when the flow (mean daily and peak) was below average. Maximum scour occurred in water year 2020 (1.8 ft ±0.5 ft) at ITD bridge structure number 27415: Boise River near Parma, Idaho. Each of the study sites experienced the single largest peak flow in water year 2022 though peak-to-peak scour depths were comparable to water years 2020 and 2021. The lack of scour during the water year 2022 peak runoff suggests the observed peak flows were not large enough to initiate any significant scour at the sites. The minimum pile tip elevation and resulting scour depth to minimum pile tip was used to compare against the observed scour values. None of the observed scour depths exceeded 20 percent of the depth to the pile tip. It is important to note that the minimum pile tip elevation and depths are provided as estimates based on the bridge design; the as-built elevations are unknown.
Bridge pier design scour equations (HEC-18 and Coarse Bed for example) were developed based on an enveloping curve approach for design applications where the goal is to predict the maximum scour depths for a given set of conditions for design applications. Because the design equations are based on an envelope curve approach, the design equations often overestimate scour by large amounts resulting in overdesigned bridge foundations (Landers and Mueller, 1996). Pier scour was calculated for each site using both Coarse Bed and HEC-18 general pier scour equations and compared against the observed scour to evaluate the risk and accuracy. The Coarse Bed pier scour equation (RRMSE =2.2; table 4) resulted in a less conservative estimate compared to the HEC-18 general pier scour equation (RRMSE =5.6) suggesting a more accurate pier scour prediction. The risk associated with higher accuracy is associated with underpredicting pier scour where observed scour is larger than predicted. The risk (reliability index) for the Coarse Bed data was 1.2 compared to 2.2 for the HEC-18 general pier scour equation suggesting that while the Coarse Bed pier scour equation may produce a more accurate calculated pier scour depth, there may be additional risk to consider with the calculation. Average-to-below-average median daily and peak flows during this study did not sufficiently test how larger peak flows (and the resulting scour) would compare to calculated scour values during larger flow events. Further real-time monitoring at the study sites or similar sites with coarse bed conditions is necessary to test how well observed scour compares to calculated scour during larger peak flow conditions.
The techniques and methods presented in this study demonstrate that real-time pier scour monitoring is an effective scour countermeasure for scour critical bridges. A relatively new rapid deployment bridge scour monitoring system might be worth considering for future installations (Henneberg and Richards, 2022). The rigid mounting configuration used in this study required a day and the use of ITD equipment and two personnel to complete. Although rigid and effective, the method used in this study limits the deployment to longer-term operations. Developed by the USGS Colorado Water Science Center, the rapid deployment bridge scour monitoring system approach allows instrumentation to be deployed ahead of floods at candidate bridge sites. The candidate bridge sites are surveyed ahead of time with pre-installed mounting plates, allowing sonar equipment to be installed on short notice ahead of floods.
As an alternative to real-time monitoring, a modified Rapid Estimation Method (REM) is a cost-effective and consistent method to quantitatively estimate total scour at bridges (Holnbeck and Parrett, 1996). The REM was originally developed by USGS for evaluating scour at bridges in Montana using limited site data to quantitatively estimate total scour (contraction, abutment, and pier scour) and can be completed within about 1–2 hours. The REM method uses a given flow estimate (often the peak flows of concern at the bridge site, in other words, 1-percent and 0.2-percent AEPs) and bridge geometry to iteratively estimate the hydraulic variables (channel width and depth, flow velocity). A basic survey at the approach and bridge sections is all that is required to compute scour at the bridge using the most recent bridge scour equations. A more detailed description of the REM is provided in Holnbeck and Parrett (1996), but the general approach and state-specific regressions could be modified and applied to bridges in Idaho. The REM is designed to be a more conservative approach to scour estimation, but the results are useful to identify bridges that may be deserving of a more in-depth scour analysis (such as a more complex level 2 scour analysis) or potential real-time monitoring (such as that used in this study). Using the updated scour equations (Coarse Bed pier scour for example), at-site geometric parameters (flow angle of attack, for example), streamflow statistics, and estimated hydrologic parameters (depth and velocities) within the REM method would provide a cost-effective method to update all bridges of interest in Idaho. With the recent implementation of the Coarse Bed pier scour equation, the pier scour estimates would be reduced at sites with channel armoring under clear-water conditions. For example, a bridge scour evaluation was completed at ITD bridge structure number 30730 on December 16, 1996 that resulted in a calculated pier scour of 23.6 ft for the 1-percent AEP peak flow event. Earlier that same year, a flood of nearly the 1-percent AEP (39,200 ft3/s) occurred on February 9, 1996 (U.S. Geological Survey, 2024c). The calculated pier scour of 23.6 ft then required a structural (buckling) analysis to be completed at the bridge site. The buckling analysis resulted in a factor of safety less than 1; therefore, the bridge was classified as scour critical. Two major observations from this scour evaluation are noted: (1) the flow angle of attack was estimated at 19 degrees and (2) the HEC-18 pier scour equation was used to estimate the pier scour. Observations from this study noted a 0-degree angle of attack at high flow. Field data and observations from this study determined that the study site is suitable for evaluation using the Coarse Bed scour equation. Recomputing the pier scour using the Coarse Bed equation decreased the local pier scour estimate by about 8 ft. Using the Coarse Bed equation and the angle of attack estimated and observed from this study further reduced the estimated scour by a total of nearly 18 ft, resulting in a new local pier scour estimate of about 6 ft. Additionally, the bridge scour evaluation (December 16, 1996) was completed about 10 months after flood of nearly the 1-percent AEP (39,200 ft3/s on February 9, 1996). Little to no local pier scour was noted on the scour evaluation at the site that resulted from the near 1-percent AEP earlier that year suggesting that the original 1-percent AEP scour estimate was severely overestimated. This example demonstrates the sensitivity to both angle of attack and the choice of pier scour equation when computing local pier scour. Erroneous and outdated local pier scour estimates could solely be responsible for the scour critical rating. The REM approach, modified for Idaho, might be a cost effective and efficient approach to provide recent and reasonable scour estimates at select sites in Idaho.
Summary
The U.S. Geological Survey (USGS), in cooperation with Idaho Transportation Department (ITD), installed and operated fixed real-time streambed elevation sonars at three scour critical bridges co-located with USGS streamflow gaging stations in Idaho to observe real-time pier scour from 2020 to 2022. Daily mean and peak streamflow conditions during the 3-year study were at or below average except for the peak flow in 2022. Each of the three sites included in the study had a coarse armored streambed. Observed pier scour at each of the three sites was less than 20 percent than the stated minimum depth to the pier pile tip. The below average streamflow during the study period may have resulted in below average scour depths. Quality assurance methods were established prior to the study to ensure consistent and accurate techniques and methods were applied to the real-time streambed elevation monitoring at each of the study sites.
Observed peak-to-peak streambed elevation changes at each of the study sites within the study period ranged from a minimum of −2.2 feet (ft; aggrading) to a maximum of 1.8 ft (degrading). The negative value (−2.2 ft ±0.2 ft) occurred at ITD bridge structure number 27415: Boise River near Parma, Idaho during water year 2021 when the flow (mean daily and peak) was below average. Maximum scour occurred in water year 2020 (1.8 ft ±0.5 ft) at ITD bridge structure number 27415: Boise River near Parma, Idaho. Each of the study sites experienced the single largest peak flow in water year 2022 though peak-to-peak scour depths were comparable to the water years 2020 and 2021. The lack of scour during the water year 2022 peak runoff suggests the observed peak flows were not large enough to initiate any significant scour at the sites. The minimum pile tip elevation and resulting scour depth to minimum pile tip was used to compare against the observed scour values.
Observed pier scour data during spring (March–June) runoff (2020–22) were compared to both Coarse Bed and Hydraulic Engineering Circular 18 (HEC-18) general pier scour design equation estimates to better understand how the observed pier scour data compared to design pier scour equation estimates during the same observational periods. To compare the observed streambed elevation change with calculated scour, the Federal Highway Administration (FHWA) Hydraulic Toolbox provided an efficient and simple approach to calculating pier scour using the bridge scour analysis tool. The comparison of observed scour to scour predicted by HEC-18 Coarse Bed and HEC-18 general scour design equations were further described in terms of both risk and accuracy. Risk was summarized as a function of reliability of overestimating scour depths, whereas accuracy was summarized as relative error. Overall, the Coarse Bed scour equation calculated a more accurate estimate of scour depth (relative root mean square error [RRMSE] =2.2) compared to the HEC-18 general scour equation (RRMSE =5.6). While proving a more accurate comparison, there was more risk (reliability index [RI] =1.2) associated with the Coarse Bed calculated results than the HEC-18 general pier scour results (RI =2.2). The risk and accuracy data are limited to the three sites for the 3-year study period and the average to below average flows. Additional data collection at the study sites or similar sites with coarse bed conditions is necessary to test risk and accuracy during larger peak flow conditions.
This study demonstrated that real-time pier scour monitoring is a useful method and countermeasure to monitor pier scour critical bridge sites. A recently developed rapid deployment monitoring method may be useful to consider for future studies. Real-time monitoring at scour critical sites may be a useful tool to confirm previous scour evaluation estimates where site inspection scour observations conflict with the scour evaluation estimates. Additionally, considering alternative scour monitoring and evaluation methods, including the rapid estimation method, and updating pier scour estimates using the most recent Coarse Bed pier scour equations, may offer a more cost-effective solution to identifying and updating the scour critical coding for bridges in Idaho. The real-time pier scour monitoring methods used for this study provided an effective monitoring countermeasure at the selected scour critical sites in Idaho.
References Cited
Airmar Technology Corporation, 2019, EchoRange™/EchoRange+™ And 200m Mini Altimeter Kit User Technical Manual (revision 1.004, February 6, 2019): Airmar Technology Corporation web page, accessed December 10, 2019, at https://www.airmartechnology.com/productdescription.html?id=112.
Ayres Associates, 2004a, Office manual—Plans of action for scour critical bridges: Idaho Transportation Department, prepared by Ayres Associates, Fort Collins, Colo., 40 p., accessed October 16, 2017, at https://www.fhwa.dot.gov/engineering/hydraulics/pubs/idpoa.pdf.
Federal Highway Administration, 1995, Recording and coding guide for the structure inventory and appraisal of the nation's bridges: Washington, D.C., U.S. Department of Transportation, Federal Highway Administration, report no. FHWA-PD-96-001, 124 p., accessed October 1, 2019, at https://www.fhwa.dot.gov/bridge/mtguide.pdf.
Federal Highway Administration, 2012b, Errata sheet—Recording and coding guide for the structure inventory and appraisal of the nation=s [sic] bridges: Washington, D.C., U.S. Department of Transportation, Federal Highway Administration, report no. FHWA-PD-96-001, 6 p., accessed October 16, 2017, at https://www.fhwa.dot.gov/bridge/errata.pdf.
Federal Highway Administration, 2012c, Pier scour in clear-water conditions with non-uniform bed materials: U.S. Department of Transportation, Federal Highway Administration, report no. FHWA-HRT-12-022, 66 p., accessed May 8, 2024, at https://highways.dot.gov/sites/fhwa.dot.gov/files/FHWA-HRT-12-022.pdf.
Federal Highway Administration, 2016, Updating HEC-18 pier scour equations for noncohesive soils: McLean, Va., U.S. Department of Transportation, Federal Highway Administration, report no. FHWA-HRT-16-045, 32 p., accessed May 8, 2024, at https://highways.dot.gov/media/3861.
Federal Highway Administration, 2019, National bridge inventory: U.S. Department of Transportation, Federal Highway Administration web page, accessed October 16, 2019, at https://www.fhwa.dot.gov/bridge/nbi/ascii.cfm.
Federal Highway Administration, 2022, Hydraulic toolbox program, version 5.4.0: U.S. Department of Transportation, Federal Highway Administration software release, accessed October 18, 2022, at https://www.fhwa.dot.gov/engineering/hydraulics/software/toolbox404.cfm.
Fosness, R.L., and Dudunake, T.J., 2024, Hydraulic assessment summary at selected real-time pier scour monitoring sites in Idaho, 2020–2022: U.S. Geological Survey data release, https://doi.org/10.5066/P90332LD.
Henneberg, M.F., 2018, Real-time streambed scour monitoring at two bridges over the Gunnison River in western Colorado, 2016–17: U.S. Geological Survey Scientific Investigation Report 2018–5123, 15 p. [Also available at https://doi.org/10.3133/sir20185123.]
Henneberg, M.F., and Richards, R.J., 2022, Implementing a rapid deployment bridge scour monitoring system in Colorado, 2019: U.S. Geological Survey Scientific Investigations Report 2022–5023, 18 p. [Also available at https://doi.org/10.3133/sir20225023.]
Holnbeck, S.R., 2011, Investigation of pier scour in coarse-bed streams in Montana, 2001 through 2007: U.S. Geological Survey Scientific Investigations Report 2011–5107, 67 p., [Also available at https://doi.org/10.3133/sir20115107.]
Kenney, T.A., 2010, Levels at gaging stations: U.S. Geological Survey Techniques and Methods, book 3, chap. A19, 60 p. [Also available at https://doi.org/10.3133/tm3A19.]
Landers, M., and Mueller, D., 1996, Evaluation of selected pier-scour equations using field data: Transportation Research Record—Journal of the Transportation Research Board, v. 1523, no. 1, p. 186–195. [Also available at https://doi.org/10.1177/0361198196152300123.]
Rydlund, P.H., Jr., and Densmore, B.K., 2012, Methods of practice and guidelines for using survey-grade global navigation satellite systems (GNSS) to establish vertical datum in the United States Geological Survey: U.S. Geological Survey Techniques and Methods, book 11, chap. D1, 102 p. with appendixes. [Also available at https://doi.org/10.3133/tm11D1.]
U.S. Army Corps of Engineers, 2015, Boise River inundation mapping study—Eagle to Star Reach, Ada County, Idaho: U.S. Army Corps of Engineers Walla Walla District, Volume 1, September 2015, 27 p., accessed February 8, 2024, at https://s3.amazonaws.com/wim.usgs.gov/FIM/furnishedFIM/BoiseID/BoiseRiverInundationMappingReport.pdf.
U.S. Geological Survey, 2019, The StreamStats program: U.S. Geological Survey web site, accessed October 10, 2023, at https://streamstats.usgs.gov/ss/.
U.S. Geological Survey, 2024a, USGS 13250000 Payette River nr Letha ID, in USGS water data for the Nation: U.S. Geological Survey National Water Information System database, accessed September 19, 2023, at https://doi.org/10.5066/F7P55KJN. [Site information directly accessible at https://waterdata.usgs.gov/usa/nwis/uv/?site_no=13250000.]
U.S. Geological Survey, 2024b, USGS 13213000 Boise River nr Parma ID, in USGS water data for the Nation: U.S. Geological Survey National Water Information System database, accessed September 19, 2023, at https://doi.org/10.5066/F7P55KJN. [Site information directly accessible at https://waterdata.usgs.gov/usa/nwis/uv/?site_no=13213000.]
U.S. Geological Survey, 2024c, USGS 12414500 St Joe River at Calder, ID, in USGS water data for the Nation: U.S. Geological Survey National Water Information System database, accessed September 19, 2023, at https://doi.org/10.5066/F7P55KJN. [Site information directly accessible at https://waterdata.usgs.gov/usa/nwis/uv/?site_no=12414500.]
Xylem, 2018, Sound velocity vertical errors and what to expect: Xylem web page, accessed October 1, 2023, at https://www.xylem.com/en-us/hypack-blogs/hypack-news-blog/sound-velocity-vertical-errors-and-what-to-expect/.
Glossary
aggradation
General and progressive buildup of the longitudinal profile of a channel bed due to sediment deposition (Federal Highway Administration, 2012a).
approach section
The river channel located about one bridge length upstream of the bridge (Federal Highway Administration, 2012a).
angle of attack
Angle that the flow velocity intersects the bent (or pier), where a flow angle of 0-degrees is parallel to the bent (or pier), and a flow angle of 90-degrees is perpendicular to the bent (or pier).
bent
A type of pier composed of multiple columns that support beams and girders. Bent vertical members are columns or piles located on top of some type of foundation, either a footing or a drilled shaft, generally located below the channel bed.
clear-water scour
Scour at a pier when there is no movement of the bed material upstream of the bridge crossing at the flow causing bridge scour (Federal Highway Administration, 2012a).
Coarse Bed pier scour equation
HEC-18 pier scour design equation. The coarse-bed pier scour equation is only for clear-water conditions where the approach flow velocity is less than the critical velocity for initiation of bed-material motion (Federal Highway Administration, 2012a).
critical velocity
Flow velocity at which movement of non-cohesive sediment particles is initiated, and at which lie-bed scour conditions occur (Federal Highway Administration, 2012a).
flow velocity
Channel-averaged (total flow divided by area) at the approach section (Federal Highway Administration, 2012a).
HEC-18
Hydraulic Engineering Circular No. 18 (Federal Highway Administration, 2012a).
HEC-18 pier scour equation
HEC-18 general design equation, based on the Colorado State University equation, is recommended for both live-bed and clear-water pier scour. The equation predicts maximum pier scour depths (Federal Highway Administration, 2012a).
live-bed scour
Scour at a pier when the bed material in the channel upstream of the bridge is moving at the flow causing bridge scour (Federal Highway Administration, 2012a).
non-cohesive sediment
Granular sediments with little to no clay that do not bond together.
pier
Bents with one column are called piers.
scour
Erosion of streambed or bank material due to flowing water (Federal Highway Administration, 2012a).
Datums
Vertical coordinate information is referenced to the North American Vertical Datum of 1988 (NAVD 88).
Horizontal coordinate information is referenced to the North American Datum of 1983 (NAD 83).
Elevation, as used in this report, refers to distance above the vertical datum.
Supplemental Information
Water year is the 12-month period from October 1 through September 30 and is designated by the calendar year in which the period ends. Thus, the water year ending September 30, 2022, is called “water year 2022.”
For more information regarding the research in this report,
contact the
Director, Idaho Water Science Center
U.S. Geological Survey
230 Collins Road
Boise, Idaho 83702-4250
https://www.usgs.gov/centers/id-water
Manuscript approved on October 1, 2024
Publishing support provided by the U.S. Geological Survey
Science Publishing Network, Tacoma Publishing Service Center
Disclaimers
Any use of trade, firm, or product names is for descriptive purposes only and does not imply endorsement by the U.S. Government.
Although this information product, for the most part, is in the public domain, it also may contain copyrighted materials as noted in the text. Permission to reproduce copyrighted items must be secured from the copyright owner.
Suggested Citation
Fosness, R.L., and Schauer, P.V., 2024, Real-time pier scour monitoring and observations at three scour-critical sites in Idaho, water years 2020–22: U.S. Geological Survey Scientific Investigations Report 2024–5095, 23 p., https://doi.org/10.3133/sir20245095.
ISSN: 2328-0328 (online)
Study Area
Publication type | Report |
---|---|
Publication Subtype | USGS Numbered Series |
Title | Real-time pier scour monitoring and observations at three scour-critical sites in Idaho, water years 2020–22 |
Series title | Scientific Investigations Report |
Series number | 2024-5095 |
DOI | 10.3133/sir20245095 |
Year Published | 2024 |
Language | English |
Publisher | U.S. Geological Survey |
Publisher location | Reston, VA |
Contributing office(s) | Alaska Science Center, Idaho Water Science Center |
Description | Report; vii, 23 p.p.; Data Release |
Country | United States |
State | Idaho |
Other Geospatial | Boise River, Payette River, St. Joe River |
Online Only (Y/N) | Y |