Federal Lands Greenhouse Gas Emissions and Sequestration in the United States—Estimates for 2005–22
Links
- Document: Report (2.18 MB pdf) , HTML , XML
- Data Release: USGS data release - Federal lands greenhouse gas emissions and sequestration in the United States—Estimates for 2005–22 - data
- Project Site: Interactive map (html) - Federal Lands Emissions and Sequestration in the United States: Estimates 2005-22
- Superseded Publications:
- Download citation as: RIS | Dublin Core
Acknowledgments
The estimates of fossil fuel-associated emissions in this report were generated by methods that relied heavily on the work of others. Data repositories used and sources of guidance on the methodology are extensively cited in the text. Specific acknowledgments are necessary for contributors who aided the authors in the interpretation of published materials and provided instruction on making the estimates included here. The authors recognize Sarah Busch, Vincent Camobreco, Mausami Desai, Kenna Rewcastle, Vasco Roma, Christopher Sherry, and John Steller of the U.S. Environmental Protection Agency for assistance in utilizing their methodology, accessing data, and reviewing the manuscript. The authors thank Department of the Interior colleagues Matthew Warren of the Bureau of Land Management and Andrew Haskett and the Data Services team of the Office of Natural Resources Revenue. Thanks are also extended to C. Özgen Karacan and Paul Selmants of the U.S. Geological Survey for helpful reviews.
Abstract
In 2016, the Secretary of the U.S. Department of the Interior requested that the U.S. Geological Survey (USGS) produce a publicly available and annually updated database of estimated greenhouse gas emissions associated with the extraction and use of fossil fuels from Federal lands. The first report in this series included emissions estimates from 2005 to 2014 and were reported for 29 States and two offshore areas. Native American and Tribal lands were not included in that analysis. This report recalculates those previous years (2005–14) with updated data and methods and extends the estimates to 2022. Nationwide emissions from fossil fuels produced on Federal lands in 2022 were 1,081.2 million metric tons of carbon dioxide equivalent (MMT CO2 Eq.) for CO2, 33.4 MMT CO2 Eq. for methane (CH4), and 4.3 MMT CO2 Eq. for nitrous oxide (N2O). Compared to 2005, the 2022 totals represent decreases in emissions for all three greenhouse gases (by 17 percent for CO2, 37 percent for CH4, and 30 percent for N2O). Emissions from fossil fuels produced on Federal lands represent, on average, 21.8 percent of U.S. emissions for CO2, 6.1 percent for CH4, and 1.3 percent for N2O over the 18 years included in this estimate. The trends and relative magnitudes of the greenhouse gas emissions estimated are roughly parallel to the Federal lands fossil fuel production volumes.
In 2021, Federal lands of the conterminous United States stored 70,424 MMT CO2 Eq. in terrestrial ecosystems. Soils stored most of the terrestrial ecosystem carbon (66 percent), followed by live vegetation (25 percent), deadwood (5 percent), and litter (4 percent). Carbon sequestration on Federal lands was highly variable over time, owing primarily to interannual variability in climate and weather, and variability in land use and land cover (LULC) change and disturbances, among these are wildfires and logging. Between 2005 and 2021, Federal lands sequestered an average of 83 MMT CO2 Eq./yr. By subtracting the cumulative effects of LULC and disturbance-related CO2 losses to the atmosphere from the total, we estimate that ecosystems at the national level sequestered CO2 at an annual mean rate of 17 MMT CO2 Eq./yr in a term called the net ecosystem exchange (NEE). This annual NEE sequestration value represents about 1.4 percent of average fossil fuel emissions over the same period.
The USGS estimates presented in this report represent an accounting for the emissions resulting from fossil fuel extraction on Federal lands and the end-use combustion of those fuels, as well as for the sequestration of carbon in terrestrial ecosystems on Federal lands. A combined net CO2 emissions estimate, which is the difference between the emitted and sequestered CO2 from both the fossil fuel and ecosystems estimates, provides context for evaluating the greenhouse gas contributions of activities on these lands. The estimates included in this report can provide context for future energy decisions, as well as a basis to track change in the future.
Introduction
In January 2016, the Secretary of the U.S. Department of the Interior tasked the U.S. Geological Survey (USGS) with producing a publicly available and annually updated database of estimated greenhouse gas emissions associated with the extraction and use (predominantly some form of combustion) of fossil fuels from Federal lands (fig. 1). In response, the USGS began a study of greenhouse gas emissions and carbon sequestration on Federal lands in the United States; that study produced the requested estimates for 2005–14 in 2018 (Merrill and others, 2018). This report is an update, with new data and methods, to that original report with estimates covering 2005–22. This report supersedes the report by Merrill and others (2018); some text from that original report is included here unchanged. National estimates of greenhouse gas emissions and sequestration are published by the U.S. Environmental Protection Agency (2024a) without reporting emissions from Federal lands specifically.
Two main types of Federal lands are included in this report. Federal mineral rights lands are relevant to fossil fuel-associated emissions estimates. Federal surface lands are relevant to ecosystems emissions and sequestration. These two land classes exist because they are often not one and the same. In the United States, the owner of the subsurface mineral rights is not necessarily the same as the surface landowner. Surface ownership is not a factor in mineral leases or the royalties paid to the Federal government. Results in this report are aggregated to the State level to avoid the complex distribution of these two Federal land types within some States. Federal lands, in this report, is a catch-all term encompassing both Federally administered mineral rights lands and Federally administered surface lands; it is used here for brevity in the text where the type of land is clear or does not require differentiation.
This report is separated into two sections: “Fossil Fuel-Associated Emissions of Greenhouse Gases from Federal Lands” and “Terrestrial Ecosystem-Associated Carbon Emissions and Sequestration on Federal Lands.” Both sections include general descriptions of the data sources, methodology, and results specific to the estimates. The report concludes with a discussion of the combined net emissions attributed to Federal lands; these are the estimates produced by summing the emissions and sequestration results from both sections. Appendixes 1 and 2 supply additional detail regarding the data sources and methodology. For ease of comparison between fossil fuel-associated emissions and ecosystems flux estimates, the values in this report are presented in units of “equivalent amounts of carbon dioxide” (CO2 Eq.) gas with positive values meaning emissions and negative values meaning sequestration.
The “Fossil Fuel-Associated Emissions of Greenhouse Gases from Federal Lands” section covers emissions of carbon dioxide (CO2), methane (CH4), and nitrous oxide (N2O) from the extraction and eventual end-use (often combustion) of fossil fuels produced from Federal leases on lands referred to as Federal mineral rights lands. Fossil fuel extraction and combustion produce greenhouse gas (GHG) emissions. Industrial injection of GHG into the subsurface for enhanced hydrocarbon production or for GHG storage are forms of geologic sequestration, however, geologic sequestration is not included in the estimate owing to the small magnitude of current projects on Federal lands and a paucity of available data. This report’s fossil fuel emissions estimate relies heavily on the established methods used by the EPA (referenced specifically throughout the report).
The “Terrestrial Ecosystem-Associated Carbon Emissions and Sequestration on Federal Lands” section deals with the sequestration and emission of carbon, reported as CO2, in terrestrial ecosystems on Federal surface lands. Ecosystems can sequester or emit GHG, therefore this report discusses changes in both carbon stocks and fluxes using a combination of methods established in the scientific literature as well as ongoing USGS research efforts (Sleeter and others, 2022, and references therein).
An independent estimate of GHG emissions associated with the administration of Federal lands is produced by the Bureau of Land Management (BLM). The BLM Specialist Report on Annual Greenhouse Gas Emissions and Climate Trends (Bureau of Land Management, 2024) is a future year emissions projection for National Environmental Protection Act (NEPA) and litigation purposes. There is no overlap in the time period of investigation of the two emissions estimates. The USGS work presented here is meant to document and record emissions and storage of GHG in the near past. BLM’s goal is to project future emissions based on current and potential management decisions to estimate environmental impacts. In addition to the time of investigation, the methods employed are also different, with BLM using a predominantly life cycle assessment (LCA) based approach that is more fitting to their needs and not as appropriate for an emissions inventory.
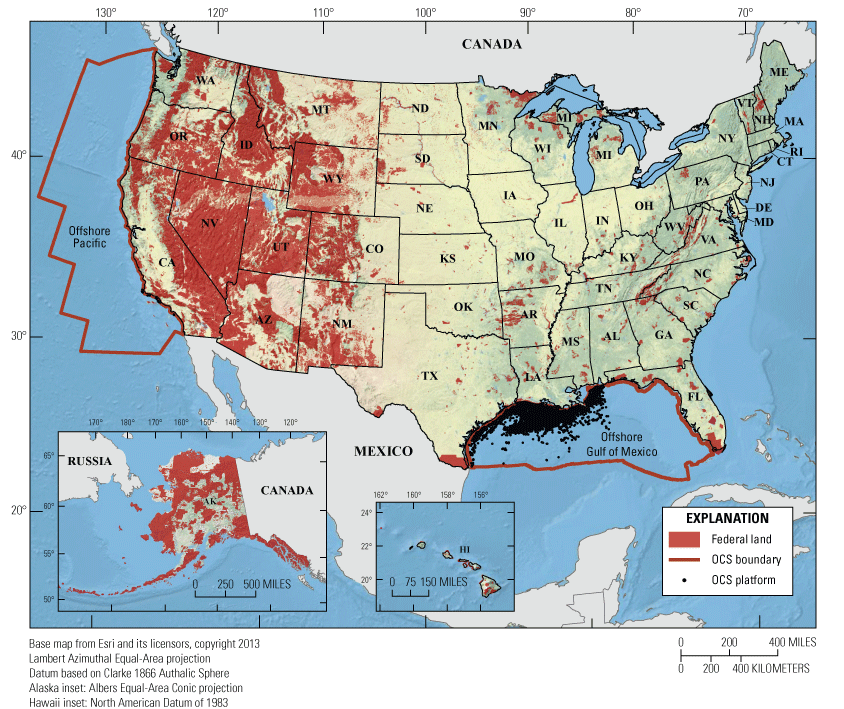
Map showing the onshore Federal surface lands (excluding Native American and Tribal lands) and offshore Federal Outer Continental Shelf (OCS) planning areas (offshore Pacific and offshore Gulf of Mexico) included in the emissions and sequestration estimates. The Federal Atlantic and Alaska OCS planning areas were excluded because they did not have fossil fuel production during the study period. Federal lands were modified from the U.S. Geological Survey Protected Areas Database of the United States (U.S. Geological Survey, 2016), and are not intended to represent the Federal mineral estate lands (not shown). OCS boundaries and platforms are from Minerals Management Service (2006a, b), Bureau of Ocean Energy Management (2011), and Bureau of Safety and Environmental Enforcement (2014). Map reproduced from Merrill and others (2018).
Fossil Fuel-Associated Emissions of Greenhouse Gases from Federal Lands
Introduction
This study includes estimated greenhouse gas (GHG) emissions (CO2, CH4, and N2O) resulting from the extraction and end-use combustion or other use of fossil fuels derived from U.S. Federal mineral rights lands, including offshore areas. The Federal Gulf of Mexico Outer Continental Shelf (OCS) and Pacific OCS planning areas are included in this report and referred to as offshore Gulf of Mexico and offshore Pacific, respectively. No other Federal OCS areas are included in this report because they did not produce fossil fuels during the period of this study.
Emissions are produced through two processes: (1) the combustion of fuel for electricity generation, mechanical work, heating, or use as a feedstock; and (2) the fugitive emission of gases during the processes of extracting and moving fuel. Fugitive emissions were attributed to the areas where the fuels originated; the location of the eventual combustion of the fuel, whether on Federal, State, private, or international territory, was not a factor. If the fuel was sourced on Federal mineral rights lands (Federal leases), it was included in the estimate regardless of where it was used. All references to emissions in this section are associated with fossil fuels extracted from Federal lands in States and offshore areas. Fossil fuels produced from Native American and Tribal lands were not included in this analysis.
Data Sources
Four main types of data support the emissions estimates in this report: (1) Federal lands fuel (oil, gas, natural CO2, and coal) production; (2) emissions from coal mines and oil and gas infrastructure (fugitive emissions); (3) national- and State-level energy-consumption and emissions data for apportioning extracted fuel to end-use fuel type and economic sector; and (4) process and fuel-specific emission factors that determine the volumes of greenhouse gases emitted by combusted fuels. A general discussion of the data sources is provided here, and an in-depth listing of data sources is provided in appendix 1.
Data on Federal mineral lands fuel production, independent of the Federal agency, are collected as part of royalty tracking by the Office of Natural Resources Revenue (ONRR) in the U.S. Department of the Interior. These data are the main input values to the emissions estimate calculations. Publicly available data provided by Office of Natural Resources Revenue (2024) via the Natural Resources Revenue Data (NRRD) website were the sole source of Federal oil and gas production data. State-level coal production data is also available via the NRRD, however, under the methodology employed in this study, coal mine-level data are required. ONRR provided mine-level coal production data to the USGS; these data are considered proprietary and not for release. ONRR also provided natural CO2 production data to the USGS by request, these data are not publicly available. Overall, the input data used in this report includes coal, oil, natural gas, and natural CO2 production from Federal mineral lands in 29 States and the Federal offshore Pacific and offshore Gulf of Mexico from 2005 to 2022. No fossil fuels were produced on Federal lands in the remaining 22 States or the Atlantic and Alaska offshore areas during the study period.
Fugitive emissions include measured or estimated releases of CH4 from underground and surface coal mines (both active and abandoned) and CO2, CH4, and N2O emissions from oil and gas infrastructure such as wells, pipelines, compressors, and storage tanks. Coal mine data were provided by the EPA but compiled by the Mine Safety and Health Administration (MSHA). The MSHA or operator measures emissions from underground coal mines, and mines exceeding a certain emissions threshold also submit emissions estimates and measurements to the EPA’s Greenhouse Gas Reporting Program (GHGRP).
National- and State-level energy production, sector usage, and refining statistics are also required to complete the emissions estimate. The source of these statistics is often the U.S. Energy Information Administration (EIA), which is part of the U.S. Department of Energy. The exception is the natural CO2 production usage, which is provided by the EPA GHGRP. These published statistics (refer to references in appendix 1) were used to generate ratios for apportioning the Federal production volumes to end-use fuel types and economic sectors. This allocation was necessary because it is often impossible to track coal, oil, or gas from a specific Federal source as it moves through the fuel supply chain, including contributions from private and State lands in pipelines, containers, or shipments, to its eventual endpoint where emissions are generated. Ratio-based scaling of Federal fuel volumes to end uses and economic sections based on national- or State-level production volumes was used throughout the estimate calculation for amounts of products refined from crude oil, industrial uses of natural gas, sector usage for coal combustion, and natural CO2 uses.
Emission factors are the values used to convert volumes of fuel combusted or extracted into amounts of greenhouse gases emitted. These conversions differ by the input fuel and the sector of the economy where that fuel is consumed. The EPA’s Inventory of U.S. Greenhouse Gas Emissions and Sinks (hereinafter U.S. GHG Inventory; U.S. Environmental Protection Agency, 2024a), an annual publication of total U.S. GHG emissions to meet U.S. Government annual commitments under the United Nations Framework Convention on Climate Change (UNFCCC), is the source of all emission factors used in this report (unless otherwise indicated). In addition, the EPA’s U.S. GHG Inventory’s calculation methodology is the basis for the methods used in this report.
General Methodology
The methods used in this report to estimate GHG emissions from fossil fuels produced on Federal lands are described in general in this section and in detail in appendix 1. The USGS methods are adapted from the more exhaustive methodology described in the EPA’s U.S. GHG Inventory and its associated annexes (U.S. Environmental Protection Agency, 2024a, b). The general procedure for all emissions estimates includes (1) apportioning the input data (fuel volumes or activity information) to the proper sector for emissions tabulation, and (2) employing a sector or fuel specific emissions factor to convert that input/activity data into GHG emissions. The process explained in this iteration of the report is similar to what is described in the initial 2005–14 report (Merrill and others, 2018). While most calculations are the same as in the prior publication, they are still described here with the methodological changes specifically noted in the following text.
The main methodological changes from the previous emissions report involve bringing methods more in line with those included in the most recent U.S. GHG Inventory (U.S. Environmental Protection Agency, 2024a) and the inclusion of new GHG estimates. For example, a report-wide change is that the integration of U.S. GHG Inventory sourced uncertainty estimates are now sector specific and inclusive of both activity data and emissions factor uncertainty. The previous version of this report only discussed the uncertainty of the whole study. Emissions associated with natural CO2 use have been added to these estimates. Federal-lease plugged oil- and gas-well fugitive emissions are also now included in the report. Inclusion of unplugged idle wells is planned for future versions of the estimate. Refer to appendix 1 for a detailed list of data sources and steps for the GHG emissions estimates included in this report.
Stationary Combustion Emissions
In 2022, Federal lease oil production totaled 1.056 billion barrels, natural gas production totaled 3.790 trillion cubic feet, and coal production totaled 262.694 million short tons (Office of Natural Resources Revenue, 2024; Office of Natural Resources Revenue, written communication, 2024). Emissions from these fuels are included in either the stationary or mobile combustion estimates.
Stationary emissions encompass greenhouse gas emissions from the combustion of fossil fuels in nonmobile (nontransportation) sectors. These sectors include the combustion of coal for electricity generation, commercial and residential use, industrial use, and coke production for metallurgical applications. Burning natural gas for electricity generation and the use of natural gas as a feedstock in industrial processes and refined crude oil fuel products that are burned to generate heat or electricity, also represent stationary emissions.
Various emission factors were used to convert the amounts of fuel combusted into estimates of emissions. Emission factors available in the literature are generally fuel or end-use sector specific. Because of their various uses, the emission factors for stationary combustion of liquid fuels are fuel specific. Emission factors for natural gas are based on the sectors of the economy where it is used. Coal combustion also uses sector specific emissions factors based on energy content, rather than coal volumes, therefore accurate coal rank data (a measure of the coal’s carbon content) is an important input to make the conversions.
Mobile Combustion Emissions
Calculations of mobile emissions are complicated by the changing technology, efficiency, and total mileage of vehicles driven, flown, and piloted in the United States in any given year. Therefore, the USGS method leans heavily on the national emissions calculations included in the U.S. GHG Inventory and associated annexes (U.S. Environmental Protection Agency, 2024a, b). The national-level data from the EPA were devolved to ratios of GHG emissions per gallon of fuel. The ratios were then multiplied by the gallons of fuels that were estimated to have been refined from crude oil produced from Federal lands. The USGS estimate therefore provides emissions estimates for only the fuels that the EPA has estimates for—motor gasoline, aviation gasoline, jet kerosene, diesel oil, residual fuel oil, and liquefied petroleum gas—fuels that account for nearly all mobile emissions.
Petroleum and Natural Gas Systems Emissions
This category covers fugitive (noncombustion) emissions calculations for greenhouse gases released during the extraction, processing, and transportation (for example, through pipelines) of natural gas and oil from Federal leases, as well as platforms in Federal offshore areas. In 2022, Federal leases included 26,861 active oil and 42,933 active gas wells, 19,157 known plugged and abandoned (P&A) oil wells, and 10,763 P&A gas wells (Bureau of Land Management, National Oil and Gas Program, written communication, 2024). In the Gulf of Mexico Federal waters, there were 1,096 oil platforms and 113 gas platforms (U.S. Environmental Protection Agency, 2024c, d). Emissions from these infrastructure elements are generally referred to as fugitive emissions. All active oil and gas production fugitive emissions estimates made by the USGS follow a national ratio-based method similar to that used for mobile emissions. Because of the complexity involved in determining the emissions from natural gas and oil infrastructure, the USGS produced a per-well ratio of emissions, from the U.S. GHG Inventory’s national emissions estimates (U.S. Environmental Protection Agency, 2024b). The ratio includes fugitive emissions from production through distribution for gas and from production through refining for oil. This ratio was multiplied by the number of wells producing oil and gas from Federal lands (Bureau of Land Management, written communication, 2024) to estimate the emissions associated with oil and gas infrastructure on those lands.
Oil and gas wells that have been plugged and abandoned are included in this report for the first time and are included with other fugitive emissions in this section. Refer to appendix 1 for more details on the emissions calculation. Orphaned wells, those that are unplugged and inactive and without a responsible party are uncommon on Federal mineral lands owing to the structure of the lease agreements. Therefore, these wells were not included in the emissions estimate though they would fall under an idle well category planned for future inclusion.
For offshore platforms, the EPA has produced emissions factors that are per platform, per volume produced, and per energy content of fuel flared. These rates were used to determine the emissions associated with platforms producing in the Federal offshore Pacific and offshore Gulf of Mexico areas.
Natural Carbon Dioxide Production Emissions
Emissions associated with natural CO2 production and use are included for the first time in this iteration of the USGS Federal lands GHG emissions estimates. These emissions are estimated over the full 2005–22 time series of investigation. Natural CO2 production data, based on sales royalties from the ONRR, are the main input to the calculation. Production of natural CO2 from Federal leases ranged from 336 to 626 billion cubic feet (bcf) or 9.52 to 17.7 billion cubic meters (bcm) between 2005 and 2022. Production totaled 364 bcf (10.3 bcm) in 2022 (Office of Natural Resources Revenue, written communication, 2024). Enhanced oil recovery using CO2 (CO2-EOR) accounted for 93 percent of U.S. natural CO2 usage in 2022 and was as high as 99.7 percent in Colorado (U.S. Environmental Protection Agency, 2024e). During CO2-EOR activities, the CO2 is eventually left in the subsurface, possibly after multiple rounds of CO2 injection and subsequent oil production. Therefore, CO2 volumes used in CO2-EOR are not considered as emissions here. In this method, as in the EPA’s U.S. GHG Inventory, other industrial end uses of natural CO2, such as food and beverage, chemical production, greenhouse operation, and fire suppression products are considered an eventual GHG emission to the atmosphere.
Active Coal Mine Emissions
Methane emissions from active underground and surface coal mines were estimated using methods outlined in the U.S. GHG Inventory (U.S. Environmental Protection Agency, 2024b). In 2022, there were 35 active mines producing coal from Federal leases; of these, 24 were surface mines and 11 were underground (Office of Natural Resources Revenue, written communication, 2024). Methane gas is released both during mining and after mining as coal degasses while in transport and processing. Emissions data for active underground coal mines are measured in the mine by the MSHA or the operators themselves, which then are provided to the EPA. For large emitters (>17,579 metric tons of CO2 Eq. per year), underground mines also report to the EPA Greenhouse Gas Reporting Program. Active surface mine emissions and also postmining emissions from surface and underground mines cannot be directly measured. Therefore, their emission rates are based on the amount of coal produced from the mine, as well as a basin-specific emission factors. A significant change in the methodology for estimating emissions from underground mines with this iteration of the report was apportioning the reported emissions for the whole mine by the proportion of total coal production that was from Federal leases. The previous methodology considered the entire mine’s active underground mine emissions as Federal, if there was any coal production from Federal leases regardless of the proportion.
Abandoned Underground Coal Mine Emissions
Even after an underground mine has stopped actively producing coal, the remaining coal releases CH4 gas for some time. When permanently closed, an underground mine is sealed, vented, or flooded with groundwater, or a combination of the three. The method of abandonment will affect the amount and rate of CH4 released. In general, mines will release the most CH4 immediately after being permanently closed, and the rate will decrease over time. The rate of decline and the effects of the geology and method of abandonment are explained in the EPA’s methodology for estimating abandoned mine emissions (Franklin and others, 2004; U.S. Environmental Protection Agency, 2004). We used a slightly simplified nonprobabilistic version of the method used by the EPA. In the 2022 year, abandoned underground coal mine emissions were estimated for eight mines that had produced from Federal coal leases. A methodological change similar to that described in the active underground mines section was also applied here where abandoned mine emissions are assigned based on the average Federal proportion of whole mine coal production. Abandoned mine methane is an active area of research at the USGS (Karacan and Warwick, 2019) and this methodology may be modified for future estimates.
Uncertainty of Emissions Estimates
In this report, emissions uncertainty is represented as a low, central, and high bound value for each calculation. The low and high bounds are percentages of the central value. We employ the sector- and total-specific uncertainty bounds provided in the U.S. GHG Inventory (U.S. Environmental Protection Agency, 2024a). Uncertainties produced by the EPA follow the Intergovernmental Panel on Climate Change (IPCC)-recommended Tier 2 uncertainty estimation methodology, a Monte Carlo stochastic simulation technique (Intergovernmental Panel on Climate Change, 2000; U.S. Environmental Protection Agency, 2024a). We believe the EPA’s method of quantifying uncertainty is applicable to the methodology used here because the USGS uses a slightly modified and simplified version of the EPA methodology in the U.S. GHG Inventory.
The EPA reported uncertainty on GHG emissions estimates is generally smaller for combustion calculations than for fugitive emissions from extractive activities. This difference in uncertainty is intuitive because combusted materials are measured for sale and therefore are well constrained in their quantities, whereas the amounts of gases emitted during extraction and transport (fugitive emissions) are often estimated or scaled up from smaller sampling efforts, resulting in higher uncertainty. For example, the U.S. GHG Inventory cited uncertainty with a 95-percent confidence interval ranging from -2 to 4 percent of the mean CO2 emissions from stationary fossil fuel combustion in 2022 (U.S. Environmental Protection Agency, 2024a). Conversely, fugitive emissions estimates exhibit wider ranges of uncertainty, with petroleum systems emissions (active and plugged wells, offshore platforms) ranging from -19 to 25 percent for CO2, and -18 to 23 percent for CH4. The EPA reported natural gas systems uncertainties have similar ranges of -12 to 15 percent for CO2 and -18 to 17 percent for CH4. Owing to the significantly larger proportion of emissions tied to the combustion of fossil fuels (lower uncertainty), the total estimated low and high bounds show less range than in individual section uncertainties. The total uncertainties of the U.S. GHG Inventory estimates were between -2 and 4 percent, -14 to 14 percent, and -17 to 26 percent for CO2, CH4 and N2O, respectively. Because totals have lower uncertainty ranges than their constituent sector level uncertainty ranges, low and high bound totals cannot be produced through their summation. This is discussed further in the appendix 1 section “Precision, Rounding, Totals, and Uncertainty of Emissions Estimates.”
Results
The USGS estimates of emissions from the combustion of fossil fuels produced on Federal lands include output values for CO2, CH4, and N2O gases in 29 States, the offshore Pacific, and offshore Gulf of Mexico for the years 2005 through 2022. Results from this estimate are presented by sector and by gas; the total number of output estimates are 19 for CO2, 21 for CH4, and 18 for N2O, plus a number of sector totals. These results, as well as their low and high bound values are available in the data release for this report (Merrill and others, 2024). Table 1 contains a summary of fossil fuel-associated sector emissions estimates for Federal lands in 2022. All results are reported in million metric tons of CO2 equivalent (MMT CO2 Eq.) to enable direct comparison of the different gases. To make the conversion, the amounts of gases are multiplied by their global warming potential (GWP), a factor that accounts for the effect a specific gas has in warming the atmosphere relative to the effect of CO2. GWPs are specific to different time spans of consideration, commonly 100 or 20 years, and are updated regularly as research progresses. The values for the three gases in this report are based on 100-year GWP from the IPCC’s 5th assessment report (AR5) (Intergovernmental Panel on Climate Change, 2013). While updated GWP values were released more recently (AR6) (Intergovernmental Panel on Climate Change, 2021), the EPA still uses AR5 GWP values to satisfy United Nations reporting requirements. Because this report commonly compares results with the U.S. GHG Inventory, we use the AR5 GWP values as well. Those values are 1 for CO2, 28 for CH4, and 265 for N2O (Intergovernmental Panel on Climate Change, 2013).
Nationwide emissions from fuels extracted from Federal lands in 2022 were 1,081.2 MMT CO2 Eq. for CO2, 33.4 MMT CO2 Eq. for CH4, and 4.3 MMT CO2 Eq. for N2O. The total uncertainty ranges on these 2022 estimates are 1,059.5 to 1,124.4 MMT CO2 Eq. for CO2, 28.8 to 38.1 MMT CO2 Eq. for CH4, and 3.5 to 5.4 MMT CO2 Eq. for N2O (appendix 1, table 1.8). Total emissions from the production and combustion of fossil fuels produced on Federal lands for the years 2005–22, as well as comparisons to U.S. fossil fuel and U.S. total emissions (U.S. Environmental Protection Agency, 2024a), are presented in table 2. Calculated average values from table 2 show that Federal lands fuels emissions from 2005 to 2022 accounted for 21.8 percent of U.S. total CO2 emissions, 6.1 percent for CH4, and 1.3 percent for N2O. Relative to total U.S. fossil fuel emissions, the same Federal lands fuels emissions accounted for 22.6 percent of CO2 emissions, 13.6 percent for CH4, and 5.3 percent for N2O.
In 2022, Wyoming, offshore Gulf of Mexico, New Mexico, Colorado, and Utah had the highest CO2 emissions from fuels produced on Federal lands (fig. 2). The CO2 emissions attributed to Federal lands in Wyoming are 41 percent of the total from Federal lands in all States and offshore areas combined. Emissions estimates for the release of CH4 are also highest for Federal lands in Wyoming (36 percent), followed by New Mexico, offshore Gulf of Mexico, Colorado, and Utah (fig. 3). The figures and tables in this report only summarize the results and are not intended to be a complete presentation of all results. The full dataset is available online (Merrill and others, 2024).
Table 1.
National totals and subtotals for several categories of greenhouse gas emissions associated with the combustion and extraction of fossil fuels from U.S. Federal lands, 2022.[The full dataset associated with this report (Merrill and others, 2024) contains similar data for 29 States and two offshore areas for 2005–22. All nonbold values are sector subtotals; the number of significant figures indicates the precision in the underlying State-level estimates. Values are in million metric tons of carbon dioxide equivalent (MMT CO2 Eq.). The emission category totals are reported to 2 decimal places and total Federal lands values are reported to 1 decimal place. CO2, carbon dioxide; CO2-EOR, enhanced oil recovery using CO2; CH4, methane; N2O, nitrous oxide; –, value not calculated]
Table 2.
National totals for greenhouse gas emissions associated with the combustion and extraction of fossil fuels from U.S. Federal lands, 2005–22.[U.S. fossil fuels and U.S. total emissions are from the U.S. Environmental Protection Agency (2024a). All values are in million metric tons of carbon dioxide equivalent (MMT CO2 Eq.). CO2, carbon dioxide; CH4, methane; N2O, nitrous oxide]
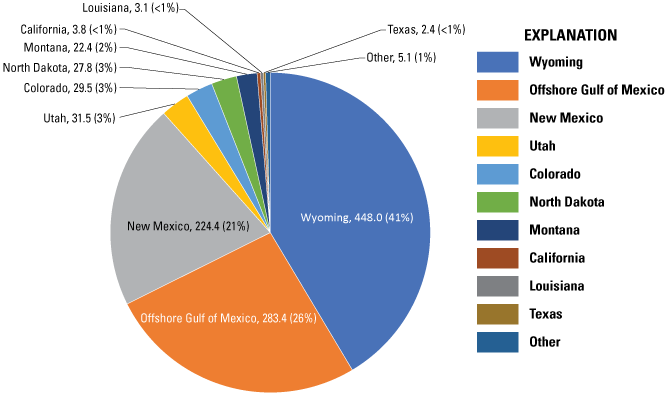
Pie chart showing carbon dioxide emissions associated with the extraction and combustion of fossil fuels produced from Federal lands in the 10 States or offshore regions with the highest emissions in 2022. Emissions are reported in million metric tons of carbon dioxide equivalent (MMT CO2 Eq.). %, percent.
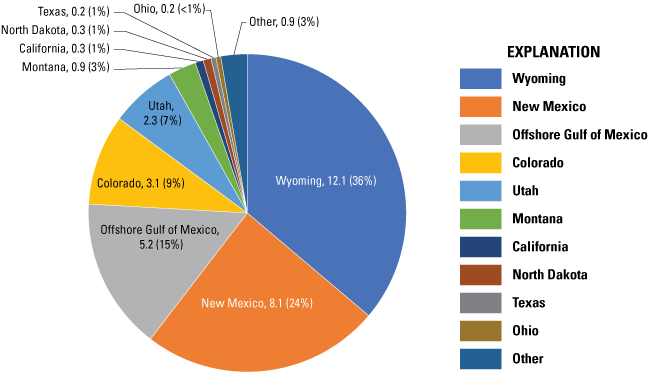
Pie chart showing methane emissions associated with the extraction and combustion of fossil fuels produced from Federal lands in the 10 States or offshore regions with the highest emissions in 2022. Emissions are reported in million metric tons of carbon dioxide equivalent (MMT CO2 Eq.). %, percent.
Discussion
The results demonstrate that the trends and relative magnitudes of the GHG emissions estimated are roughly parallel to the Federal lands production volumes (Office of Natural Resources Revenue, 2024). Figure 4 shows the correlated declining trends in combined (oil, gas, coal) fossil fuel production, represented in total energy content units (U.S. Energy Information Administration, 2024a) and the emissions associated with those fuels for the nation as well as the top three places of origin for emissions. States that produced the most fuel from Federal lands resulted in the highest estimated emissions for CO2, CH4, and N2O when those fuels were produced and combusted. Trends in emissions over the 18-year timeframe reflect changes in production volumes; however, in States where multiple fuels were produced, these relationships may not be evident or direct. Although emission factors, numbers of producing wells, vehicle efficiency, and sector usage rates all change from year to year, they have minimal effect on the final emissions results compared to the amount of fossil fuel production. Any significant changes in production will result in a similar change in the attributed emissions.
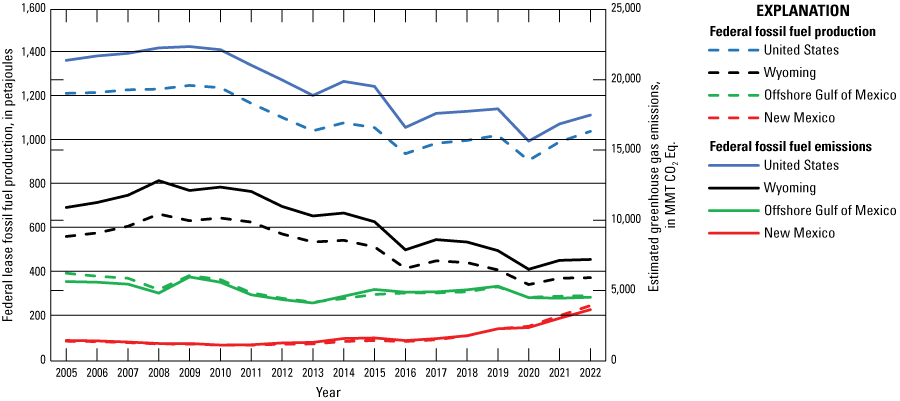
Graph of combined (oil, gas, and coal) Federal lease fossil fuel production in petajoules of energy content compared to estimated greenhouse gas emissions in million metric tons of CO2 equivalent (MMT CO2 Eq.), 2005–22. Data presented is Federal emissions for total United States, as well as Wyoming, New Mexico, and offshore Gulf of Mexico. Note that 1 petajoule = 278 gigawatt hours, or roughly the amount of energy needed to power 12,000 U.S. homes for a year (U.S. Energy Information Administration, 2024b).
Emissions from fossil-fuel activities on Federal lands have declined over time. Figure 5 depicts the emissions for each year relative to the emissions from 2005, the first year of this estimate. After increasing from 2005 to a peak in 2008–2010, Federal emissions generally declined until 2022. The 2022 totals represent decreases in emissions for all three greenhouse gases relative to 2005 values, with reductions of 17 percent for CO2, 37 percent for CH4, and 30 percent for N2O. The main driving factor of this trend is reduced fossil fuel production and fuel switching. However, although not explicit in the data, the effects of emissions reductions through engineering improvements and other mitigations may have driven this trend as well. The decrease in CH4 emissions from 2020–2022, while CO2 and N2O emissions have increased, is mostly due to changes in emissions factors used in the petroleum systems fugitive emissions calculations. Those factors are based on U.S. Environmental Protection Agency (2024a) reported 2022 CH4 emissions from production activities and pneumatic controllers, which are significantly lower than previous years. Methane emissions reductions were also present in natural gas systems, and underground coal mines, but to a lesser extent.
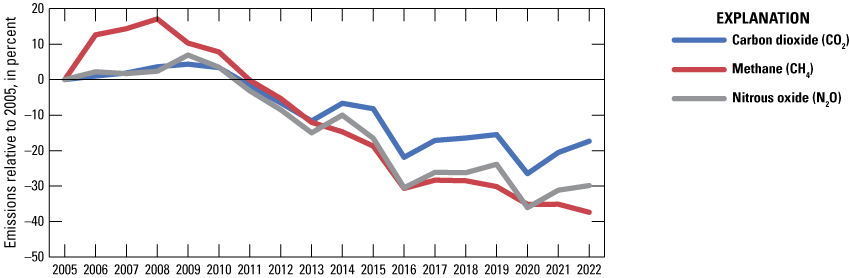
Graph of estimated Federal lease-associated greenhouse gas emissions presented as a percentage relative to the 2005 initial year of this report. Results for three greenhouse gases (carbon dioxide [CO2], methane [CH4], and nitrous oxide [N2O]) are provided.
The reduction in estimated fossil fuel-associated Federal lands emissions from 2005 to 2022 is also observed in both United States fossil fuel and total emissions reported by the EPA (table 2), however, the reduction in Federal emissions is marginally greater. This is evident in the slight declining slope of the lines in figure 6, with Federal lands emissions plotted as a percentage of U.S. fossil fuel and total emissions. For CO2, Federal emissions, as a share of the U.S. fossil fuel and total emissions, have declined from a 2009 peak of nearly 25 percent to around 21 percent in 2022, despite a slight increase in recent years (fig. 6). Similar declining trends were seen in both CH4 and N2O. Significant CH4 and N2O emissions are also produced through noncombustion activities like agriculture, livestock, landfills, and wastewater treatment, therefore the Federal fossil fuel percentage of these gases compared to total U.S. emissions is lower. When comparing Federal fossil fuel to U.S. fossil fuel emissions, the relatively lower percentages for CH4 and N2O compared to CO2 are due to the different mix of oil, gas, and coal production between Federal and non-federal lands as well as differences in fugitive emissions. Overall, while U.S. total, U.S. fossil fuel, and Federal lease fossil fuel emissions are all decreasing, the latter is decreasing by a slightly larger amount.
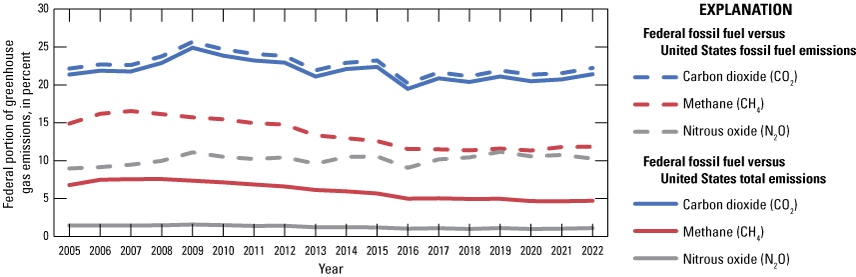
Graph of estimated Federal lease-associated greenhouse gas emissions presented as a percentage relative to the U.S. fossil fuel and U.S. total emissions estimated in the U.S. greenhouse gas (GHG) Inventory, 2005–22 (U.S. Environmental Protection Agency, 2024a). Results for three greenhouse gases (carbon dioxide [CO2], methane [CH4], and nitrous oxide [N2O]) are provided.
Terrestrial Ecosystem-Associated Carbon Emissions and Sequestration on Federal Lands
Introduction
For the ecosystems emissions and sequestration part of this report, we estimated the annual amount of CO2 that was stored and emitted by terrestrial ecosystems, including forests, grasslands, shrublands, and wetlands. Plants convert CO2 to carbon through photosynthesis, which is expressed as gross primary productivity (GPP). Refer to table 3 for a summary of the terms introduced in this section. The terms include stocks, the amount of carbon present in a carbon reservoir, and fluxes, annual changes to those stocks. Autotrophic respiration (Ra) is the release of CO2 back to the atmosphere by plants through respiration. The difference between GPP and Ra is referred to as net primary productivity (NPP), or the amount of CO2 fixed or stored in new vegetation each year. Ecosystems also respire CO2 through the decay and decomposition of dead organic matter (deadwood and litter), referred to as heterotrophic respiration (Rh). The total net ecosystem productivity (NEP) can thus be estimated as GPP + (Ra + Rh).
Changes to ecosystems, typically caused by urbanization, expansion and contraction of agricultural land, afforestation, wildfires, and forest harvest are considered forms of land use and land use and land cover (LULC) change. These changes typically result in the removal of carbon from the ecosystem and are therefore considered emissions or removals for the purpose of this report. Combustion of carbon associated with land use and land-use change and disturbance are subtracted from NEP to estimate net ecosystem exchange (NEE), which reflects the exchange of carbon stored within ecosystems and the atmosphere on an annual basis. NEE is an appropriate value for comparison with annual fossil fuel-associated emissions and is used again in this report where the concept of net emissions is introduced. Additionally, we report net biome productivity (NBP), which includes carbon removed from ecosystems but not necessarily released to the atmosphere. When forests are harvested, carbon enters the harvest wood products (HWP) pool, where it can remain for decades or longer. In this report, harvested wood products are not considered in the calculation of net emissions to the atmosphere and are assumed to be lateral fluxes of carbon. In the full dataset associated with this report (Merrill and others, 2024), we provide estimates of most of the stocks and fluxes in table 3. Estimates are included for carbon stocks such as total ecosystem carbon (TEC), live, soil, deadwood, litter, and annual carbon fluxes such as, NPP, NEP, NBP, NEE, Rh, combustion, and harvest, for Federal lands of the conterminous United States for the period 2005–21.
Table 3.
Explanation of carbon stock and flux terms from the terrestrial ecosystem sequestration calculations.[Terms are grouped by carbon stock or typical flux type. This table may be useful when examining the results in tables 4 and 5. CO2, carbon dioxide; LULC, land use and land cover]
Data Sources and Methodology
This USGS study used the Land Use and Carbon Scenario Simulator (LUCAS) to model changes in land use and land cover, natural disturbances, and climate, and the corresponding changes in ecosystem carbon balance. LUCAS is a spatially explicit simulation model used to forecast landscape dynamics; it uses a fully coupled state-and-transition simulation model (STSM) to track changes in land use and land cover (Daniel and others, 2016) along with a stock and flow model (SF) to track changes in ecosystem carbon balance (Daniel and others, 2018). The stock flow model follows a carbon gain-loss approach, where carbon is transferred between predefined carbon pools each year. The LUCAS model was run on an annual time step from 2001–2021 at a spatial resolution of 1-km x 1-km for the entire conterminous United States. To capture model uncertainty, we ran 20 Monte Carlo realizations of the model using a range of forest type growth rates.
The LUCAS model has been used for a range of applications including estimating LULC change and ecosystem carbon dynamics in Hawaii (Sleeter and others 2017; Selmants and others, 2021), the conterminous United States (Sleeter and others, 2018), and in California to assess the effects of alternative scenarios of LULC and climate change (Sleeter and others, 2019) and the effect of natural climate solutions (Marvin and others, 2023). A complete description of the LUCAS approach can be found in Sleeter and others (2022) including the primary differences between the approach used in this study and efforts such as the EPA’s U.S. GHG Inventory and other process-based modeling efforts.
Initial state class maps (for example, land cover and land use) for the year 2001 were developed using land cover maps from the National Land Cover Database (NLCD; Homer and others, 2007), existing vegetation type (EVT) from the Landfire program (Rollins, 2008), location and types of wetlands from the National Oceanic and Atmospheric Administration’s (NOAA) Coastal Change and Analysis Program (C-CAP) (National Oceanic and Atmospheric Administration, 2024), and location and types of grasslands and shrublands from NLCD’s Rangeland Condition Monitoring Assessment and Projection (RCMAP) project (Rigge and others, 2024). In total, we identify and model 52 unique LULC classes. Initial stand age for all forest areas was derived from data from Williams and others (2020).
This study utilized annual and semiannual maps of land cover and ecosystem disturbances to track changes over time. Data from the NCLD for the years 2001, 2004, 2006, 2008, 2011, 2013, 2016, 2019, and 2021 were analyzed to identify areas where urbanization, agricultural expansion, and agricultural contraction occurred between 2001 and 2021. Areas of forest harvest were identified using annual Landfire Disturbance data (LANDFIRE, 2023) and areas where fire occurred (low, medium, and high severity) were identified using annual maps from the Monitoring Trends in Burn Severity project (Eidenshink and others, 2007).
To model the effects of climate variability and change on ecosystem carbon cycling we used annual maps of temperature and precipitation from the Parameter-elevation Regressions on Independent Slopes Model (PRISM) database (PRISM Climate Group, 2023). An external model of net primary productivity (NPP) was derived for forest and non-forest cells (Del Grosso and others, 2008) and used to modify the annual baseline NPP rates of the model (Sleeter and others, 2022). Additionally, annual temperature maps were used to scale the annual rate of decomposition of dead organic matter (Sleeter and others, 2022).
Federally administered surface lands in the conterminous United States (hereinafter Fed-CONUS) were identified from the USGS Protected Areas Database (PADUS) version 1.4 (U.S. Geological Survey, 2016). A mask was created by selecting all PADUS polygons that had Federal owner designation. These included lands managed by the Bureau of Land Management, U.S. Department of Agriculture Forest Service, U.S. Department of Defense, U.S. Department of Energy, Bureau of Reclamation, U.S. Army Corps of Engineers, National Park Service, National Oceanic and Atmospheric Administration, Natural Resources Conservation Service, and other Federal agencies. Native American and Tribal lands were not included in this analysis. The Federal lands layer was combined with U.S. State boundaries from the U.S. Census Bureau (https://www.census.gov/geographies/mapping-files/time-series/geo/carto-boundary-file.html) to provide a unique State identification code for each Federal land cell. Although small changes in the extent of Federal lands may have taken place over the period of study, we assumed that the extent of Federal lands was unchanging over time.
We used the Federal lands mask described above to extract the 14 key LUCAS carbon stock and flux variables listed in table 3. The carbon stocks were total ecosystem carbon (TEC), live vegetation, soil, deadwood, and litter. Carbon sequestration fluxes included, gross primary productivity, net primary productivity, net ecosystem productivity, net biome productivity, and net ecosystem exchange. The carbon emission fluxes were autotrophic respiration, heterotrophic respiration, combustion associated with wildfire and LULC change, and carbon removals associated with forest harvest and LULC change. The LUCAS output data consist of tabular data summaries stored in a database format and annual maps of each carbon stock and flux variable at 1-km x 1-km resolution. Each map was stored as a GeoTIFF file with each layer representing a single year between 2001 and 2021. To facilitate comparisons with the fossil fuel-associated emissions estimates and EPA’s U.S. GHG Inventory estimates, all carbon stocks and sequestration are represented as negative values and all carbon releases, removals, or losses to the atmosphere are represented as positive values. Additional methodological details are provided in appendix 2 of this report.
Results
The carbon sequestration results were calculated for various timeframes depending on geographic area and specific output. For comparison with the fossil fuel-associated emissions, results were provided by State and for the years 2005–21 only. The full dataset supporting the following summary of results is available in the companion data release to this report (Merrill and others, 2024). In 2005, lands of the Fed-CONUS stored 71,101 MMT CO2 Eq. in terrestrial ecosystems (total ecosystem carbon [TEC]). By 2021, carbon storage was estimated at 70,424 MMT CO2 Eq., representing a decrease of 0.9 percent, or 677 MMT CO2 Eq. Soils stored most of the TEC (66 percent), followed by live vegetation (25 percent), deadwood (5 percent), and litter (4 percent). Over the 17-year period, there was a mean net loss of 1,177 MMT CO2 Eq. in live vegetation and a mean net loss of 134 MMT CO2 Eq. in litter, while 143 MMT CO2 Eq. was sequestered in soils, and 490 MMT CO2 Eq. was added to deadwood pools (table 4).
Between 2005 and 2021, Federal lands sequestered an average of 83 million metric tons of carbon dioxide equivalent per year (MMT CO2 Eq./yr) (annual NEP), the difference between an average gain through NPP of 1,534 MMT CO2 Eq./yr and an average loss through ecosystem Rh of 1,445 MMT CO2 Eq./yr. Additional losses of carbon from terrestrial ecosystems resulted from wildfire, logging, and other land use and land use changes. We estimated direct emissions to the atmosphere resulting from LULC and disturbances at 67 MMT CO2 Eq./yr. By subtracting the cumulative effects of LULC- and disturbance-related CO2 losses to the atmosphere from the NEP, we estimated that ecosystems at the national level sequestered CO2 at a mean rate of 17 MMT CO2 Eq./yr (net ecosystem exchange [NEE]) with an additional 52 MMT CO2 Eq./yr transferred from ecosystems to HWP. We did not model the decay and turnover of HWP pools in this report.
Carbon sequestration on Federal lands was highly variable over time, owing primarily to interannual variability in climate and weather and the variability in LULC and disturbances. Between 2005 and 2021, mean annual NPP for lands in the Fed-CONUS ranged from 1,306 to 1,734 MMT CO2 Eq./yr. Over the same period, NEE varied between -348 MMT CO2 Eq./yr and 376 MMT CO2 Eq./yr, meaning sequestering and also emitting carbon to the atmosphere (fig. 7). The large variation in the size of the land sink was due, in part, to variability in the magnitude of disturbances. For example, combustion emissions in the Fed-CONUS ranged from 15 MMT CO2 Eq./yr in 2019 to 165 MMT CO2 Eq./yr in 2020 (fig. 7).
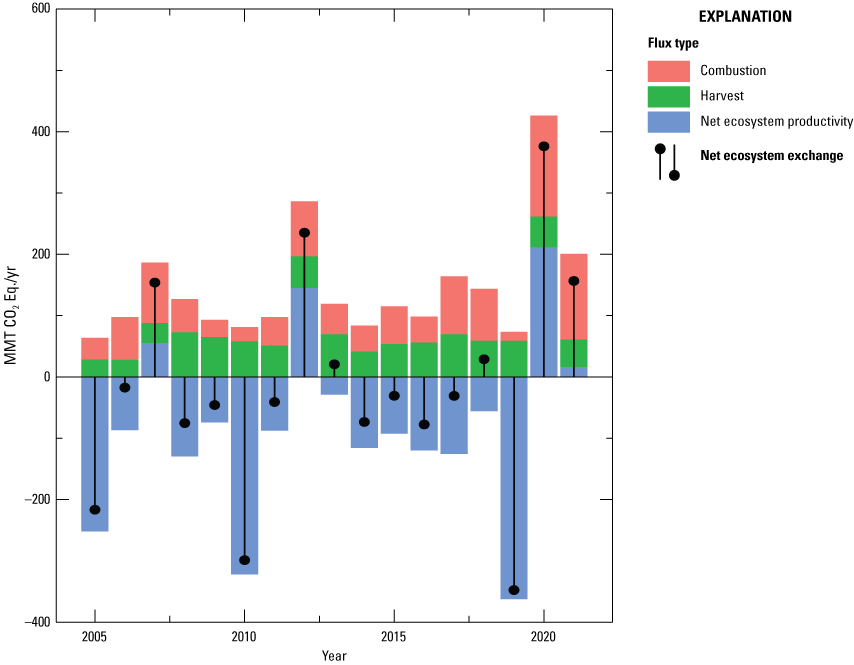
Graph of carbon fluxes on Federally administered surface lands in the conterminous United States (Fed-CONUS), 2005–21. Combustion (including fires), harvested wood products (Harvest), and net ecosystem productivity (NEP) are shown as bars. Net ecosystem exchange (NEE), the result of combustion added to NEP, which can be either net CO2 storage (-) or net CO2 emissions (+), is represented by a dot on a black line. MMT CO2 Eq./yr, million metric tons of carbon dioxide equivalent per year.
Ten States accounted for over 70 percent of the carbon storage on lands in the Fed-CONUS (table 5). The largest amount was stored in California, followed by Montana, Oregon, Idaho, Colorado, Nevada, Wyoming, Utah, New Mexico, and Washington. California stored 12 percent (8,259 MMT CO2 Eq.) of the total carbon storage on lands in the Fed-CONUS and accounted for 12 percent of the Federal land area. Conversely, Nevada accounted for the largest proportion of Fed-CONUS land area (14 percent) but stored only 7 percent of the total carbon (4,720 MMT CO2 Eq.). Of the 20 States with the largest Federal land area, the largest carbon storage densities were in Florida (921 metric tons of carbon dioxide equivalent per hectare [MT CO2 Eq./ha]), Minnesota (806 MT CO2 Eq./ha), Virginia (756 MT CO2 Eq./ha), and Michigan (751 MT CO2 Eq./ha), owing primarily to the formation of deep, organically rich peat soils.
Table 4.
Carbon stocks and fluxes for Federal lands in the conterminous United States, 2005–21.[Units are in million metric tons of carbon dioxide equivalent (MMT CO2 Eq.) for stocks and million metric tons of carbon dioxide equivalent per year (MMT CO2 Eq./yr) for fluxes. Because of rounding, averages may not add to totals shown. Negative values indicate a net carbon sink or sequestration, and positive values indicate a net carbon source to the atmosphere or emissions. TEC, total ecosystem carbon; Live, carbon storage in live vegetation; Deadwood, carbon storage in dead organic matter; Litter, carbon storage in litter; Soil, carbon storage in soils; NPP, net primary productivity; Rh, heterotrophic respiration; NEP, net ecosystem productivity; NBP, net biome productivity; NEE, net ecosystem exchange; Harvest, carbon loss from forest harvest; Combustion, carbon loss from combustion including wildfire. Refer to table 3 and the text for further explanation of carbon stocks and fluxes]
California had the highest rate of NPP, averaging 171 MMT CO2 Eq./yr. Nevada, which has the largest land area in the Fed-CONUS, averaged 108 MMT CO2 Eq./yr in NPP. The difference in mean annual carbon uptake can be attributed primarily to the proportion of forest lands found in California compared with Nevada. Forests remove carbon from the atmosphere at a much higher rate than regions dominated by grasslands and shrublands. Of the States with more than 5 million hectares of Federal land area, Washington had the highest rate of carbon uptake, averaging 11 metric tons of carbon dioxide equivalent per hectare per year (MT CO2 Eq./ha/yr). The highest uptake rate overall for States with more than 1 million hectares of Federal lands was found in Florida, which averaged 37 MT CO2 Eq./ha/yr.
When ecosystem respiration, LULC, and emissions from disturbances were considered, Florida was the largest net sink (most negative NEE) of carbon on Federal lands at a rate of -2.9 MMT CO2 Eq./yr. Missouri sequestered (negative NEE) 2.8 MMT CO2 Eq./yr, Minnesota sequestered 2.5 MMT CO2 Eq./yr, Michigan sequestered 2.2 MMT CO2 Eq./yr, and Virginia sequestered 1.9 MMT CO2 Eq./yr.
California had the largest mean annual carbon emissions from wildfire and LULC change at 21.7 MMT CO2 Eq./yr, followed by Idaho and Oregon, which averaged 8.3 and 6.7 MMT CO2 Eq./yr, respectively. The largest forest harvest-related carbon losses were found in California and Oregon, which averaged 8.2 and 7.5 MMT CO2 Eq./yr, respectively.
Table 5.
Average annual carbon stocks and fluxes for Federal lands in the conterminous United States, 2005–21.[Units are in million metric tons of carbon dioxide equivalent (MMT CO2 Eq.) for stocks and million metric tons of carbon dioxide equivalent per year (MMT CO2 Eq./yr) for fluxes. Because of rounding, averages may not add to totals shown. Negative values indicate a net carbon sink or sequestration, and positive values indicate a net carbon source to the atmosphere or emissions. TEC, total ecosystem carbon; Live, carbon storage in live vegetation; Deadwood, carbon storage in dead organic matter; Litter, carbon storage in litter; Soil, carbon storage in soils; NPP, net primary productivity; NEP, net ecosystem productivity; NBP, net biome productivity; NEE, net ecosystem exchange; Rh, heterotrophic respiration; Harvest, carbon loss from forest harvest; Combustion, carbon loss from combustion including wildfire. Refer to table 3 and the text for further explanation of carbon stocks and fluxes]
Discussion
Collectively, lands in the Fed-CONUS sequestered carbon at a rate of -16.7 MMT CO2 Eq./yr. From 2005 to 2021, this resulted in the cumulative sequestration of 283.8 MMT CO2 Eq. An additional 888.1 MMT CO2 Eq. (52.4 MMT CO2 Eq./yr) was transferred from ecosystems to harvested wood products pools. For this report, we did not account for the decay and decomposition harvested wood products. Ecosystems were a net sink of carbon in 11 of the 17 years analyzed in this study. Of the six years where ecosystems were a net source of emissions to the atmosphere (NEE was positive), poor climate conditions (such as drought) resulting in reductions in NPP, and wildfire were the primary drivers. In four of the years included in this analysis (2007, 2012, 2020, 2021), NEP was estimated to be positive (a net source of emissions to the atmosphere) indicating poor growing conditions due to climate conditions. Those same years included four of the six largest fire emission years. From 2005 to 2019, fire resulted in the direct combustion of 55.7 MMT CO2 Eq./yr, whereas in 2020 and 2021, combustion was nearly three times the historical average at 165 MMT CO2 Eq./yr and 140 MMT CO2 Eq./yr, respectively.
This study reveals a declining trend over time in vegetation productivity (NPP) and an increasing trend in ecosystem heterotrophic respiration (Rh) resulting from climate variability and change. Combined, the effects of climate conditions have resulted in a decline in the sink strength of ecosystems on Federal lands. While harvest removals have remained relatively stable over time, combustion from severe fire has not. Since 2020, primarily due to fires, ecosystems on Federal lands have contributed to the net accumulation of greenhouse gases in the atmosphere.
Combined Net Emissions and Sequestration Results
Combining the fossil fuel extraction and combustion emissions with the ecosystems emissions and sequestration estimates provides an informative summary result that includes both anthropogenic emissions and sequestration by ecosystems on Federal lands. This results in the combined net emissions value, which is negative if carbon is stored and positive if carbon is emitted. It is the sum of the total fossil fuel CO2 emission value and the NEE, the net exchange of greenhouse gases between ecosystems and the atmosphere. A positive combined net emission result indicates that emissions are greater than sequestration, whereas a negative value indicates that sequestration is greater than emissions. Ecosystems data, calculated for the period 2005 to 2021, are provided in this report for comparison with fossil fuel-associated emissions estimates. In addition, sequestration data were not available for the Federal offshore areas, Alaska, or Hawaii, so net emissions values are not available for those areas. The combined net emissions results for 2005–21 are for the conterminous onshore United States Federal lands only and are provided in table 6.
Annual combined net emissions data for the United States show variations that reflect the variation in the ecosystems NEE data. These variations are described in the terrestrial ecosystem-associated carbon emissions and sequestration “Results” section. In general, fossil fuel-associated emissions data show increasing or decreasing long-term trends but not significant annual variation. Whereas most major fossil fuel-producing States have positive combined net emissions, some States with lesser production alternate between positive and negative combined net emissions because ecosystem NEE (sequestration) values may be larger than the State’s fossil fuel-associated emissions. The average fossil fuel-associated emissions for CO2 over the period 2005–21 was 1,201.9 MMT CO2 Eq., the average ecosystems NEE during that period was -16.7 MMT CO2 Eq.; this suggests that over this time ecosystems offset roughly 1.4 percent of fossil fuel activity CO2 emissions. In specific years, owing to NEE variability, the offset is as high as 31.5 percent, as seen in 2019 (a low fire year on Fed-CONUS), but can also be additive to the fossil fuel associated total emissions. In 2020, a year with high emissions on Federal lands from forest fires, ecosystems contributed CO2 emissions to the atmosphere equivalent to 40 percent of the Federal lands-sourced fossil fuel emissions for the year.
Table 6.
Combined net emissions for Federal lands in the United States, 2005–21.[All units are in million metric tons of carbon dioxide equivalent per year. Positive values indicate a net carbon emission, and negative values indicate a net carbon sequestration. Annual net ecosystem exchange was not available for Alaska, Hawaii, and the offshore areas; therefore, totals are for the conterminous U.S. For net emissions results from 2006–2009 and 2011–2014, refer to the associated data release (Merrill and others, 2024). Note that the “State total” for each State is for all years combined from 2005 to 2021. –, values were not calculated owing to unavailability of annual terrestrial ecosystem-associated carbon emissions and sequestration]
Conclusions
The USGS has produced estimates of fossil fuel-associated greenhouse gas emissions (2005–22) and terrestrial ecosystem-associated carbon emissions and sequestration (2005–21) for the Federal lands of the United States. Emissions associated with fossil fuel extraction and end-use combustion parallel production levels. States with significant fossil fuel production from Federal lands generally have higher estimated greenhouse gas emissions. Some States are significant producers of fossil fuels from non-Federal lands (such as State and private lands), and have similar magnitude emissions associated with those activities, but those non-Federal lands emissions are not estimated here. Nationwide emissions from fuels extracted from Federal lands in 2022 were 1,081.2 MMT CO2 Eq. for CO2, 33.4 MMT CO2 Eq. for CH4, and 4.3 MMT CO2 Eq. for N2O. The 2022 Federal lands fossil fuel emissions represent decreases in emissions for all three greenhouse gases relative to 2005 values, with reductions of 17 percent for CO2, 38 percent for CH4, and 30 percent for N2O (table 2, fig. 5). While total U.S. emissions are also down over the same period (U.S. Environmental Protection Agency, 2024a), the Federal portion of those total emission has declined at a slightly greater rate. Emissions from fossil fuels produced on Federal lands represent, on average, 21.8 percent of total U.S. emissions for CO2, 6.1 percent for CH4, and 1.3 percent for N2O over the 18 years included in this estimate.
Estimates of ecosystem carbon sequestration on Federal surface lands show that the amounts are highly variable owing to climate and weather, wildfire, land use and land use changes, and other factors. States with the largest forests or Federal land holdings do not necessarily have the most significant ecosystem sequestration because soils retain more carbon than living matter does and ecosystems release carbon as well as store it. Of note, owing to LULC and disturbance such as wildfires, some years result in net emissions of CO2 to the atmosphere from Federal lands ecosystems, therefore not offsetting any fossil-fuel associated emissions. These factors highlight the complexity of the ecosystem sequestration calculation that is accounted for in the estimated results. The combined net emission values included in this report are an informative, though simplistic, way of combining the two estimates.
These results are not intended or appropriate for ranking or comparing the States for many reasons. Firstly, the proportion of Federal mineral and surface lands to total lands varies considerably across each State. Secondly, these estimates report fossil fuel-associated emissions by the State of origin rather than the State where the emissions occurred. The data required to track all fossil fuels to their location of eventual end-use combustion are not available because of the structure of the United States’ energy and industrial infrastructure. Thirdly, this report addresses the Federal lands-based scope that was given to the USGS; it was not designed to produce results for comparing States.
These USGS estimates provide an accounting for the emissions associated with fossil fuel extraction on Federal lands, the end-use combustion of those fuels, and the ecosystems sequestration and emissions of carbon on Federal lands. Emissions estimates presented here for 2005–22, were produced with updated methodologies and input data, and therefore replace estimates for 2005–14 made in a previous report (Merrill and others, 2018). This information may provide context for future energy decisions, as well as serve as a reference to compare future changes in greenhouse gas emissions and carbon sequestration on Federal lands.
References Cited
Bureau of Land Management, 2024, 2023 BLM specialist report on annual greenhouse gas emissions and climate trends from coal, oil, and gas exploration and development on the Federal mineral estate: Bureau of Land Management report, unpaginated, accessed June 28, 2024, at https://www.blm.gov/content/ghg/.
Bureau of Ocean Energy Management, 2011, BOEM Pacific active oil and gas platforms: Bureau of Ocean Energy Management vector digital data, accessed March 21, 2017, at https://www.boem.gov/PC-plat.zip.
Bureau of Safety and Environmental Enforcement, 2014, Outer Continental Shelf oil and natural gas platforms—Gulf of Mexico region NAD 27: Bureau of Safety and Environmental Enforcement vector digital data, accessed March 21, 2017, at https://www.data.boem.gov/Mapping/Files/platform.zip.
Daniel, C.J., Frid, L., Sleeter, B.M., and Fortin, M.J., 2016, State‐and‐transition simulation models—A framework for forecasting landscape change: Methods in Ecology and Evolution, v. 7, no. 11, p.1413–1423, accessed September 30, 2024, at https://doi.org/10.1111/2041-210X.12597.
Daniel, C.J., Sleeter, B.M., Frid, L., and Fortin, M.J., 2018, Integrating continuous stocks and flows into state‐and‐transition simulation models of landscape change: Methods in Ecology and Evolution, v. 9, no. 4, p. 1133–1143, September 30, 2024, at https://doi.org/10.1111/2041-210X.12952.
Del Grosso, S., Parton, W., Stohlgren, T., Zheng, D., Bachelet, D., Prince, S., Hibbard, K., and Olson, R., 2008, Global potential net primary production predicted from vegetation class, precipitation, and temperature: Ecology. v. 89, no. 8, p. 2117–2126, accessed September 30, 2024, at https://doi.org/10.1890/07-0850.1.
Eidenshink, J., Schwind, B., Brewer, K., Zhu, Z.-L., Quayle, B., and Howard, S., 2007, A project for monitoring trends in burn severity: Fire Ecology, v. 3, no. 1, p. 3–21. [Also available at https://doi.org/10.4996/fireecology.0301003.]
Franklin, P., Scheehle, E., Collings, R.C., Coté, M.M., and Pilcher, R.C., 2004, Proposed methodology for estimating emission inventories from abandoned coal mines—Prepared for the 2006 IPCC [Intergovernmental Panel on Climate Change] National Greenhouse Gas Inventories Guidelines, Fourth Authors/Experts Meeting, Energy—Methane Emissions for Coal Mining and Handling, Arusha, Tanzania, [Sept. 28–30, 2004]: U.S. Environmental Protection Agency white paper, variously paged, accessed May 2, 2017, at https://www.epa.gov/sites/production/files/2016-03/documents/methodology_abandoned_coalmines.pdf.
Intergovernmental Panel on Climate Change, 2000, Quantifying uncertainties in practice, Hairaishi, T., Nyenzi, B., Odingo, R., Penman, J., and Habetsion, S., eds., chap. 6 of IPCC good practice guidance and uncertainty management in national greenhouse gas inventories: Intergovernmental Panel on Climate Change (IPCC) Plenary, 16th session, Montreal, Canada, May 1–8, 2000, 34 p., accessed July 25, 2024, at https://www.ipcc-nggip.iges.or.jp/public/gp/english/.
Intergovernmental Panel on Climate Change, 2013, Climate change 2013—The physical science basis—Contribution of Working Group I to the fifth assessment report of the Intergovernmental Panel on Climate Change [Stocker, T.F., Qin, D., Plattner, G.-K., Tignor, M., Allen, S.K., Boschung, J., Nauels, A., Xia, Y., Bex, V., and Midgleyet, P.M., eds.]: Cambridge, United Kingdom, and New York, Cambridge University Press, 1,585 p. [Also available at http://www.ipcc.ch/report/ar5/wg1.]
Intergovernmental Panel on Climate Change, 2021, Climate change 2021—The physical science basis—Contribution of Working Group I to the sixth assessment report of the Intergovernmental Panel on Climate Change [Masson-Delmotte, V., Zhai, P., Pirani, A., Connors, S.L., Péan, C., Berger, S., Caud, N., Chen, Y., Goldfarb, L., Gomis, M.I., Huang, M., Leitzell, K., Lonnoy, E., Matthews, J.B.R., Maycock, T.K., Waterfield, T., Yelekçi, O., Yu, R., and Zhou, B., eds.]: Cambridge, United Kingdom, and New York, Cambridge University Press, 2,391 p., accessed April 10, 2024, at https://doi.org/10.1017/9781009157896.
Karacan, C.Ö., and Warwick, P.D., 2019, Assessment of coal mine methane (CMM) and abandoned mine methane (AMM) resource potential of longwall mine panels—Example from northern Appalachian Basin, USA: International Journal of Coal Geology, v. 208, p. 37–53, accessed June 25, 2024, at https://doi.org/10.1016/j.coal.2019.04.005.
LANDFIRE, 2023, LANDFIRE Annual Disturbance layer (June 2023, last update): U.S. Geological Survey and U.S. Department of Agriculture web page, accessed July 18, 2023, at https://landfire.gov/disturbance/annualdisturbance.
Marvin, D.C., Sleeter, B.M., Cameron, D.R., Nelson, E., and Plantinga, A.J., 2023, Natural climate solutions provide robust carbon mitigation capacity under future climate change scenarios: Scientific Reports, v. 13, no. 1, 12 p., accessed September 30, 2024, at https://doi.org/10.1038/s41598-023-43118-6.
Merrill, M.D., Sleeter, B.M., and Freeman, P.A., 2024, Federal lands greenhouse gas emissions and sequestration in the United States—Estimates for 2005–22 - data: U.S. Geological Survey data release, https://doi.org/10.5066/P14CVD9R.
Merrill, M.D., Sleeter, B.M., Freeman, P.A., Liu, J., Warwick, P.D., and Reed, B.C., 2018, Federal lands greenhouse emissions and sequestration in the United States—Estimates for 2005–14: U.S. Geological Survey Scientific Investigations Report 2018–5131, 31 p., accessed May 20, 2019, at https://doi.org/10.3133/sir20185131.
Minerals Management Service, 2006a, Digital Offshore Cadastre (DOC)—Gulf of Mexico Federal Outer Continental Shelf (OCS) administrative boundaries (preliminary): Minerals Management Service vector digital data, accessed August 14, 2018, at https://www.boem.gov/Administrative-Boundaries/.
Minerals Management Service, 2006b, Digital Offshore Cadastre (DOC)—Pacific Federal Outer Continental Shelf (OCS) administrative boundaries (preliminary): Minerals Management Service vector digital data, accessed August 14, 2018, at https://www.boem.gov/Administrative-Boundaries/.
National Oceanic and Atmospheric Administration, 2024, NOAA Coastal Change Analysis Program (C-CAP) regional 30 meter land cover [year 2001 data file]: National Oceanic and Atmospheric Administration Office for Coastal Management web page, accessed January 2024, at www.coast.noaa.gov/htdata/raster1/landcover/bulkdownload/30m_lc/.
Office of Natural Resources Revenue, 2024, Natural resources revenue data—Production disposition by month: Office of Natural Resources Revenue web page, accessed April 24, 2024, at https://revenuedata.doi.gov/downloads/production-by-disposition/.
PRISM Climate Group, 2023, PRISM climate data: PRISM Climate Group, Oregon State University web page, accessed June 15, 2023, at https://prism.oregonstate.edu.
Rigge, M.B., Bunde, B., Postma, K., and Shi, H., 2024, Rangeland condition monitoring assessment and projection (RCMAP) fractional component time-series across the western U.S. 1985–2023: U.S. Geological Survey data release, accessed September 30, 2024, at https://doi.org/10.5066/P9SJXUI1.
Rollins, M.G., 2008, LANDFIRE—A nationally consistent vegetation, wildland fire, and fuel assessment: International Journal of Wildland Fire, v. 18, no. 3, p. 235–249, accessed October 1, 2010, at https://doi.org/10.1071/WF08088.
Selmants, P.C., Sleeter, B.M., Liu, J., Wilson, T.S., Trauernicht, C., Frazier, A.G., and Asner, G.P., 2021, Ecosystem carbon balance in the Hawaiian Islands under different scenarios of future climate and land use change: Environmental Research Letters, v. 16, no. 10, 13 p., accessed September 30, 2024, at https://doi.org/10.1088/1748-9326/ac2347.
Sleeter, B.M., Frid, L., Rayfield, B., Daniel, C., Zhu, Z., and Marvin, D.C., 2022, Operational assessment tool for forest carbon dynamics for the United States—A new spatially explicit approach linking the LUCAS and CBM-CFS3 models: Carbon Balance and Management, v. 17, no. 1, 26 p., accessed September 30, 2024, at https://doi.org/10.1186/s13021-022-00201-1.
Sleeter, B.M., Liu, J., Daniel, C.J., Hawbaker, T.J., Wilson, T.S., Fortini, L.B., Jacobi, J.D., Selmants, P.C., Giardina, C.P., Litton, C.M., and Hughes, R.F., 2017, Projected future carbon storage and carbon fluxes in terrestrial ecosystems of Hawai‘i from changes in climate, land use, and disturbance, chap. 8 in Selmants, P.C., Giardina, C.P., Jacobi, J.D., and Zhu, Z., eds., Baseline and projected future carbon storage and carbon fluxes in ecosystems of Hawai‘i: U.S. Geological Survey Professional Paper 1834, p. 107–128, accessed September 30, 2024, at https://doi.org/10.3133/pp1834.
Sleeter, B.M., Liu, J., Daniel, C., Rayfield, B., Sherba, J., Hawbaker T.J., Zhu, Z., Selmants, P.C., and Loveland, T.R., 2018, Effects of contemporary land-use and land-cover change on the carbon balance of terrestrial ecosystems in the United States: Environmental Research Letters, v. 13, no. 4, 13 p., accessed September 30, 2024, at https://doi.org/10.1088/1748-9326/aab540.
Sleeter, B.M., Marvin, D.C., Cameron, D.R., Selmants, P.C., Westerling, A.L., Kreitler, J., Daniel, C.J., Liu, J., and Wilson, T.S., 2019, Effects of 21st-century climate, land use, and disturbances on ecosystem carbon balance in California: Global Change Biology, v. 25, no. 10, p. 3334–3353, accessed September 30, 2024, at https://doi.org/10.1111/gcb.14677.
U.S. Energy Information Administration, 2024a, Energy conversion calculators: U.S. Energy Information Administration web page, accessed August 6, 2024, at https://www.eia.gov/energyexplained/units-and-calculators/energy-conversion-calculators.php.
U.S. Energy Information Administration, 2024b, Energy use in homes: Information Administration web page, accessed August 6, 2024, at https://www.eia.gov/energyexplained/use-of-energy/homes.php.
U.S. Environmental Protection Agency, 2004, Methane emissions from abandoned coal mines in the United States—Emissions inventory methodology and 1990–2002 emissions estimates: U.S. Environmental Protection Agency [report] EPA 430–R–04–001, 54 p. [Also available at https://nepis.epa.gov/Exe/ZyPDF.cgi/600004JM.PDF?Dockey=600004JM.PDF.]
U.S. Environmental Protection Agency, 2024a, Inventory of U.S. greenhouse gas emissions and sinks—1990–2022: U.S. Environmental Protection Agency [report] EPA 430–R–24–004, variously paged, plus additional online data tables, accessed April 5, 2024, at https://www.epa.gov/ghgemissions/inventory-us-greenhouse-gas-emissions-and-sinks-1990-2022.
U.S. Environmental Protection Agency, 2024b, Annexes to the inventory of U.S. GHG emissions and sinks [—to accompany U.S. Environmental Protection Agency, Inventory of U.S. greenhouse gas emissions and sinks—1990–2022, [report] EPA 430–R–24–004]: U.S. Environmental Protection Agency, 595 p., accessed April 5, 2024, at https://www.epa.gov/ghgemissions/inventory-us-greenhouse-gas-emissions-and-sinks-1990-2022.
U.S. Environmental Protection Agency, 2024c, Annex 3.5—Methodology for estimating CH4, CO2, and N2O emissions from petroleum systems, in Natural gas and petroleum systems in the GHG inventory—Additional information on the 1990–2022 GHG inventory (published April 2024) [—to accompany U.S. Environmental Protection Agency, Inventory of U.S. greenhouse gas emissions and sinks—1990–2022, [report] EPA 430–R–24–004]: U.S. Environmental Protection Agency, 23 tables, accessed April 5, 2024, at https://www.epa.gov/ghgemissions/natural-gas-and-petroleum-systems-ghg-inventory-additional-information-1990-2022-ghg.
U.S. Environmental Protection Agency, 2024d, Annex 3.6—Methodology for estimating CH4, CO2, and N2O emissions from natural gas systems, in Natural gas and petroleum systems in the GHG inventory—Additional information on the 1990–2022 GHG inventory (published April 2024) [—to accompany U.S. Environmental Protection Agency, Inventory of U.S. greenhouse gas emissions and sinks—1990–2022, [report] EPA 430–R–24–004]: U.S. Environmental Protection Agency, 11 tables, accessed April 5, 2024, at https://www.epa.gov/ghgemissions/natural-gas-and-petroleum-systems-ghg-inventory-additional-information-1990-2022-ghg.
U.S. Environmental Protection Agency, 2024e, Facility level information on greenhouse gases tool (FLIGHT)—Greenhouse gas emissions from large facilities: U.S. Environmental Protection Agency web page, accessed April 17, 2024, at https://ghgdata.epa.gov/ghgp/main.do.
U.S. Geological Survey, 2016, Protected areas—Protected areas database of the United States (PAD-US) (ver. 1.4): U.S. Geological Survey Gap Analysis Project database, accessed September 16, 2017, at http://gapanalysis.usgs.gov/padus/.
Williams, C.A., Hasler, N., Gu, H., and Zhou, Y., 2020, Forest carbon stocks and fluxes from the NFCMS, conterminous USA, 1990–2010 [dataset] (ver. 2): Oak Ridge National Laboratory, Distributed Active Archive Center web page, accessed September 30, 2024, at https://doi.org/10.3334/ORNLDAAC/1829.
Glossary
[Also refer to table 3, “Explanation of carbon stock and flux terms from the terrestrial ecosystem sequestration calculations”]
abandoned coal mine emissions
Emissions of methane, such as from offgassing or desorption, occurring after the cessation of extraction activities at a surface or underground coal mine.
active coal mine emissions
Emissions of methane from ongoing extraction activities at a surface or underground coal mine.
combustion
The process of burning fossil fuels to release energy.
emission factor
A value that describes the greenhouse gas content of a fuel used in a particular sector of the economy or that indicates the amount of greenhouse gas emitted by a particular extraction activity.
estimated emissions
Calculated estimates of greenhouse gases released by activities in various sectors of the economy.
Federal lands
Context dependent catch-all term for various classes of land administered by various agencies of the U.S. Federal Government.
Federal mineral rights land
Subsurface lands with mineral (and fossil fuel) rights administered by various agencies of the U.S. Federal Government.
Federal surface lands
Surface land administered by various agencies of the U.S. Federal Government, may or may not have same footprint as underlying Federal mineral lands.
fossil fuel
A natural hydrocarbon material that is extracted and then combusted to release energy. Examples include coal, natural gas, crude oil, and various liquid petroleum products.
greenhouse gas
Gases that cause the amount of solar thermal energy retained by the Earth’s atmosphere to increase when their concentrations increase. The greenhouse gases discussed in this report are carbon dioxide (CO2), methane (CH4), and nitrous oxide (N2O).
method of abandonment
The process by which an underground coal mine is closed. Examples include sealing, venting, and flooding. The abandonment process determines the emission factor used to calculate the amount of methane gas released from the abandoned mine over time.
mobile combustion emissions
Emissions of greenhouse gases from fossil fuel combustion in the transportation sector.
offshore
Areas seaward of State waters boundaries that are under the administration of the Bureau of Ocean Energy Management. Examples include the offshore Pacific and offshore Gulf of Mexico
petroleum and natural gas systems emissions
Emissions of greenhouse gases from ongoing extraction activities and product transportation in the petroleum and natural gas industries.
stationary combustion emissions
Emissions of greenhouse gases produced during the combustion of fossil fuels in all nontransportation sectors, including electricity generation, industrial feedstocks, and residential and commercial heating.
Appendix 1. Detailed Methods: Fossil Fuel-Associated Emissions of Greenhouse Gases from Federal Lands
Introduction
The methods described in this appendix are either similar to those described in, or are dependent on, outputs from the U.S. Environmental Protection Agency (EPA) Inventory of U.S. Greenhouse Gas Emissions and Sinks (hereinafter U.S. GHG Inventory; U.S. Environmental Protection Agency, 2024a). The methods were modified where necessary in terms of complexity, scope, and outputs to suit the goals of this U.S. Geological Survey (USGS) study. Therefore, the U.S. GHG Inventory and associated annexes (U.S. Environmental Protection Agency, 2024a, b) are the best detailed sources for the general methods described here. This section highlights general steps, sources for inputs, and areas where the USGS method differs from that of the U.S. GHG Inventory. Tables 1.1 through 1.7 include summary information on the sources for all input data used.
Input Data
Oil, gas, coal, and natural carbon dioxide production data were downloaded or provided by the Office of Natural Resources Revenue (ONRR). Oil and gas data, along with State-level coal data are available for public download from the ONRR Natural Resources Revenue Data (NRRD) website (Office of Natural Resources Revenue, 2024). For oil and gas production data, ONRR “production disposition by month” datasets, were filtered using specific disposition codes (table 1.1) to limit the volumes included in the estimate to those that were eventually combusted as a fuel. The codes not included were for volumes that were reinjected, or disposed of, and volumes accounted for elsewhere using emissions factors, such as flaring and venting. Including the disposition codes with flared and vented volumes would double count those volumes in the current method but could lead to a more accurate estimate in a modified future methodology. The disposition codes included from the NRRD are presented in table 1.1. A full description of the codes is available from the Office of Natural Resources Revenue (2023).
Table 1.1.
Office of Natural Resources Revenue disposition codes included in this report.[Codes are from the Office of Natural Resources Revenue (2023)]
Stationary Combustion Emissions
Stationary combustion emissions estimates are based on coal, oil, and natural gas production data. Mine-level coal production data were obtained from the ONRR via direct request. This propriety data is not publicly available, but State-level data is available from ONRR’s Natural Resources Revenue Data (NRRD) system (Office of Natural Resources Revenue, 2024). The end use of Federal lease specific coal is not available; therefore State-level data is the closest approximation of sector usage. Federal lease coal production was apportioned to coal use sectors (electricity generation, commercial and residential, industrial, and coking coal production) based on State-level sector coal end-use proportions in compiled data from the 2005–2022 editions of the U.S. Energy Information Administration (EIA) Annual Coal Distribution Report (U.S. Energy Information Administration, 2022). For example, in 2022, 76 percent of Colorado State coal was reported to be used in electricity generation, and 98 percent in Wyoming (U.S. Energy Information Administration, 2022); therefore, 76 and 98 percent respectively of the Federal lease coal from those States would have an electrical power generation emissions factor applied to them.
In the final step, the sector coal amounts were multiplied by carbon dioxide (CO2), methane (CH4), and nitrous oxide (N2O) emission factors to estimate the amounts of gases emitted. The emission factors used are specific to each sector and were reported by the EPA (U.S. Environmental Protection Agency, 2024b) and references therein. One change made to the stationary coal usage methodology was the incorporation of mine-specific coal rank for energy content, versus assumed rank blends based on economic sector.
The method used to estimate emissions for natural gas follows the same general method described above for coal. Publicly available ONRR production data from the NRRD were the starting point. Data covering natural gas consumption by sector are from the U.S. Energy Information Administration (2024a). Sector proportions specific to noncombustion industrial natural-gas feedstock use ranged from 1.6 to 2.9 percent and are from the EIA Manufacturing Energy Consumption Survey (U.S. Energy Information Administration, 2021), which is released every 4–5 years. Data for years between surveys were estimated using a linear-fit trendline with an r2 correlation of 0.88. Emission factors for the stationary combustion of natural gas were from the EPA (U.S. Environmental Protection Agency, 2023). The amount of carbon stored in products made using industrial nonfuel natural gas was accounted for using a storage factor (U.S. Environmental Protection Agency, 2024b, table A–41).
Estimation of emissions from the stationary combustion of various petroleum products started with State-level crude oil production data from the ONRR (Office of Natural Resources Revenue, 2024). The crude oil volumes were assigned to State production volumes of refined products using compiled annual national-level refining data (U.S. Energy Information Administration, 2023a, Petroleum Supply Annual table 23). State of origin-based crude oil refining information is not available for liquid fuels owing to the comingled nature of liquid fuel transportation in pipelines. The resulting volumes were apportioned to end-use sectors by using sector proportion data obtained from the EIA Monthly Energy Review (U.S. Energy Information Administration, 2023b, Monthly Energy Review tables 3.5 and 3.7). Lastly, the estimated sector-specific petroleum product volumes were multiplied by the CO2, CH4, and N2O emission factors for each refined product from the EPA (U.S. Environmental Protection Agency, 2023). Refer to table 1.2 for the inputs and sources mentioned in this section.
Table 1.2.
Inputs and sources for the stationary combustion greenhouse gas emissions estimate.Input | Source |
---|---|
Coal production volumes from Federal lands | Office of Natural Resources Revenue, direct request |
Crude oil, and natural gas production volumes from Federal lands | Office of Natural Resources Revenue (2024), Natural Resources Revenue data |
Coal: sector use proportions | U.S. Energy Information Administration (2022), Annual Coal Distribution Report |
Coal: sector-specific emission factors | U.S. Environmental Protection Agency (2024a, b) |
Natural gas: sector use proportions | U.S. Energy Information Administration (2024a), Monthly Energy Review table 4.3 |
Natural gas: emission factors | U.S. Environmental Protection Agency (2023) |
Natural gas: nonenergy storage factor | U.S. Environmental Protection Agency (2024b), table A–41 |
Petroleum products: refining data | U.S. Energy Information Administration (2023a), Petroleum Supply Annual table 23 |
Petroleum products: sector use proportions | U.S. Energy Information Administration (2023b), Monthly Energy Review tables 3.5 and 3.7 |
Petroleum products: sector-specific emission factors | U.S. Environmental Protection Agency (2023) |
Mobile Sector Combustion Emissions
Estimates of emissions associated with combustion from mobile (transportation) uses were generated from ratios of emissions to fuel volumes combusted as reported by the U.S. GHG Inventory (U.S. Environmental Protection Agency, 2024a). Crude oil production volumes from the Federal lands in each State were obtained from the ONRR NRRD. The crude oil data were partitioned into estimated petroleum products (for example, gasoline, diesel oil, jet fuel, lubricants) on the basis of national refining products data (U.S. Energy Information Administration, 2023a, Petroleum Supply Annual table 23). Sector use information for each fuel was used to determine the proportions that were consumed in mobile uses (U.S. Energy Information Administration, 2023b).
The ratios of the U.S. annual mobile emissions of CO2, CH4, and N2O (U.S. Environmental Protection Agency, 2024a) to the annual mobile fuel consumption (U.S. Environmental Protection Agency, 2024b) provided values of emissions per gallon of fuel consumed (by gas and type of fuel). These values were multiplied by the volumes of combusted fuels sourced from Federal lands to estimate the emissions attributed to each fuel. This method reasonably assumes that the national emissions ratios are representative of the emissions ratios expected for fuels from Federal lands. No major changes were made to the methodology in this section compared to the previous report. Refer to table 1.3 for the inputs and sources mentioned in this section.
Table 1.3.
Inputs and sources for the mobile sector combustion greenhouse gas emissions estimate.Input | Source |
---|---|
Crude oil production volumes from Federal lands | Office of Natural Resources Revenue (2024), Natural Resources Revenue data |
Petroleum products: refining data | U.S. Energy Information Administration (2023a), Petroleum Supply Annual table 23 |
Petroleum products: sector use proportions | U.S. Energy Information Administration (2023b), Monthly Energy Review tables 3.5 and 3.7 |
Annual mobile fuel consumption | U.S. Environmental Protection Agency (2024b), table A–68 |
Annual carbon dioxide mobile emissions | U.S. Environmental Protection Agency (2024a), table 3–13 |
Annual methane mobile emissions | U.S. Environmental Protection Agency (2024a), table 3–14 |
Annual nitrous oxide mobile emissions | U.S. Environmental Protection Agency (2024a), table 3–15 |
Petroleum and Natural Gas Systems Emissions
The emissions in this section are calculated for known Federal lease active wells, plugged wells, offshore platforms, and their associated infrastructure. For active wells, total national emissions from petroleum and natural gas systems, excluding offshore platform emissions (U.S. Environmental Protection Agency, 2024a) were divided by the national active oil and natural gas well counts (U.S. Environmental Protection Agency, 2024c, d), resulting in ratios of CO2, CH4, and N2O emissions per well for petroleum and natural gas systems. These national ratios were then multiplied by the numbers of Federal producing oil and gas wells in a State to estimate emissions. For the years included in this report (2005–22), State-level Federal lands well counts were available for 2014, 2016, 2019, 2020, and 2022 (Bureau of Land Management, written communication, 2014, 2016, 2019, 2020, 2022). To generate Federal lands well counts for years prior to 2014, the 2014 State-level Federal lands wells were divided by the 2014 total U.S. State well counts (U.S. Energy Information Administration, 2024b), to produce a State-specific Federal lands to total well ratio. This ratio was then used to approximate Federal lands well counts for each State from available annual State well totals in 2005–13). In some cases, this method resulted in active well count estimates of zero in years where some minimal production was reported, therefore a well count of zero was impossible. In these cases, the active well count was manually adjusted to one well. For the years 2015, 2017, 2018, and 2021 (years without data requested from Bureau of Land Management [BLM], due to system upgrades and USGS or BLM staff unavailability), the missing well counts were calculated as the average of the prior and following years with data.
State-level counts for documented plugged and abandoned (P&A) Federal lease oil and gas wells, a new addition to this emissions estimate, were provided by BLM by request for the year 2021. These total P&A well counts are not available retroactively, however, annual changes to P&A status for wells are available. The 2021 year served as a base year from which changes were made based on annual new P&A well status data from 2005 to 2022, also received from BLM by request. To make up for the unavailable P&A State-level well counts, the complete input dataset was built starting with the 2021 count and subtracting the new 2021 P&A wells to get a new 2020 total. This was repeated back to 2005, and forward to 2022. This sometimes would yield subzero values in the earlier years of the time series. In these cases, the count of documented P&A wells was set to zero. Unplugged idle, shut in, and orphaned well emissions estimates are not included in this report owing to well count unavailability, but this is targeted as a future addition to the estimate.
Total offshore oil-and-gas platform counts and emissions factors were obtained from the EPA (U.S. Environmental Protection Agency, 2024c, d). Production data for Federal platforms, though also available from EPA, is sourced from ONRR to be consistent with all other production data in this report. Following the EPA methodology, offshore Gulf of Mexico Outer Continental Shelf (OCS) vent/leak emissions are based on the size of the platform, and flaring emissions are based on the reported total fuel content flared. In the offshore Pacific, OCS oil emissions from vent/leak and flaring are based on the total barrels of oil produced. Pacific OCS gas production emissions factors are not provided by U.S. Environmental Protection Agency (2024d); therefore, Alaska State waters emissions factors have been used as an analog emissions factor.
Table 1.4.
Inputs and sources for the petroleum and natural gas systems greenhouse gas emissions estimate.[CO2, carbon dioxide; CH4, methane; N2O, nitrous oxide]
Input | Source |
---|---|
CO2, CH4, and N2O natural gas systems emissions | U.S. Environmental Protection Agency (2024a), tables 3–66, 3–75, and 3–77 |
CO2, CH4, and N2O petroleum systems emissions | U.S. Environmental Protection Agency (2024a), tables 3–46, 3–44, and 3–48 |
National producing oil well counts | U.S. Environmental Protection Agency (2024c), table 3.5–5 |
U.S. producing gas well counts | U.S. Environmental Protection Agency (2024d), table 3.6–7 |
State producing oil well counts | U.S. Energy Information Administration (2024b), appendix C |
State producing gas well counts | U.S. Energy Information Administration (2024b), appendix C |
Federal lands producing oil and gas well counts | Bureau of Land Management (written communication, 2014, 2016, 2019, 2020, 2022). |
Federal plugged and abandoned well count | Bureau of Land Management (written communication, 2014, 2016, 2018, 2019, 2020, 2022) |
Offshore platform counts and emission factors | U.S. Environmental Protection Agency (2024c, d), tables 3.5–3, 3.5–5, 3.5–8, 3.5–11, 3.6–2, 3.6–7, 3.6–12, and 3.6–15 |
Natural Carbon Dioxide Production Emissions
Natural CO2 end uses include CO2-enhanced oil recovery (CO2-EOR), food and beverage production, chemical production, greenhouse operations, water treatment, research, and other purposes. All uses except for CO2-EOR result in the eventual release of the CO2 to the atmosphere and are therefore sources of emissions. Based on this assumption, the method to determine emitted GHG from the total produced is simply to remove the amount used for CO2-EOR. Fugitive CO2 emissions (leaking from infrastructure) are accounted for in the fugitive emissions section and are therefore not also included here as it would be double counted.
Annual CO2 production volumes were provided by the Office of Natural Resources Revenues (written communication, 2024). Data for the end use of CO2 from large production facilities from 2010–22 was available in the EPA Facility Level Information on GreenHouse gases Tool (FLIGHT) (U.S. Environmental Protection Agency, 2024e). Because data availability begins in 2010, the calculation inputs for 2005–09 in this estimate are replaced with the average of EPA facility data from 2010–12. Annual national and State CO2-EOR usage ratios were produced with data from the States of Colorado, Mississippi, New Mexico, and West Virginia. Texas, Utah, and Wyoming, States with Federal lease CO2 production (but no facility data), use national CO2-EOR usage averages in this calculation.
Table 1.5.
Inputs and sources for the natural CO2 greenhouse gas emissions estimate.[CO2, carbon dioxide]
Input | Source |
---|---|
Natural CO2 production from Federal leases | Office of Natural Resources Revenues (written communication, 2024) |
Facility level CO2 end usage | U.S. Environmental Protection Agency (2024e), Facility Level Information on GreenHouse gases Tool (FLIGHT), Subpart pp, Greenhouse Gas Reporting Program data |
Active Coal Mine Emissions
Fugitive emissions of CH4 from active underground coal mining were calculated as the sum of four kinds of emissions: ventilation, degasification, recovered/used (a negative value), and postmining. Emissions of CH4 from ventilation are measured directly by the Mine Safety and Health Administration (MSHA) or reported by mine operators to MSHA, then compiled by EPA and eventually provided to the USGS. Degasification, as well as amounts of CH4 recovered is reported directly to the EPA. Reports of emissions are whole mine values, therefore they are apportioned in each year by the percentage of total mine production associated with Federal leases (a ratio based on proprietary mine-level ONRR production data); this is a change from overestimates in the previous report (Merrill and others, 2018) where whole mine data was used regardless of the Federal production portion. Some active mines are not included in the EPA data provided to the USGS and some mines have noncontinuous or mostly missing data. These data are based on direct measurements and are preferred over emissions factor-based estimates, though the methodology may be modified in future estimates to use emissions factors when data are missing or atypical. For underground mines, postmining CH4 emissions are the product of (1) annual production, (2) the basin-specific in situ coal CH4 content, and (3) a fixed postmining emission factor of 0.325 (U.S. Environmental Protection Agency, 2024b).
Active surface mine emissions of CH4 are the product of (1) annual production, (2) basin-specific in situ coal CH4 content, and (3) a fixed surface mining emission factor of 1.5 (U.S. Environmental Protection Agency, 2024b). The method for estimating postmining emissions for active surface mines is the same as that described above for post-mining emissions for active underground mines. Refer to table 1.6 for the inputs and sources mentioned in this section.
Table 1.6.
Inputs and sources for the active coal mining greenhouse gas emissions estimate.[CH4, methane; MSHA, Mine Safety and Health Administration]
Input | Source |
---|---|
Production, fuel type, and basin information | Office of Natural Resources Revenue (written communication, 2024) |
Basin-specific in situ coal CH4 content | U.S. Environmental Protection Agency (2024b), table A–98 |
Annual ventilation CH4 volumes | U.S. Environmental Protection Agency (written communication, 2024), Inventory of U.S. Greenhouse Gas Emissions and Sinks project team (data reported by MSHA) |
Annual degasification CH4 volumes | U.S. Environmental Protection Agency (written communication, 2024), Inventory of U.S. Greenhouse Gas Emissions and Sinks project team (data reported by MSHA) |
Annual recovered/used CH4 volumes | U.S. Environmental Protection Agency (written communication, 2024), Inventory of U.S. Greenhouse Gas Emissions and Sinks project team (data reported by MSHA) |
Postmining emission factors | U.S. Environmental Protection Agency (2024b), table A–98 |
Abandoned Coal Mine Emissions
The CH4 emissions from abandoned underground coal mines were calculated with emissions decline curves that used the active emissions on the day of abandonment as the initial point of decline in time. The variables that control the type of decline curve are specific to the method of abandonment (sealing, venting, or flooding). Emissions from mines with an unknown abandonment method were determined by calculating the emissions for all three methods of abandonment and then using a weighted average of the outputs based on region-specific abandonment-method statistics. The EPA’s methodology (Franklin and others, 2004; U.S. Environmental Protection Agency, 2004) for abandoned mines was the general method applied. However, unlike the EPA’s probabilistic approach, this USGS estimate is deterministic and was obtained by using region-specific median inputs in the decline curve calculations rather than a range of potential values. This simplification does not significantly affect the results and is in line with the simplified approach used in the USGS estimate. Refer to table 1.7 for the inputs and sources mentioned in this section.
Table 1.7.
Inputs and sources for the abandoned coal mine greenhouse gas emissions estimate.[MSHA, Mine Safety and Health Administration]
Input | Source |
---|---|
Abandoned Federal lease mines | U.S. Environmental Protection Agency (written communication, 2024), Inventory of U.S. Greenhouse Gas Emissions and Sinks project team (some data reported by MSHA) |
Date of abandonment | U.S. Environmental Protection Agency (written communication, 2024), Inventory of U.S. Greenhouse Gas Emissions and Sinks project team (some data reported by MSHA) |
Average emissions at abandonment | U.S. Environmental Protection Agency (written communication, 2024), Inventory of U.S. Greenhouse Gas Emissions and Sinks project team (some data reported by MSHA) |
Abandonment method | U.S. Environmental Protection Agency (written communication, 2024), Inventory of U.S. Greenhouse Gas Emissions and Sinks project team (some data reported by MSHA) |
Decline curve values | Franklin and others (2004); U.S. Environmental Protection Agency (2004) |
Abandonment method by basin | Franklin and others (2004); U.S. Environmental Protection Agency (2004) |
Exported Fuels Emissions
A line-item emissions estimate for exported fuels provided in the previous report (Merrill and others, 2018) is no longer included in the current study, but those fuels and their emissions are still accounted for in other sections. Estimating the emissions within a geographic area is not the goal of this emissions estimate. This estimate looks at the fuels originating within the Federal lands and then determines their end use emissions regardless of location. Therefore, the previous and current version of this estimate included exported fuels, but not imported fuels. This conceptual framework allows the estimates within this report to generally reflect the emissions associated with the energy production and management practices applied on U.S. Federal lands.
Oil or gas from specific Federal lease wells or even fields cannot be traced through the U.S. energy system. Coal from some mines may be traceable because of the method of transportation, but those data are not available to the USGS. A review of previous exported fuels emissions methods indicates that the assumptions required for the inclusion of export specific emissions estimates have too high an uncertainty for inclusion as a line-item output. Instead, the emissions associated with those exports are included within the other emissions totals.
Exported and imported fuel combustion and use is the main area where the USGS GHG emissions estimates depart from the U.S. GHG Inventory (U.S. Environmental Protection Agency, 2024a). The Intergovernmental Panel on Climate Change’s “2006 IPCC Guidelines for National Greenhouse Gas Inventories” (Intergovernmental Panel on Climate Change, 2006) specified that emissions are counted in the nation where they were emitted; therefore, the U.S. GHG Inventory includes combustion emissions from imported fuel, but not exported fuel. The USGS includes exported fuels, but not as a line item and excludes imported fuels.
Results Comparison to Previous 2005–14 Estimate
The emissions estimates in this report span from 2005 to 2022; this provides a 10-year overlap with the 2005 to 2014 time series of the previous report by Merrill and others (2018). When comparing the overlapping values from the two reports for total 2005–14 average emissions, CO2 emissions were 4.6 percent lower in this report; CH4 emissions were 4.6 percent higher in this report; and N2O emissions were 5.3 percent lower in this report. These are significant and inconsistent differences worth investigation. Differences in the emissions estimates from those common years are discussed below in terms of the impact of input data, methodological factors, and errors.
Input data, generally the production volumes of various extracted fuels are, as expected, the most important predictor of estimated emissions. In this report, the specific version of the input data (but not the source) has changed, as described in the “Data Sources” and “Input Data” sections. The change in total fuels used for the overlapping years of the time series is quite small. Owing to the requirement for proprietary coal mine specific data in this estimate, the publicly available coal production data was not used; however, at the State level, the public dataset was nearly identical to that used in this study. For oil and gas, the new dataset had 0.019 percent less total oil production and 0.14 percent less gas production over the same 2005 to 2014 time period relative to the original. Overall, these fuel production differences are negligible compared to the magnitude of the emissions estimate differences. Therefore, input data is not the source of any emissions estimate differences between the original (2005–2014) and current study for 2005 to 2022.
This leaves methodology and miscalculations as the remaining factors causing the reported differences. Also of note, is that while production totals show insignificant differences, the allotment of oil and gas between Federal offshore (Gulf of Mexico and Pacific) and the Federal leases of the coastal States have changed in the source data. Therefore, some State and offshore specific input volumes have changed, changes that are evident in State-level emissions totals.
Methodological changes between this report and the previous report by Merrill and others (2018) have resulted in most of the observed differences between the two time-series estimates. These changes include constant values such as emissions factors and conversion rates, but also the methods themselves, such as new processes or the inclusion of new emissions estimates. Starting with CO2, a process change using mine-specific coal rank in this report for energy content, versus assumed rank blends based on economic sector, has resulted in the most significant difference between the two time-series’ CO2 estimates. This is due to the large role of Wyoming coal production in the emissions estimates. Most Federal leases in Wyoming produce subbituminous coal; the previous method used assumed rank mixes based on end use with coal energy content (in million British thermal units per short ton of coal) that were an overestimate for the rank of Wyoming coal. This 13 percent decline combined with the 85 percent Federal coal share from Wyoming led to the lower overall CO2 emissions estimates in this report.
Unlike the relative decrease in CO2 emissions, CH4 emissions were approximately 4.6 percent higher on average in the updated estimate; though most of this increase is from 2005 to 2010. Some of the increase is due to a change in 100-year Global Warming Potential (GWP) for CH4 used in the studies from a value of 25 to 28. The previous report used GWP values from IPCC’s fourth assessment report (AR4) (Intergovernmental Panel on Climate Change, 2007). This 12 percent increase in GWP accounts for most of the increase in estimated emissions on a CO2 equivalent basis. A clerical error in the previous estimate for active surface coal-mining fugitive emissions in Wyoming was discovered when producing the current estimate. This resulted in an under-reporting of 2–3 million metric tons of carbon dioxide equivalent (MMT CO2 Eq.) of CH4 emissions per annum in the previous report by Merrill and others (2018). That error has been rectified in this report and is part of the higher emissions now reported in the overlapped time series. Balancing out some of these increases are decreases in the EPA-reported underground active and abandoned coal-mine emissions. Additionally, the previous methodology included whole mine emissions regardless of the non-zero portion of production that was Federal. This resulted in over attributing emissions to Federal leases. The current method apportions emissions by the percentage of Federal lease production in a mine per year.
An average five percent decline in estimated N2O emissions in this report is the result of multiple factors. A decrease in the GWP for N2O from a value of 298 in the original study to 265 would yield an 11 percent decrease; however, increases in reported EPA mobile N2O emissions, which are used as a scaling factor, reduce the impact of the GWP change. Additional smaller increases are the result of the addition of N2O emissions in the fugitive emission calculations for both petroleum and natural gas systems, these changes were modifications to the EPA’s U.S. GHG Inventory methodology since the previous report.
Precision, Rounding, Totals, and Uncertainty of Emissions Estimates
The emissions estimates summarized in this report and provided in the associated dataset (Merrill and others, 2024) were formatted to indicate their precision. For all non-total values, meaning those estimates that represent a single sector, gas, State, and year combination, the number of significant figures indicates the level of precision inherent in the calculations that generated that number. In the calculations used to generate the estimates, less precise sector ratios or emission factors are commonly the parameters with the least precision, and they limit the estimate’s precision.
For the convenience of the reader, this report and the associated dataset include totals for grouped sectors, or emissions categories, such as stationary combustion, mobile combustion, coal mine fugitive emissions, and grand totals. Unlike the individual sector values, the totals in this report and associated dataset do not intend to represent a level of precision; these totals are rounded values. These totals are formatted in a specific way to capture the 12 orders of magnitude in range that are present in the various estimates of this dataset. Totals greater than or equal to 1.0 MMT CO2 Eq. are rounded to the first decimal place, whereas values less than 1.0 MMT CO2 Eq. are shown with two significant figures. Applying either criteria to both large and small values will result in over rounded or unreported values. This method of representing the estimates, used only for totals, facilitates the comparison of a wide range of emissions totals.
Emissions category totals and U.S. Federal lands totals are provided in table 1.8 by greenhouse gas (GHG) and with uncertainty bounds (low, most likely, and high). Note that summing individual values in this report’s data release (Merrill and others, 2024) without exercising care can produce incorrect totals. There are two main reasons that incorrect totals can be produced: (1) small compounding errors from summing rounded totals, and (2) if summing low and high bound values, a significant error is introduced by incorrectly representing uncertainty at the totals level. The following text further explains these two reasons.
Performing calculations on the rounded total values, such as summing all stationary combustion totals rather than all individual combustion sector emissions, to obtain national combustion emissions, may incur compound rounding errors. These errors are small in the “most likely” estimates, but can be larger in the low or high bound totals, as explained below. Users of the dataset are advised to perform calculations on the individual values rather than the rounded totals when working on most likely emissions estimates.
Summing any low or high uncertainty bound values or subtotals to produce totals may greatly over and underestimate the actual low and high totals reported. Therefore, this is not recommended. Individual values have uncertainty bounds that are specific to that sector. The uncertainty bounds for totals are generally much narrower in range, which is due to some of the largest sources of emissions having low uncertainty driving the uncertainty of the total. Therefore, for those who wish to produce their own totals, it is recommended to perform summations on most likely values and then apply the proper, or best fitting, uncertainty bounds available from the EPA’s U.S. GHG Inventory (U.S. Environmental Protection Agency, 2024a). All totals in this report were calculated to avoid compound rounding errors and use the appropriate uncertainty for low and high bound totals.
Table 1.8.
Total greenhouse gas estimates for the stationary, mobile, and coal mine emission categories in addition to a grand total from this report.[Values are in million metric tons of carbon dioxide equivalent (MMT CO2 Eq.). Totals are presented by greenhouse gas (carbon dioxide [CO2], methane [CH4], and nitrous oxide [N2O]) and by low bound (Low), most likely (not symbolized), and high bound (High) estimates. These totals are produced from unrounded values. Similar totals produced from the data release (Merrill and others, 2024) may deviate at the decimal level due to compounding of rounding errors]
References Cited
Franklin, P., Scheehle, E., Collings, R.C., Coté, M.M., and Pilcher, R.C., 2004, Proposed methodology for estimating emission inventories from abandoned coal mines—Prepared for the 2006 IPCC [Intergovernmental Panel on Climate Change] National Greenhouse Gas Inventories Guidelines, Fourth Authors/Experts Meeting, Energy—Methane Emissions for Coal Mining and Handling, Arusha, Tanzania, [Sept. 28–30, 2004]: U.S. Environmental Protection Agency white paper, variously paged, accessed May 2, 2017, at https://www.epa.gov/sites/production/files/2016-03/documents/methodology_abandoned_coalmines.pdf.
Intergovernmental Panel on Climate Change, 2006, 2006 IPCC guidelines for national greenhouse gas inventories [Eggleston, S., Buendia, L., Miwa, K., Ngara, T., and Tannabe, K., eds.]: Hayama, Japan, Institute for Global Environmental Strategies, accessed May 20, 2017, at https://www.ipcc-nggip.iges.or.jp/public/2006gl/.
Intergovernmental Panel on Climate Change, 2007, Climate change 2007—The physical science basis—Contribution of Working Group I to the fourth assessment report of the Intergovernmental Panel on Climate Change [Solomon, S., Qin, D., Manning, M., Marquis, M., Averyt, K., Tignor, M.M.B., Miller, H.L., Jr., and Chen, Z., eds.]: Cambridge, United Kingdom, and New York, Cambridge University Press, 996 p. [Also available at https://www.ipcc.ch/pdf/assessment-report/ar4/wg1/ar4_wg1_full_report.pdf.]
Merrill, M.D., Sleeter, B.M., and Freeman, P.A., 2024, Federal lands greenhouse gas emissions and sequestration in the United States—Estimates for 2005–22 - data: U.S. Geological Survey data release, https://doi.org/10.5066/P14CVD9R.
Merrill, M.D., Sleeter, B.M., Freeman, P.A., Liu, J., Warwick, P.D., and Reed, B.C., 2018, Federal lands greenhouse emissions and sequestration in the United States—Estimates for 2005–14: U.S. Geological Survey Scientific Investigations Report 2018–5131, 31 p., accessed May 20, 2019, at https://doi.org/10.3133/sir20185131.
Office of Natural Resources Revenue, 2023, Minerals production reporter handbook (release 3.1): Office of Natural Resources Revenue handbook, appendix 1 [disposition/adjustment codes], 11 p., accessed February 5, 2024, at https://www.onrr.gov/document/MPRH-Appendix-I.pdf.
Office of Natural Resources Revenue, 2024, Natural resources revenue data—Production disposition by month: Office of Natural Resources Revenue web page, accessed April 24, 2024, at https://revenuedata.doi.gov/downloads/production-by-disposition/.
U.S. Energy Information Administration, 2021, Table 2.1, nonfuel (feedstock) use of combustible energy, 2018, in Manufacturing energy consumption survey (MECS)—2018 MECS survey data: U.S. Energy Information Administration, 22 p., accessed September 15, 2024, at https://www.eia.gov/consumption/manufacturing/data/2018/pdf/Table2_1.pdf.
U.S. Energy Information Administration, 2022, Annual coal distribution report 2022, by coal origin State: U.S. Energy Information Administration [report], 94 p., accessed January 31, 2024, at https://www.eia.gov/coal/distribution/annual/.
U.S. Energy Information Administration, 2023a, Table 23, Percent yield of petroleum products by PAD and refining districts, 2023, in Petroleum supply annual, v. 1: U.S. Energy Information Administration, 1 p., accessed November 21, 2023, at https://www.eia.gov/petroleum/supply/annual/volume1/.
U.S. Energy Information Administration, 2023b, Table 3.5, Petroleum products supplied by type and table 3.7, Petroleum consumption by sector, in Monthly energy review, November 2023: U.S. Energy Information Administration, 296 p., accessed December 1, 2023, at https://www.eia.gov/totalenergy/data/monthly/archive/00352311.pdf.
U.S. Energy Information Administration, 2024a, Table 4.3, Natural gas consumption by sector, in Monthly energy review, February 2023: U.S. Energy Information Administration, p. 106, accessed February 28, 2024, at https://www.eia.gov/totalenergy/data/monthly/archive/00352302.pdf.
U.S. Energy Information Administration, 2024b, The distribution of U.S. oil and natural gas wells by production rate with data through 2022, December 2023: U.S. Energy Information Administration, appendix C [Excel table in separate file], accessed April 10, 2024, at https://www.eia.gov/petroleum/wells/.
U.S. Environmental Protection Agency, 2004, Methane emissions from abandoned coal mines in the United States—Emissions inventory methodology and 1990–2002 emissions estimates: U.S. Environmental Protection Agency, [report] EPA 430–R–04–001, 54 p. [Also available at https://nepis.epa.gov/Exe/ZyPDF.cgi/600004JM.PDF?Dockey=600004JM.PDF.]
U.S. Environmental Protection Agency, 2023, Emission factors for greenhouse gas inventories: U.S. Environmental Protection Agency, 7 p., accessed September 2023, at https://www.epa.gov/climateleadership/ghg-emission-factors-hub.
U.S. Environmental Protection Agency, 2024a, Inventory of U.S. greenhouse gas emissions and sinks—1990–2022: U.S. Environmental Protection Agency, [report] EPA 430–R–24–004, variously paged, plus additional online data tables, accessed April 5, 2024, at https://www.epa.gov/ghgemissions/inventory-us-greenhouse-gas-emissions-and-sinks-1990-2022.
U.S. Environmental Protection Agency, 2024b, Annexes to the inventory of U.S. GHG emissions and sinks [—to accompany U.S. Environmental Protection Agency, Inventory of U.S. greenhouse gas emissions and sinks—1990–2022, [report] EPA 430–R–24–004]: U.S. Environmental Protection Agency, 595 p., accessed April 5, 2024, at https://www.epa.gov/ghgemissions/inventory-us-greenhouse-gas-emissions-and-sinks-1990-2022.
U.S. Environmental Protection Agency, 2024c, Annex 3.5—Methodology for Estimating CH4, CO2, and N2O emissions from petroleum systems, in Natural gas and petroleum systems in the GHG inventory—Additional information on the 1990–2015 GHG inventory (published April 2024) [—to accompany U.S. Environmental Protection Agency, Inventory of U.S. greenhouse gas emissions and sinks—1990–2022, [report] EPA 430–R–24–004]: U.S. Environmental Protection Agency, 23 tables, accessed April 5, 2024, at https://www.epa.gov/ghgemissions/natural-gas-and-petroleum-systems-ghg-inventory-additional-information-1990-2022-ghg.
U.S. Environmental Protection Agency, 2024d, Annex 3.6—Methodology for estimating CH4, CO2, and N2O emissions from natural gas systems, in Natural gas and petroleum systems in the GHG inventory—Additional information on the 1990–2022 GHG inventory (published April 2024) [—to accompany U.S. Environmental Protection Agency, Inventory of U.S. greenhouse gas emissions and sinks—1990–2022, [report] EPA 430–R–24–004]: U.S. Environmental Protection Agency, 11 tables, accessed April 5, 2024, at https://www.epa.gov/ghgemissions/natural-gas-and-petroleum-systems-ghg-inventory-additional-information-1990-2022-ghg.
U.S. Environmental Protection Agency, 2024e, Facility level information on greenhouse gases tool (FLIGHT)—Greenhouse gas emissions from large facilities: U.S. Environmental Protection Agency web page, accessed April 17, 2024, at https://ghgdata.epa.gov/ghgp/main.do.
Appendix 2. Detailed Methods: Terrestrial Ecosystem-Associated Carbon Emissions and Sequestration on Federal Lands
Introduction
For this report, we used a state-and-transition simulation model (STSM) with fully coupled stocks and flows (the Land Use and Carbon Scenario Simulator [LUCAS] model) to estimate changes in ecosystem carbon balance for all Federal land areas in the conterminous United States. Within these areas, we estimated the changes in carbon stocks and fluxes for forests, grasslands, shrublands, and wetlands resulting from processes associated with agricultural contraction and expansion, urbanization, forest harvest, and wildfire. Historical changes in land use and land cover (LULC) were estimated from a range of remote sensing-based datasets. Carbon dynamics within the LUCAS model were calibrated with growth curves derived from forest inventory and analysis (FIA) data from the U.S. Department of Agriculture Forest Service (Gray and others, 2012). A complete description of the methods used can be found in Sleeter and others (2022).
LULC Initial Conditions
The LUCAS model used here estimated changes in ecosystem carbon balance for the period 2001–21. The model was initialized for the year 2001 using the following approach. The 2001 National Land Cover Database (NLCD) (Homer and others, 2007) was used to define the LULC class for each pixel. To identify forest land use, we assumed that if a cell was ever classified as forest in any of the NLCD time periods, then it was assumed to be forest in 2001. This approach accounts for cells that were recently harvested or disturbed, and therefore likely classified as either shrubland or herbaceous cover, to be correctly identified as forest. Forest cells were then assigned a forest type-group using Landfire’s Existing Vegetation Type (Rollins, 2008), and stand age was derived from Williams and others (2020). For cells classified as either shrubland or herbaceous cover, we assigned a grassland/shrubland type based on data from the Rangeland Condition Monitoring Assessment and Projection (RCMAP) project (Rigge and others, 2024), while cells mapped as wetlands in NLCD were assigned a wetland type from NOAA’s Coastal Change and Analysis Program (C-CAP) data (National Oceanic and Atmospheric Administration, 2024). Wetlands are subdivided into four classes: estuarine herbaceous, estuarine forested, palustrine herbaceous, and palustrine forested. Wetlands not included within the NOAA C-CAP data extent that were classified as herbaceous wetlands in NLCD were assumed to be palustrine herbaceous wetlands. In areas where C-CAP had coverage, we used C-CAP to distinguish between palustrine and estuarine categories. All other LULC classes remained the same as their 2001 NLCD classification.
LULC Change and Disturbance
We modeled the following LULC conversions for this report: urbanization, agricultural expansion, agricultural contraction, and intensification of development areas. The NLCD time series of maps was used to identify areas experiencing LULC changes and the rates of change were annualized and stochastically selected within each time step. Forest harvest was modeled using an annual time series of maps from Landfire Disturbance (LANDFIRE, 2023). We identified both clearcut harvest and selection harvest transitions from the Landfire Disturbance product and produced annual maps of these areas as inputs into the model. The same approach was used for fire, where areas of high, moderate, and low severity fires were mapped from the Monitoring Trends in Burn Severity database (Eidenshink and others, 2007). For both fire and harvest transitions, we assumed the original land-cover class returned post disturbance. For clearcut harvest and high severity fire, the stand age was reset to zero.
Carbon Model
This study followed the same approach and methods as that described in detail in Sleeter and others (2022). LUCAS defines 14 carbon pools, including 5 live pools and 9 pools characterizing dead organic matter such as deadwood and litter. Carbon is transferred between pools on an annual timestep based on coefficients derived from the Carbon Budget Model of the Canadian Forest Sector (CBM-CFS3; Kurz and others, 2009). The LUCAS model does not have an explicit depth limitation to tracking soil carbon pools. Rather, the model follows the same general approach as the CBM-CFS3 model (Kurz and others, 2009) and works to find an equilibrium with the whole system, but it does not infer any specific depth. Rates of net primary productivity (NPP) are further modified on an annual timestep for each grid cell based on an external model of NPP (Del Grosso and others, 2008; Sleeter and others, 2022). Additionally, we included uncertainty in baseline NPP as derived from an analysis of plot data from the U.S. Department of Agriculture (Gray and others, 2012). As such, each Monte Carlo iteration of the LUCAS model samples from a predefined distribution of NPP for each forest type-group. Annual maps of temperature are used to scale the rate of decomposition in deadwood and litter pools for each timestep and grid cell (Sleeter and others, 2022). We did not include the effects of changes in precipitation on decomposition other than the base rates, which were described in Kurz and others (2009) and Sleeter and others (2022). We appreciate this may be an important aspect to turnover of dead organic matter pools, however, it was beyond the scope of this report.
Sleeter and others (2022) describe the complete methodology for modeling carbon used in this report. However, it does not describe the parameters used for grasslands, shrublands, and wetlands. We used the Integrated Biosphere Simulator (IBIS) dynamic global vegetation model to derive estimates of NPP and biomass turnover for 3 grassland types and 6 shrubland classes. For wetlands we used estimates derived by Stagg and others (2017, 2018).
Model Simulations
For this report, the LUCAS model was run for the period 2001–21 on an annual timestep. We simulated LULC change and ecosystem carbon dynamics at a spatial resolution of 1-km x 1-km for all cells in the conterminous United States. Twenty Monte Carlo realizations of the model were run. Results summarized all pixels, which were classified as Federal lands while omitting cells classified as Bureau of Indian Affairs. Annual, State-level outputs from this work are available in this report’s associated data release (Merrill and others, 2024).
References Cited
Del Grosso, S., Parton, W., Stohlgren, T., Zheng, D., Bachelet, D., Prince, S., Hibbard, K., and Olson, R., 2008, Global potential net primary production predicted from vegetation class, precipitation, and temperature: Ecology. v. 89, no. 8, p. 2117–2126. [Also available at https://doi.org/10.1890/07-0850.1.]
Eidenshink, J., Schwind, B., Brewer, K., Zhu, Z.-L., Quayle, B., and Howard, S., 2007, A project for monitoring trends in burn severity: Fire Ecology, v. 3, no. 1, p. 3–21. [Also available at https://doi.org/10.4996/fireecology.0301003.]
Gray, A.N., Brandeis, T.J., Shaw, J.D., McWilliams, W.H., and Miles, P.D., 2012, Forest inventory and analysis database of the United States of America (FIA), in Dengler, J., Oldeland, J., Jansen, F., Chytry, M., Ewald, J., Finckh, M., Glockler, F., Lopez-Gonzalez, G., Peet, R.K., and Schaminee, J.H.J., eds., Vegetation databases for the 21st century: Biodiversity and Ecology, v. 4, p. 225–231. [Also available at https://doi.org/10.7809/B-E.00079.]
Kurz, W.A., Dymond, C.C., White, T.M., Stinson, G., Shaw, C.H., Rampley, G.J., Smyth, C., Simpson, B.N., Neilson, E.T., Trofymow, J.A., Metsaranta, J., and Apps, M.J., 2009, CBM-CFS3—A model of carbon-dynamics in forestry and land-use change implementing IPCC standards: Ecological Modelling, v. 220, no. 4, p. 480–504. [Also available at https://doi.org/10.1016/j.ecolmodel.2008.10.018.]
LANDFIRE, 2023, LANDFIRE Annual Disturbance layer (June 2023, last update): U.S. Geological Survey and U.S. Department of Agriculture web page, accessed July 18, 2023, at https://landfire.gov/disturbance/annualdisturbance.
Merrill, M.D., Sleeter, B.M., and Freeman, P.A., 2024, Federal lands greenhouse gas emissions and sequestration in the United States—Estimates for 2005–22 - data: U.S. Geological Survey data release, https://doi.org/10.5066/P14CVD9R.
National Oceanic and Atmospheric Administration, 2024, NOAA Coastal Change Analysis Program (C-CAP) regional 30 meter land cover [year 2001 data file]: National Oceanic and Atmospheric Administration Office for Coastal Management web page, accessed January 2024, at https://www.coast.noaa.gov/htdata/raster1/landcover/bulkdownload/30m_lc/.
Rigge, M.B., Bunde, B., Postma, K., and Shi, H., 2024, Rangeland condition monitoring assessment and projection (RCMAP) fractional component time-series across the western U.S. 1985–2023: U.S. Geological Survey data release, accessed September 30, 2024 at https://doi.org/10.5066/P9SJXUI1.
Rollins, M.G., 2008, LANDFIRE—A nationally consistent vegetation, wildland fire, and fuel assessment: International Journal of Wildland Fire, v. 18, no. 3, p. 235–249, accessed October 1, 2010, at https://doi.org/10.1071/WF08088.
Stagg, C.L., Baustian, M.M., Perry, C.L., Carruthers, T.J.B., and Hall, C.T., 2018, Direct and indirect controls on organic matter decomposition in four coastal wetland communities along a landscape salinity gradient: Journal of Ecology, v. 106, no. 2, p. 655–670. [Also available at https://doi.org/10.1111/1365-2745.12901.]
Stagg, C.L., Schoolmaster, D.R., Jr., Piazza, S.C., Snedden, G., Steyer, G.D., Fischenich, C.J., and McComas, R.W., 2017, A landscape-scale assessment of above- and belowground primary production in coastal wetlands—Implications for climate change-induced community shifts: Estuaries and Coasts, v. 40, p. 856–879. [Also available at https://doi.org/10.1007/s12237-016-0177-y.]
Williams, C.A., Hasler, N., Gu, H., and Zhou, Y., 2020, Forest carbon stocks and fluxes from the NFCMS, conterminous USA, 1990–2010 [dataset] (ver. 2): Oak Ridge National Laboratory, Distributed Active Archive Center web page, accessed September 30, 2024, at https://doi.org/10.3334/ORNLDAAC/1829.
Conversion Factors
Abbreviations
BLM
Bureau of Land Management
C-CAP
Coastal Change and Analysis Program
CH4
methane
CO2
carbon dioxide
CO2-EOR
enhanced oil recovery using CO2
CO2 Eq.
carbon dioxide equivalent
EIA
U.S. Energy Information Administration
EPA
U.S. Environmental Protection Agency
Fed-CONUS
Federally administered surface lands in the conterminous United States
GHG
greenhouse gas
GHGRP
Greenhouse Gas Reporting Program
GPP
gross primary productivity
HWP
harvest wood products
IBIS
Integrated Biosphere Simulator
km
kilometer
LUCAS
Land Use and Carbon Scenario Simulator
LULC
land use and land cover
m
meter
MMT CO2 Eq.
million metric tons of carbon dioxide equivalent
MMT CO2 Eq./yr
million metric tons of carbon dioxide equivalent per year
MSHA
Mine Safety and Health Administration
MT CO2 Eq./ha
metric tons of carbon dioxide equivalent per hectare
MT CO2 Eq./ha/yr
metric tons of carbon dioxide equivalent per hectare per year
NBP
net biome productivity
NEE
net ecosystem exchange
NEP
net ecosystem productivity
NEPA
National Environmental Protection Act
NLCD
National Land Cover Database
N2O
nitrous oxide
NOAA
National Oceanic and Atmospheric Administration
NPP
net primary productivity
NRRD
Natural Resources Revenue Data
OCS
Outer Continental Shelf
ONRR
Office of Natural Resources Revenue
P&A
plugged and abandoned
PADUS
Protected Areas Database
Ra
autotrophic respiration
RCMAP
Rangeland Condition Monitoring Assessment and Projection
Rh
heterotrophic respiration
TEC
total ecosystem carbon
USGS
U.S. Geological Survey
For more information about this publication, contact
Director, Geology, Energy & Minerals Science Center
U.S. Geological Survey
956 National Center
12201 Sunrise Valley Drive
Reston, VA 20192
For additional information visit https://www.usgs.gov/centers/geology-energy-and-minerals-science-center
Publishing support provided by the Reston Publishing Service Center
Disclaimers
Any use of trade, firm, or product names is for descriptive purposes only and does not imply endorsement by the U.S. Government.
Although this information product, for the most part, is in the public domain, it also may contain copyrighted materials as noted in the text. Permission to reproduce copyrighted items must be secured from the copyright owner.
Suggested Citation
Merrill, M.D., Sleeter, B.M., and Freeman, P.A., 2024, Federal lands greenhouse gas emissions and sequestration in the United States—Estimates for 2005–22: U.S. Geological Survey Scientific Investigations Report 2024–5103, 39 p., https://doi.org/10.3133/sir20245103. [Supersedes USGS Scientific Investigations Report 2018–5131.]
ISSN: 2328-0328 (online)
Study Area
Publication type | Report |
---|---|
Publication Subtype | USGS Numbered Series |
Title | Federal lands greenhouse gas emissions and sequestration in the United States: Estimates for 2005–22 |
Series title | Scientific Investigations Report |
Series number | 2024-5103 |
DOI | 10.3133/sir20245103 |
Year Published | 2024 |
Language | English |
Publisher | U.S. Geological Survey |
Publisher location | Reston, VA |
Contributing office(s) | Geology, Energy & Minerals Science Center |
Description | Report: viii, 39 p.; Data Release |
Country | United States |
Online Only (Y/N) | Y |
Additional Online Files (Y/N) | N |
Google Analytic Metrics | Metrics page |