National Assessment of Coastal Vulnerability to Sea-Level Rise: Preliminary Results for the U.S. Pacific Coast
Risk Variables
In order to develop a database for a national-scale assessment of coastal vulnerability, relevant information has been gathered from local, state and federal agencies, as well as academic institutions. The compilation of this database is integral to mapping potential coastal changes due to sea-level rise. This database loosely follows an earlier database developed by Gornitz and White (1992). A comparable assessment of the sensitivity of the Canadian coast to sea-level rise is presented by Shaw et al.(1998).
The input data for this database of coastal vulnerability have been assembled using the original, and sometimes variable, horizontal resolution, which then was resampled to a 3-minute grid cell. A data set for each risk variable is then linked to each grid point. For mapping purposes, data stored in the 3-minute grid is transferred to a 1:2,000,000 vector shoreline with each segment of shoreline lying within a single grid cell.
Click on chart for larger image
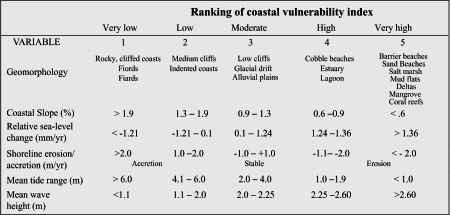 |
|
Table 1. Ranking of coastal vulnerability index variables for the U.S. Pacific Coast. |
Table 1 summarizes the six physical variables used here:
- geomorphology,
- coastal slope (percent),
- rate of relative sea-level rise (mm/yr),
- shoreline erosion and accretion rates (m/yr),
- mean tidal range (m) and
- mean wave height (m).
As described below, each variable is assigned a relative risk value based on the potential magnitude of its contribution to physical changes on the coast as sea-level rises. The designated ranges are based on previously defined ranges and on histograms and statistical summaries which, for certain variables, describe the regional trends along the U.S. Pacific Coast.
The geomorphology variable expresses the relative erodibility of different landform types (Table 1). These data were derived from state geologic maps and USGS 1:250,000 scale topographic maps, as well as correlated with data compiled in the Cascadia beach-shoreline database, Pacific Northwest Region (Peterson et al., 1994), and with the Living with the Shore book series (Griggs and Savoy, 1985; Terich, 1987; Komar, 1997).
The regional slope permits an evaluation of not only the relative risk of inundation, but also the potential rapidity of shoreline retreat, because low-sloping coastal regions should retreat faster than steeper regions (Pilkey and Davis, 1987). The regional slope of the coastal zone was calculated from a grid of topographic and bathymetric elevations extending landward and seaward of the shoreline. In order to compute the slope from the subaerial coastal plain to the submerged continental shelf, the slope for each grid cell was calculated by defining elevation extremes within a 10 km radius for each individual grid cell along the shoreline. In areas where the shelf/slope break is less than 10 km offshore, the slope was recalculated with a more appropriate radius. For the U.S. Pacific coast, elevation data were obtained from the U.S. Navy ETOPO5 digital topographic and bathymetric elevation database with elevations to the nearest 1 meter for 5-minute grid cells. These data were subsampled to 3-minute (approximately 5 km) resolution to be consistent with our other coastal databases (Thieler and Hammar-Klose, 1999).
The relative sea-level change variable is derived from the increase (or decrease) in annual mean water elevation over time as measured at tide gauge stations along the coast (e.g., Emery and Aubrey, 1991). Relative sea-level change data were obtained for 12 National Ocean Service (N.O.S.) data stations and contoured along the coastline. This variable inherently includes both global eustatic sea-level rise as well as local isostatic or tectonic land motion. Relative sea-level change data are a historical record, and thus show change for only recent time scales (past 50-100 yr).
Shoreline erosion and accretion rates for the U.S. have been compiled by May and others (1983) and Dolan and others (1985) into the Coastal Erosion Information System (CEIS) (May and others, 1982). CEIS includes shoreline change data for the Atlantic, Gulf of Mexico, Pacific and Great Lakes coasts, as well as major bays and estuaries. The data in CEIS are drawn from a wide variety of sources, including published reports, historical shoreline change maps, field surveys and aerial photo analyses. However, the lack of a standard method among coastal scientists for analyzing shoreline changes has resulted in the inclusion of data utilizing a variety of reference features, measurement techniques, and rate-of-change calculations. Thus, while CEIS represents the best available data for the U.S. as a whole, much work is needed to accurately document regional and local erosion rates.
Where higher-quality data are available, we replace and augment the CEIS data with shoreline change data obtained from states and local agencies. In this report, for example, the erosion rate data for the ocean coast of Washington State and Clatsop County, Oregon were provided by the Coastal Monitoring and Analysis Program, Washington Department of Ecology, Olympia, Washington (R. C. Daniels, pers. comm., 2000). These long-term annual erosion rates for the ocean coast are derived from N.O.S. T-Sheets for the years 1926 - 30 and orthophotoquads for the years 1994 - 96; rates for the bays are derived from N.O.S. T-Sheets from 1950 and orthophotoquads for the years 1994 - 96.
Tide range is linked to both permanent and episodic inundation hazards. Tide range data were obtained from the N.O.S. for 160 tide stations along the U.S. Pacific coast; the values were contoured along the coastline.
Wave height is used here as an indicator of wave energy, which drives the coastal sediment budget. Wave energy increases as the square of the wave height; thus the ability to mobilize and transport beach/coastal material is a function of wave height. In this report, we use hindcast nearshore mean wave height data for the period 1956-1975 obtained from the U.S. Army Corps of Engineers Wave Information Study (WIS) (see references in Hubertz et al., 1996). The model wave heights were compared to historical measured wave height data obtained from the NOAA National Data Buoy Center. Wave height data for 182 WIS stations along the U.S. Pacific coast were contoured along the coastline.
|