Geologic Carbon Management Options for the North Atlantic-Appalachian Region
Links
- Document: Report (4.32 MB pdf) , HTML , XML
- Download citation as: RIS | Dublin Core
Introduction
The U.S. Geological Survey (USGS) North Atlantic-Appalachian Region is developing the regionwide capacity to provide timely science support for decision-makers attempting to enhance carbon removal, sequestration, and emissions mitigation to meet national atmospheric carbon reduction goals. The U.S. Environmental Protection Agency (EPA) reported that in 2021, the fourteen States and the District of Columbia in the northeastern region account about for approximately 18 percent of the total national greenhouse gas (GHG) emissions (fig. 1). This Fact Sheet provides a summary of USGS science information and ongoing and new investigations or data-collection programs that may help the northeastern region decrease the release of carbon-containing GHG to the atmosphere.
Geologic Carbon Storage
Geologic carbon sequestration is the process of storing carbon dioxide (CO2) in underground geologic formations. Carbon dioxide gas can be captured from industrial processes (for example, at factories and power plants) or from the atmosphere by direct air-capture facilities. The captured CO2 is compressed to form a liquid, and then is injected underground into porous rock formations in geologic basins.
Onshore and Offshore Geologic Carbon Storage Areas in the Northeastern Region
In 2013, the USGS identified and assessed several onshore areas in the northeastern region where CO2 could be stored in underground geologic reservoirs, and Gupta (2019) identified and assessed offshore areas adjacent to the northeastern region that could be suitable for geologic CO2 storage (fig. 1). The USGS has the expertise to assess subsurface geologic resources in the northeastern region, including areas with the potential for subsurface CO2 and energy storage.
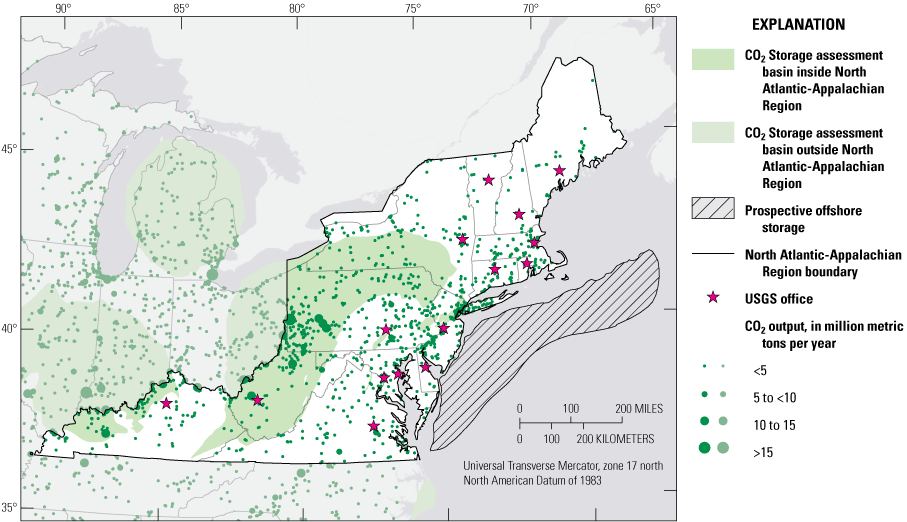
Map showing potential onshore and offshore geologic subsurface carbon dioxide (CO2) storage areas and industrial CO2 source locations in the U.S. Geological Survey (USGS) North Atlantic-Appalachian Region (NAAR) and surrounding areas; darker shaded colors are in the NAAR. USGS offices in the NAAR are also indicated on the map. Data sources: U.S. Geological Survey Geologic Carbon Dioxide Storage Resources Assessment Team, 2013; National Carbon Sequestration Database and Geographic Information System Map Team, 2015; Gupta, 2019.
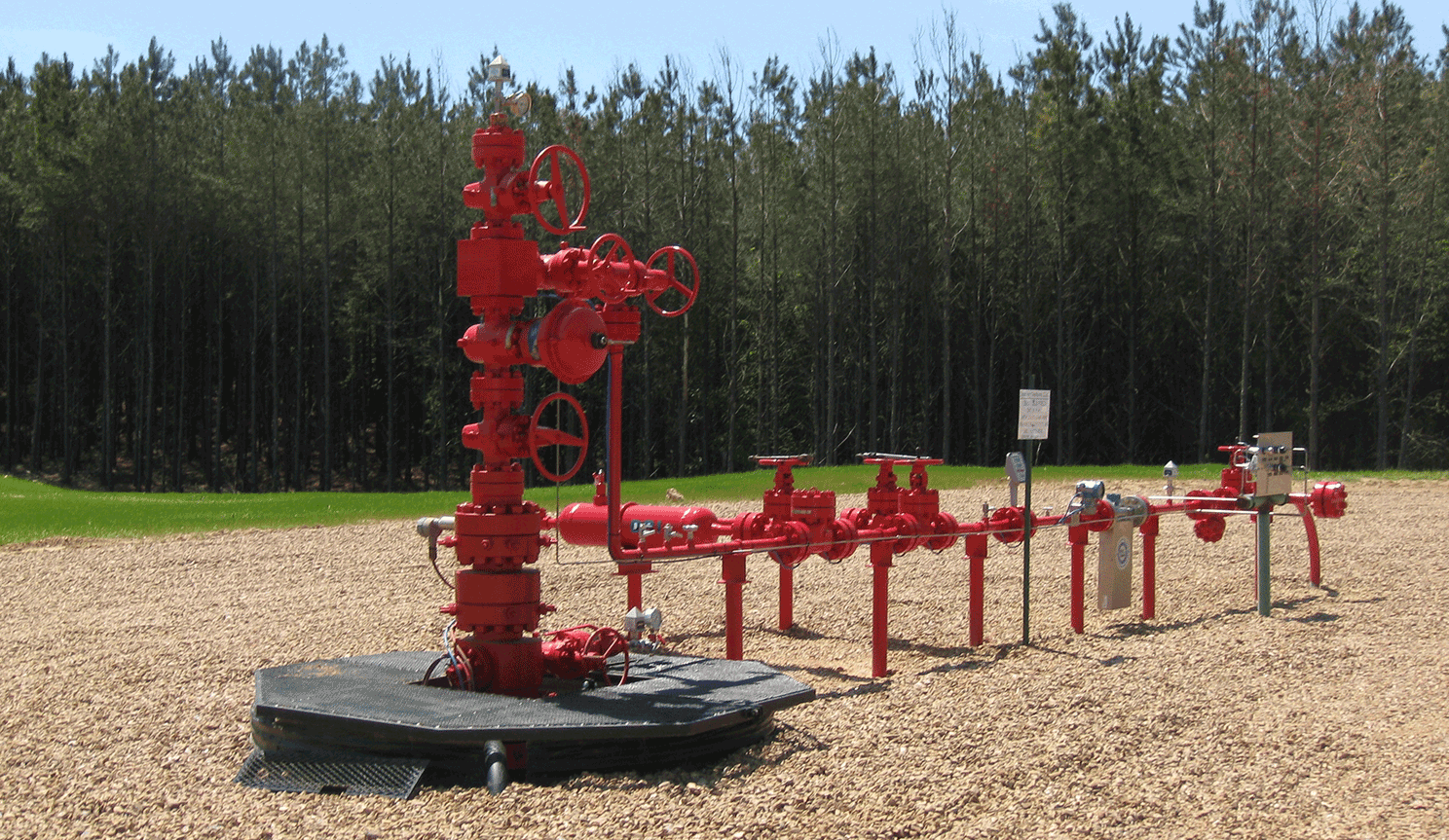
Carbon dioxide injection well. Photograph by Peter Warwick, U.S. Geological Survey.
Carbon Dioxide Mineralization Opportunities in the Northeastern Region
Carbon dioxide mineralization is a chemical process by which CO2 becomes part of a solid mineral, such as a carbonate. This chemical reaction happens naturally when certain rocks are exposed to CO2, and this process can also be sped up artificially. An advantage of CO2 mineralization is that CO2 can be effectively trapped in the newly formed mineral. Rocks that have the potential for CO2 mineralization are mostly igneous (primarily mafic and ultramafic) or metamorphic (for example, rocks that contain asbestos) rather than sedimentary rocks. There are two primary types of geologic CO2 mineralization: injection of CO2 or a mixture of CO2 and water into rock formations deep underground, and exposing broken pieces of rock at Earth’s surface, such as leftover rocks from mining (mine tailings), to CO2 (Blondes and others, 2019). Areas within and adjacent to the northeastern region where CO2 mineralization might be feasible are shown in figure 2; however, more research is needed to quantify the total CO2 storage potential at each of these sites. Nationally, CO2 mineralization storage resources are poorly defined. Globally, CO2 mineralization may have the potential of sequestering up to 60,000,000 billion metric tons of CO2 (Kelemen and others, 2019).
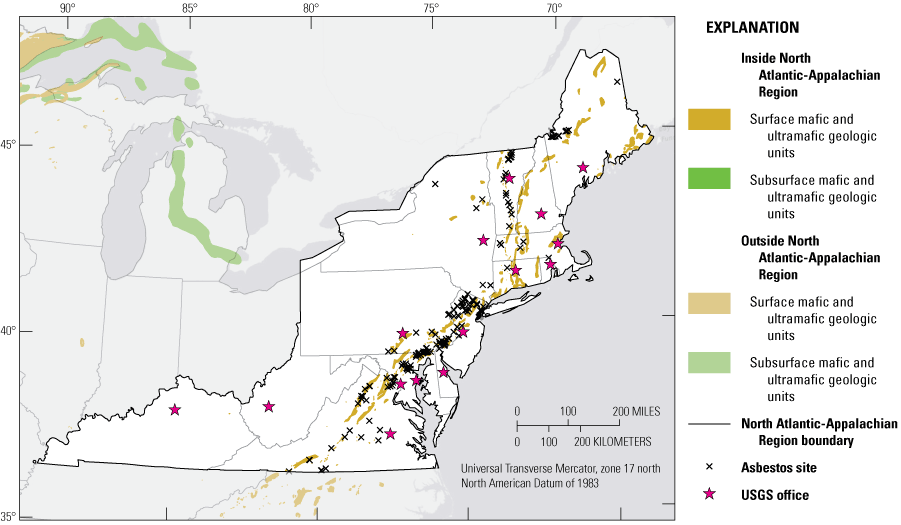
Map showing potential areas for carbon dioxide mineralization that include asbestos sites and mafic and ultramafic geologic units in the U.S. Geological Survey (USGS) North Atlantic-Appalachian Region (NAAR) and surrounding areas; darker shaded colors are in the NAAR. USGS offices in the NAAR are also indicated on the map. Asbestos sites are historic asbestos mines, historic asbestos prospects, and natural asbestos occurrences. Data sources: Blondes and others, 2019, 2022, and references therein.
Adding Carbon Dioxide-Reactive Minerals to Coastal Beaches and Wetlands
On geologic timescales, weathering of some kinds of igneous rocks is an important control on atmospheric CO2 concentrations because this geochemical process removes CO2 from the atmosphere. One approach that may help with CO2 capture and storage is the addition of amendments such as olivine (a mineral found in mafic and ultramafic igneous rocks) to coastal beaches and wetland areas. When olivine weathers, the process not only removes CO2 from the atmosphere, it also increases ocean alkalinity by sequestering CO2 in seawater in the form of a bicarbonate ion. Seawater bicarbonate ions make up about 85 to 90 percent of seawater alkalinity and have a residence time of about 100,000 years in seawater (Emerson and Hedges, 2008), making this olivine weathering process an effective way to sequester CO2. Ocean CO2 removal technologies based on this weathering process could mitigate both ocean acidification and climate change (Montserrat and others, 2017; National Academies of Sciences, Engineering, and Medicine, 2022).
The northeastern region has over 2,000 kilometers of coastline with associated coastal wetlands that might be potential candidates for the application of mafic and ultramafic mineral amendments (fig. 1). Additional research is needed to evaluate and model the effects of application of mineral amendments to salt marshes, beaches, coastal wetlands, and similar environments, including effects on biota, soils, and coastal ocean chemistry. Models of the CO2 storage potential resulting from large-scale applications of mineral amendments to coastal environments need to be developed. Ultrabasic sand amendments could be spread in thin layers or combined with dredge spoils that are added to coastal wetlands as a way to use natural materials to increase elevation resilience relative to sea-level rise. This is particularly important in the approximately 25 percent of U.S. wetlands that are in degraded condition and have subsided soils (Kroeger and others, 2017). More research is needed to identify suitable application sites and potential effects on the environment.
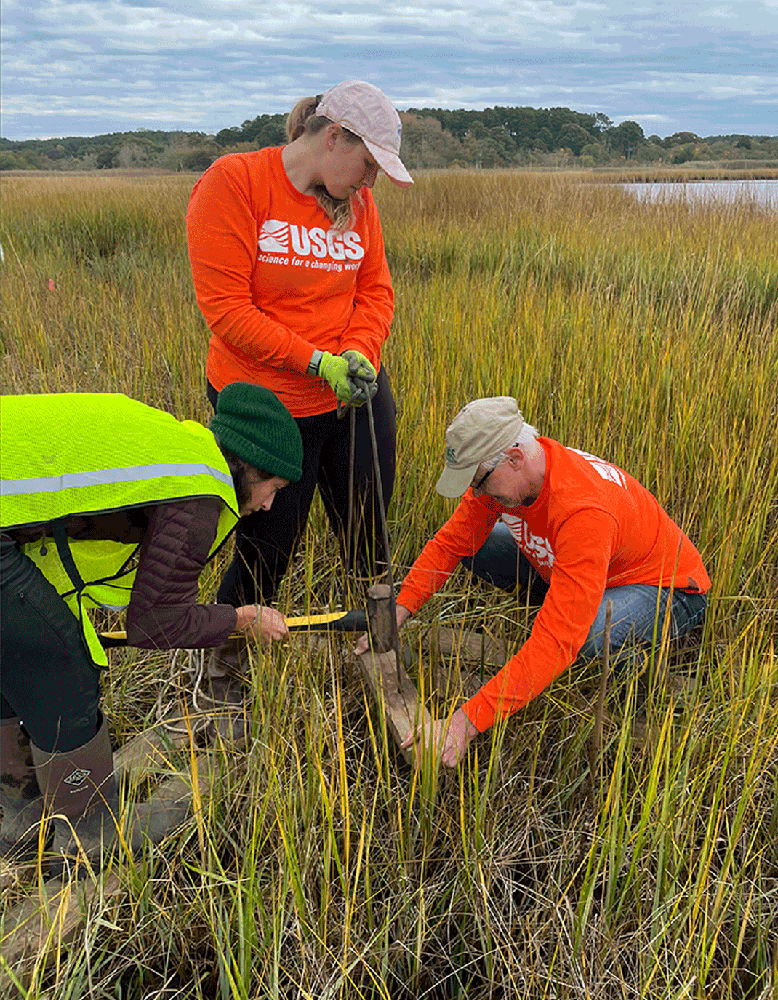
USGS and National Park Service scientists collect a soil core from a salt marsh site where the mineral olivine was applied to study its role in capturing carbon dioxide in tidal wetlands. Photograph by U.S. Geological Survey.
Methane Emission Mitigation
Many opportunities exist in the northeastern region to reduce GHG emissions of methane (CH4) that is released from abandoned and unplugged orphaned oil and gas wells and active and abandoned coal mines.
Abandoned and Unplugged Orphaned Oil and Gas Wells
Fugitive or leaking GHG emissions, particularly CH4 from unproductive abandoned oil and gas wells, are significant sources of GHG that can be remediated through proper well decommissioning or plugging. Unplugged orphaned wells are nonproducing wells that no longer have an operator to properly plug them. Some abandoned wells may be plugged; however, because of the methods and materials used or age of the plugging material, these wells can also be a source of emissions. The EPA estimated that all abandoned oil and gas wells in the United States, including orphaned or idle wells, emitted 276,000 metric tons of CH4 in 2020. The 2021 Bipartisan Infrastructure Law, formally called the Infrastructure Investment and Jobs Act (Public Law 117-58, 135 stat. 429), provides funding to plug these wells and reduce their GHG emissions.
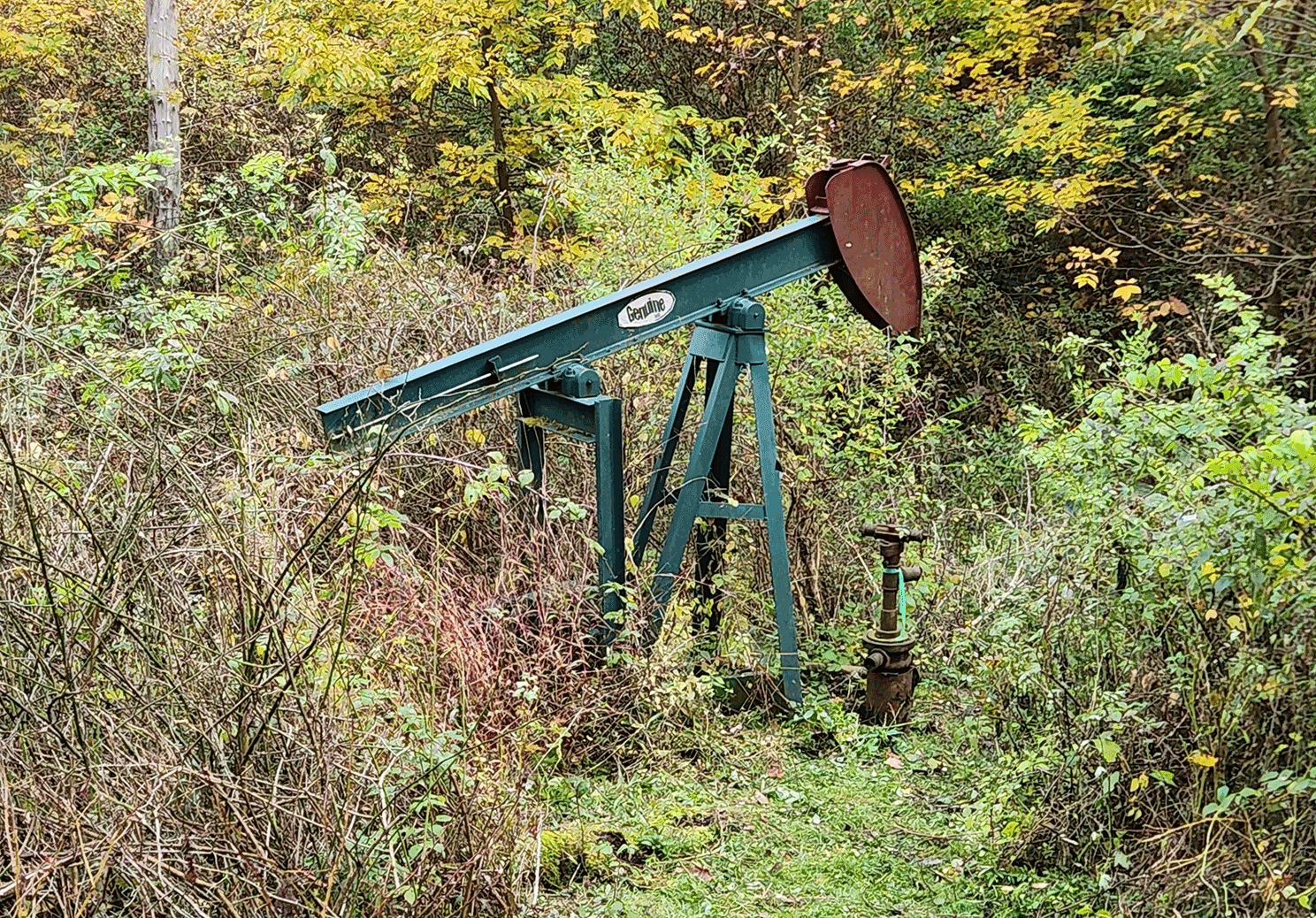
Orphaned well in Pennsylvania. Photo by Nicholas Gianoutsos, U.S. Geological Survey.
During the early history of oil and gas exploration in the United States, locations of many oil and gas wells often were not well-documented, and some of these wells were subsequently abandoned or orphaned. Without location data for abandoned or orphaned oil and gas wells, emissions cannot be checked, and these wells cannot be prioritized for proper decommissioning. Records of abandoned and orphaned oil and gas well locations from oil- and gas-producing States may or may not be complete, but a preliminary national dataset of locations of unplugged orphaned oil and gas wells was recently published by the USGS (Grove and Merrill, 2022; Merrill and others, 2023) (fig. 3). This dataset can be used for planning which orphaned wells to prioritize for decommissioning. The USGS is also assisting other Bureaus in the Department of the Interior with measurements of CH4 emissions from unplugged orphaned oil and gas wells before and after plugging and site remediation. Improved measurements of CH4 emissions will help to better characterize the GHG emission reductions resulting from plugging orphaned wells.
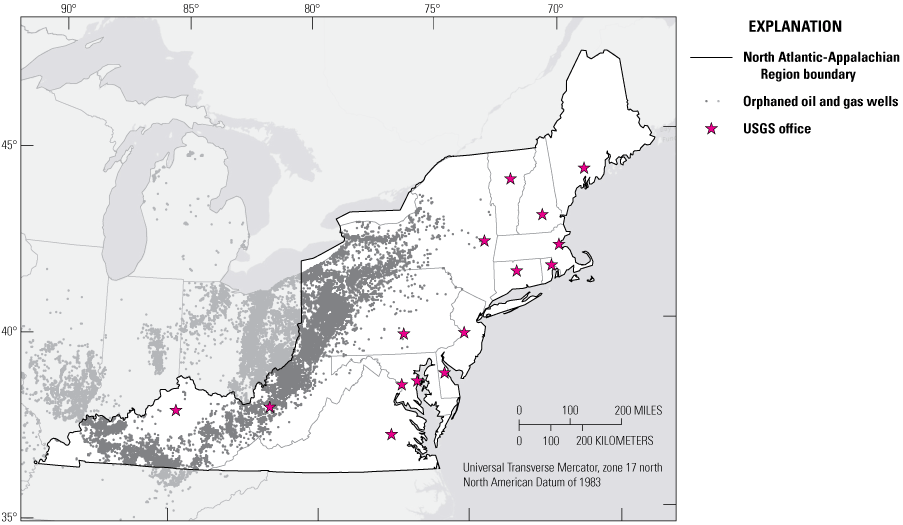
Map showing unplugged orphaned oil and gas wells in the U.S. Geological Survey (USGS) North Atlantic-Appalachian Region (NAAR) and surrounding areas; darker shaded colors are in the NAAR. USGS offices in the NAAR are also indicated on the map. Data source: Grove and Merrill, 2022.
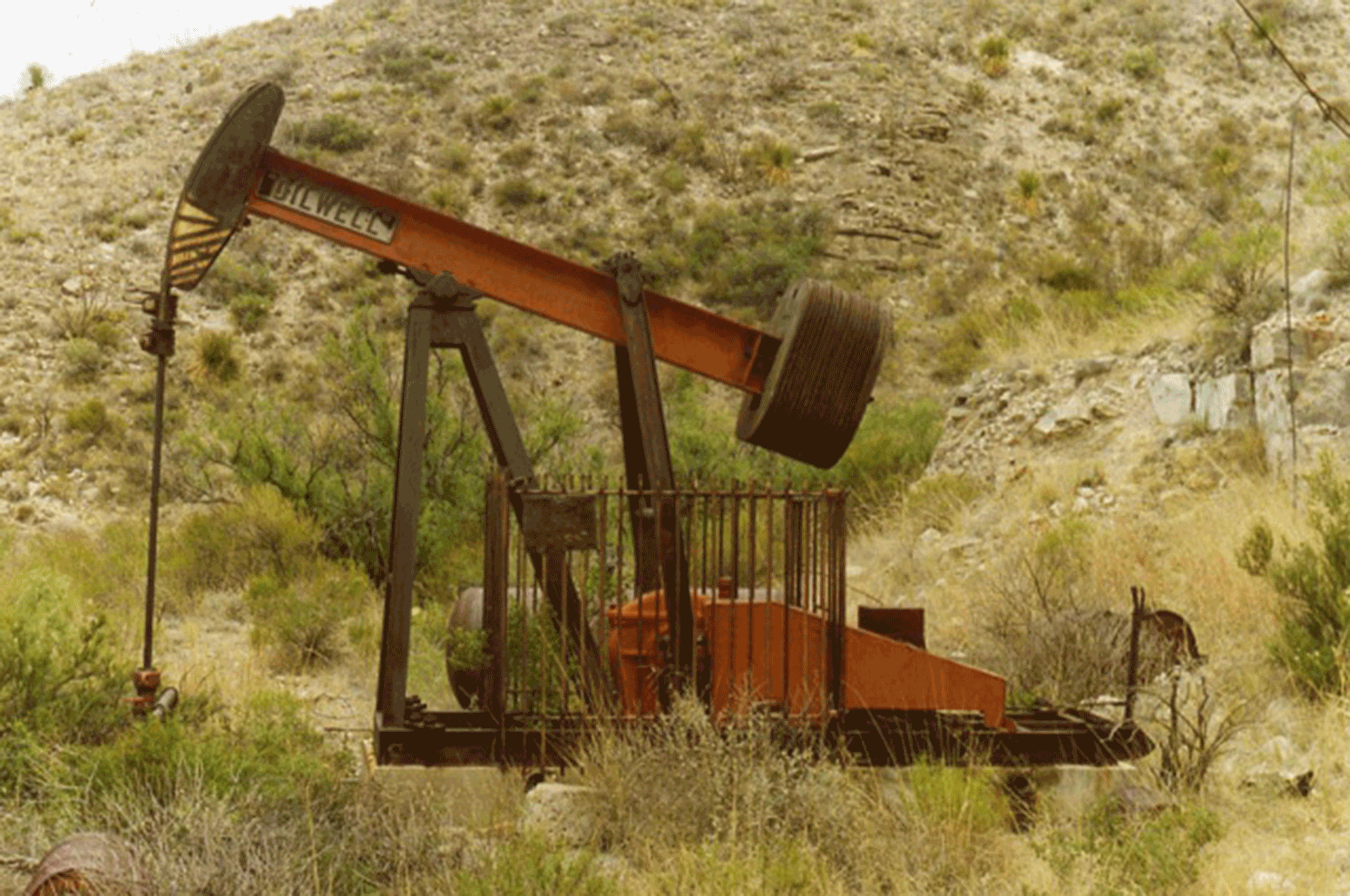
Abandoned pump-jack well in the Guadalupe Mountains National Park, Texas. Photograph by National Park Service.
Active and Abandoned Coal Mines
All coal mines release CH4 during mining, and the amount released depends on the geologic setting of the mine and operational and design factors. Gassy active underground coal mines emit more than 100,000 cubic feet of CH4 per day. The EPA reported that CH4 emissions from coal mining and abandoned coal mines accounted for about 7 percent of total U.S. CH4 emissions in 2021. Removal of CH4 from coal beds and capture of CH4 released during mining are effective methods to reduce emissions from active mines. With global trends in energy transitions from fossil fuels to renewable energy sources and the declining need for coal to produce steam, many coal mines are closing, with more likely to close. Thousands of closed coal mines are potentially leaking CH4 from surface deformations, boreholes, or mine-shaft seals (fig. 4). These mines that are leaking CH4 can be identified, and the best available research and assessment methodologies can be used to quantify and mitigate CH4 emissions with a particular focus on high-emission sources.
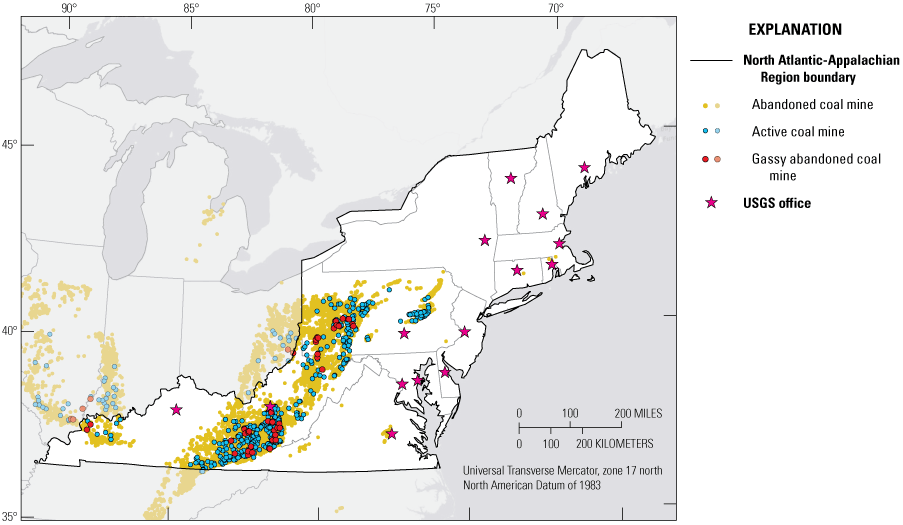
Map showing abandoned and active coal mines, and abandoned very gassy coal mines (underground mines that emitted more than 200,000 cubic feet of CH4 per day when they were active) in the U.S. Geological Survey (USGS) North Atlantic-Appalachian Region (NAAR) and surrounding areas; darker shaded colors are in the NAAR. USGS offices in the NAAR are also indicated on the map. Data sources: U.S. Environmental Protection Agency, 2017; Office of Surface Mining Reclamation and Enforcement, 2022; Mine Safety and Health Administration, 2022.
Unlike other greenhouse gases, CH4 can easily be converted to usable energy. Methane emissions from active and abandoned coal mines can be captured and utilized to alleviate both the global warming effects of CH4 and to create another energy sector in nearby communities where coal mining jobs may have been lost. Methane from active and abandoned coal mines can be viewed as a beneficial resource from what would otherwise be waste. Capture and utilization of CH4 that is complemented by research as well as inventory and forecast studies to minimize the GHG effect of these emissions can support of the U.S. Methane Emissions Reduction Action Plan (White House, 2021), and to take full advantage of their resource potential. The USGS is developing measurement strategies to better monitor and assess CH4 emissions at active and abandoned coal mines.
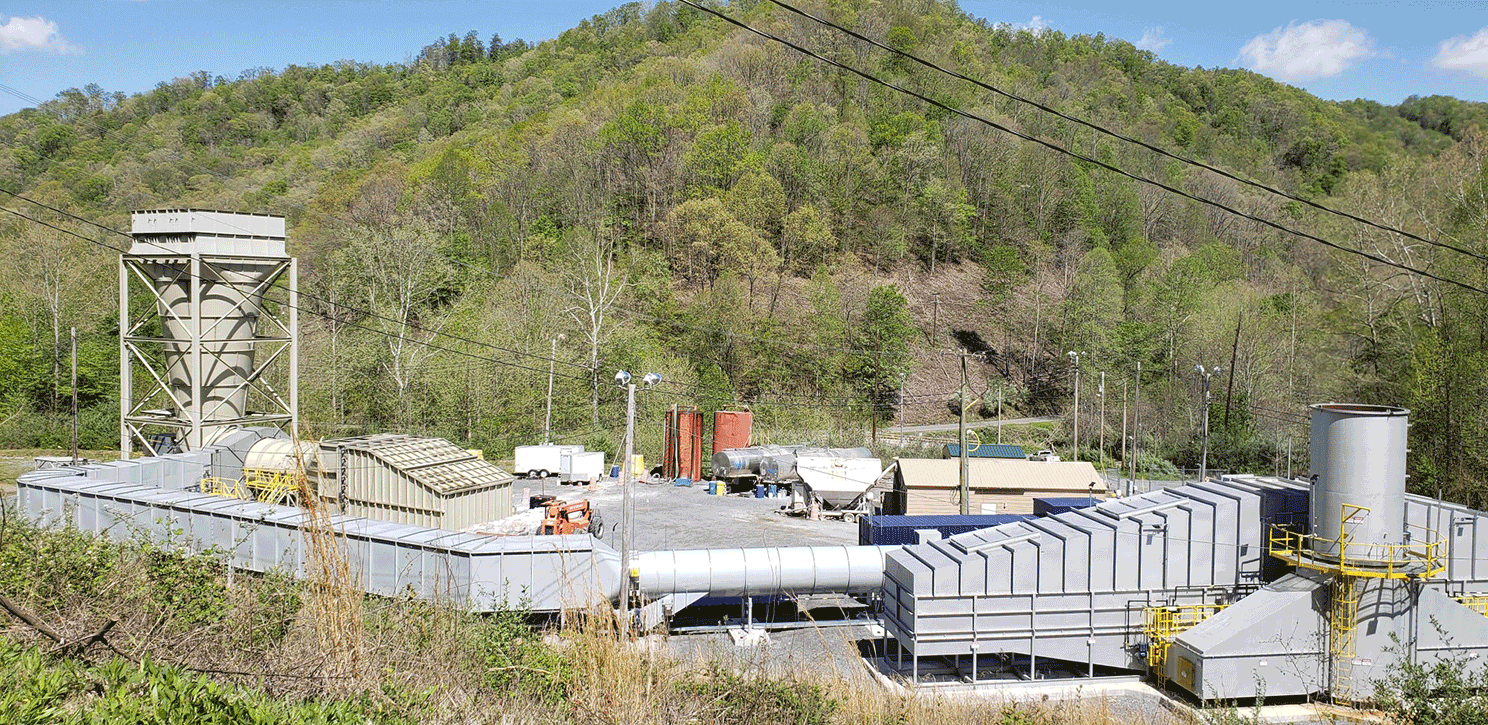
An underground coal mine, southwestern Virginia, ventilation exhaust fan and the methane oxidation unit. Photo by Özgen Karacan, U.S. Geological Survey.
Summary
This Fact Sheet provides an overview of existing USGS science information, ongoing and new investigations, or data-collection programs that identify areas in the northeastern region that may be suitable for enhanced carbon removal and sequestration to meet national atmospheric carbon reduction goals. This includes identifying potential sites for geologic carbon dioxide (CO2) storage, CO2 mineralization, enhanced mineral weathering in coastal environments, and methane mitigation opportunities at unplugged orphaned and abandoned oil and gas wells and active and abandoned coal mines in the northeastern region.
References Cited
Blondes, M.S., Merrill, M.D., Anderson, S.T., and DeVera, C.A., 2019, Carbon dioxide mineralization feasibility in the United States: U.S. Geological Survey Scientific Investigations Report 2018–5079, 29 p., accessed April 10, 2020, at https://doi.org/10.3133/sir20185079.
Blondes, M.S., Merrill, M.D., Anderson, S.T., DeVera, C.A., Agyepong, L.A., and Cahan, S.M., 2022, Geologic formations and mine locations for potential CO2 mineralization: U.S. Geological Survey data release, accessed February 24, 2023, at https://doi.org/10.5066/P9D92L53.
Grove, C.A., and Merrill, M.D., 2022, United States documented unplugged orphaned oil and gas well dataset: U.S. Geological Survey data release, accessed February 17, 2023, at https://doi.org/10.5066/P91PJETI.
Gupta, N., 2019, Mid-Atlantic U.S. offshore carbon storage resource assessment project—Final technical report: U.S. Department of Energy, National Energy Technology Laboratory report DOE-Battelle-FE26087, prepared by Battelle Memorial Institute, Columbus, Ohio, 776 p., accessed August 24, 2022, at https://www.osti.gov/biblio/1566748.
Kelemen, P., Benson, S.M., Pilorgé, H., Psarras, P., and Wilcox, J., 2019, An overview of the status and challenges of CO2 storage in minerals and geological formations: Frontiers in Climate, v. 1, article 9, 20 p., accessed March 7, 2023, at https://doi.org/10.3389/fclim.2019.00009.
Kroeger, K.D., Crooks, S., Moseman-Valtierra, S., and Tang, J., 2017, Restoring tides to reduce methane emissions as a new and potent Blue Carbon intervention: Scientific Reports 7, article 11914, 12 p., accessed March 14, 2023, at https://www.nature.com/articles/s41598-017-12138-4.
Merrill, M.D., Grove, C.A., Gianoutsos, N.J., and Freeman, P.A., 2023, Analysis of the United States documented unplugged orphaned oil and gas well dataset: U.S. Geological Survey Data Report 1167, 10 p., accessed February 17, 2023, at https://doi.org/10.3133/dr1167.
Mine Safety and Health Administration, 2022, Mine data retrieval system: U.S. Department of Labor, Mine Safety and Health Administration, online dataset, accessed September 21, 2022, at https://www.msha.gov/mine-data-retrieval-system.
Montserrat, F., Renforth, P., Hartmann, J., Leermakers, M., Knops, P., and Meysman, F.J.R., 2017, Olivine dissolution in seawater–implications for CO2 sequestration through enhanced weathering in coastal environments: Environmental Science & Technology, v. 51, no. 7, p. 3960–3972, accessed January 25, 2022, at https://doi.org/10.1021/acs.est.6b05942.
National Academies of Sciences, Engineering, and Medicine, 2022, A research strategy for ocean-based carbon dioxide removal and sequestration: Washington, D.C., The National Academies Press, 322 p., accessed February 24, 2023, at https://doi.org/10.17226/26278.
National Carbon Sequestration Database and Geographic Information System Map Team, 2015, NATCARB Sources 2013: U.S. Department of Energy, National Energy Technology Laboratory, online dataset, edition v1502, accessed September 16, 2022, at https://edx.netl.doe.gov/dataset/natcarb-alldata-v1502.
Office of Surface Mining Reclamation and Enforcement, 2022, Abandoned mine land inventory system, e.AMLIS: Office of Surface Mining Reclamation and Enforcement online database, accessed September 26, 2022, at https://www.osmre.gov/programs/e-amlis.
U.S. Environmental Protection Agency, 2017, Abandoned coal mine methane opportunities database: U.S. Environmental Protection Agency, EPA 430-R21-008, 22 p., accessed October 12, 2022, at https://www.epa.gov/sites/default/files/2016-03/documents/amm_opportunities_database.pdf.
U.S. Geological Survey Geologic Carbon Dioxide Storage Resources Assessment Team, 2013, National assessment of geologic carbon dioxide storage resources—Results (ver. 1.1, September 2013): U.S. Geological Survey Circular 1386, 41 p., accessed April 10, 2020, at https://pubs.usgs.gov/circ/1386/. [Supersedes version 1.0, released in June 2013.]
White House, 2021, U.S. methane emissions reduction action plan: White House Office of Domestic Climate Policy, 20 p., accessed January 11, 2022, at https://www.whitehouse.gov/wp-content/uploads/2021/11/US-Methane-Emissions-Reduction-Action-Plan-1.pdf.
For more information, please contact:
Center Director
Geology, Energy & Minerals Science Center
U.S. Geological Survey
12201 Sunrise Valley Drive
Reston, VA 20192
Telephone: 703-648-6543
https://www.usgs.gov/centers/geology-energy-and-minerals-science-center/connect
Publication support provided by the Reston Publishing Support Center
Edited by Sara A. Marcus
Design and layout by David Bruce
All photos by the U.S. Geological Survey except where noted.
Disclaimers
Any use of trade, firm, or product names is for descriptive purposes only and does not imply endorsement by the U.S. Government.
Although this information product, for the most part, is in the public domain, it also may contain copyrighted materials as noted in the text. Permission to reproduce copyrighted items must be secured from the copyright owner.
Suggested Citation
Warwick, P.D., Blondes, M.S., Brennan, S.T., Cahan, S.M., Karacan, C.Ö., Kroeger, K.D., and Merrill, M.D., 2023, Geologic carbon management options for the North Atlantic-Appalachian Region: U.S. Geological Survey Fact Sheet 2023–3038, 6 p., https://doi.org/10.3133/fs20233038.
ISSN: 2327-6932 (online)
Study Area
Publication type | Report |
---|---|
Publication Subtype | USGS Numbered Series |
Title | Geologic carbon management options for the North Atlantic-Appalachian Region |
Series title | Fact Sheet |
Series number | 2023-3038 |
DOI | 10.3133/fs20233038 |
Year Published | 2023 |
Language | English |
Publisher | U.S. Geological Survey |
Publisher location | Reston, VA |
Contributing office(s) | Geology, Energy & Minerals Science Center |
Description | 6 p. |
Country | United States |
State | Connecticut, Delaware, Maine, Maryland, Massachusetts, New Hampshire, New Jersey, New York, Pennsylvania, Rhode Island, Vermont, Virginia |
Online Only (Y/N) | Y |
Additional Online Files (Y/N) | N |
Google Analytic Metrics | Metrics page |