Effects of Noise from Oil and Gas Development on Raptors and Songbirds—A Science Synthesis to Inform National Environmental Policy Act Analyses
Links
- Document: Report (3.12 MB pdf) , HTML , XML
- Related Works:
- Structured Science Syntheses to Inform Decision Making on Federal Public Lands
- Effects of Noise from Oil and Gas Development on Ungulates and Small Mammals—A Science Synthesis to Inform National Environmental Policy Act Analyses
- Effects of Culverts on Habitat Connectivity in Streams—A Science Synthesis to Inform National Environmental Policy Act Analyses
- Download citation as: RIS | Dublin Core
Acknowledgments
We would like to thank Patrick Anderson, Renee Chi, Emma Dietrich, Kyle Ebenhoch, Jay Johnson, Sam Jordan, Megan McLachlan, Jennifer Meineke, Alexandra Stoneburner, Geoff Walsh, and Carmia Woolley for reviews of early drafts of this report. We also thank Elroy Masters, Claudia Mengelt, Daryl Ratajczak, and Janna Simonsen for their involvement in the conceptualization of this science synthesis and their support throughout the writing process for this report. We thank Tim Hammond for helping us understand many of the nuances of applying this science synthesis to management decisions. We are grateful to Erin Bayne and Jesse Barber for independent peer reviews of this work. Finally, we gratefully acknowledge Colorado State University and FORT Writes guided writing sessions hosted by Kristina Quynn and Kimi Conro for providing expertise, time, incentive, and accountability that aided in the writing process.
Logan M. Maxwell and Tait K. Rutherford contributed equally to this work.
Funding for this work was provided by the Bureau of Land Management National Operations Center. This science synthesis project was supported in part by the U.S. Geological Survey Ecosystems Mission Area, Land Management Research Program. Land Management Research Program scientists work with resource managers to provide science and data products to help Federal, State, and local agencies and Native American Tribes conserve, manage, and restore our Nation’s diverse socioecological systems, often focused on management of public lands and resources at landscape scales.
Executive Summary
Background: The U.S. Geological Survey is working with Federal land management agencies to develop a series of science synthesis reports. These reports synthesize science information to support environmental effects analyses that agencies perform in accordance with the National Environmental Policy Act (NEPA). This report focuses specifically on the effects of noise from oil and gas development on North American raptors, songbirds, and other small avian species (fig. 1).
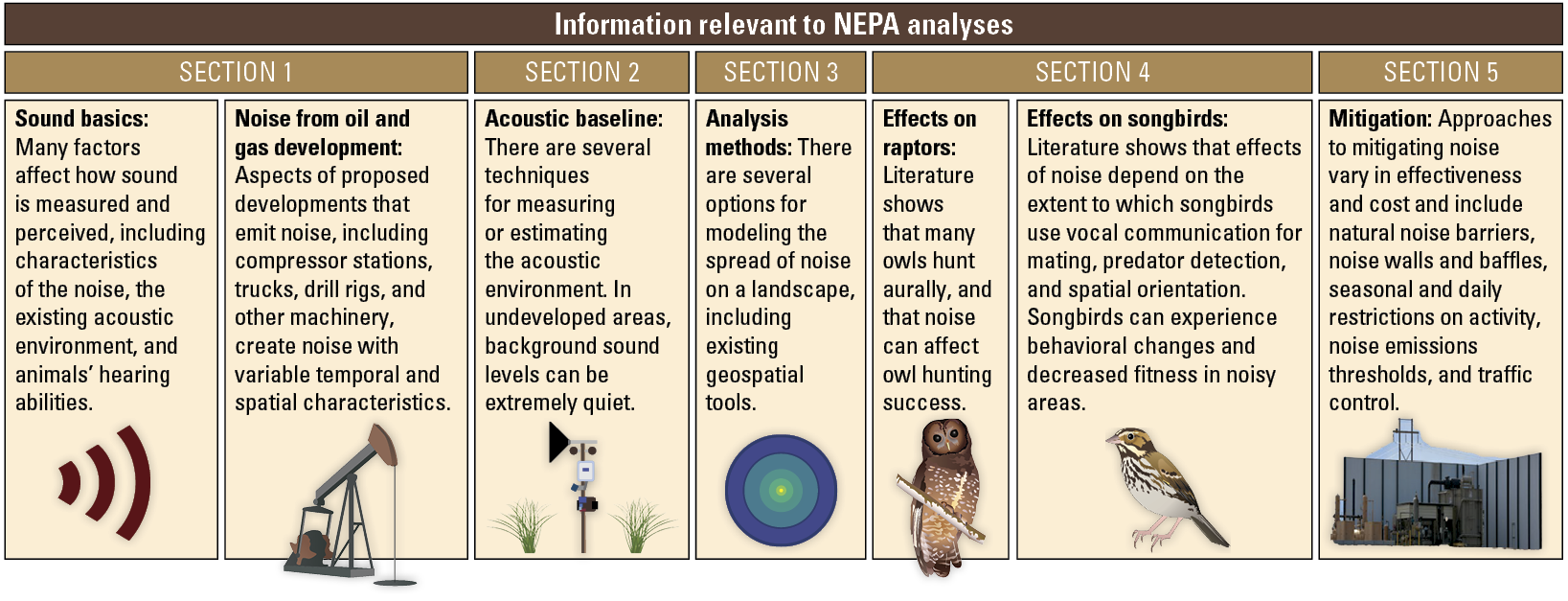
Summary of the information contained in this report by section. From left to right, illustration by Tracey Saxby, Integration and Application Network, licensed under a Creative Commons Attribution 4.0 ShareAlike License, https://ian.umces.edu/media-library/noise/; Image by Tracey Saxby, Integration and Application Network, licensed under a Creative Commons Attribution 4.0 ShareAlike License, https://ian.umces.edu/media-library/petroleum-industry-oil-rig/; Image by Lance McNew, U.S. Geological Survey, public domain, https://www.usgs.gov/media/images/acoustic-recording-device-seward-peninsula-tundra-Alaska; Image by Dylan Taillie, Integration and Application Network, licensed under a Creative Commons Attribution 4.0 ShareAlike License, https://ian.umces.edu/media-library/bouteloua-curtipendula-sideoats-grama/; Image by Tait Rutherford, U.S. Geological Survey; Image by Kim Kraeer and Lucy Van Essen-Fishman, Integration and Application Network, licensed under a Creative Commons Attribution 4.0 ShareAlike License, https://ian.umces.edu/media-library/strix-occidentalis-spotted-owl/; Image created by Kim Kraeer and Lucy Van Essen-Fishman, Integration and Application Network, licensed under a Creative Commons Attribution 4.0 ShareAlike License, https://ian.umces.edu/media-library/passerculus-sandwichensis-savannah-sparrow/; Photograph by Tait Rutherford, U.S. Geological Survey. NEPA, National Environmental Policy Act.
How this report can inform a NEPA analysis: We organized the sections of this report to align with standard elements of NEPA environmental effects analyses. The report synthesizes science information relevant to characterizing a proposed action and alternatives (section 1), characterizing the affected environment (section 2), identifying issues for analysis and potential environmental effects for each issue (sections 3 and 4), and mitigating potential adverse effects of the proposed action (section 5).
Noise produced by oil and gas development: Identifying the duration and extent of noise across the landscape is critical to understanding potential effects of proposed oil and gas activities on the landscape. Oil and gas development is a common source of human-caused noise on public lands. Human-caused noise in oil and gas fields comes from diverse sources such as heavy construction and drilling machinery, hydraulic fracturing, long-term production machinery, truck traffic, aircraft, and human activity. Noise from heavy equipment, machinery, trucks, and aircraft is generally intense across a broad spectrum of audible frequencies. Some types of infrastructure (for example, compressor stations that aid in transportation of natural gas) can produce sound 24 hours per day through the course of several years of production.
The perceived loudness of noise for an animal depends on existing ambient sound levels in the environment and the animal’s hearing sensitivity, which can vary by species and individual. For example, human development, weather such as wind or rain, and surface water, such as rivers, can elevate background sound levels. In addition, background sound levels may vary by season because changes in humidity and ground cover, including snow cover, can affect sound propagation. A decrease in background sound level would result in an increase in an animal’s perceived loudness of noise. Undeveloped landscapes can be extremely quiet, which allows an animal to hear a noise farther away from the source than in areas near cities, roads, or rivers. Common techniques for measuring the existing acoustic environment and estimating the acoustic environment without human-caused noises include field data collection using a sound level meter or inference from previously measured or published data.
Effects of noise from oil and gas development on raptors and songbirds: Research has found that human-caused noise can affect wildlife health and behavior. Noise has varied and largely negative effects at individual, population, and community levels across wildlife taxa, which highlights the potential for local and cross-species variation in noise effects. Few studies have focused on the effects of human-caused noise from oil and gas development on raptors, and those studies primarily focused on owls because they rely on hearing for hunting. Some studies in oil and gas fields have shown that noise did not affect owl occupancy or space use. However, other studies have documented that noise can impede owl hunting abilities, increase stress, and decrease reproductive success. In songbirds and other small avian species, several studies have documented effects of noise from oil and gas development on behavior, occupancy, community composition, distributions, and reproductive success—an indicator of fitness. Whether individuals are affected by noise from oil and gas development can vary by species and habitat system and is often dependent on the degree to which a species is able to adjust its calls to counteract the masking effects of the noise. Many studies also explored the combined effects of oil and gas infrastructure and noise on songbirds.
Mitigating the effects of noise from oil and gas development on raptors and songbirds: Techniques suggested in the scientific literature for reducing noise emissions or negative effects of noise emissions on birds include sound barriers, seasonal and daily timing restrictions, traffic control, and spatial considerations during project design. Siting infrastructure and wells to take advantage of natural sound barriers and reduce the footprint of road networks can be an effective tool for noise reduction. Many studies also explored the combined effects of oil and gas infrastructure and noise on songbirds.
Conclusion: This science synthesis can facilitate the use of science information in public lands decisions about oil and gas development. This report can be incorporated by reference in NEPA documents, cited as supplemental information, or used as a general reference for identifying literature or gaps in the available science about the effects of noise from oil and gas development on raptors, songbirds, and other small avian species.
Methods for developing this synthesis: Rutherford and others (2023) introduced a methodology for developing science syntheses to inform analyses conducted under the NEPA. Relevant introductory and methods text from that report is reproduced herein. This and other science syntheses build on the foundation and methodology developed in Rutherford and others (2023) and apply it to new topics of management concern on public lands in the Western United States. In addition, the content of this report overlaps the content of Rutherford and others (2023). Much of this report’s content not specific to raptors and songbirds is reproduced from Rutherford and others (2023) and includes updates and refinements as appropriate.
We used a structured search of recent scientific literature, supplemented by snowballing, to find published science about noise levels produced during oil and gas development, methods for analyzing sound propagation, the effects of noise on raptors and songbirds, and techniques to reduce noise emissions and their effects on raptors and songbirds. This report was coproduced by staff from the Bureau of Land Management, the U.S. Fish and Wildlife Service, and the U.S. Geological Survey.
Purpose of This Report
Federal land management agencies permit and plan for many uses and activities on public lands across the United States. Per the National Environmental Policy Act of 1969 (NEPA; 42 U.S.C. 4321 et seq.), Federal agencies must analyze and disclose potential environmental effects of major Federal actions that may significantly affect the quality of the human environment. Regulations for implementing the NEPA require “the integrated use of the natural and social sciences” in agency planning and decision making (40 CFR § 1501.2). Science is foundational to understanding how proposed Federal actions may affect natural resources, ecosystems, and human communities.
The purpose of this report is to synthesize science information relevant to environmental effects analyses for proposed oil and gas development activities on public lands. Science syntheses can be useful mechanisms for sharing science information with public land managers to inform their decisions (Seavy and Howell, 2010; Ryan and others, 2018). Science syntheses integrate knowledge and research findings to increase the generality, applicability, and accessibility of that information (Wyborn and others, 2018).
This report focuses specifically on the effects of noise from various aspects of onshore oil and gas development on North American raptors, songbirds, and other small avian species and identifies methods for characterizing noise and analyzing and mitigating its effects. Human-caused noise is widespread on public lands across the United States, and oil and gas development is a common source of that noise (Buxton and others, 2017). A substantial and growing body of research shows that human-caused noise can negatively affect wildlife health, behavior, population status, and fitness (Francis, 2015; Shannon and others, 2016; Dominoni and others, 2020; Sordello and others, 2020; Francis and others, 2023). Noise has been shown to have molecular-, physiological-, behavioral-, population-, and community-level effects across wildlife taxa (Kight and Swaddle, 2011), highlighting the importance of understanding the effects of noise on specific taxa and species (Blickley and Patricelli, 2010).
How to Use This Report
The content, structure, and section numbering of this report are designed to support NEPA analyses, and they reflect the steps of project planning and environmental effects analysis (table 1). This report is meant to be a general reference for considering and applying science information and could be used, for example, as follows:
-
• incorporated by reference in NEPA documents or to directly provide language for use in NEPA documents,
-
• included as supplemental information to a NEPA document, or
-
• used as a resource to gather literature and identify gaps in available science related to the management decision and context.
When incorporating by reference, drawing language, or citing information from this report, use the Maxwell and others (2024) suggested citation on page ii of this report.
To demonstrate a possible application of this report to a NEPA analysis, we developed a flowchart that illustrates generic methods for analyzing the potential effects of oil and gas development on raptors and songbirds and where to find the corresponding information in this report (fig. 2). Although the information in this report generally is organized stepwise as shown in the flowchart, the science synthesized in each section may inform multiple components of an analysis. In addition, the information can be applied iteratively, in a different order than the order presented in this report, or to aspects of project planning outside of the environmental effects analysis.
This report provides additional content that may be helpful for further exploring the topics discussed in the science synthesis. We describe in detail the methods used to conduct the literature search, including search terms, databases searched, and dates of the search. We also provide a glossary of technical terms, and all glossary terms appear in bold at first mention in the report. Appendixes 1 and 2 contain detailed compilations of quantitative study results about the effects of noise from oil and gas development on raptors and songbirds. The appendixes provide a reference for finding relevant studies and results by species or habitat community.
Table 1.
How the information in this report can inform steps in project planning and National Environmental Policy Act (NEPA) analysis.[Table modified from Rutherford and others (2023). Information about NEPA analysis is available in the Council on Environmental Quality NEPA regulations at 40 CFR 1500 et seq. and in department- and agency-specific policy and guidance]
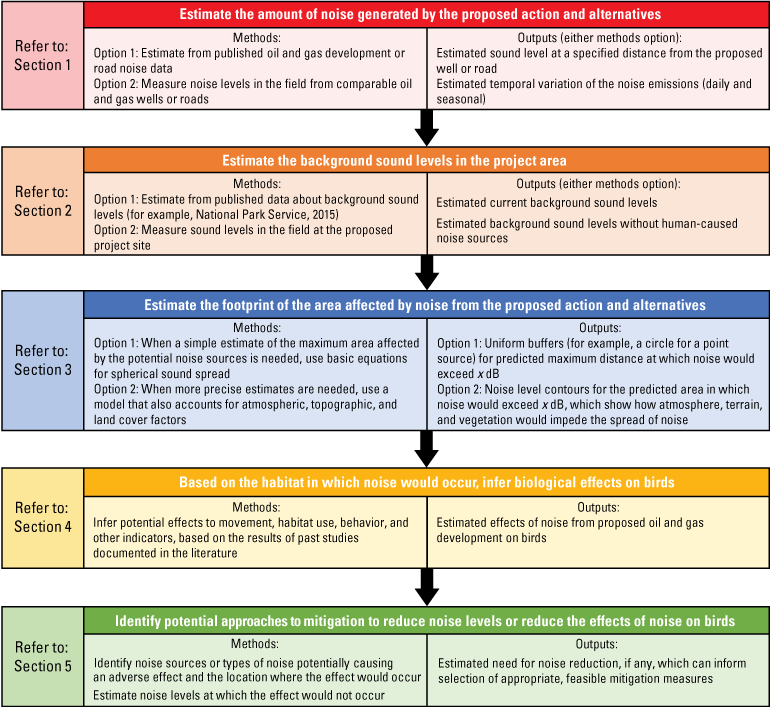
An example of potential methods in an analysis of the effects of noise from oil and gas development on raptors and songbirds with references to the sections of this report that synthesize science information relevant to each methods step. These steps could be iterative and revisiting steps or conducting preliminary analyses may be necessary. Modified from Rutherford and others (2023). dB, decibels.
Caveats to Use of This Report
This report is a science synthesis rather than a comprehensive literature review. In addition, this report does not provide all information necessary to complete a full environmental effects analysis or make conclusions regarding the significance of environmental effects. Resource planners and managers may need to supplement the information contained in this synthesis with local information. Information about specific design elements of the proposed project, local landscape conditions, and potential environmental effects from factors other than noise can complement the information contained in this synthesis.
This report focuses on data about noise and does not provide information about how to obtain wildlife data. Information and data about the distribution and status of local bird populations are crucial to understanding what species, how many individuals, and how much habitat may be affected by noise from proposed oil and gas development. In addition, this report focuses on onshore oil and gas development and does not address the effects of offshore oil and gas development on raptors and songbirds. Although the content of this report is focused on noise from onshore oil and gas development, we note that this report may be useful for other types of actions on public lands. Many actions that occur on public lands involve machinery, traffic, and human activity and may produce noise like the noise associated with oil and gas development activities. However, because of the specificity of the science synthesized in this report, we urge caution when generalizing its content to other types of actions.
Science Synthesis—Effects of Noise From Oil and Gas Development on Raptors and Songbirds
The following numbered sections are the science synthesis content of this report. The science synthesis sections are numbered to reflect their potential stepwise nature, as shown in figure 2, and facilitate internal referencing among sections. Much of this synthesis overlaps the content of Rutherford and others (2023), which addressed the effects of noise from oil and gas development on ungulates and small mammals. In particular, section “1. Characterizing Noise Caused by Oil and Gas Development,” section “2. Characterizing the Acoustic Environment,” section “3. Methods for Predicting Noise Occurrence on the Landscape,” and section “5. Mitigating the Effects of Noise from Oil and Gas Development on Raptors and Songbirds” largely address the same body of science as the corresponding sections of Rutherford and others (2023). Much of the content of this report is reproduced from Rutherford and others (2023) and includes updates and refinements as appropriate.
1. Characterizing Noise Caused by Oil and Gas Development
1.1. Sound Basics
Sound consists of vibrations occurring in a medium, such as air or water. Noise is an undesired sound. Sound data are generally reported using several standard metrics. Two common metrics for characterizing sound are sound level, also referred to as “intensity,” and sound frequency. For a particular receiver, such as an animal, changes in sound level within the receiver’s hearing capabilities are perceived as changes in loudness, and changes in frequency within the receiver’s hearing capabilities are perceived as changes in pitch. We describe sound level and frequency in more detail in the following sections, “1.1.1 Sound Level” and “1.1.2 Sound Frequency,” and we use these two metrics throughout this report to characterize sound.
1.1.1. Sound Level
Sound level is the intensity or amplitude of a sound and is commonly measured as either sound pressure or sound power.
-
• Sound pressure (the most common metric for intensity in the literature cited in this report) is a measure of change in pressure caused by sound waves. Sound waves attenuate with distance from a sound source. Therefore, sound pressure varies based on the spatial relation between a sound source and a sound receiver. Sound pressure level is measured and reported as the sound pressure at the location of a receiver. Sound pressure at a receiver varies through time as sound sources move or change. As a result, when reporting sound pressure levels, different statistics can be used to characterize different aspects of the acoustic environment through time at the receiver. Sound pressure level (L) statistics include the maximum sound pressure level (Lmax), minimum sound pressure level (Lmin), and logarithmic average of the sound pressure level (also known as the equivalent sound level [Leq]), during the timeframe of interest. In addition, studies often report the exceedance percentile (Lx), or the sound pressure level that was exceeded x percent of the timeframe of interest (Ambrose and Florian, 2014).
-
• Sound power (a less common metric for intensity in the literature cited in this report) is a measure of the sound energy produced by a sound source per unit of time. Sound power is fixed per sound source and unrelated to the position of a sound receiver.
The ability to perceive sound varies based on acoustic context and an animal’s particular hearing capabilities. Acoustic context affects the perceived loudness of a sound because loud existing sounds can mask a new sound of a similar or lower sound level, meaning the existing sounds raise the sound level threshold at which the new sound would be heard. In other words, an animal would generally perceive a specific sound level as louder in quiet environments and quieter in loud environments. For example, a whisper that sounds quiet inside a noisy building would be perceived as much louder in an undeveloped desert on a calm night. In addition, an animal’s ability to hear a sound that is greater than background sound levels and the perceived loudness of that sound (if they can hear it) varies among species because of evolved anatomic differences that limit species' hearing capabilities by frequency (Dooling, 1992; Heffner and others, 2001; Houser and others, 2017).
To help represent variations in sound perception, sound pressure level is often decibel weighted according to acoustic contexts and receiver capabilities (Houser and others, 2017). Weighting functions are mathematical equations that transform sound pressure level measurements to account for differences in auditory sensitivity among species or acoustic context (fig. 3; Houser and others, 2017). For example, one of the most common weighting functions to represent perceived loudness of sounds to average human hearing is A-weighting, which is measured in A-weighted decibels (dBA; Houser and others, 2017). For reference, a soft whisper is approximately 30 dBA when measured 1–5 meters (m) from the whisperer, and a chainsaw cutting wood at full throttle is greater than 110 dBA when measured less than 1 m from the chainsaw (refer to table 2 for additional examples; Berger and others, 2016). Sound measurements can also be weighted for other species with known hearing sensitivities (Pater and others, 2009; Houser and others, 2017). For example, weighting functions were developed for Strix occidentalis lucida (Mexican spotted owl; Delaney and others, 1999), Megascops asio (eastern screech owl; Brittan-Powell and others, 2005), and Leuconotopicus borealis (red-cockaded woodpecker; Delaney and others, 2011). Another standard weighting system developed for industrial contexts is C-weighted decibels (dBC). C-weighting favors lower frequencies to better represent human sensitivities to high sound pressure levels and is often used to measure sound from industrial sources and peak sound levels (Houser and others, 2017). Unweighted sound levels are sometimes reported as Z-weighted (dBZ), which is equivalent to unweighted dB between 10 and 20,000 hertz (Hz; Houser and others, 2017), or flat frequency response (dBF), for which the frequency range is undefined (Blickley and Patricelli, 2013). Conversion of sound measurements between decibel weighting systems is dependent on sound frequency (fig. 3). Because most studies report a single sound level, which is an integrated measure of sound levels from across the set of frequencies on which that sound has occurred (refer to section “1.1.2. Sound Frequency”; Engineering ToolBox, 2003), comparing observed sound level results across weighting systems is difficult. Therefore, in this science synthesis, we report sound level results as they were documented in the original study without converting between weighting systems.
Table 2.
Range of measured sound pressure levels of several common sound sources.[Table modified from Rutherford and others (2023). All data are summarized in Berger and others (2016). All reported sound pressure levels are A-weighted to represent perceived loudness to average human hearing and are the range of measurements reported in several studies. Duration of measurement may vary across studies and is not always reported in the original study (Berger and others, 2016); measurements listed in this table are assumed to represent average sound levels when the sound source is active (that is, from the start to the end of a whisper, while the refrigerator motor is running, while the air conditioner motor is running, and so forth). Perceived loudness of a sound level also depends on acoustic context, and quieter background sound levels result in an increase in perceived loudness. Refer to Berger and others (2016) for additional details and references to the original studies in which data were collected. dBA, A-weighted decibel; m, meter; ~, about]
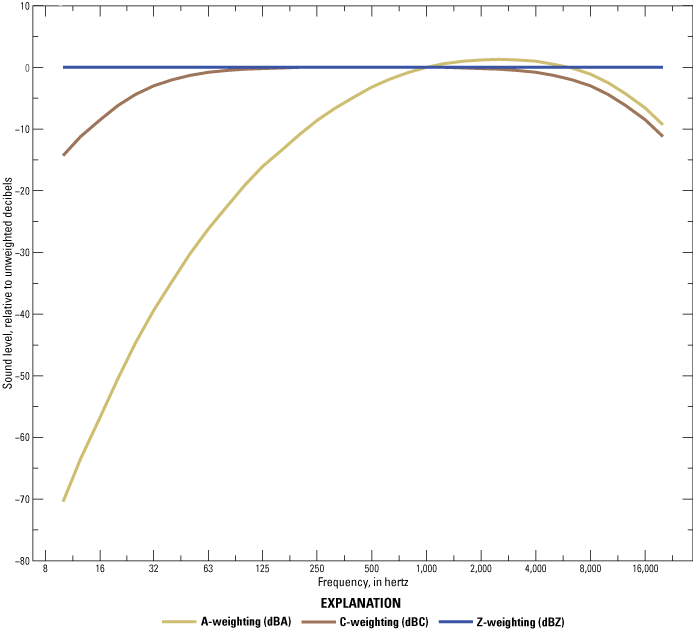
Difference between weighted and unweighted metrics for sound level (decibels [dB]) across frequencies 10–20,000 hertz (Hz). A-weighted decibels represent general perceived loudness of a sound to average human hearing. C-weighted decibels favor lower frequencies and are often used to represent perceived loudness to human hearing in industrial contexts that have typically high sound levels. Z-weighted decibels are equivalent to unweighted decibels from 10 to 20,000 Hz. Data from “American National Standards Institute/Acoustical Society of America S1.4-2014/Part 1/International Electrotechnical Commission 61672-1:2013” (Acoustical Society of America, 2014). Reproduced from Rutherford and others (2023).
1.1.2. Sound Frequency
Frequency is a measure of the wavelength of sound. Frequency is measured in hertz (Hz), and changes in frequency within an animal’s hearing capabilities are perceived as changes in the pitch of a sound. A pure tone is a sound that occurs at a single frequency (Acoustical Society of America, undated). Other sounds occur at multiple frequencies, which can range from a small set of harmonic frequencies, such as a note from a musical instrument, to a broad range of frequencies (also known as broadband), such as the sounds produced by equipment used during oil and gas development (Harrison and others, 1980). The distance sound travels and decays in different media varies by frequency. Lower frequency sounds attenuate less through the atmosphere and travel farther than higher frequency sounds (Naguib and Wiley, 2001; Pater and others, 2009).
Hearing capabilities for frequency differ among species and individuals within a species. Most terrestrial birds are most sensitive to a middle range of frequencies and become progressively less sensitive to lower and higher frequencies (Dooling, 1992; Dyson and others, 1998). This pattern is similar to human hearing, which can be used to illustrate the concept. Human hearing generally ranges from 20 to 20,000 Hz, and at the extreme ends of this range, humans can hear sounds only at high sound levels. For example, if a sound’s frequency is greater than approximately 18,000 Hz, a human with average hearing capabilities can only perceive that sound as audible if the sound level exceeds 70 dB. Conversely, humans can, on average, hear sounds between 2,000 and 4,000 Hz even when they occur at very low sound levels (less than approximately −6 dB; Jackson and others, 1999).
1.2. Characteristics of Noise From Oil and Gas Development
Sections 1.2 and 1.3 Highlights
• Noise during oil and gas development can range from short duration, such as a seismic blast or passing truck, to chronic, such as a compressor station.
• Environmental factors such as vegetation, snow, wind, temperature, humidity, and topography affect the distance noise can travel.
• Noise characteristics vary across phases of oil and gas development, including exploration, construction, drilling and completion, production, and surface reclamation.
• Noise sources include helicopters, trucks, human activity, cannons, compressors, generators, drills, and other machinery, which cause low-frequency noise that can travel long distances.
• The primary method for estimating noise from a proposed action and alternatives is inference from data collected around analogous past oil and gas development based on field measurements or published data.
1.2.1. Temporal Factors That Affect Noise From Oil and Gas Development
Sound can be characterized by the onset, consistency, and regularity of the sound source. The onset of sound can be rapid and startling (for example, a gunshot) or gradual (for example, a car passing; Francis and Barber, 2013; Gill and others, 2015). During oil and gas development, noise consistency can vary with the phase of oil and gas operations (refer to section “1.2.3. Noise During the Phases of Oil and Gas Development”). Chronic noises are typically generated during the production phase and include compressors and pumpjacks, which can run 24 hours per day (Francis and others, 2009). Chronic high amplitude infrastructure, such as compressor stations, can generate considerable noise and be the dominant noise source at a site. In an active oil and gas field in northern Alberta, Canada, chronic noise affected overall sound levels more than other noise sources such as traffic (Sánchez and others, 2022).
The temporal characteristics of sound can vary on short and long time scales. For example, intermittent noise can occur throughout development because of human presence, traffic, and machinery from exploration through surface reclamation. The overall noise footprint of oil and gas development can vary from year to year across the broad scale of oil and gas fields (in other words, the total geographic area above an oil or gas reservoir) as activity shifts geographically and sets of well pads move through phases of development and maintenance (Sawyer and others, 2017). In fields where such broader spatial patterns in development occur, sound could increase at the landscape scale in areas undergoing active drilling and construction, whereas areas containing sets of wells shifting to the production phase could become quieter. In addition, density of wells in an area can affect the temporal consistency of noise. For example, increased density of development could cause an increase in the frequency of truck travel and associated noise along access roads.
Noise may also vary temporally because background sound levels change with weather and seasons. Background sound levels can substantially affect the perceived loudness of noise from oil and gas development for an animal because greater differences between the sound level of the noise and the background sound levels result in greater perceived loudness. Weather, such as rain or wind, may temporarily cause increases in background sound levels, which would reduce the audibility of other sounds. Seasonal variation in factors such as snow cover, litter, soil moisture, and foliage can cause cyclical changes in background sound levels (Attenborough, 2002).
1.2.2. Spatial Factors That Affect Noise From Oil and Gas Development
Sound propagates through space away from a source. In an idealized scenario without sound barriers or atmosphere, sound would propagate spherically away from the source and attenuate according to the inverse square law, which roughly equates to a reduction of 6 dBA for every doubling of distance past approximately 15 m (Attenborough, 2002). However, environmental factors can act as sound barriers or reflectors, modifying patterns of sound attenuation (Francis and Barber, 2013). For example, the spread of sound through space can be affected by topographical features, vegetation structure, humidity, wind, temperature, soil moisture, and open water (Naguib and Wiley, 2001; Attenborough, 2002; Keyel and others, 2018; Boycott and others, 2019). Evidence from several studies illustrates sound attenuation based on environmental context:
-
• In forested areas, trees and ground litter generally increase the rate of sound attenuation (Naguib and Wiley, 2001; Attenborough, 2002). However, more nuanced effects can result from variation in forest vegetation structure and ground characteristics at small spatial scales (Boycott and others, 2019). For example, in an oil and gas field in northern Alberta, Canada, regardless of levels of industrial development, mature (40–100 years) boreal forest had the lowest sound levels compared with young (0–40 years) and old (100 years or older) forest at sites across the landscape (Sánchez and others, 2022).
-
• In open dryland ecosystems such as grasslands and Artemisia spp. (sagebrush) ecosystems, sound attenuation is primarily affected by atmospheric variables such as wind, which can mask low-frequency sound (Boycott and others, 2019). In a grassland study, noise from pipeline construction was greater than 65 dBA at the source and attenuated to 49 dBA at 250 m but remained above existing background sound levels (30–40 dBA) as much as 500 m from the source (Sutter and others, 2016). In a study in a sagebrush ecosystem, sound levels depended on distance to oil and gas activity, and landscape sound levels were not affected by human-caused noise at distances greater than 3,200 m from the source (Ambrose and others, 2021).
-
• In arctic habitats, frozen ground covered by a porous layer of snow can efficiently absorb sound, and strong winds can mask human-caused noises (Blix and Lentfer, 1992). In a study that measured noise from oil and gas exploration activities near simulated Ursus maritimus (polar bear) dens in Alaska, noise exceeded background levels within 100 m of the sound source, but strong wind prevented exceedance of background sound levels farther away (Blix and Lentfer, 1992). The porosity of snow changes how it absorbs or reflects sounds. Fresh snow is porous and absorbs more sound than dry ground, whereas snow that has a hard surface crust can reflect more sound than dry ground and increase sound spread (Attenborough, 2002).
In addition to environmental factors that affect sound attenuation and propagation, the location, movement, and concentration of sources can affect sound intensity across space. For example, because of frequent traffic during construction and well development, intense noise can be generated at a well pad and along access roads (Hays and others, 2017). At the scale of an oil and gas field, activity can concentrate in parts of the field as sets of wells progress through the phases of development (Walker and others, 2020). In areas that have higher densities of wells, noise likely increases in spatial consistency. Concentration or dispersion of individual noise sources can affect the total sound in an area because spatial and temporal overlap of sounds can cause aggregate effects (Francis and Barber, 2013; Gill and others, 2015; Sánchez and others, 2022). For example, when two broadband sound sources of equal sound level overlap, the total sound level is approximately 3 dB greater than either of the individual sound sources alone (Engineering ToolBox, 2003). Each additional sound source of equal sound level would add progressively less to the total sound level; 10 sound sources of equal sound level would be 10 dB greater than any of the individual sound sources alone (Engineering ToolBox, 2003). When a noise source produces much higher sound pressure level than other noise sources, such as chronic noise from a natural gas compressor station, noise from the other noise sources is minimally additive to the sound levels in the area and the most intense noise source is dominant (Engineering ToolBox, 2003; Sánchez and others, 2022). With a difference of 6 dB or greater between the sound pressure levels of two sound sources, the total sound pressure level is less than 1 dB greater than the independent sound pressure level of the more intense sound source (Engineering ToolBox, 2003).
1.2.3. Noise During the Phases of Oil and Gas Development
The phases of onshore oil and gas development consist of exploration, construction, drilling and completion, production, and surface reclamation. Each phase involves a broad set of noise sources ranging from human presence to industrial machinery.
Most studies that characterize noise from oil and gas development have focused on measuring aggregate sound occurring at a location during construction, drilling and completion, or production. Fewer studies have documented noise during oil and gas exploration and reclamation. Construction, drilling and completion, and production can last many years at a single well location. Each of these development phases use machinery and vehicles that produce high amplitude noise across frequencies (Scobie and others, 2016; Radtke and others, 2017). This section of the report synthesizes information from studies that have measured sound level and frequency near oil and gas wells. Table 3 provides a detailed list of sound levels observed during past studies.
Table 3.
Observed sound levels, type of sound source, and distance from sound source for noise emitted during different phases of oil and gas development.[Table modified from Rutherford and others (2023). This table presents a selection of sound sources based on information available in the literature and is not inclusive of all sound sources or types of oil and gas development. Sound levels are presented as they were measured and weighted in the original study. Reported sound levels may consist of single locations or averages across several locations, and we refer the reader to the original study for further information about the site-specific and landscape context in which these sound levels were observed, including vegetation or other incidental or intentional barriers to sound propagation. Additional noise data summarized from unpublished reports are available in Hays and others (2017). m, meter; dBA, A-weighted decibel; min, minute; Leq, equivalent sound level; dBC, C-weighted decibel; h, hour; mo, month; L50, median sound pressure level; s, second; dBZ, Z-weighted decibel; Lx, exceedance percentile; dB, decibel; avg., average; <, less than; >, greater than; SEL, sound exposure level]
Although identifying sound levels from specific phases and particular sources associated with each phase is important to characterizing noise from a proposed action, oil and gas development may occur in fields that have high densities of wells in various phases of development. In higher density fields, noise from wells is likely cumulative because of the spatial and temporal overlap of noise from individual wells and roads. In addition, the phases of development are commonly cyclical at a well pad. Multiple iterations of drilling, production, and interim reclamation can occur through time at a single location.
Exploration
Exploration involves several stages of geophysical study to identify probable locations for oil and gas deposits, followed by seismic survey and drilling of exploration wells. During the exploration phase, noise is typically intermittent and may be produced by helicopters, truck traffic, and detonation machinery, such as cannons or air guns, which are used for seismic testing (Brittingham and others, 2014). Noise from seismic vibrators is greatest at low frequencies, typically below 105 Hz (Bagaini and others, 2014). In a simulation of seismic testing noise, Bradshaw and others (1997) used a propane cannon to produce noise at amplitudes ranging from 90 to 110 dB at 2 m from the cannon mouth.
Construction, Drilling, and Completion
The construction and drilling and completion phases cover a wide variety of activities. Well pad and pipeline construction may last months to years and involve heavy machinery, road construction, and increased traffic. During drilling, a drill rig and drilling machinery produce noise while creating a borehole. Common intermediate steps associated with drilling and completion include hydraulic fracturing and well testing, which can involve flaring.
Hydraulic fracturing is a postdrilling technique to fracture low-permeability formations at the bottom of a borehole to obtain oil and gas contained in rock (Tiemann and Vann, 2015). Hydraulic fracturing typically has a higher sound pressure level than the drilling process because the fracturing of the rock itself can generate intense noises. In addition, horizontal drilling for hydraulic fracturing can last longer than traditional vertical drilling (Hays and others, 2017; Radtke and others, 2017). Hydraulic fracturing also requires higher volumes of truck traffic than other phases because of the continuous need for materials used during the fracturing process. Allshouse and others (2019) observed approximately 9 semitrailer trucks per hour, 24 hours per day during hydraulic fracturing activities, and an additional 8 pickup trucks per hour during the daytime.
Flaring is a noise-producing process step that occurs in some locations during well testing, after hydraulic fracturing, and can last days to weeks. Flaring can also occur during maintenance and to prevent hazards from overpressurized equipment (Cushing and others, 2020). Noise levels from flaring result from combustion of gases and depend on the volume of gas burning per unit of time; higher rates of flaring result in higher noise levels (Dowling and Mahmoudi, 2015).
Well completion can involve various techniques of preparing a borehole for production, such as inserting production tubing, casing, and any necessary downhole tools. Flowback is a part of well completion during which hydraulic fracturing fluids mixed with oil and gas flow out at high rates prior to production. Traffic volume can also be high during flowback activities (Massachusetts Institute of Technology, 2011). Allshouse and others (2019) observed 13.5 pickup trucks per hour during the daytime during flowback.
Like other stages of oil and gas development, most of the sound produced during construction, drilling, hydraulic fracturing, and well completion is high intensity and broadband and most intense at lower frequencies. During oil pipeline construction in Canada, noise was broadband (6.3–20,000 Hz) and remained higher than background sound levels as much as 1 kilometer (km) from the pipeline (Sutter and others, 2016). In a Colorado study, continuous noise from construction and drilling at sites that had 9.8-m sound barrier walls led to an increase of 8.7–17.4 dBA over background sound levels measured 320–550 m from the source (Blair and others, 2018a). Noise varied throughout the day; noise increased in the morning, decreased midday, and increased again in the afternoon (Blair and others, 2018a). In another Colorado study, the dominant frequency during drilling was 63 Hz, whereas the dominant frequency during hydraulic fracturing and completion was 125 Hz (Radtke and others, 2017). Sound pressure levels can vary depending on the types of machinery used during each development phase and the landscape around the well site. For example, Radtke and others (2017) documented that completion produced slightly lower sound pressure levels on average than drilling and hydraulic fracturing, whereas a different study found that flowback during completion produced higher sound pressure levels than drilling, hydraulic fracturing, and production (table 3; Allshouse and others, 2019). These differences indicate the importance of considering site and action-specific factors when estimating noise from a proposed action and alternatives (refer to section “1.3. Guidance for Estimating Potential Noise from Proposed Oil and Gas Development”).
Production
During production, noise may originate from compressors, generators, engines, pumpjacks, screw pumps, and other large equipment that operate continuously for several years. Acute or shorter-term noises, like many of the noises produced during drilling and completion, can also occur because of activities such as maintenance traffic or the need for a workover rig to replace completions when downhole problems occur. The types of production equipment in a field may vary based on the type of well and the amount of processing that occurs in that field. For example, fields that include gas production may more often have noisy equipment associated with initial gas processing and transportation, such as compressor stations. Compressors and generator-powered well pumps (for example, pumpjacks and screw pumps) continuously produce sound at high amplitudes across frequencies and produce higher sound pressure at low frequencies (fig. 4; Koper and others, 2016; Warrington and others, 2018). Grid-powered pumpjacks and screw pumps are generally quieter than their generator-powered counterparts (table 3; Koper and others, 2016). In a Colorado study, sound pressure level observations at production sites were at least 15 dBA lower than at drilling, hydraulic fracturing, and completion sites (table 3), and dominant frequencies were very low (16–31.5 Hz; Radtke and others, 2017).
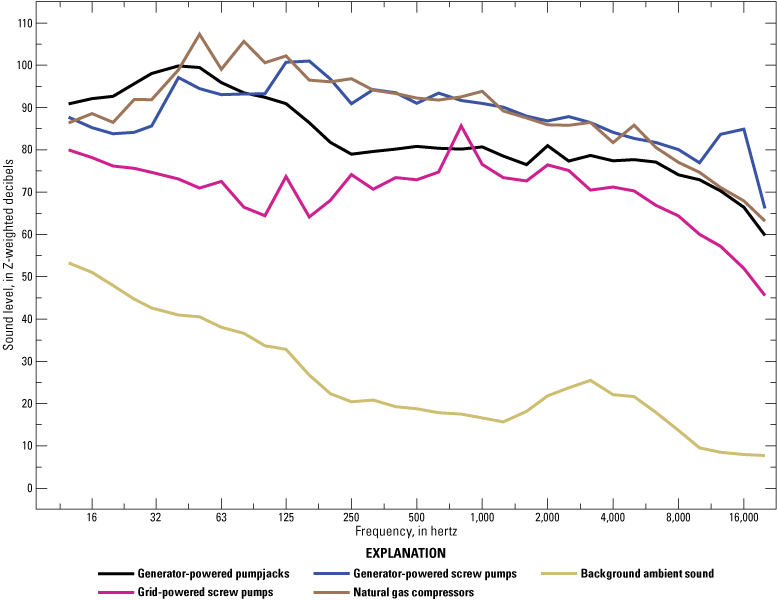
Average unweighted sound pressure levels (Z-weighted decibels [dBZ]) at frequencies of 12.5–20,000 hertz (Hz) for generator-powered pumpjacks (sample size [n] equals [=] 4), generator-powered screw pumps (n=5), grid-powered screw pumps (n=5), natural gas compressor stations (n=4), and background sound levels in an oil and gas field in mixed-grass prairie in southern Alberta, Canada. All measurements for infrastructure were taken less than 10 meters from the sound source. Background sound was recorded at control sites (n=41), which included sites without infrastructure and inactive infrastructure sites. Measurements were taken in each 1/3-octave frequency band and averaged across sites. This figure represents sound levels from production equipment in a particular study system; sound levels can vary by environmental context and equipment and well type, among other variables. Data from Warrington and others (2018; used with permission of Oxford University Press). Modified from Warrington and others (2018) and Rutherford and others (2023).
Traffic during the production phase is typically lower than during construction and drilling and completion because traffic is limited to intermittent machinery maintenance (Zammerilli and others, 2014). Allshouse and others (2019) observed an average of 1 semitrailer truck and 1 pickup truck per hour during the daytime at a well in the production phase in Colorado.
Reclamation
Surface reclamation is a set of activities intended to initiate ecosystem processes that will eventually return a site to its predisturbance ecosystem function. Reclamation generally involves recontouring and revegetating roads and well pads, which can entail various noise producing activities, including use of heavy machinery to move soil and prepare it for planting. Interim reclamation can begin as soon as well completion is finalized, which means noise from interim reclamation can be concurrent with noise from production. Final reclamation occurs after production has closed and the well is plugged and abandoned (U.S. Department of the Interior and U.S. Department of Agriculture, 2007). We did not find literature measuring noise specific to reclamation or well abandonment activities; however, Sutter and others (2016) reported lumped measurements from construction and reclamation activities at an oil pipeline in Canada (table 3).
Traffic Noise (All Phases)
Traffic noise occurs throughout each phase of oil and gas development and varies because of differences in traffic volume and vehicle type among phases (Radtke and others, 2017; Allshouse and others, 2019). Traffic noise along a specific road can be highly varied depending on the type of vehicle, road gradient, road surface, vehicle speed, and traffic volume (Byrnes and others, 2012). For example, paved roads may generally have higher vehicle speeds and greater traffic volume than unpaved roads, although these factors can be location and situation specific. Chen and Koprowski (2015) measured how vehicle speed affects noise levels and found that driving at 24 kilometers per hour (km/h) resulted in 1 dBA lower Leq and 1.5 dBA lower Lmax than driving at 40 km/h. An average well may have more than 1,100 heavy-truck trips for servicing the well during its lifetime (Zammerilli and others, 2014). Most traffic occurs during construction, drilling, and completion and decreases during production to infrequent traffic for maintenance of the well (Zammerilli and others, 2014). Traffic unrelated to oil and gas activity can also be common and presents potential cumulative noise effects when combined with oil and gas-related traffic (Scobie and others, 2016).
1.3. Guidance for Estimating Potential Noise from Proposed Oil and Gas Development
Noise from proposed oil and gas development can be estimated based on published data from past similar actions or gathered in the field from ongoing similar actions. Because of the variability of atmospheric, vegetation, and topographic characteristics across study systems, published data can provide a wide range of sound level estimates (for example, table 3). Therefore, measuring sound levels near other oil and gas development in the project or planning area typically is a more accurate method for estimating noise from a proposed action and alternatives. The National Park Service (NPS; NPS, 2013a) provided a step-by-step technical guide for acoustic monitoring. Blickley and Patricelli (2013) also provided technical recommendations for accurate sound measurements in the field. Types of equipment, timing and location of measurements, and the presence of unexpected, nontarget sounds are all important technical considerations when gathering field data (Blickley and Patricelli, 2013; NPS, 2013a). Blickley and Patricelli (2013) recommended specialized technical training for all staff collecting field sound measurements. A sound level meter is the most common equipment used for measuring sound in the field. Recommendations for calibrating and positioning sound level meters, excluding interference, and selecting timeframes for measurement are available in Blickley and Patricelli (2013), NPS (2013a), Patricelli and others (2013), and Ambrose and Florian (2014). In general, selection of location and timing of measurements can be informed by physical characteristics or life history traits of the potentially affected animal species (Blickley and Patricelli, 2013). Location and timing of measurements can also vary by management goal, type of noise, or type of anticipated effects, such as whether animals are expected to flush in response to acute noise or experience increased stress in a chronically noisy location (Blickley and Patricelli, 2013).
In the absence of field data, published data can inform characterization of noise expected from a proposed action and alternatives. Published data appear in gray literature and peer-reviewed studies (table 3). In addition, traffic data in existing oil and gas fields can be gathered from State and national public traffic databases or from existing publications (for example, Zammerilli and others, 2014; Allshouse and others, 2019).
2. Characterizing the Acoustic Environment
Section 2 Highlights
• Researchers recommend considering field data and previously published data when characterizing the acoustic environment.
• Common sound measurement techniques can help to identify the relative contributions of natural and human-caused sounds to the acoustic environment.
• Several previously published resources provide information about background sound levels in undeveloped landscapes in the United States.
The acoustic environment consists of all the sounds at a location. The acoustic environment varies across a landscape (spatially) and through time (temporally). Characteristics of the acoustic environment can provide a reference condition for measuring potential noise effects of a proposed action and alternatives. Data about the acoustic environment can be gathered at the site of a proposed project or extrapolated from publicly available datasets or other published information. Blickley and Patricelli (2013) recommended considering previously published data in addition to new field data, when possible, because of the difficulty of measuring sound levels in very quiet environments and identifying natural sound levels in areas already greatly affected by human-caused noise. Equipment, temporal and spatial factors, and unexpected sounds are all important considerations when gathering field data to describe the acoustic environment (Blickley and Patricelli, 2013; NPS, 2013a). Use of sensitive sound measurement equipment is especially important when measuring background sound levels; many sound level meters (those meters that have a rating of class 2 [refer to Acoustical Society of America, 2014]) cannot measure sound levels quieter than 35 dBA, which exceeds background sound levels in most undeveloped landscapes (Blickley and Patricelli, 2013). Mismeasurement of background sound levels can result in underestimation of the spatial extent of the audibility of a noise and have implications for effects analysis (refer to section “3. Methods for Predicting Noise Occurrence on the Landscape”) and noise management practices such as exceedance thresholds (refer to section “5. Mitigating the Effects of Noise From Oil and Gas Development on Raptors and Songbirds”). Section “1.3. Guidance for Estimating Potential Noise from Proposed Oil and Gas Development” contains an additional description of resources containing guidelines for gathering acoustic data.
In many landscapes, natural sound sources (for example, wind, bird songs, and rivers) and human-caused sound sources contribute to the acoustic environment (Harrison and others, 1980; Gomes and others, 2021). Common sound measurement techniques can be used to identify the relative contributions of different types of sounds to the acoustic environment and facilitate analysis of potential cumulative effects (that is, the effects of each alternative considered in combination with the effects of existing human-caused noise sources). A standard metric for characterizing the contributions of natural sounds to the acoustic environment is the sound pressure level that is exceeded 90 percent of the time (L90; Ambrose and Florian, 2014). This metric represents consistent, low intensity sounds and does not capture infrequent, short-duration sounds such as occasional vehicles passing on low-traffic roads, which makes L90 useful for characterizing background sound levels in undeveloped areas (Ambrose and Florian, 2014). To characterize the total acoustic environment, including existing human-caused sounds, Ambrose and others (2021) recommended using L50 or Leq, which capture the median and logarithmic average of sound measurements.
Previously published data and models about background sound levels can provide a reference point to supplement local field data, which can be useful because of the difficulty of accurately measuring background sound levels (Blickley and Patricelli, 2013). Modeled background sound levels for the United States are available from the NPS (2015). The model uses field-collected ambient sound data from NPS units from 2000 to 2011 to estimate the contributions of human-caused sound to background sound levels nationwide at a 270-m resolution (Mennitt and others, 2014). In addition to this model, observational data from past studies can be used to infer background sound levels in particular landscapes. Most published observations for largely undisturbed environments report background sound levels less than 30 dBA. For example, Lynch and others (2011) measured median L90 across 43 national parks in the United States at 21.8 dBA. In desert areas in southern California that were usually undisturbed by human-caused noises, 44 percent of daily sound measurements were between 20.5 and 25 dBA (Brattstrom and Bondello, 1983). Patricelli and others (2013) recommended using 16–20 dBA as the background acoustic environment for undeveloped sagebrush ecosystems. However, correcting for sound level meter self noise, Ambrose and others (2021) measured nighttime sagebrush sites as somewhat quieter: average Leq of 24 dBA, L50 of 11 dBA, and L90 of 8 dBA. Across the full 24 hours of measurements, they reported an average Leq of 26 dBA, L50 of 19 dBA, and L90 of 14 dBA (Ambrose and others, 2021).
3. Methods for Predicting Noise Occurrence on the Landscape
Section 3 Highlights
• Sound propagation modeling is a method for estimating where sound from a proposed action will occur on the landscape.
• Sound propagation models depend on three basic types of data: (1) sound level of the noise source, (2) sound level of the acoustic environment, and (3) environmental variables that affect the spread of sound.
• One simple option for estimating how far a noise could propagate is to consider only spherical sound spread, which can give a rough estimate of the maximum distance a sound will travel. This option is less complex and time intensive but also less precise.
• A second option is to use a geospatial sound modeling tool. This option is more precise but also more complex and time intensive.
• The results of sound propagation modeling can be superimposed on wildlife habitat or occurrence data to predict the area in which individuals and populations may experience effects from noise.
3.1. Overview of Sound Propagation Modeling and Data Needs
An estimate of where and when noise will occur in a species’ habitat can provide the basis for assessing the effects of that noise on the species. A key method for estimating the locations in which noise from a proposed oil and gas development action will be audible is sound propagation modeling. Fundamentally, a sound propagation model shows how far a sound will travel based on the sound level at the source and environmental barriers that impede the spread of the sound. Spread and impedance are based on standardized equations for calculating the movement of sound waves (International Organization for Standardization, 1996). In addition, a sound propagation model accounts for the background acoustic environment because a noise is generally audible when it has a greater sound pressure level than ambient sound levels. Thus, a sound propagation model relies on three basic types of data:
-
• Sound level of the noise source (refer to sections “1.2. Characteristics of Noise From Oil and Gas Development” and “1.3. Guidance for Estimating Potential Noise from Proposed Oil and Gas Development” for information about gathering or estimating these data). An estimate of the sound level at the source will likely vary through time (refer to section “1.2.1. Temporal Factors that Affect Noise From Oil and Gas Development”), and the estimate should be based on relevant periods for the species (for example, maximum potential sound level during the nesting season).
-
• Sound level of the acoustic environment (refer to section “2. Characterizing the Acoustic Environment” for information about gathering or estimating these data). Noise levels must be compared to background sound levels to determine where the noise will be audible.
-
• Environmental factors including temperature, humidity, wind, elevation, topography, vegetation and ground cover, soil moisture, open water, and any other barriers (for example, a building or wall) that affect the spread of sound (refer to section “3.2. Options for Estimating Sound Propagation” for information about gathering and using these data). Because environmental factors generally impede the spread of sound, models that incorporate more variables typically output a smaller estimated noise footprint (fig. 5A, B, C).
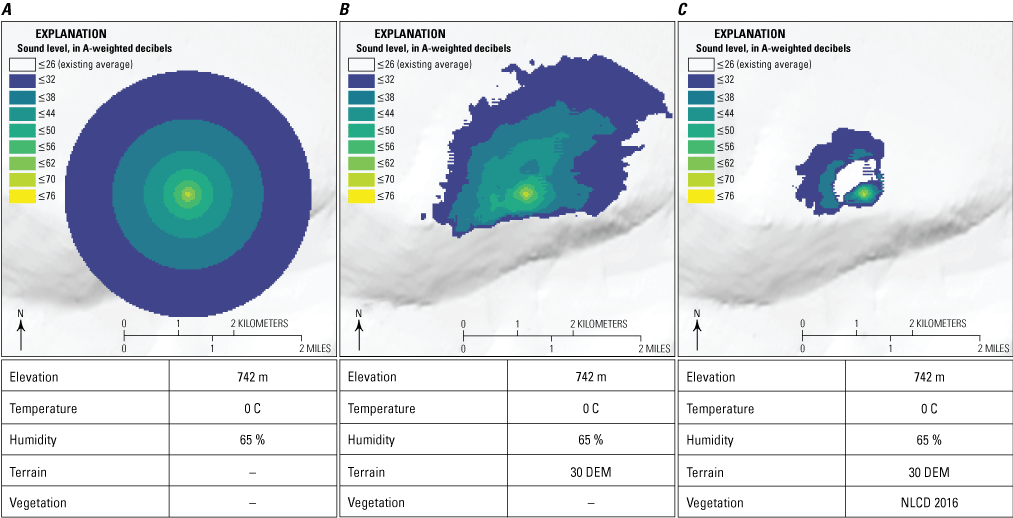
Differences in the outputs of the Noise Model Simulation (NMSim) sound propagation model (Ikelheimer and Plotkin, 2005) for a hypothetical illustrative scenario. Each panel shows a model output that includes progressively more impedance variables. A, model includes impedance from atmospheric variables and does not include impedance from terrain (digital elevation model [DEM], available at https://www.usgs.gov/programs/national-geospatial-program/national-map) and land cover (National Land Cover Database [NLCD], available at https://www.mrlc.gov/); B, model includes impedance from atmospheric variables and terrain and does not include impedance from land cover; C, model includes impedance from atmospheric variables, terrain, and land cover. The noise source is a hypothetical stationary, heavy truck that produces sound at 71 A-weighted decibels (dBA) measured 15 meters (m) from the truck. The color gradients in each panel indicate the area in which the noise is perceived at different sound levels. A downhill slope in terrain causes sound to travel somewhat farther to the northeast in panel B than on flat terrain in panel A, and an uphill slope impedes sound travel to the south. Inclusion of land cover in panel C substantially reduces the distance sound travels in all directions. We produced all images using the NMSim model within the Sound Mapping Tools package (Keyel and others, 2017) in ArcGIS Pro (Esri) and RStudio (Posit). Modified from Rutherford and others (2023). ≤, less than or equal to; m, meter; C, degrees Celsius; %, percent; —, no data.
The output of a sound propagation model is an estimate of the spatial extent of the noise at various sound levels, also known as sound level contours. The estimated spatial extent can be superimposed on habitat and species distribution data to quantify amounts of habitat and numbers of individuals potentially affected and identify locations that may exceed thresholds for effects, if known (refer to Ambrose and others [2021] for an example of an effect threshold). Information about the amount of habitat or number of animals affected by noise can help infer effects on indicators for individual or population health, such as physiology, behavior, abundance, or fitness, based on past studies described in the literature (refer to section “4. Potential Effects of Noise From Oil and Gas Development on Raptors and Songbirds”).
3.2. Options for Estimating Sound Propagation
We outline two options for estimating sound propagation in sections “3.2.1. Option 1—Estimate Sound Propagation with Basic Equations for Spherical Spread of Sound” and “3.2.2. Option 2—Geospatial Sound Propagation Modeling Tools,” which differ based on complexity and precision. Although complex models for sound propagation can yield more precise results by incorporating environmental variables that impede sound spread (fig. 5C), simpler approaches are often more practical. The first option provides a method to roughly estimate maximum sound level contours around a stationary point noise source. This method relies on an accurate estimate of sound levels at a reference distance close to the noise source. This first option does not incorporate environmental variables and is based on general physical principles for the way sound waves attenuate spherically from a stationary point source with distance. This option is easily calculated but does not offer precise outputs and should be used with caution.
The second option consists of using geospatial modeling tools that incorporate atmospheric, topographic, and land cover factors to provide more precise estimates of sound propagation. This option requires more skills and time to prepare data, learn models, and run models using geographic information systems (GIS) software.
3.2.1. Option 1—Estimate Sound Propagation with Basic Equations for Spherical Spread of Sound
Sound waves attenuate spherically from a stationary point source according to standard physical principles (International Organization for Standardization, 1996). Without atmosphere, wind, terrain, or land cover, sound pressure level decreases approximately 6 dB for every doubling of the distance that a sound wave travels. Table 4 provides example calculations of sound level values for propagation of sounds that are 75, 80, and 90 dB measured 15 m from the source.
Table 4.
Example calculation of spherical sound propagation without atmospheric, terrain, or landcover attenuation at three initial sound levels (75, 80, and 90 decibels [dB]) measured at a reference distance of 15 meters (m) from the source.[Table modified from Rutherford and others (2023). Sound levels decrease 6 dB for every doubling of distance from the source]
Under hypothetical conditions where environmental factors do not hinder the spread of sound, this basic calculation can be used to intentionally overestimate noise propagation. In other words, it can provide rough estimates of the maximum circular sound level contours around a stationary noise source. However, a critical caveat is that this method only overestimates sound levels when used to calculate sound propagation farther than the reference distance because it ignores environmental factors that impede the spread of sound. When calculating out from an estimated reference distance of 15 m, for example, all calculations farther than 20 m would be overestimates if there was a tall wall 20 m from the noise source. Conversely, using this method to estimate sound levels closer to a noise source than the reference distance would result in underestimates of sound levels. For example, if an estimate of 60 dB at a reference distance 100 m from the noise source were used to calculate 66 dB at 50 m, this method would underestimate sound level at 50 m if there were a sound barrier 75 m from the noise source.
In addition, this method should be used with caution because it is sensitive to slight changes in estimates at short distances from the noise source. For example, an estimate of 80 dB at 20 m yields a calculation of 56 dB at 360 m, whereas an estimate of 80 dB at 15 m yields 56 dB at 240 m, which results in a much smaller estimate of the area experiencing sound levels greater than 56 dB. Weighted sound level metrics such as dBA may be less suitable for this method because they apply different filters across frequency bands (refer to section “1.1.1. Sound Level”). Furthermore, for linear noise sources, such as busy highways that have continuous traffic noise, noise attenuates slower (Barber and others, 2011), and this method may not be appropriate for calculating propagation from linear sources.
An expanded version of this method for manually calculating sound propagation using basic physical equations is available in Harrison and others (1980). In addition to pure spherical spread, Harrison and others (1980) provide data that facilitate calculating impedance factors, including atmosphere, vegetation and ground cover, wind, and barriers.
3.2.2. Option 2—Geospatial Sound Propagation Modeling Tools
Many sound propagation modeling tools have been developed for different types of noise sources in various environmental contexts. The Noise Model Simulation (NMSim) sound propagation model (Ikelheimer and Plotkin, 2005; a link to the software is available in Betchkal and Balantic [2023]) is commonly used in public lands contexts (Keyel and others, 2017). The NPS has used NMSim in environmental effects analyses for oversnow vehicles (NPS, 2013b) and air travel (NPS, 2011). SPreAD-GIS (a link to the software is available in Keyel and others [2017]) is another sound propagation model developed for managing noise on public lands (Harrison and others, 1980; Reed and others, 2012; Keyel and others, 2017). The NMSim and SPreAD-GIS use identical methods to model atmospheric absorption and general spherical spread of sound. However, they differ in their calculation of how land cover, topography, and wind affect sound spread (Keyel and others, 2017). In addition, SPreAD-GIS is limited to modeling frequencies 125–2,000 Hz, whereas NMSim is not frequency-range limited (Keyel and Reed, 2017).
Although not specific to public lands contexts, many other sound modeling tools are available. For example, governments around the world use standard models for predicting noise emissions from highways (Federal Highway Administration, 2021; Khan and others, 2021). Each sound modeling tool has different limitations depending on its intended application, which is often specific to noise source and environmental context. In addition, tools that are not regularly maintained (for example, annually or biennially) may not function on newer versions of the underlying software (for example, Esri ArcGIS).
Specific software needs, data needs, and user guides are typically available in the documentation for each modeling tool. The three general types of environmental data used by these sound propagation models are atmospheric, topographic, and land cover. Average atmospheric data for a location, including temperature, humidity, and elevation, are generally easily obtainable (refer to, for example, the National Centers for Environmental Information, available at https://www.ncei.noaa.gov/), and inputting these data into a sound propagation model is relatively simple. In comparison, although topographic and land cover data are relatively easily obtainable (refer to, for example, The National Map, available at https://www.usgs.gov/programs/national-geospatial-program/national-map, for elevation data and the Multi-Resolution Land Characteristics Consortium, available at https://www.mrlc.gov/, for land cover data), they are geospatial data for which processing for sound propagation modeling is somewhat complex and requires experience using GIS software.
4. Potential Effects of Noise From Oil and Gas Development on Raptors and Songbirds
This section provides information relevant to the potential effects of noise from oil and gas development on raptors and songbirds. Information in this section may facilitate identification of issues for detailed analysis of the environmental consequences of a proposed action and alternatives.
4.1. How and Why Noise Affects Birds
Across taxonomic groups, existing research indicates many effects of human-caused noise on wildlife (Shannon and others, 2016; Kunc and Schmidt, 2019). Animals’ responses to noise vary based on characteristics of the noise (Francis and Barber, 2013). Acute noise can startle animals and cause a self-preservation response such as flight. Chronic noise can mask acoustic cues necessary for communication, predator detection, prey localization, habitat selection, or spatial awareness (Barber and others, 2010). Individuals that remain in noisy habitats can experience difficulties with auditory communication and environmental awareness that may increase visual vigilance behaviors at the cost of foraging (Frid and Dill, 2002; Bednarz, 2020). In addition, noise at varying frequencies and durations can distract animals from other sensory inputs, such as visual or tactile signals (Chan and others, 2010; Francis and Barber, 2013). Human-caused noise can also interfere with predator-prey dynamics and result in decreased hunting success for predators (Mason and others, 2016) or increased visual vigilance for prey species that are reliant on audible cues (Quinn and others, 2006; Sweet and others, 2022).
Among terrestrial wildlife, avian species’ responses to human-caused noise are relatively well studied (Shannon and others, 2016; Sordello and others, 2020). Investigations about the effects of noise on songbirds are especially well represented in the literature because of songbirds’ reliance on frequent vocal communication. Noise affects bird communication differently based on the spectral frequency of vocalizations (Francis, 2015) and the plasticity of their songs in the presence of noise (Roca and others, 2016). Strigiformes (owls) are also well represented in the literature because of their reliance on sounds for nocturnal hunting (Sordello and others, 2020). Studies indicate varying effects of human-caused noise on birds based on each species’ habitat, diet, and other life history traits, especially their reliance on acoustic cues for communication, foraging, and reproduction (Francis, 2015; Senzaki and others, 2020), which highlights the importance of understanding and analyzing the effects of noise on birds at the level of individual taxa and species. In addition, individuals of the same species may experience different effects of noise depending on traits such as body size, nutritional state, life stage, sex, and prior exposure to noise (Kleist and others, 2018; Harding and others, 2019). Overall, although effects are variable among bird species and individuals, many studies have found behavioral and physiological responses to noise among birds, including oil and gas development and traffic noise, as synthesized in the following sections. Ultimately, noise has been connected to negative fitness effects at local scales (McClure and others, 2013; Ware and others, 2015; Kleist and others, 2018) and across avian taxa at the national scale in the United States (Senzaki and others, 2020).
4.2. Effects of Noise on Raptors
Section 4.2 Highlights
• Many raptors rely on vision rather than hearing for hunting prey, but owls and northern harriers rely on a combination of sight and sound and can hunt using sound alone.
• Simulated compressor station and traffic noise can reduce hunting success of owls.
• Noise from vehicle traffic was associated with increased stress levels and reduced reproductive success in northern spotted owls.
• Studies in oil and gas fields in boreal forest and mixed-grass prairie have found that sound levels are not associated with owl occupancy or habitat use.
4.2.1. Why Noise Can Affect Raptors
Among raptors, owls are most often studied for their response to human-caused noise because most owl species have been shown to rely to some extent on sound for hunting. Most owl species are nocturnal; therefore, they must use a combination of sight and hearing to detect prey and have more sensitive hearing than other types of birds (Clark and others, 2020). Many owl species exhibit morphological adaptations to enable acute hearing and silent flight, such as asymmetrical ears, facial disks, and fringed wing feathers (Clark and others, 2020). Several studies have shown that noise can reduce owl hunting success by masking the sounds of prey (Mason and others, 2016; Senzaki and others, 2016). Circus hudsonius (northern harrier) is another species of raptor that has adapted to hunt using sound (Rice, 1982) but has not been studied for its response to human-caused noise.
In addition to hunting, noise can affect raptor habitat use, nesting behavior and success, and physiology. In one study, Strix virgata (mottled owl) tended to choose areas that were less noisy, such as green spaces, within urban areas (Marín-Gómez and others, 2020). In another study, male Falco peregrinus (peregrine falcon) attended the nest less often during incubation and brooding in response to noise from aircraft overflights, whereas females attended the nest more often (Palmer and others, 2003). Exposure to high intensity noise affected maturation in young Tyto alba (barn owl; Efrati and Gutfreund, 2011) and noise was associated with increased stress hormone responses in mottled owls (Strasser and Heath, 2013).
4.2.2. Studies About How Noise From Oil and Gas Development Can Affect Raptors
Few studies have investigated the effects of noise specific to oil and gas development on raptors, and all studies focused on owl species (table 1.1). Experimental evidence has shown that simulated oil and gas well noise can mask prey sounds and reduce owl hunting success in low-light conditions (Mason and others 2016). The odds of a successful nighttime hunt for Aegolius acadicus (northern saw-whet owl) decreased 8 percent for every 1 dBA increase in simulated compressor noise, which replicated sound measured 50–800 m from a compressor, and no hunting successes occurred when compressor noise was greater than 61 dBA (Mason and others, 2016). Owls have highly sensitive low-frequency hearing compared to songbirds and humans (Heffner and Heffner, 2010; Clark and others, 2020), and broadband compressor noise can overlap the sounds of prey movements and cause potential masking effects (Mason and others, 2016).
Meanwhile, studies in active oil and gas fields detected small or no effect of noise on owl habitat occupancy or use (Scobie and others, 2016; Shonfield and Bayne, 2017). Shonfield and Bayne (2017) studied the effects of noise from well pads with chronic noise (from compressors or oil processing plants) or intermittent noise (from traffic) on occupancy of Strix varia (barred owl), Bubo virginianus (great horned owl), and Aegolius funereus (boreal owl) in an oil field in a boreal forest ecosystem in Alberta, Canada. Chronic industrial noise did not affect the occupancy or habitat use of any of the three species (Shonfield and Bayne, 2017). The authors of the study speculated that because they measured occupancy at the scale of the home range of the species, which was larger than the noise footprint of the oil and gas infrastructure, owls may have been using areas outside of the noise footprint within their study sites (Shonfield and Bayne, 2017). The authors of the study also speculated that their results that showed no change in habitat use in noisy areas may have reflected nonhunting behaviors, which may not be as affected by noise as nighttime hunting (Shonfield and Bayne, 2017).
In a mixed-grass prairie oil and gas field in southern Alberta and Saskatchewan, Canada, the presence of infrastructure and surface disturbance (for example, roads, well pads, compressor stations, buildings, and towns) had greater effect than sensory disturbance (for example, noise and artificial light) on space use of Athene cunicularia (burrowing owl) during twilight and nighttime (Scobie and others, 2016). The authors of the study speculated that land-use changes altered the distribution of prey habitat and provided perches, which had a beneficial effect on burrowing owl hunting that outweighed the potentially negative masking effects of noise from well pads and roads (Scobie and others, 2016).
4.2.3. Studies About How Traffic Noise Can Affect Raptors
Traffic noise has been shown to affect hunting success, levels of stress hormones, and reproductive success in raptors. Using simulated traffic and prey rustling sounds, Senzaki and others (2016) found that elevated traffic noise impaired prey detection and hunting success in Asio flammeus (short-eared owl) and Asio otus (long-eared owl). The threshold at which owls became less able to detect prey was at approximately 40 dBA of traffic noise, which simulated the sound of traffic 120 m away traveling at 60 km/h.
Stress responses to traffic noise and associated reproductive effects have been studied in a few species. An acute, experimental increase in vehicle noise from 1 hour of high-volume motorcycle traffic on a northern California forest road was associated with fewer fledged Strix occidentalis caurina (northern spotted owl) and increased stress hormone levels in adult owls nesting or roosting within 800 m of the road (Hayward and others, 2011). In another forest study in northern Arizona, log trucks on forest roads 78–167 m from three Accipiter gentilis (northern goshawk) nests caused alert response during nearly 75 percent of truck passes (average 61.9 dB) but did not result in movement or flushing, and all three nests successfully fledged young (Grubb and others, 2013).
In addition to traffic noise, roads present a variety of physical effects that contribute to changes in land cover, habitat connectivity, perch availability, prey distribution, and artificial light at night that can generally affect space use, density, and occupancy of owls (Silva and others, 2012; Scobie and others, 2014). In mixed-grass prairie ecosystems in southern Alberta and Saskatchewan, Canada, where oil and gas development is common, burrowing owls were found to favor the use of roads, most likely because of the vegetation characteristics that supported small mammal populations (Scobie and others, 2014; Scobie and others, 2016). However, among roads, burrowing owls tended to avoid roads that had vehicle speeds greater than 80 km/h (Scobie and others, 2014).
4.2.4. Information Gaps Regarding the Effects of Noise From Oil and Gas Development on Raptors
Literature about the effects of noise from oil and gas development on raptors is limited to studies on owls in boreal forests and mixed-grass prairie. Additional studies about noise effects on owls during daytime resting periods when they are susceptible to predation could improve understanding of how to avoid disturbing individuals when oil and gas operations are most active. Further research about owl distributions and fitness related to sound pressure levels in oil and gas fields could help develop an understanding of thresholds for effects and inform mitigation techniques. Future study about the effects of noise on predator-prey dynamics could also inform understanding of community or ecosystem effects. No research studies reviewed in this synthesis focused on the effects of noise specific to oil and gas fields on raptor species other than owls. Further research about how noise affects other raptors could inform general understanding of the ecological effects of oil and gas development.
4.3. Effects of Noise on Songbirds and Other Small Avian Species
Section 4.3 Highlights
• Songbirds rely heavily on sound for communication with young, alarm calls, and mating.
• The effects of human-caused noise on songbirds vary depending on the noise source, species, habitat type, and time of year or phenology.
• Noise from oil and gas development can increase avoidance of the area and decrease abundance for multiple songbird species across ecosystems.
• Songbird species that have lower frequency songs may avoid noise from oil and gas development, whereas species that rely on higher frequency songs or can adapt song features may be more tolerant of noise.
• Some songbird species experience increased physiological stress and reduced reproductive or nest success in noisy oil and gas fields.
• Traffic noise can negatively affect physiology, behavior, and reproduction of songbirds.
4.3.1. Why Noise Can Affect Songbirds and Other Small Avian Species
Songbirds are a large group of perching bird species in the order Passeriformes. Songbirds rely on acoustic communication for information about their environment and are frequently studied for their response to human-caused noise (Shannon and others, 2016). Although songbirds are often a focus of noise studies, many studies investigate effects on bird communities and include other relatively small avian species such as Passeriformes (flycatchers), Apodiformes (hummingbirds), and Columbiformes (doves). Among these diverse groups of birds, the effects of human-caused noise depend on factors such as habitat type, noise source, and life history traits of the species (Francis and Barber, 2013). Noise can mask sounds such as alarm calls, mating songs, or communication with young (Francis and others, 2009). Intermittent or abrupt noise changes may be perceived as threats, whereas chronic noise may lead to additive stress responses through time (Francis and Barber, 2013).
4.3.2. Studies About How Oil and Gas Development Can Affect Songbirds and Other Small Avian Species
Many studies have investigated the effects of noise from oil and gas development on songbirds and communities of small avian species (table 5; refer to app. 2 for detailed results of studies by taxonomic group and community). Avian species and communities across habitats, including northern mixed-grass prairie, sagebrush steppe, mixed Pinus spp. (pinyon) and Juniperus spp. (juniper) woodlands (hereafter, pinyon-juniper woodlands), and boreal forest, demonstrate variable tolerance to noise (app. 2; table 2.1; Bayne and others, 2008; Kleist and others, 2017; Nenninger and Koper, 2018; Cinto Mejia and others, 2019). Kleist and others (2017) showed that noise levels were equally or more important than land cover classes in determining occupancy of an avian community in pinyon-juniper woodlands in northern New Mexico because of strong avoidance of noisy areas by half (2 of 4) of the study species. Bayne and others (2008) found that approximately one-third of species (7 of 23) in a boreal forest avian community in northern Alberta, Canada, showed some degree of avoidance of noise-producing compressor stations compared to quiet well pads, and Cinto Mejia and others (2019) found that 1 of 5 species in a sagebrush community had decreased abundance in response to compressor noise. The boreal and sagebrush studies found overall negative effects of noise on bird community density and abundance (Bayne and others, 2008; Cinto Mejia and others, 2019). Studies in mixed-grass prairie systems have shown varying results for abundance near different types of infrastructure among and within species (Nenninger and Koper, 2018; Rosa and Koper, 2022). Noise may have variable effects on songbirds in grassland habitats because of the heterogeneity of the natural acoustic environment caused by wind and other sounds, which may also facilitate adaptation to increased human-caused noise (Curry and others, 2018a; Nenninger and Koper, 2018).
Community-level effects of noise are driven by the noise tolerances of the individual species that comprise the community. The ability to tolerate noisy environments is associated with song spectral frequency (Francis and others, 2011c). Human-caused noise is typically most intense at low spectral frequencies, and birds that have low-frequency vocalizations may be more affected by the masking effects of human-caused noise than species that have high-frequency vocalizations or species that rely less heavily on vocalizations (Francis and others, 2011b). Species that have lower frequency songs tend to avoid noisy areas (Francis, 2015) and experience some negative fitness consequences in noisier areas (Senzaki and others, 2020). In the context of oil and gas well development, Melospiza lincolnii (Lincoln's sparrow) was tolerant of industrial disturbance and high intensity, low-frequency noise but avoided areas that had high intensity, high-frequency noise that overlapped the frequency of its song (Sánchez and others, 2022). Other studies have demonstrated varying degrees of vocal plasticity among species in the presence of noise from well pad infrastructure (Francis and others, 2011a, c, 2012a; Curry and others, 2018a, b). In addition, one study found that physiological differences among individuals were associated with responsiveness to frequency-adjusted songs in the presence of pumpjacks (Curry and others, 2018b). However, in a sagebrush avian community, Cinto Mejia and others (2019) suggested that observed variation in species abundance in response to simulated noise was not associated with species song frequency.
Although many species tolerate noise, studies have found primarily negative or neutral reproductive and physiological effects of noise on species that nest in noisy areas (table 5). In one study, chronic noise was associated with stress hormone levels in multiple secondary cavity nesting species (Kleist and others, 2018). In the same study, increased noise was associated with reduced hatching success for Sialia mexicana (western bluebird), which was the most common species at noisy sites (Kleist and others, 2018). The authors suggested their results indicated an ecological trap where species tolerant of noise during nest site selection experienced negative downstream fitness consequences during nesting (Kleist and others, 2018). In another study, although noise did not affect nest site selection, Sialia sialis (eastern bluebird) and Tachycineta bicolor (tree swallow) experienced lower incubation rates and lower hatching success at noisy sites (Williams and others, 2021). In that study, noise was not associated with food provisioning or probability of hatched young fledging, and the authors of the study suggested that noise affected reproductive fitness primarily during the incubation phase, potentially because of reduced prey abundance or increased visual vigilance when foraging during the incubation phase (Williams and others, 2021).
Table 5.
Effects of noise from oil and gas development on songbirds and other small avian species by type of effect and species or habitat community.[The findings presented in this table are limited to the results of studies within the scope of this report; this table does not provide a comprehensive review of literature regarding each type of noise effect. kHz, kilohertz; <, less than; >, greater than; dB, decibel; spp., several species of]
Noise can also affect fitness by inducing behavioral effects during nesting, such as reduced pairing success (Habib and others, 2007), delayed territory defense (Kleist and others, 2016), reduced provisioning rates (Ng and others, 2019) and incubation rates (Williams and others, 2021), and reduced antipredator behaviors when feeding nestlings (Antze and Koper, 2018). Fitness outcomes have included reduced hatching rates (Kleist and others, 2018; Williams and others, 2021) and physiological outcomes have included changes to stress hormone levels that indicated heightened stress loads (Curry and others, 2018b; Kleist and others, 2018). In a mixed-grass prairie community, intermittent drilling noise was associated with decreased nest survival for Passerculus sandwichensis (savannah sparrow), Anthus spragueii (Sprague’s pipit), and Pooecetes gramineus (vesper sparrow; Rosa and Koper, 2022). Conversely, Bernath-Plaisted and Koper (2016) showed that savannah sparrow and vesper sparrow nesting success was lower at sites that had infrastructure and was not related to noise. Noise can also change predator dynamics; in a pinyon-juniper bird community, nest success was higher in noisy areas, likely because of observed reduced daily predation rates, especially from the common predator Aphelocoma californica (California scrub-jay; Francis and others, 2009).
Findings specific to noise complement additional literature that has demonstrated effects from the suite of physical and sensory disturbances common in oil and gas fields (Northrup and Wittemyer, 2013). Increased density of well pads is associated with decreased abundance for some sagebrush and grassland birds (Gilbert and Chalfoun, 2011; Hamilton and others, 2011), and decreased density of birds near edges of oil and gas development features has been shown for several grassland birds (Thompson and others, 2015). Other studies have demonstrated effects to reproductive success because of habitat loss, increased predation, or increased cover of nonnative herbaceous plants in oil and gas fields (Hethcoat and Chalfoun, 2015a, b; Ludlow and others, 2015; Farwell and others, 2019).
4.3.3. Studies About How Noise From Traffic Can Affect Songbirds and Other Small Avian Species
The effects of traffic noise have been widely studied for songbirds, especially in urban settings (Slabbekoorn and Ripmeester, 2008; Sordello and others, 2020). An increasing number of studies have measured the effects of traffic noise in rural and natural systems (McClure and others, 2013; Ware and others, 2015; McClure and others, 2017; Injaian and others, 2018). Traffic noise may affect songbird physiology, behavior, and reproductive success. For example, simulated road traffic noise played 6 hours every other day resulted in reduced vigilance in adult tree swallows, decreased nestling body size, and increased nestling physiological stress (Injaian and others, 2018).
Some studies have shown community-level effects of traffic noise on songbirds. Simulated road noise in mountain shrublands resulted in decreased abundance for 13 of 22 commonly observed bird species when noise levels of the simulated road averaged 55 dBA, which was 11 dBA higher than background sound levels (McClure and others, 2013). Haemorhous cassinii (Cassin’s finch) was positively associated with the simulated road noise, whereas Bombycilla cedrorum (cedar waxwing) and Setophaga petechia (yellow warbler) avoided the road noise completely (McClure and others, 2013). A follow-up study found that traffic noise led to lower body condition and stopover productivity for hatch-year and adult songbirds, and that younger birds avoided roads more than adults, which resulted in age-structure shifts across the community (McClure and others, 2017). In another study, more than one-third of migratory birds avoided simulated road noise (approximately 55 dBA), and when individuals remained in the noisy area, body condition decreased across the bird community, and a few species were unable to improve body condition during their migratory stopovers (Ware and others, 2015).
In addition to noise, roads are associated with many other well-studied effects to songbirds, such as habitat loss and fragmentation, mortality from vehicle collisions, air and water contamination, edge effects, and light at night (Kociolek and others, 2011).
4.3.4. Information Gaps Regarding the Effects of Noise From Oil and Gas Development on Songbirds and Other Small Avian Species
The studies synthesized in this report collectively show the potential for negative community-level, behavioral, and reproductive effects of noise from oil and gas development on various songbird species across boreal forest, mixed-grass prairie, pinyon-juniper, and sagebrush ecosystems. To build upon this existing body of work, further research that relates songbird responses to sound pressure levels across species could be useful for understanding thresholds for effects and identifying mitigation techniques. In addition, more information about the cumulative or interactive effects of noise with other environmental factors, such as changes to land cover or vegetation composition, may be needed. Finally, although many studies have shown negative fitness, reproductive, and physiological consequences, including increased stress, lower clutch sizes, or decreased parental care, research that measures theses effects throughout longer timeframes may inform understanding of community and ecosystem-level effects.
5. Mitigating the Effects of Noise From Oil and Gas Development on Raptors and Songbirds
Section 5 Highlights
• Projects can be designed to help reduce noise emissions and the effects of noise from oil and gas development on birds.
• Approaches to mitigation could include the following:
o siting wells to take advantage of natural topographic and vegetation sound barriers,
o concentrating wells spatially,
o adding artificial sound barriers, such as walls around well pads or blankets or baffles on compressor stations,
o implementing seasonal or daily restrictions on oil and gas activity,
o reducing traffic volume and speed, and
o closing roads after completion of operations to prevent recreational use.
Projects can be designed to help reduce noise emissions and the effects of noise from oil and gas development on birds. Techniques to reduce noise primarily entail adjusting the timing and spatial configuration of development or adding supplementary components or structures to reduce sound propagation. Because of differences in species’ habitats, life history traits, and noise sensitivity, use of multiple approaches may be necessary to benefit a broader range of taxa (Blickley and Patricelli, 2010). Techniques to reduce noise can be applied prior to, during, and after various phases of oil and gas development to address different noise sources in and around a well pad through time.
5.1. Well Pad Siting and Infrastructure Considerations
Ortega (2012) and Keyel and others (2018) recommended considering landscape and habitat-specific features when planning the spatial configuration of well pads and infrastructure. These studies indicated that natural topographic and vegetation barriers may be useful for attenuating noise. Using hypothetical well locations, Keyel and others (2018) documented how sound propagation modeling can identify locations that optimize the use of topographic barriers to block noise. Differences in terrain at alternative well locations as little as 1 km from one another reduced the area of noise propagation by as much as 65 percent for drilling noise and 35 percent for compressor station noise (Keyel and others, 2018).
Concentrating disturbance and minimizing new road construction can also shrink the noise footprint. Concentrating drilling operations at multiwell pads and placing well pads near existing roads may reduce total noise emissions (Thompson and others, 2015). Multiwell pads may also increase the efficiency of onsite physical noise attenuation measures such as sound barriers (Bayne and others, 2008); however, the high intensity of operations can cause high noise effects in the immediate vicinity (Allshouse and others, 2019). Installing remote liquid-gathering systems, such as pipelines, can reduce physical habitat disturbance, noise generated at a well pad, and the number of trucks needed to transport resources (Sawyer and others, 2009).
5.2. Sound Barriers
Sound reduction technology can take many forms, such as walls (fig. 6A, B), blankets, baffles, or vegetation. Installing sound barrier walls around centralized compressor stations can decrease the distance noise travels (Ekici and Bougdah, 2003; Radtke and others, 2017). For example, a study in a pinyon-juniper landscape showed that sound-reducing walls around compressors reduced the size of the affected soundscape by 70 percent, which resulted in nest success and occupancy levels similar to levels that would be expected in an area without compressors (Francis and others, 2011d). In Colorado, drilling sites that had 9.8-m tall sound barrier walls had approximately 6 dBA lower sound levels (average 59 dBA across 3 sites) than sites without barriers (average 65 dBA across 4 sites), measured 107 m from the source (Radtke and others, 2017). Similarly, noise blankets on generators during drilling at natural gas well sites reduced sound levels approximately 4 dBA when measured 152 m and 305 m from the source (Witter and others, 2013).
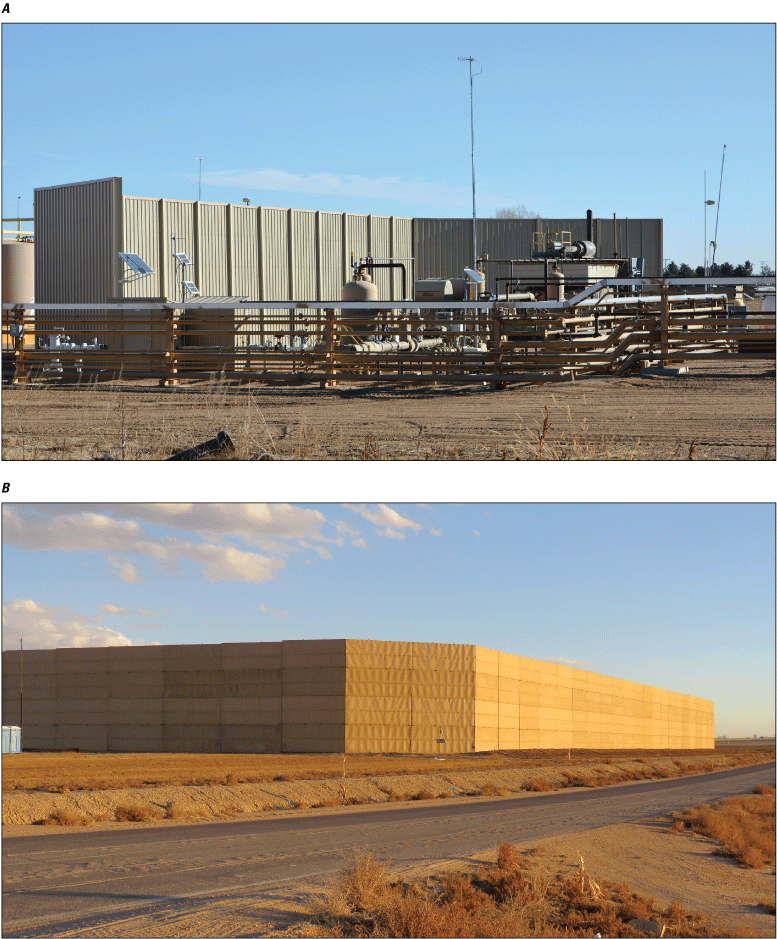
A, Metal sound dampening walls around a natural gas compressor in Colorado. B, Taller padded sound barrier around a well pad in Colorado. [Photographs from Tait Rutherford and Patrick Anderson, U.S. Geological Survey.]
Noise barriers can be placed at sound-deflecting angles and use construction materials that absorb sound (Ekici and Bougdah, 2003). Ishizuka and Fujiwara (2004) documented that 3-m high soft T-shaped barriers had the same effectiveness as 10-m high I-shaped barriers in blocking road traffic noise. Vegetation barriers or berms around noise producing infrastructure or near roadways may also absorb some sound, particularly in forested areas where higher leaf and stem density increase sound absorption potential (Slabbekoorn and Ripmeester, 2008).
5.3. Seasonal and Daily Timing Restrictions and Sound Level Thresholds
Seasonal and daily timing restrictions on oil and gas activity are a tool to minimize noise effects (among other disturbances) to wildlife during crucial life history stages, such as nesting, brood rearing, and wintering. For example, in a range-wide management plan for Centrocercus urophasianus (greater sage-grouse), the Bureau of Land Management (BLM) and U.S. Department of Agriculture Forest Service prohibited projects from creating greater than 10 dB increases above background sound levels at the perimeter of sage-grouse leks in the morning during the breeding season (BLM, 2015a, b; U.S. Department of Agriculture Forest Service, 2015a, b). However, relative noise exceedance thresholds can be challenging to implement because of the difficulty associated with measuring background sound levels in quiet environments, which can result in overestimation of the sound level at which effects to a species may occur (refer to section “2. Characterizing the Acoustic Environment”).
Absolute sound level thresholds are an alternative to relative noise exceedance thresholds. Absolute thresholds can provide stable, easily measurable mitigation targets that facilitate decision making by managers and industry operators, although thresholds for effects can be difficult to precisely determine for individual species and are highly variable among species (refer to apps. 1 and 2). Some studies may provide information about ecosystem-specific thresholds for specific species (for example, Ambrose and others, 2021). In another example, a range-wide conservation plan for Tympanuchus pallidicinctus (lesser prairie-chicken) recommended that noise from well pads and compressor stations in suitable habitat not exceed 75 dB measured 30 feet from a facility boundary (Van Pelt and others, 2013). The plan also suggested other seasonal and daily activity restrictions, although not noise specific, such as restricting nonemergency projects during the lekking and nesting season, and, if emergency projects must occur in the breeding season, requiring that activity not occur in the morning (Van Pelt and others, 2013).
In general, thresholds and timing restrictions are informed by species life history and phenology, past studies regarding effects, and decision-making processes to ensure such measures are protective for species and practicable for oil and gas operators and land managers.
5.4. Traffic Control
Because traffic noise is dependent on vehicle speed and road surface, measures to control these two variables can reduce noise effects on wildlife. Options include lowering speed limits and improving road surfaces (Shannon and others, 2016). Both measures include tradeoffs. Lowering the speed of a vehicle increases the duration of the noise exposure for an animal in the area and results in a longer, lower intensity noise compared to a shorter, higher intensity noise. In addition, although improved road surfaces can reduce noise levels compared to vehicles traveling the same speed on a rougher road surface, they also allow for higher driving speeds, which can offset the intended noise reduction.
At the scale of an entire oil and gas field, management of total road length using road network planning, siting new well pads and infrastructure near existing roads, and concentrating development (for example, on multiwell pads) to limit new road construction are potential strategies to reduce overall traffic noise (Thompson and others, 2015). In addition, after oil and gas development, deconstructing roads during the reclamation process can limit future public access and reduce potential traffic noise effects (Northrup and others, 2021).
Methods for Developing This Science Synthesis
Rutherford and others (2023) introduced a methodology for developing science syntheses to inform analyses conducted under the NEPA. This and other syntheses build on that foundation and methodology and apply it to new topics of management concern on public lands in the Western United States. Therefore, relevant introductory and methods text from Rutherford and others (2023)x is reproduced herein. In addition, this science synthesis covers many of the same bodies of science as Rutherford and others (2023), which addressed the effects of noise from oil and gas development on ungulates and small mammals. Much of the content of this report that is not specific to raptors and songbirds is reproduced from Rutherford and others (2023) and includes updates and refinements as appropriate. The following methods describe the literature searches conducted for this report, which overlap the searches conducted for Rutherford and others (2023).
We did a literature search to gather science about the effects of noise from oil and gas development on birds. We sought information relevant to NEPA analyses (Carter and others, 2023), including data about the acoustic environment, studies demonstrating effects of noise on birds, methods for analyzing potential effects, and the efficacy of management practices to reduce potential adverse effects. Our literature search had two components: (1) structured searches using a literature map and online databases and (2) snowballing (Wäldchen and Mäder, 2018) to identify literature for topics for which structured searches were not necessary.
First, we identified noise literature published prior to 2019 using a recent systematic literature map (Sordello and others, 2020). The map includes a total of 1,794 peer reviewed and gray literature publications (Sordello and others, 2020). Using data provided by the authors, we filtered this literature map by taxonomic class (“birds”) and language (“English”), which resulted in 513 publications. We then manually filtered titles and abstracts to identify studies specific to the effects of noise from oil and gas development on raptors and songbirds. We focused on wild, terrestrial North American taxa potentially occurring on public lands, although we expected that the search would return potentially useful results for species not meeting these criteria. We reviewed studies not meeting these criteria if they were relevant to understanding noise effects from oil and gas development. We did not focus on greater sage-grouse and other gallinaceous birds (for example, quail and turkeys). Noise effects on sage-grouse have been addressed elsewhere in detail in the Federal land management context (Tull, 2015; Ambrose and others, 2021).
To find literature outside of the timeframe captured by Sordello and others (2020), we searched for literature published between January 1, 2019, and June 7, 2022 (date of search), using two citation indices (Web of Science, Scopus; accessed through the U.S. Geological Survey [USGS] Library) and two Federal Government publication databases (USGS ScienceBase and USGS Publications Warehouse; Kleist and Enns, 2021). We used the search phrase ([“anthropogenic noise” OR “anthropogenic sound” OR “noise pollution” OR “sound pollution”] AND [“bird*”]). This search returned 210 publications. We then manually filtered titles and abstracts to identify studies specific to the effects of noise from oil and gas development on raptors and songbirds.
These structured searches of the Sordello and others (2020) literature map and online databases directly informed section “4. Potential Effects of Noise From Oil and Gas Development on Raptors and Songbirds” of this science synthesis, which addressed the core question of identifying potential relationships between noise from oil and gas development and birds. The structured searches also indirectly informed the other sections of this synthesis, which address the broader topics of understanding potential noise from oil and gas development (section “1. Characterizing Noise Caused by Oil and Gas Development”), collecting data to characterize an acoustic environment (section “2. Characterizing the Acoustic Environment”), modeling the spatial extent of the potential spread of noise from a proposed action (section “3. Methods for Predicting Noise Occurrence on the Landscape”), and mitigating noise effects (section “5. Mitigating the Effects of Noise From Oil and Gas Development on Raptors and Songbirds”).
For sections 1, 2, 3, and 5, we gathered additional literature by snowballing (Wäldchen and Mäder, 2018) from key review articles and performing supplemental searches with online databases and search engines. These sections addressed broader topics, for which literature regarding core concepts was sufficient to inform their inclusion in a NEPA analysis. Substantial amounts of literature were available about each of these topics, but a structured search to review the broader literature was inessential to address the specific issue of the effects of noise from oil and gas development on raptors and songbirds.
We synthesized information returned in the structured searches and snowballing component according to our objective to inform NEPA analyses. Rather than reporting all the literature we found, we synthesize only the literature applicable to informing analyses of the potential effects of noise from oil and gas development projects on birds. As such, this synthesis does not constitute a comprehensive literature review of all effects of noise on songbirds and raptors. In addition, it is possible that our literature search methods missed some relevant studies.
Throughout the development of this report, we worked with staff from the BLM, U.S. Fish and Wildlife Service, and USGS to coproduce this report (Beier and others, 2017). We refined the structure and content of the report through close collaboration with multiple BLM staff throughout scoping, writing, and review.
References Cited
Acoustical Society of America, [undated], ASA standards working group—Acoustical terminology: Acoustical Society of America web page, accessed March 4, 2024, at https://asastandards.org/working-groups-portal/asa-standard-term-database/. [Dropdown search term: ANSI/ASA S1.1-2013.]
Allshouse, W.B., McKenzie, L.M., Barton, K., Brindley, S., and Adgate, J.L., 2019, Community noise and air pollution exposure during the development of a multi-well oil and gas pad: Environmental Science & Technology, v. 53, no. 12, p. 7126–7135, accessed April 12, 2022, at https://doi.org/10.1021/acs.est.9b00052.
Ambrose, S., and Florian, C., 2014, Sound levels at greater sage-grouse leks, Pinedale Anticline Project Area, Wyoming, April 2013: Cheyenne, Wyo., Wyoming Game and Fish Department, prepared by Sandhill Company, 133 p., accessed April 28, 2022, at https://eplanning.blm.gov/public_projects/nepa/77515/176004/214443/SageGrouseSound_2013_PAPO_AR.pdf.
Ambrose, S., Florian, C., Olnes, J., MacDonald, J., and Hartman, T., 2021, Sagebrush soundscapes and the effects of gas-field sounds on greater sage-grouse: Western Birds, v. 52, no. 1, p. 23–46, accessed July 25, 2022, at https://doi.org/10.21199/WB52.1.2.
Antze, B., and Koper, N., 2018, Noisy anthropogenic infrastructure interferes with alarm responses in Savannah sparrows (Passerculus sandwichensis): Royal Society Open Science, v. 5, no. 5, art. 172168, 13 p., accessed July 21, 2022, at https://doi.org/10.1098/rsos.172168.
Attenborough, K., 2002, Sound propagation close to the ground: Annual Review of Fluid Mechanics, v. 34, no. 1, p. 51–82, accessed May 30, 2023, at https://doi.org/10.1146/annurev.fluid.34.081701.143541.
Bagaini, C., Laycock, M., Readman, C., Coste, E., and Anderson, C., 2014, Seismo-acoustic characterization of a seismic vibrator: Society of Exploration Geophysicists Technical Program Expanded Abstracts, p. 25–29, accessed June 5, 2023, at https://doi.org/10.1190/segam2014-1126.1.
Barber, J.R., Burdett, C.L., Reed, S.E., Warner, K.A., Formichella, C., Crooks, K.R., Theobald, D.M., and Fristrup, K.M., 2011, Anthropogenic noise exposure in protected natural areas—Estimating the scale of ecological consequences: Landscape Ecology, v. 26, no. 9, p. 1281–1295, accessed August 25, 2023, at https://doi.org/10.1007/s10980-011-9646-7.
Barber, J.R., Crooks, K.R., and Fristrup, K.M., 2010, The costs of chronic noise exposure for terrestrial organisms: Trends in Ecology & Evolution, v. 25, no. 3, p. 180–189, accessed March 21, 2022, at https://doi.org/10.1016/j.tree.2009.08.002.
Bayne, E.M., Habib, L., and Boutin, S., 2008, Impacts of chronic anthropogenic noise from energy-sector activity on abundance of songbirds in the boreal forest: Conservation Biology, v. 22, no. 5, p. 1186–1193, accessed March 30, 2022, at https://doi.org/10.1111/j.1523-1739.2008.00973.x.
Bednarz, P.A., 2020, Do decibels matter? A review of effects of traffic noise on terrestrial small mammals and bats: Polish Journal of Ecology, v. 68, no. 4, p. 323–333, accessed September 13, 2022, at https://doi.org/10.3161/15052249PJE2020.68.4.005.
Beier, P., Hansen, L.J., Helbrecht, L., and Behar, D., 2017, A how-to guide for coproduction of actionable science: Conservation Letters, v. 10, no. 3, p. 288–296, accessed March 23, 2022, at https://doi.org/10.1111/conl.12300.
Berger, E.H., Neitzel, R., and Kladden, C.A., 2016, Noise Navigator sound level database with over 1700 measurement values (ver. 1.8, August 2016): Indianapolis, Ind., 3M Personal Safety Division, E-A-RCAL Laboratory, University of Michigan, E-A-R 88-34/HP, accessed November 22, 2023, at https://www.google.com/url?sa=t&rct=j&q=&esrc=s&source=web&cd=&cad=rja&uact=8&ved=2ahUKEwiZzfaJ6OKEAxWpEDQIHUslCTwQFnoECBEQAQ&url=https%3A%2F%2Fmultim edia.3m.com%2Fmws%2Fmedia%2F1262312O%2F3m-noise-navigator.xlsx&usg=AOvVaw3C-s2OG2RXSGYFu79VhKvU&opi=89978449.
Bernath-Plaisted, J., and Koper, N., 2016, Physical footprint of oil and gas infrastructure, not anthropogenic noise, reduces nesting success of some grassland songbirds: Biological Conservation, v. 204, pt. B, p. 434–441, accessed July 13, 2022, at https://doi.org/10.1016/j.biocon.2016.11.002.
Betchkal, D.H., and Balantic, C., 2023, NMSIM-Python: NMSIM-Python software release, accessed June 19, 2023, at https://github.com/dbetchkal/NMSIM-Python.
Blair, B.D., Brindley, S., Dinkeloo, E., McKenzie, L.M., and Adgate, J.L., 2018a, Residential noise from nearby oil and gas well construction and drilling: Journal of Exposure Science & Environmental Epidemiology, v. 28, no. 6, p. 538–547, accessed April 8, 2022, at https://doi.org/10.1038/s41370-018-0039-8.
Blair, B.D., Brindley, S., Hughes, J., Dinkeloo, E., McKenzie, L.M., and Adgate, J.L., 2018b, Measuring environmental noise from airports, oil and gas operations, and traffic with smartphone applications—Laboratory and field trials: Journal of Exposure Science & Environmental Epidemiology, v. 28, no. 6, p. 548–558, accessed August 25, 2023, at https://doi.org/10.1038/s41370-018-0077-2.
Blickley, J.L., and Patricelli, G.L., 2010, Impacts of anthropogenic noise on wildlife—Research priorities for the development of standards and mitigation: Journal of International Wildlife Law & Policy, v. 13, no. 4, p. 274–292, accessed April 20, 2022, at https://doi.org/10.1080/13880292.2010.524564.
Blickley, J.L., and Patricelli, G.L., 2013, Noise monitoring recommendations for greater sage-grouse habitat in Wyoming: Pinedale Anticline Project Office and Wyoming Game and Fish Pinedale Office, prepared by University of California, 18 p., accessed May 31, 2022, at https://eplanning.blm.gov/public_projects/nepa/77515/176004/214441/SageGrouseSound_2012_PAPO_Recommendations.pdf.
Blix, A.S., and Lentfer, J.W., 1992, Noise and vibration levels in artificial polar bear dens as related to selected petroleum exploration and developmental activities: Arctic, v. 45, no. 1, p. 20–24, accessed March 31, 2022, at https://doi.org/10.14430/arctic1369.
Boycott, T.J., Gao, J., and Gall, M.D., 2019, Deer browsing alters sound propagation in temperate deciduous forests: PLoS ONE, v. 14, no. 2, art. e0211569, 20 p., accessed May 30, 2023, at https://doi.org/10.1371/journal.pone.0211569.
Boyle, M.D., Soneja, S., Quirós-Alcalá, L., Dalemarre, L., Sapkota, A.R., Sangaramoorthy, T., Wilson, S., Milton, D., and Sapkota, A., 2017, A pilot study to assess residential noise exposure near natural gas compressor stations: PLoS ONE, v. 12, no. 4, art. E0174310, 15 p., accessed March 29, 2023, at https://doi.org/10.1371/journal.pone.0174310.
Bradshaw, C.J.A., Boutin, S., and Hebert, D.M., 1997, Effects of petroleum exploration on woodland caribou in northeastern Alberta: The Journal of Wildlife Management, v. 61, no. 4, p. 1127–1133, accessed April 14, 2022, at https://doi.org/10.2307/3802110.
Brattstrom, B.H., and Bondello, M.C., 1983, Effects of off-road vehicle noise on desert vertebrates, in Webb, R.H., and Wilshire, H.G., eds., Environmental effects of off-road vehicles—Impacts and management in arid regions: New York, Springer-Verlag, p. 167–206, accessed May 24, 2022, at https://doi.org/10.1007/978-1-4612-5454-6_9.
Brittan-Powell, E.F., Lohr, B., Hahn, D.C., and Dooling, R.J., 2005, Auditory brainstem responses in the eastern screech owl—An estimate of auditory thresholds: The Journal of the Acoustical Society of America, v. 118, no. 1, p. 314–321, accessed July 12, 2023, at https://doi.org/10.1121/1.1928767.
Brittingham, M.C., Maloney, K.O., Farag, A.M., Harper, D.D., and Bowen, Z.H., 2014, Ecological risks of shale oil and gas development to wildlife, aquatic resources and their habitats: Environmental Science & Technology, v. 48, no. 19, p. 11034–11047, accessed April 5, 2023, at https://doi.org/10.1021/es5020482.
Bureau of Land Management, 2015a, Bureau of Land Management Casper, Kemmerer, Newcastle, Pinedale, Rawlins, and Rock Springs Field Offices approved resource management plan amendment for greater sage-grouse: Cheyenne, Wyo., U.S. Department of the Interior, Bureau of Land Management, Wyoming State Office, 111 p., accessed March 7, 2024, at https://eplanning.blm.gov/public_projects/lup/103347/143767/177179/002_Wyoming_ARMPA_Main-Body.pdf.
Bureau of Land Management, 2015b, Record of decision and approved resource management plan amendments for the Rocky Mountain Region, including the greater sage-grouse sub-regions of Lewistown, North Dakota, Northwest Colorado, Wyoming and the approved resource management plans for Billings, Buffalo, Cody, HiLine, Miles City, Pompeys Pillar National Monument, South Dakota, Worland: Washington, D.C., U.S. Department of the Interior, Bureau of Land Management, 328 p., accessed March 7, 2024, at https://eplanning.blm.gov/public_projects/lup/105596/143663/176863/2015_Rocky_Mountain_Region_Record_GRSG_ROD_ARMPA_508.pdf.
Buxton, R.T., McKenna, M.F., Mennitt, D., Fristrup, K., Crooks, K., Angeloni, L., and Wittemyer, G., 2017, Noise pollution is pervasive in U.S. protected areas: Science, v. 356, no. 6337, p. 531–533, accessed April 7, 2022, at https://doi.org/10.1126/science.aah4783.
Byrnes, P., Goosem, M., and Turton, S.M., 2012, Are less vocal rainforest mammals susceptible to impacts from traffic noise?: Wildlife Research, v. 39, no. 4, p. 355–365, accessed April 28, 2022, at https://doi.org/10.1071/WR11010.
Carter, S.K., Haby, T.S., Meineke, J.K., Foster, A.C., McCall, L.E., Espy, L.D., Gilbert, M.A., Herrick, J.E., and Prentice, K.L., 2023, Prioritizing science efforts to inform decision making on public lands: Frontiers in Ecology and the Environment, v. 21, no. 10, p. 453–460, accessed March 7, 2024, at https://doi.org/10.1002/fee.2672.
Chan, A.A.Y.-H., Stahlman, W.D., Garlick, D., Fast, C.D., Blumstein, D.T., and Blaisdell, A.P., 2010, Increased amplitude and duration of acoustic stimuli enhance distraction: Animal Behaviour, v. 80, no. 6, p. 1075–1079, accessed May 4, 2022, at https://doi.org/10.1016/j.anbehav.2010.09.025.
Chen, H.L., and Koprowski, J.L., 2015, Animal occurrence and space use change in the landscape of anthropogenic noise: Biological Conservation, v. 192, p. 315–322, accessed April 29, 2022, at https://doi.org/10.1016/j.biocon.2015.10.003.
Cinto Mejia, E., McClure, C.J.W., and Barber, J.R., 2019, Large-scale manipulation of the acoustic environment can alter the abundance of breeding birds—Evidence from a phantom natural gas field: Journal of Applied Ecology, v. 56, no. 8, p. 2091–2101, accessed July 13, 2022, at https://doi.org/10.1111/1365-2664.13449.
Clark, C.J., LePiane, K., and Liu, L., 2020, Evolution and ecology of silent flight in owls and other flying vertebrates: Integrative Organismal Biology, v. 2, no. 1, 32 p., accessed August 29, 2022, at https://doi.org/10.1093/iob/obaa001.
Colorado Oil and Gas Conservation Commission, 2022, Glossary of oil and gas terms: Colorado Oil and Gas Conservation Commission web page, accessed May 2, 2023, at https://cogcc.state.co.us/COGIS_Help/glossary.htm.
Curry, C.M., Antze, B., Warrington, M.H., Des Brisay, P., Rosa, P., and Koper, N., 2018a, Ability to alter song in two grassland songbirds exposed to simulated anthropogenic noise is not related to pre-existing variability: Bioacoustics, v. 27, no. 2, p. 105–130, accessed November 29, 2022, at https://doi.org/10.1080/09524622.2017.1289123.
Curry, C.M., Des Brisay, P.G., Rosa, P., and Koper, N., 2018b, Noise source and individual physiology mediate effectiveness of bird songs adjusted to anthropogenic noise: Scientific Reports, v. 8, no. 1, art. 3942, 9 p., accessed September 16, 2022, at https://doi.org/10.1038/s41598-018-22253-5.
Cushing, L.J., Vavra-Musser, K., Chau, K., Franklin, M., and Johnston, J.E., 2020, Flaring from unconventional oil and gas development and birth outcomes in the Eagle Ford Shale in South Texas: Environmental Health Perspectives, v. 128, no. 7, art. 77003, 9 p., accessed July 10, 2023, at https://doi.org/10.1289/EHP6394.
Delaney, D.K., Grubb, T.G., Beier, P., Pater, L.L., and Reiser, M.H., 1999, Effects of helicopter noise on Mexican spotted owls: The Journal of Wildlife Management, v. 63, no. 1, p. 60–76, accessed July 28, 2022, at https://doi.org/10.2307/3802487.
Delaney, D.K., Pater, L.L., Carlile, L.D., Spadgenske, E.W., Beaty, T.A., and Melton, R.H., 2011, Response of red-cockaded woodpeckers to military training operations: Wildlife Monographs, v. 177, no. 1, p. 1–38, accessed November 22, 2023, at https://doi.org/10.1002/wmon.3.
Dominoni, D.M., Halfwerk, W., Baird, E., Buxton, R.T., Fernández-Juricic, E., Fristrup, K.M., McKenna, M.F., Mennitt, D.J., Perkin, E.K., Seymoure, B.M., Stoner, D.C., Tennessen, J.B., Toth, C.A., Tyrrell, L.P., Wilson, A., Francis, C.D., Carter, N.H., and Barber, J.R., 2020, Why conservation biology can benefit from sensory ecology: Nature Ecology & Evolution, v. 4, no. 4, p. 502–511, accessed November 17, 2023, at https://doi.org/10.1038/s41559-020-1135-4.
Dooling, R.J., 1992, Hearing in birds, in Webster, D.B., Popper, A.N., and Fay, R.R., eds., The evolutionary biology of hearing: New York, Springer-Verlag, p. 545–559, accessed July 18, 2023, at https://doi.org/10.1007/978-1-4612-2784-7_33.
Dowling, A.P., and Mahmoudi, Y., 2015, Combustion noise: Proceedings of the Combustion Institute, v. 35, no. 1, p. 65–100, accessed March 8, 2024, at https://doi.org/10.1016/j.proci.2014.08.016.
Dyson, M.L., Klump, G.M., and Gauger, B., 1998, Absolute hearing thresholds and critical masking ratios in the European barn owl—A comparison with other owls: Journal of Comparative Physiology A, v. 182, no. 5, p. 695–702, accessed July 12, 2023, at https://doi.org/10.1007/s003590050214.
Efrati, A., and Gutfreund, Y., 2011, Early life exposure to noise alters the representation of auditory localization cues in the auditory space map of the barn owl: Journal of Neurophysiology, v. 105, no. 5, p. 2522–2535, accessed July 28, 2022, at https://doi.org/10.1152/jn.00078.2011.
Ekici, I., and Bougdah, H., 2003, A review of research on environmental noise barriers: Building Acoustics, v. 10, no. 4, p. 289–323, accessed May 3, 2023, at https://doi.org/10.1260/135101003772776712.
Engineering ToolBox, 2003, Decibel a, b and c—Sound pressure filters that compensates for the hearing sensed by the human ear: The Engineering ToolBox web page, accessed April 6, 2023, at https://www.engineeringtoolbox.com/decibel-d_59.html.
Farwell, L.S., Wood, P.B., Brown, D.J., and Sheehan, J., 2019, Proximity to unconventional shale gas infrastructure alters breeding bird abundance and distribution: The Condor, v. 121, no. 3, 20 p., accessed September 14, 2022, at https://doi.org/10.1093/condor/duz020.
Federal Highway Administration, 2021, Technical manual—Traffic Noise Model 3.1: Washington, D.C., U.S. Department of Transportation Federal Highway Administration Office of Natural Environment FHWA-HEP-21-041, 150 p., accessed March 7, 2024, at https://www.fhwa.dot.gov/environment/noise/traffic_noise_model/old_versions/tnm_version_31/fhwahep21041.pdf.
Francis, C.D., 2015, Vocal traits and diet explain avian sensitivities to anthropogenic noise: Global Change Biology, v. 21, no. 5, p. 1809–1820, accessed September 19, 2022, at https://doi.org/10.1111/gcb.12862.
Francis, C.D., and Barber, J.R., 2013, A framework for understanding noise impacts on wildlife—An urgent conservation priority: Frontiers in Ecology and the Environment, v. 11, no. 6, p. 305–313, accessed March 24, 2022, at https://doi.org/10.1890/120183.
Francis, C.D., Kleist, N.J., Davidson, B.J., Ortega, C.P., and Cruz, A., 2012a, Chapter 4—Behavioral responses by two songbirds to natural-gas-well compressor noise: Ornithological Monographs, v. 74, no. 1, p. 36–46, accessed August 30, 2022, at https://doi.org/10.1525/om.2012.74.1.36.
Francis, C.D., Kleist, N.J., Ortega, C.P., and Cruz, A., 2012b, Noise pollution alters ecological services—Enhanced pollination and disrupted seed dispersal: Proceedings of the Royal Society B—Biological Sciences, v. 279, no. 1739, p. 2727–2735, accessed April 1, 2022, at https://doi.org/10.1098/rspb.2012.0230.
Francis, C.D., Ortega, C.P., and Cruz, A., 2009, Noise pollution changes avian communities and species interactions: Current Biology, v. 19, no. 16, p. 1415–1419, accessed March 30, 2022, at https://doi.org/10.1016/j.cub.2009.06.052.
Francis, C.D., Ortega, C.P., and Cruz, A., 2011a, Different behavioural responses to anthropogenic noise by two closely related passerine birds: Biology Letters, v. 7, no. 6, p. 850–852, accessed September 22, 2022, at https://doi.org/10.1098/rsbl.2011.0359.
Francis, C.D., Ortega, C.P., and Cruz, A., 2011b, Noise pollution filters bird communities based on vocal frequency: PLoS ONE, v. 6, no. 11, art. E27052, 8 p., accessed November 1, 2023, at https://doi.org/10.1371/journal.pone.0027052.
Francis, C.D., Ortega, C.P., and Cruz, A., 2011c, Vocal frequency change reflects different responses to anthropogenic noise in two suboscine tyrant flycatchers: Proceedings of the Royal Society B—Biological Sciences, v. 278, no. 1714, p. 2025–2031, accessed September 22, 2022, at https://doi.org/10.1098/rspb.2010.1847.
Francis, C.D., Paritsis, J., Ortega, C.P., and Cruz, A., 2011d, Landscape patterns of avian habitat use and nest success are affected by chronic gas well compressor noise: Landscape Ecology, v. 26, no. 9, p. 1269–1280, accessed September 16, 2022, at https://doi.org/10.1007/s10980-011-9609-z.
Francis, C.D., Phillips, J.N., and Barber, J.R., 2023, Background acoustics in terrestrial ecology: Annual Review of Ecology, Evolution, and Systematics, v. 54, no. 1, p. 351–373, accessed November 9, 2023, at https://doi.org/10.1146/annurev-ecolsys-102220-030316.
Frid, A.F., and Dill, L.M., 2002, Human-caused disturbance stimuli as a form of predation risk: Conservation Ecology, v. 6, no. 1, art. 11, 16 p., accessed August 16, 2022, at https://doi.org/10.5751/ES-00404-060111.
Gilbert, M.M., and Chalfoun, A.D., 2011, Energy development affects populations of sagebrush songbirds in Wyoming: The Journal of Wildlife Management, v. 75, no. 4, p. 816–824, accessed September 19, 2022, at https://doi.org/10.1002/jwmg.123.
Gill, S.A., Job, J.R., Myers, K., Naghshineh, K., and Vonhof, M.J., 2015, Toward a broader characterization of anthropogenic noise and its effects on wildlife: Behavioral Ecology, v. 26, no. 2, p. 328–333, accessed March 22, 2022, at https://doi.org/10.1093/beheco/aru219.
Gomes, D.G.E., Francis, C.D., and Barber, J.R., 2021, Using the past to understand the present—Coping with natural and anthropogenic noise: BioScience, v. 71, no. 3, p. 223–234, accessed November 22, 2023, at https://doi.org/10.1093/biosci/biaa161.
Grubb, T.G., Pater, L.L., Gatto, A.E., and Delaney, D.K., 2013, Response of nesting northern goshawks to logging truck noise in northern Arizona: The Journal of Wildlife Management, v. 77, no. 8, p. 1618–1625, accessed July 21, 2022, at https://doi.org/10.1002/jwmg.607.
Habib, L., Bayne, E.M., and Boutin, S., 2007, Chronic industrial noise affects pairing success and age structure of ovenbirds Seiurus aurocapilla: Journal of Applied Ecology, v. 44, no. 1, p. 176–184, accessed July 13, 2022, at https://doi.org/10.1111/j.1365-2664.2006.01234.x.
Hamilton, L.E., Dale, B.C., and Paszkowski, C.A., 2011, Effects of disturbance associated with natural gas extraction on the occurrence of three grassland songbirds: Avian Conservation and Ecology, v. 6, no. 1, 19 p., accessed September 19, 2022, at https://doi.org/10.5751/ACE-00458-060107.
Harding, H.R., Gordon, T.A.C., Eastcott, E., Simpson, S.D., and Radford, A.N., 2019, Causes and consequences of intraspecific variation in animal responses to anthropogenic noise: Behavioral Ecology, v. 30, no. 6, p. 1501–1511, accessed March 30, 2022, at https://doi.org/10.1093/beheco/arz114.
Hays, J., McCawley, M., and Shonkoff, S.B.C., 2017, Public health implications of environmental noise associated with unconventional oil and gas development: Science of The Total Environment, v. 580, p. 448–456, accessed March 31, 2023, at https://doi.org/10.1016/j.scitotenv.2016.11.118.
Hayward, L.S., Bowles, A.E., Ha, J.C., and Wasser, S.K., 2011, Impacts of acute and long-term vehicle exposure on physiology and reproductive success of the northern spotted owl: Ecosphere, v. 2, no. 6, art. 65, p. 1–20, accessed July 21, 2022, at https://doi.org/10.1890/ES10-00199.1.
Heffner, H., Jr., and Heffner, H.E., 2010, The behavioral audiogram of whitetail [sic] deer (Odocoileus virginianus): The Journal of the Acoustical Society of America, v. 127, no. 3, p. EL111–EL114, accessed May 11, 2022, at https://doi.org/10.1121/1.3284546.
Heffner, R.S., Koay, G., and Heffner, H.E., 2001, Audiograms of five species of rodents—Implications for the evolution of hearing and the perception of pitch: Hearing Research, v. 157, no. 1–2, p. 138–152, accessed June 2, 2022, at https://doi.org/10.1016/S0378-5955(01)00298-2.
Hethcoat, M.G., and Chalfoun, A.D., 2015a, Energy development and avian nest survival in Wyoming, USA—A test of a common disturbance index: Biological Conservation, v. 184, p. 327–334, accessed September 19, 2022, at https://doi.org/10.1016/j.biocon.2015.02.009.
Hethcoat, M.G., and Chalfoun, A.D., 2015b, Towards a mechanistic understanding of human-induced rapid environmental change—A case study linking energy development, nest predation and predators: Journal of Applied Ecology, v. 52, no. 6, p. 1492–1499, accessed September 19, 2022, at https://doi.org/10.1111/1365-2664.12513.
Houser, D.S., Yost, W., Burkard, R., Finneran, J.J., Reichmuth, C., and Mulsow, J., 2017, A review of the history, development and application of auditory weighting functions in humans and marine mammals: The Journal of the Acoustical Society of America, v. 141, no. 3, p. 1371–1413, accessed December 1, 2022, at https://doi.org/10.1121/1.4976086.
Ikelheimer, B., and Plotkin, K., 2005, Noise Model Simulation (NMSim) user's manual: National Park Service, prepared by Wyle Laboratories, Arlington, Va., under contract no. 1443-CX2000-98-038, 123 p., accessed May 12, 2022, at https://nwtteis.com/portals/nwtteis/files/references/Ikelheimer_2004_NMSim_User_Manual.pdf.
Injaian, A.S., Taff, C.C., and Patricelli, G.L., 2018, Experimental anthropogenic noise impacts avian parental behaviour, nestling growth and nestling oxidative stress: Animal Behaviour, v. 136, p. 31–39, accessed June 23, 2023, at https://doi.org/10.1016/j.anbehav.2017.12.003.
International Organization for Standardization, 1996, Acoustics—Attenuation of sound during propagation outdoors—Part 2—General method of calculation: Geneva, Switzerland, International Organization for Standardization, 24 p., accessed June 29, 2023, at https://puc.sd.gov/commission/dockets/electric/2019/el19-003/KMExhibit9.pdf.
Ishizuka, T., and Fujiwara, K., 2004, Performance of noise barriers with various edge shapes and acoustical conditions: Applied Acoustics, v. 65, no. 2, p. 125–141, accessed August 22, 2022, at https://doi.org/10.1016/j.apacoust.2003.08.006.
Jackson, L.L., Heffner, R.S., and Heffner, H.E., 1999, Free-field audiogram of the Japanese macaque (Macaca fuscata): The Journal of the Acoustical Society of America, v. 106, no. 5, p. 3017–3023, accessed May 16, 2023, at https://doi.org/10.1121/1.428121.
Keyel, A.C., and Reed, S.E., 2017, Sound Mapping Tools—An ArcGIS toolbox for modeling the propagation of sounds in a wildland setting (ver. 4.4, June 2017): Fort Collins, Colo., Colorado State University, 50 p., accessed September 11, 2023, at https://docs.google.com/document/d/0B4ir69pS-kSTSmY0S3JUQ3Y5X1U/edit?resourcekey=0-lA0wacsHsZqJyFT2LDbtng.
Keyel, A.C., Reed, S.E., McKenna, M.F., and Wittemyer, G., 2017, Modeling anthropogenic noise propagation using the Sound Mapping Tools ArcGIS toolbox: Environmental Modelling & Software, v. 97, p. 56–60, accessed May 12, 2022, at https://doi.org/10.1016/j.envsoft.2017.07.008.
Keyel, A.C., Reed, S.E., Nuessly, K., Cinto-Mejia, E., Barber, J.R., and Wittemyer, G., 2018, Modeling anthropogenic noise impacts on animals in natural areas: Landscape and Urban Planning, v. 180, p. 76–84, accessed March 31, 2022, at https://doi.org/10.1016/j.landurbplan.2018.08.011.
Khan, J., Ketzel, M., Jensen, S.S., Gulliver, J., Thysell, E., and Hertel, O., 2021, Comparison of road traffic noise prediction models—CNOSSOS-EU, Nord2000 and TRANEX: Environmental Pollution, v. 270, art. 116240, 13 p., accessed May 3, 2023, at https://doi.org/10.1016/j.envpol.2020.116240.
Kight, C.R., and Swaddle, J.P., 2011, How and why environmental noise impacts animals—An integrative, mechanistic review: Ecology Letters, v. 14, no. 10, p. 1052–1061, accessed March 23, 2022, at https://doi.org/10.1111/j.1461-0248.2011.01664.x.
Kleist, N.J., and Enns, K.D., 2021, USGS BiblioSearch—A python tool to facilitate searching, cleaning, and compiling of literature citations from across multiple databases (ver. 1.0.0): U.S. Geological Survey software release, accessed June 5, 2023, at https://doi.org/10.5066/P9EW8BO5.
Kleist, N.J., Guralnick, R.P., Cruz, A., and Francis, C.D., 2016, Anthropogenic noise weakens territorial response to intruder's songs: Ecosphere, v. 7, no. 3, art. e01259, 11 p., accessed September 15, 2022, at https://doi.org/10.1002/ecs2.1259.
Kleist, N.J., Guralnick, R.P., Cruz, A., and Francis, C.D., 2017, Sound settlement—Noise surpasses land cover in explaining breeding habitat selection of secondary cavity‐nesting birds: Ecological Applications, v. 27, no. 1, p. 260–273, accessed March 31, 2022, at https://doi.org/10.1002/eap.1437.
Kleist, N.J., Guralnick, R.P., Cruz, A., Lowry, C.A., and Francis, C.D., 2018, Chronic anthropogenic noise disrupts glucocorticoid signaling and has multiple effects on fitness in an avian community: Proceedings of the National Academy of Sciences of the United States of America, v. 115, no. 4, p. E648–E657, accessed March 30, 2022, at https://doi.org/10.1073/pnas.1709200115.
Kociolek, A.V., Clevenger, A.P., St. Clair, C.C., and Proppe, D.S., 2011, Effects of road networks on bird populations: Conservation Biology, v. 25, no. 2, p. 241–249, accessed March 8, 2024, at https://doi.org/10.1111/j.1523-1739.2010.01635.x.
Koper, N., Leston, L., Baker, T.M., Curry, C., and Rosa, P., 2016, Effects of ambient noise on detectability and localization of avian songs and tones by observers in grasslands: Ecology and Evolution, v. 6, no. 1, p. 245–255, accessed December 1, 2022, at https://doi.org/10.1002/ece3.1847.
Kunc, H.P., and Schmidt, R., 2019, The effects of anthropogenic noise on animals—A meta-analysis: Biology Letters, v. 15, no. 11, art. 20190649, 5 p., accessed March 30, 2022, at https://doi.org/10.1098/rsbl.2019.0649.
Ludlow, S.M., Brigham, R.M., and Davis, S.K., 2015, Oil and natural gas development has mixed effects on the density and reproductive success of grassland songbirds: The Condor, v. 117, no. 1, p. 64–75, accessed September 19, 2022, at https://doi.org/10.1650/CONDOR-14-79.1.
Lynch, E., Joyce, D., and Fristrup, K., 2011, An assessment of noise audibility and sound levels in U.S. National Parks: Landscape Ecology, v. 26, no. 9, p. 1297–1309, accessed May 31, 2022, at https://doi.org/10.1007/s10980-011-9643-x.
Marín-Gómez, O.H., García-Arroyo, M., Sánchez-Sarria, C.E., Sosa-López, J.R., Santiago-Alarcon, D., and MacGregor-Fors, I., 2020, Nightlife in the city—Drivers of the occurrence and vocal activity of a tropical owl: Avian Research, v. 11, no. 1, art. 9, 14 p., accessed July 28, 2022, at https://doi.org/10.1186/s40657-020-00197-7.
Massachusetts Institute of Technology, 2011, The future of natural gas—An interdisciplinary MIT study: Cambridge, Mass., Massachusetts Institute of Technology, 287 p., accessed April 6, 2023, at https://energy.mit.edu/wp-content/uploads/2011/06/MITEI-The-Future-of-Natural-Gas.pdf.
Mason, J.T., McClure, C.J.W., and Barber, J.R., 2016, Anthropogenic noise impairs owl hunting behavior: Biological Conservation, v. 199, p. 29–32, accessed March 31, 2022, at https://doi.org/10.1016/j.biocon.2016.04.009.
McClure, C.J.W., Ware, H.E., Carlisle, J., Kaltenecker, G., and Barber, J.R., 2013, An experimental investigation into the effects of traffic noise on distributions of birds—Avoiding the phantom road: Proceedings of the Royal Society B—Biological Sciences, v. 280, no. 1773, art. 20132290, 9 p., accessed September 16, 2022, at https://doi.org/10.1098/rspb.2013.2290.
McClure, C.J.W., Ware, H.E., Carlisle, J.D., and Barber, J.R., 2017, Noise from a phantom road experiment alters the age structure of a community of migrating birds: Animal Conservation, v. 20, no. 2, p. 164–172, accessed September 16, 2022, at https://doi.org/10.1111/acv.12302.
Mennitt, D., Sherrill, K., and Fristrup, K., 2014, A geospatial model of ambient sound pressure levels in the contiguous United States: The Journal of the Acoustical Society of America, v. 135, no. 5, p. 2746–2764, accessed May 17, 2022, at https://doi.org/10.1121/1.4870481.
Naguib, M., and Wiley, R.H., 2001, Estimating the distance to a source of sound—Mechanisms and adaptations for long-range communication: Animal Behaviour, v. 62, no. 5, p. 825–837, accessed May 30, 2023, at https://doi.org/10.1006/anbe.2001.1860.
National Park Service [NPS], 2011, Special flight rules area in the vicinity of Grand Canyon National Park—Actions to substantially restore natural quiet: National Park Service draft environmental impact statement DES 10-60, v. 1, 674 p., accessed May 16, 2022, at https://parkplanning.nps.gov/document.cfm?parkID=65&projectID=28052&documentID=38849.
National Park Service [NPS], 2013a, Acoustical monitoring—Training manual: Fort Collins, Colo., U.S. Department of the Interior National Park Service, 100 p., accessed April 29, 2022, at https://www.nps.gov/subjects/sound/upload/NSNSDTrainingManual_AcousticalAmbientMonitoring-508.pdf.
National Park Service [NPS], 2013b, Yellowstone National Park—winter use plan / supplemental environmental impact statement: U.S. Department of the Interior National Park Service, 442 p., accessed May 12, 2022, at https://parkplanning.nps.gov/document.cfm?parkID=111&projectID=40806&documentID=51874.
National Park Service [NPS], 2015, Geospatial sound modeling: U.S. Department of the Interior National Park Service web page, accessed June 5, 2023, at https://irma.nps.gov/DataStore/Reference/Profile/2217356.
Nenninger, H.R., and Koper, N., 2018, Effects of conventional oil wells on grassland songbird abundance are caused by presence of infrastructure, not noise: Biological Conservation, v. 218, p. 124–133, accessed July 13, 2022, at https://doi.org/10.1016/j.biocon.2017.11.014.
Ng, C.S., Des Brisay, P.G., and Koper, N., 2019, Chestnut-collared longspurs reduce parental care in the presence of conventional oil and gas development and roads: Animal Behaviour, v. 148, p. 71–80, accessed September 16, 2022, at https://doi.org/10.1016/j.anbehav.2018.12.001.
Northrup, J.M., Anderson, C.R., Jr., Gerber, B.D., and Wittemyer, G., 2021, Behavioral and demographic responses of mule deer to energy development on winter range: Wildlife Monographs, v. 208, no. 1, p. 1–37, accessed April 11, 2022, at https://doi.org/10.1002/wmon.1060.
Northrup, J.M., and Wittemyer, G., 2013, Characterising the impacts of emerging energy development on wildlife, with an eye towards mitigation: Ecology Letters, v. 16, no. 1, p. 112–125, accessed April 13, 2022, at https://doi.org/10.1111/ele.12009.
Ortega, C.P., 2012, Chapter 2—Effects of noise pollution on birds—A brief review of our knowledge: Ornithological Monographs, no. 74, p. 6–22, accessed April 22, 2022, at https://doi.org/10.1525/om.2012.74.1.6.
Palmer, A.G., Nordmeyer, D.L., and Roby, D.D., 2003, Effects of jet aircraft overflights on parental care of peregrine falcons: Wildlife Society Bulletin, v. 31, no. 2, p. 499–509, accessed July 21, 2022, at https://www.jstor.org/stable/3784331.
Pater, L.L., Grubb, T.G., and Delaney, D.K., 2009, Recommendations for improved assessment of noise impacts on wildlife: The Journal of Wildlife Management, v. 73, no. 5, p. 788–795, accessed August 19, 2022, at https://doi.org/10.2193/2006-235.
Patricelli, G.L., Blickley, J.L., and Hooper, S.L., 2013, Recommended management strategies to limit anthropogenic noise impacts on greater sage-grouse in Wyoming: Human–Wildlife Interactions, v. 7, no. 2, p. 230–249, accessed April 28, 2022, at https://doi.org/10.26077/7qfc-6d14.
Quinn, J.L., Whittingham, M.J., Butler, S.J., and Cresswell, W., 2006, Noise, predation risk compensation and vigilance in the chaffinch Fringilla coelebs: Journal of Avian Biology, v. 37, no. 6, p. 601–608, accessed July 19, 2023, at https://doi.org/10.1111/j.2006.0908-8857.03781.x.
Radtke, C., Autenrieth, D.A., Lipsey, T., and Brazile, W.J., 2017, Noise characterization of oil and gas operations: Journal of Occupational and Environmental Hygiene, v. 14, no. 8, p. 659–667, accessed March 28, 2023, at https://doi.org/10.1080/15459624.2017.1316386.
Reed, S.E., Boggs, J.L., and Mann, J.P., 2012, A GIS tool for modeling anthropogenic noise propagation in natural ecosystems: Environmental Modelling & Software, v. 37, p. 1–5, accessed May 12, 2022, at https://doi.org/10.1016/j.envsoft.2012.04.012.
Rice, W.R., 1982, Acoustical location of prey by the marsh hawk—Adaptation to concealed prey: The Auk, v. 99, no. 3, p. 403–413, accessed August 29, 2022, at https://doi.org/10.1093/auk/99.3.403.
Roca, I.T., Desrochers, L., Giacomazzo, M., Bertolo, A., Bolduc, P., Deschesnes, R., Martin, C.A., Rainville, V., Rheault, G., and Proulx, R., 2016, Shifting song frequencies in response to anthropogenic noise—A meta-analysis on birds and anurans: Behavioral Ecology, v. 27, no. 5, p. 1269–1274, accessed September 21, 2022, at https://doi.org/10.1093/beheco/arw060.
Rosa, P., and Koper, N., 2022, Impacts of oil well drilling and operating noise on abundance and productivity of grassland songbirds: Journal of Applied Ecology, v. 59, no. 2, p. 574–584, accessed September 16, 2022, at https://doi.org/10.1111/1365-2664.14075.
Rutherford, T.K., Maxwell, L.M., Kleist, N.J., Teige, E.C., Lehrter, R.J., Gilbert, M.A., Wood, D.J.A., Johnston, A.N., Mengelt, C., Tull, J.C., Haby, T.S., and Carter, S.K., 2023, Effects of noise from oil and gas development on ungulates and small mammals—A science synthesis to inform National Environmental Policy Act analyses: U.S. Geological Survey Scientific Investigations Report 2023–5114, 44 p., accessed March 8, 2024, at https://doi.org/10.3133/sir20235114.
Ryan, C.M., Cerveny, L.K., Robinson, T.L., and Blahna, D.J., 2018, Implementing the 2012 forest planning rule—Best available scientific information in forest planning assessments: Forest Science, v. 64, no. 2, p. 159–169, accessed March 23, 2022, at https://doi.org/10.1093/forsci/fxx004.
Sánchez, N.V., Sandoval, L., Hedley, R.W., St. Clair, C.C., and Bayne, E.M., 2022, Relative importance for Lincoln’s sparrow (Melospiza lincolnii) occupancy of vegetation type versus noise caused by industrial development: Frontiers in Ecology and Evolution, v. 10, art. 810087, 15 p., accessed July 13, 2022, at https://doi.org/10.3389/fevo.2022.810087.
Sawyer, H., Kauffman, M.J., and Nielson, R.M., 2009, Influence of well pad activity on winter habitat selection patterns of mule deer: The Journal of Wildlife Management, v. 73, no. 7, p. 1052–1061, accessed April 7, 2022, at https://doi.org/10.2193/2008-478.
Sawyer, H., Korfanta, N.M., Nielson, R.M., Monteith, K.L., and Strickland, D., 2017, Mule deer and energy development—Long-term trends of habituation and abundance: Global Change Biology, v. 23, no. 11, p. 4521–4529, accessed April 13, 2022, at https://doi.org/10.1111/gcb.13711.
Scobie, C., Bayne, E., and Wellicome, T., 2014, Influence of anthropogenic features and traffic disturbance on burrowing owl diurnal roosting behavior: Endangered Species Research, v. 24, no. 1, p. 73–83, accessed July 28, 2022, at https://doi.org/10.3354/esr00577.
Scobie, C.A., Bayne, E.M., and Wellicome, T.I., 2016, Influence of human footprint and sensory disturbances on night-time space use of an owl: Endangered Species Research, v. 31, p. 75–87, accessed May 3, 2022, at https://doi.org/10.3354/esr00756.
Seavy, N.E., and Howell, C.A., 2010, How can we improve information delivery to support conservation and restoration decisions?: Biodiversity and Conservation, v. 19, no. 5, p. 1261–1267, accessed May 6, 2022, at https://doi.org/10.1007/s10531-009-9752-x.
Senzaki, M., Barber, J.R., Phillips, J.N., Carter, N.H., Cooper, C.B., Ditmer, M.A., Fristrup, K.M., McClure, C.J.W., Mennitt, D.J., Tyrrell, L.P., Vukomanovic, J., Wilson, A.A., and Francis, C.D., 2020, Sensory pollutants alter bird phenology and fitness across a continent: Nature, v. 587, no. 7835, p. 605–609, accessed July 22, 2022, at https://doi.org/10.1038/s41586-020-2903-7.
Senzaki, M., Yamaura, Y., Francis, C.D., and Nakamura, F., 2016, Traffic noise reduces foraging efficiency in wild owls: Scientific Reports, v. 6, art. 30602, 7 p., accessed April 1, 2022, at https://doi.org/10.1038/srep30602.
Shannon, G., McKenna, M.F., Angeloni, L.M., Crooks, K.R., Fristrup, K.M., Brown, E., Warner, K.A., Nelson, M.D., White, C., Briggs, J., McFarland, S., and Wittemyer, G., 2016, A synthesis of two decades of research documenting the effects of noise on wildlife: Biological Reviews, v. 91, no. 4, p. 982–1005, accessed March 21, 2022, at https://doi.org/10.1111/brv.12207.
Shonfield, J., and Bayne, E.M., 2017, The effect of industrial noise on owl occupancy in the boreal forest at multiple spatial scales: Avian Conservation & Ecology, v. 12, no. 2, art. 13, 14 p., accessed July 21, 2022, at https://doi.org/10.5751/ACE-01042-120213.
Silva, C.C., Lourenço, R., Godinho, S., Gomes, E., Sabino-Marques, H., Medinas, D., Neves, V., Silva, C., Rabaça, J.E., and Mira, A., 2012, Major roads have a negative impact on the tawny owl Strix aluco and the little owl Athene noctua populations: Acta Ornithologica, v. 47, no. 1, p. 47–54, accessed July 28, 2022, at https://doi.org/10.3161/000164512X653917.
Slabbekoorn, H., and Ripmeester, E.A., 2008, Birdsong and anthropogenic noise—Implications and applications for conservation: Molecular Ecology, v. 17, no. 1, p. 72–83, accessed September 21, 2022, at https://doi.org/10.1111/j.1365-294X.2007.03487.x.
Sordello, R., Ratel, O., Flamerie De Lachapelle, F., Leger, C., Dambry, A., and Vanpeene, S., 2020, Evidence of the impact of noise pollution on biodiversity—A systematic map: Environmental Evidence, v. 9, no. 1, art. 20, 27 p., accessed March 31, 2022, at https://doi.org/10.1186/s13750-020-00202-y.
Sorrels, J.L., Coburn, J., Bradley, K., and Randall, D., 2019, Flares, chap. 1 of Section 3.2—VOC destruction controls of Section 3—VOC controls of EPA air pollution control cost manual (7th ed.): Washington, D.C., U.S. Environmental Protection Agency, 71 p., accessed July 10, 2023, at https://www.epa.gov/economic-and-cost-analysis-air-pollution-regulations/cost-reports-and-guidance-air-pollution.
Stinchcomb, T.R., Brinkman, T.J., and Betchkal, D., 2020, Extensive aircraft activity impacts subsistence areas—Acoustic evidence from Arctic Alaska: Environmental Research Letters, v. 15, no. 11, art. 115005, 10 p., accessed April 14, 2022, at https://doi.org/10.1088/1748-9326/abb7af.
Strasser, E.H., and Heath, J.A., 2013, Reproductive failure of a human-tolerant species, the American kestrel, is associated with stress and human disturbance: Journal of Applied Ecology, v. 50, no. 4, p. 912–919, accessed July 28, 2022, at https://doi.org/10.1111/1365-2664.12103.
Sutter, G.C., Davis, S.K., Skiffington, J.C., Keating, L.M., and Pittaway, L.A., 2016, Nesting behaviour and reproductive success of Sprague’s pipit (Anthus spragueii) and vesper sparrow (Pooecetes gramineus) during pipeline construction: The Canadian Field-Naturalist, v. 130, no. 2, p. 99–109, accessed September 16, 2022, at https://doi.org/10.22621/cfn.v130i2.1833.
Sweet, K.A., Sweet, B.P., Gomes, D.G.E., Francis, C.D., and Barber, J.R., 2022, Natural and anthropogenic noise increase vigilance and decrease foraging behaviors in song sparrows: Behavioral Ecology, v. 33, no. 1, p. 288–297, accessed November 22, 2023, at https://doi.org/10.1093/beheco/arab141.
Thompson, S.J., Johnson, D.H., Niemuth, N.D., and Ribic, C.A., 2015, Avoidance of unconventional oil wells and roads exacerbates habitat loss for grassland birds in the North America Great Plains: Biological Conservation, v. 192, p. 82–90, accessed September 19, 2022, at https://doi.org/10.1016/j.biocon.2015.08.040.
Tiemann, M., and Vann, A., 2015, Hydraulic fracturing and Safe Drinking Water Act regulatory issues: Washington, D.C., Congressional Research Service Report R41760, 34 p., accessed March 29, 2023, at https://crsreports.congress.gov/product/pdf/R/R41760.
Tull, J.C., 2015, Acoustic impacts and greater sage-grouse—A review of current science, sound measurement protocols, and management recommendations: Nevada Department of Wildlife Habitat Division, 12 p. [Also available at https://wgfd.wyo.gov/media/14766/download?inline.]
U.S. Department of the Interior and U.S. Department of Agriculture, 2007, Surface operating standards and guidelines for oil and gas exploration and development (4th ed.): Denver, Colo., Bureau of Land Management BLM/WO/ST-06/021+3071/REV 07, 84 p., accessed March 8, 2024, at https://www.blm.gov/sites/blm.gov/files/Gold%20Book%202007%20Revised.pdf.
U.S. Department of Agriculture Forest Service, 2015a, Greater sage-grouse record of decision for Idaho and southwest Montana, Nevada and Utah: U.S. Department of Agriculture Forest Service, 272 p., accessed April 28, 2022, at https://www.fs.usda.gov/sites/default/files/sage-grouse-great-basin-rod.pdf.
U.S. Department of Agriculture Forest Service, 2015b, Greater sage-grouse record of decision for northwest Colorado and Wyoming: U.S. Department of Agriculture Forest Service, 182 p., accessed April 28, 2022, at https://www.fs.usda.gov/sites/default/files/sage-grouse-rocky-mountain-rod.pdf.
Van Pelt, W.E., Kyle, S., Pitman, J., Klute, D., Beauprez, G., Shoeling, D., Janus, A., and Haufler, J., 2013, The lesser prairie-chicken range-wide conservation plan: Cheyenne, Wyo., Western Association of Fish and Wildlife Agencies, 367 p., accessed December 2, 2022, at https://eplanning.blm.gov/public_projects/2021616/200533749/20078003/250084185/Exhibit%20219%20-%20LPC%20rangewide%20conservation%20plan.pdf.
Wäldchen, J., and Mäder, P., 2018, Plant species identification using computer vision techniques—A systematic literature review: Archives of Computational Methods in Engineering, v. 25, no. 2, p. 507–543, accessed March 8, 2024, at https://doi.org/10.1007/s11831-016-9206-z.
Walker, B.L., Neubaum, M.A., Goforth, S.R., and Flenner, M.M., 2020, Quantifying habitat loss and modification from recent expansion of energy infrastructure in an isolated, peripheral greater sage-grouse population: Journal of Environmental Management, v. 255, art. 109819, 15 p., accessed June 9, 2022, at https://doi.org/10.1016/j.jenvman.2019.109819.
Ware, H.E., McClure, C.J.W., Carlisle, J.D., and Barber, J.R., 2015, A phantom road experiment reveals traffic noise is an invisible source of habitat degradation: Proceedings of the National Academy of Sciences of the United States of America, v. 112, no. 39, p. 12105–12109, accessed September 16, 2022, at https://doi.org/10.1073/pnas.1504710112.
Warrington, M.H., Curry, C.M., Antze, B., and Koper, N., 2018, Noise from four types of extractive energy infrastructure affects song features of Savannah sparrows: The Condor, v. 120, no. 1, p. 1–15, accessed September 24, 2022, at https://doi.org/10.1650/CONDOR-17-69.1.
Williams, D.P., Avery, J.D., Gabrielson, T.B., and Brittingham, M.C., 2021, Experimental playback of natural gas compressor noise reduces incubation time and hatching success in two secondary cavity-nesting bird species: Ornithological Applications, v. 123, no. 1, 11 p., accessed September 14, 2022, at https://doi.org/10.1093/ornithapp/duaa066.
Witter, R.Z., McKenzie, L., Stinson, K.E., Scott, K., Newman, L.S., and Adgate, J., 2013, The use of health impact assessment for a community undergoing natural gas development: American Journal of Public Health, v. 103, no. 6, p. 1002–1010, accessed March 31, 2023, at https://doi.org/10.2105/AJPH.2012.301017.
Wyborn, C., Louder, E., Harrison, J., Montambault, J., Montana, J., Ryan, M., Bednarek, A., Nesshöver, C., Pullin, A., Reed, M., Dellecker, E., Kramer, J., Boyd, J., Dellecker, A., and Hutton, J., 2018, Understanding the impacts of research synthesis: Environmental Science & Policy, v. 86, p. 72–84, accessed April 5, 2022, at https://doi.org/10.1016/j.envsci.2018.04.013.
Zammerilli, A., Murray, R.C., Davis, T., and Littlefield, J., 2014, Environmental impacts of unconventional natural gas development and production: U.S. Department of Energy National Energy Technology Laboratory, prepared by Booz Allen Hamilton, Inc., Energy Sector Planning and Analysis under contract no. DE-FE0004001, 139 p.
Glossary
acoustic environment
“sound at the receiver from all sound sources as modified by the environment.” (NPS, 2013a, p. 94)
background sound level
“the [sound] level in a given environment without contributions from the source of interest.” (Ambrose and others, 2021, p. 25)
completion
“The installation of permanent wellhead equipment for the production of oil and gas.” (Colorado Oil and Gas Conservation Commission, 2022)
decibel
“a logarithmic measure of acoustic intensity, calculated by 10 log10 (sound intensity/reference sound intensity).” (Barber and others, 2010, p. 180)
decibel weighting
“auditory weighting functions transform sound measurements to take into account the frequency-dependent aspects of auditory sensitivity. They are mathematical functions used to emphasize frequencies where animals (human and non-human) are more sensitive and de-emphasize frequencies where animals are less sensitive.” (Houser and others, 2017, p. 1371–1372)
drilling
“The using of a rig and crew for the drilling, suspension, completion, production testing, capping, plugging and abandoning, deepening, plugging back, sidetracking, redrilling or reconditioning of a well (except routine cleanout and pump or rod pulling operations) or the converting of a well to a source, injection, observation, or producing well, and including stratigraphic tests. Also includes any related environmental studies. Associated costs include completion costs but do not include equipping costs.” (Colorado Oil and Gas Conservation Commission, 2022)
equivalent sound level (Leq)
“the logarithmic average (i.e., on an energy basis) of sound pressure levels over a specific time period. ‘Energy averaged’ sound levels are logarithmic values, and as such are generally much higher than arithmetic averages. Leq values are typically calculated for a specific time period (1-hour and 12-hour time periods are often used). Leq values are computed from all of the 1-second Leq values for the specific time period. Leq must be used carefully in quantifying natural ambient sound levels because occasional loud sound levels may heavily influence (increase) the Leq value, even though sound levels for that period of time are typically lower.” (Ambrose and Florian, 2014, p. 13–14)
exceedance percentile (Lx)
“this metric is the sound pressure level (L), in decibels, exceeded x percent of the time for the specified measurement period. L50 is the sound pressure level exceeded 50 percent of the time (L50 is the same as the median).” (Ambrose and Florian, 2014, p. 14)
exploration phase
“The phase of operations which covers the search for oil and gas by carrying out detailed geological and geophysical surveys followed up where appropriate by exploratory drilling.” (Colorado Oil and Gas Conservation Commission, 2022)
field
“A geographical area under which an oil or gas reservoir lies.” (Colorado Oil and Gas Conservation Commission, 2022)
final reclamation
“To achieve final reclamation of a recently drilled dry hole, the well site must be recontoured to original contour or a contour that blends with the surrounding landform, stockpiled topsoil evenly redistributed, and the site revegetated. To achieve final reclamation of a formerly producing well, all topsoil and vegetation must be restriped from all portions of the old well site that were not previously reshaped to blend with the surrounding contour. All disturbed areas are then recontoured back to the original contour or a contour that blends with the surrounding landform, topsoil is redistributed, and the site revegetated.” (U.S. Department of the Interior and U.S. Department of Agriculture, 2007, p. 46–47)
flaring
“A high-temperature oxidation process used to burn waste gases containing combustible components such as volatile organic compounds (VOCs), natural gas (or methane), carbon monoxide (CO), and hydrogen (H2). The waste gases are piped to a remote, usually elevated location, and burned in an open flame in ambient air using a specially designed burner tip, auxiliary fuel, and, in some cases, assist gases like steam or air to promote mixing for nearly complete (e.g. [for example], ≥ 98%) destruction of the combustible components in the waste gas.” (Sorrels and others, 2019, chap. 1, p. 1)
fracturing
“A method of breaking down a formation by pumping fluid at very high pressures. The objective is to increase production rates from a reservoir.” (Colorado Oil and Gas Conservation Commission, 2022)
frequency
“for a periodic signal, the maximum number of times per second that a segment of the signal is duplicated. For a sinusoidal signal, the number of cycles (the number of pressure peaks) in one second (Hz). Frequency equals the speed of sound (~340 ms-1) divided by wavelength.” (Barber and others, 2010, p. 180)
interim reclamation
“Interim reclamation consists of minimizing the footprint of disturbance by reclaiming all portions of the well site not needed for production operations. The portions of the cleared well site not needed for operational and safety purposes are recontoured to a final or intermediate contour that blends with the surrounding topography as much as possible. Sufficient level area remains for setup of a workover rig and to park equipment.” (U.S. Department of the Interior and U.S. Department of Agriculture, 2007, p. 45)
Lmax
“the maximum sound pressure level for a given period.” (Ambrose and Florian, 2014, p. 14)
Lmin
“the minimum sound pressure level for a given period.” (Ambrose and Florian, 2014, p. 14)
masking
“the amount or the process by which the threshold of detection for a sound is increased by the presence of the aggregate of other sounds.” (Barber and others, 2010, p. 180)
noise
“(a) Undesired sound. By extension, noise is any unwanted disturbance within a useful frequency band, such as undesired electric waves in a transmission channel or device. (b) Erratic, intermittent, or statistically random oscillation.” (Acoustical Society of America, undated, sec. 2.32)
sound
“(a) Oscillation in pressure, stress, particle displacement, particle velocity, etc., propagated in a medium with internal forces (e.g., elastic or viscous), or the superposition of such propagated oscillation. (b) Auditory sensation evoked by the oscillation described in (a).” (Acoustical Society of America, undated, sec. 2.01)
sound exposure level
“A level quantity often used for auditory damage risk criteria ***. This metric takes both level and duration of sound into account. Sound exposure is the time integral of the square of the instantaneous sound pressure.” (Houser and others, 2017, p. 1375)
sound power
“For a specified sound source, sound energy radiated per unit of time. Unit, watt (W); symbols, W or P.” (Acoustical Society of America, undated, sec. 2.71)
sound power level
“Ten times the logarithm to the base ten of the ratio of a given sound power, in a stated frequency band, to the reference value for sound power.” (Acoustical Society of America, undated, sec. 3.07)
sound pressure
“sound pressure is the instantaneous difference between the actual pressure produced by a sound wave and the average barometric pressure at a given point in space. Not all pressure fluctuations detected by a microphone are sound (e.g., wind over the microphone). Sound pressure is measured in Pascals (Pa), Newtons per square meter, which is the metric equivalent of pounds per square inch.” (Ambrose and Florian, 2014, p. 14)
sound pressure level
“the logarithmic form of sound pressure. Generally, sound pressure level refers to unweighted sound pressure levels of one-third octave bands.” (Ambrose and Florian, 2014, p. 14)
surface reclamation
“A restoration of the surface as for productivity or usefulness.” (Colorado Oil and Gas Conservation Commission, 2022)
Appendix 1. Results of Studies About the Effects of Noise From Oil and Gas Development On Raptors
Table 1.1.
Brief findings from studies about the effects of noise from oil and gas development on species in the family Strigidae (owls).[Findings from each study that are not specific to the effects of noise are not included in the table. dBA, A-weighted decibel; %, percent; ~, about; km2, square kilometer; dB, decibel; m, meter; >, greater than; <, less than; NA, not applicable]
Noise type and description | Study system and geographic location | Relevant study findings | Intensity of effect | Reference |
---|---|---|---|---|
Researchers simulated oil and gas well compressor noise between 46 and 73 dBA. | Experimental conditions in field-placed flight tent | Increased noise levels decreased hunting success, prey detection, prey strike, and prey capture per strike. | The odds of a successful hunt decreased by 8% per 1 dBA increase. No hunting successes occurred in noise conditions >61 dBA. | Mason and others (2016) |
Researchers observed noise in an active oil and gas field. At the site scale (~2.5 km2 with a well pad in the center), noise treatments were categorical and included sites with chronic noise from compressors or oil processing plants, averaging ~82 dB and sites with intermittent noise from road traffic, averaging ~77 dB. Control sites had no human-caused noise, averaging ~74 dB. At the scale of an individual noise recording unit (radius of 800 m), sound level was analyzed as a continuous variable. | Upland boreal forest (Alberta, Canada) | Noise did not affect occupancy (measured at the site scale) or habitat use (measured at the recording unit scale). Increased noise was associated with a decrease in owl detection probability at the site and recording unit scales. | Effects are assumed to be long-term because the study occurred at sites subject to chronic noise. Detection probability decreased from >50% to <25% from quiet sites to chronic noise sites and from recording unit locations with <60 dB to locations with >110 dB. | Shonfield and Bayne (2017) |
Researchers observed sound levels at owl locations in an active oil and gas field containing compressor stations, drilling machinery, and traffic noise. Noise was analyzed as a continuous variable. | Mixed-grass prairie (Alberta, Saskatchewan, Canada) | Surface disturbance and infrastructure were stronger predictors of owl space use than noise. Noise did not affect home range size. | Effects are assumed to be long-term because the study occurred in an oil and gas field subject to chronic noise. | Scobie and others (2016) |
Researchers observed noise in an active oil and gas field. At the site scale (~2.5 km2 with a well pad in the center), noise treatments were categorical and included sites with chronic noise from compressors or oil processing plants, averaging ~82 dB and sites with intermittent noise from road traffic, averaging ~77 dB. Control sites had no anthropogenic noise, averaging ~74 dB. At the scale of an individual noise recording unit (radius of 800 m), sound level was analyzed as a continuous variable. | Upland boreal forest (Alberta, Canada) | Noise did not affect occupancy (measured at the site scale), habitat use (measured at the recording unit scale), or detection probability (measured at both scales). | NA | Shonfield and Bayne (2017) |
Researchers observed noise in an active oil and gas field. At the site scale (~2.5 km2 with a well pad in the center), noise treatments were categorical and included sites with chronic noise from compressors or oil processing plants, averaging ~82 dB and sites with intermittent noise from road traffic, averaging ~77 dB. Control sites had no anthropogenic noise, averaging ~74 dB. At the scale of an individual noise recording unit (radius of 800 m), sound level was analyzed as a continuous variable. | Upland boreal forest (Alberta, Canada) | Noise did not affect occupancy (measured at the site scale) or habitat use (measured at the recording unit scale). Noise was associated with a slight decline in detection probability at the scale of individual recording units. | Effects are assumed to be long-term because the study occurred at sites subject to chronic noise. Recording units did not detect individuals in areas averaging more than 93 dB. | Shonfield and Bayne (2017) |
Appendix 2. Results of Studies About the Effects of Noise From Oil and Gas Development On Songbirds and Other Small Avian Species
Table 2.1.
Brief findings from studies about the effects of noise from oil and gas development on avian communities in boreal forest, Pinus spp. (pinyon)-Juniperus spp. (juniper), and Artemisia spp. (sagebrush) habitats.[Findings from each study that are not specific to the effects of noise are not included in the table. m, meter; dBA, A-weighted decibel; ha, hectare; %, percent; km2, square kilometer]
Noise type and description | Study system and geographic location | Relevant study findings | Intensity of effect | Reference |
---|---|---|---|---|
Researchers observed noise in an active oil and gas field. The study included four noise categories: sound levels near to (100–300 m) and far from (400–700 m) compressor stations and quiet well pads. The average noise level near compressor stations was 48 dBA. Sound levels were not reported for quiet well pads or point count locations far from compressors. | Upland boreal forest (Alberta, Canada) | The density of the passerine community was lowest at sites near compressor stations. | Effects are assumed to be long-term because the study occurred at sites subject to chronic noise. Density was 19 birds per 10 ha at sites near compressors, 22 birds per 10 ha at sites far from compressors, 32 birds per 10 ha at sites near quiet well pads, and 29 birds per 10 ha at sites far from quiet well pads. | Bayne and others (2008) |
Researchers observed noise near well pads with and without compressors in an active oil and gas field. Sound levels were not reported. Noise was analyzed as a categorical and continuous variable. | Pinyon-juniper woodlands and shrublands (New Mexico) | Noise did not affect community nest density. Sites with compressors had lower nesting species richness and different species composition than quiet well pads. Nest sites were farther from the well pad at study sites with compressors than at quiet well pads. Daily nest survival was higher at sites with compressors than quiet well pads. Sites with compressors had less nest predation than quiet will pads, and increases in noise level were associated with lower daily nest predation. | Effects are assumed to be long-term because the study occurred at sites subject to chronic noise. Thirty-two species nested at quiet well pad sites and 14 species were unique to quiet well pad sites, whereas 21 species nested at sites with compressors, and 3 species were unique to sites with compressors. Nest sites were an average of 222 m from the well pad at study sites with compressors and an average of 201 m from the well pad at quiet well pad study sites. Predation caused nest failure at 32% of nests at quiet well pad sites and 13% of sites with compressors. Daily nest survival was predicted to be 22% higher at sites with compressors compared to quiet well pad sites across a 23-day nest cycle. | Francis and others (2009) |
Researchers simulated natural gas compressor noise at 6–8 treatment sites and compared the effects to 6–7 control sites across a 100 km2 study area. Noise treatments were broadband 50 m from the sound source (average 56 dBA), broadband 250 m from the sound source (average 46 dBA), narrowband 50 m from the sound source (average 58 dBA), and narrowband 250 m from the sound source (average 40 dBA). Average sound levels at control sites ranged from 31 to 37 dBA. Noise was analyzed as a categorical and continuous variable. | Sagebrush steppe (Idaho) | Abundance was lower at sites subject to broadband treatments than at control sites. Abundance decreased with increased noise levels at broadband sites. | The effect was measured for the duration of the experiment, which occurred during the breeding season in 2 consecutive years. Abundance was 20% lower at broadband sites at 50 m and 250 m combined compared to control sites. Noise was associated with an 8% reduction in abundance per 9 dBA at broadband sites. | Cinto Mejia and others (2019) |
Table 2.2.
Brief findings from studies about the effects of noise from oil and gas development on Psaltriparus minimus (bushtit; family Aegithalidae).[Findings from each study that are not specific to the effects of noise are not included in the table. dBA, A-weighted decibel]
Noise type and description | Study system and geographic location | Relevant study findings | Intensity of effect | Reference |
---|---|---|---|---|
Researchers observed noise near well pads with (range of 46–68 dBA) and without (range of 32–46 dBA) compressors in an active oil and gas field. Noise was analyzed as a categorical variable. | Pinus spp. (pinyon)-Juniperus spp. (juniper) woodlands and shrublands (New Mexico) | Noise did not affect abundance. The number of nests was higher on sites with compressors compared to quiet well pads. | Effects are assumed to be long-term because the study occurred at sites subject to chronic noise. | Francis and others (2011b) |
Table 2.3.
Brief findings from studies about the effects of noise from oil and gas development on Eremophila alpetrsi (horned lark; family Alaudidae).[Findings from each study that are not specific to the effects of noise are not included in the table. km2, square kilometer; dBA, A-weighted decibel; m, meter; NA, not applicable]
Noise type and description | Study system and geographic location | Relevant study findings | Intensity of effect | Reference |
---|---|---|---|---|
Researchers simulated natural gas compressor noise at 6–8 treatment sites and compared the effects to 6–7 control sites across a 100 km2 study area. Noise treatments were broadband 50 m from the sound source (average 56 dBA), broadband 250 m from the sound source (average 46 dBA), narrowband 50 m from the sound source (average 58 dBA), and narrowband 250 m from the sound source (average 40 dBA). Average sound levels at control sites ranged from 31 to 37 dBA. Noise was analyzed as a categorical and continuous variable. | Artemisia spp. (sagebrush) steppe (Idaho) | Noise did not affect abundance. | NA | Cinto Mejia and others (2019) |
Table 2.4.
Brief findings from studies about the effects of noise from oil and gas development on Calcarius ornatus (chestnut-collared longspur; family Calcariidae).[Findings from each study that are not specific to the effects of noise are not included in the table. dBC, C-weighted decibel; m, meter; NA, not applicable; h/day, hour per day; %, percent; ha, hectares]
Noise type and description | Study system and geographic location | Relevant study findings | Intensity of effect | Reference |
---|---|---|---|---|
Researchers observed noise at active well and gas compressor sites and control sites. Noise was estimated to range from 68 dBC to 78 dBC at sites with generator-powered pumpjacks, 59 to 65 dBC at sites with grid-powered pumpjacks, 75.5 to 82.5 dBC at sites with generator-powered screwpumps, 57 to 61 dBC at sites with grid-powered screwpumps, and 79 to 85 dBC at sites with compressor stations. Control sites were quiet, inactive well pads that had sound levels estimated to range from 47 to 57 dBC. | Mixed-grass prairie (Alberta, Canada) | Noise treatment (active sites) did not affect nesting success. | NA | Bernath-Plaisted and Koper (2016) |
Researchers observed noise at active well sites and control sites. Noise was estimated to range from 62 to 73 dBC 10 m from wells. Control sites were quiet, inactive well pads. Sound levels were not reported for control sites. | Mixed-grass prairie (Alberta, Canada) | Noise treatment (active wells) did not affect abundance. | NA | Nenninger and Koper (2018) |
Researchers observed noise at active well and compressor station sites and control sites. Noise levels averaged 61–81 dBC 10 m from wells or compressors. Control sites were quiet, inactive well pads that had sound levels ranging from 47 to 57 dBC. | Mixed-grass prairie (Alberta, Canada) | Nocturnal attentiveness was lower at active sites compared to control sites. Males mate-provisioned more often at active sites compared to control sites. Males provisioned nestlings less often at active sites compared to control sites. Noise did not affect other indicators of nest attentiveness or nest success. | Effects are assumed to be long-term because the study occurred at sites subject to chronic noise. | Ng and others (2019) |
Researchers simulated three types of well pad noise at treatment sites and compared the effects to two types of control sites. Treatments included simulated drilling noise (84–92 dBC 10 m from speaker) broadcasted 24 h/day for two 10-day periods, simulated generator-powered pumpjack noise (68–78 dBC 10 m from speaker) broadcasted for 24 h/day for approximately 95 days, and simulated grid-powered pumpjacks (59–65 dBC 10 m from speaker) broadcasted 24 h/day for approximately 87 days. Control sites for noise treatments were quiet, infrastructure-only sites or sites without infrastructure (48–54 dBC). | Mixed-grass prairie (Alberta, Canada) | Abundance decreased near simulated drilling and grid-powered pumpjacks. Noise simulations did not affect nest success. Nestling body condition was greater at all noise sites and quiet infrastructure control sites compared to control sites without infrastructure across scales. Clutch sizes were larger at sites with simulated drilling noise and grid-powered pumpjack noise. | The effects of duration of noise (10-day drilling noise simulations compared to 87- and 95-day production noise simulations) were not described. Abundance declined approximately 30% with increased proximity to simulated drilling and grid-powered pumpjack noise relative to quiet infrastructure sites. Abundance effects occurred in the immediate vicinity of simulated noise but not at the site scale (64.7 ha). Clutch sizes averaged 5.5 eggs near simulated drilling noise and 4 eggs at quiet infrastructure sites and control sites without infrastructure. | Rosa and Koper (2022) |
Table 2.5.
Brief findings from studies about the effects of noise from oil and gas development on species in the family Cardinalidae (cardinals and grosbeaks).[Findings from each study that are not specific to the effects of noise are not included in the table. m, meter; dBA, A-weighted decibel]
Noise type and description | Study system and geographic location | Relevant study findings | Intensity of effect | Reference |
---|---|---|---|---|
Researchers observed noise in an active oil and gas field. The study included four noise categories: sound levels near to (100–300 m) and far from (400–700 m) compressor stations and quiet well pads. The average noise level near compressor stations was 48 dBA. Sound levels were not reported for quiet well pads or point count locations far from compressors. | Upland boreal forest (Alberta, Canada) | Individuals were less likely to occur at compressor sites compared to well pad sites. Density effects were not measured for this species. | Effects are assumed to be long-term because the study occurred at sites subject to chronic noise. | Bayne and others (2008) |
Researchers observed noise near well pads with and without compressors in an active oil and gas field. Sound levels were not reported. Noise was analyzed as a categorical and continuous variable. | Pinus spp. (pinyon)-Juniperus spp. (juniper) woodlands and shrublands (New Mexico) | Individuals strongly preferred quiet well pads more than study sites with compressors for nest site selection. | Effects are assumed to be long-term because the study occurred at sites subject to chronic noise. All five observed nests were at quiet well pad study sites. | Francis and others (2009) |
Researchers observed noise near well pads with (range of 46–68 dBA) and without (range of 32–46 dBA) compressors in an active oil and gas field. Noise was analyzed as a categorical variable. | Pinyon-juniper woodlands and shrublands (New Mexico) | Abundance was lower on sites with compressors compared to quiet well pads. The number of nests was lower on sites with compressors compared to quiet well pads. | Effects are assumed to be long-term because the study occurred at sites subject to chronic noise. | Francis and others (2011b) |
Researchers observed noise near well pads with (range of 46–68 dBA) and without (range of 32–46 dBA) compressors in an active oil and gas field. Noise was analyzed as a categorical variable. | Pinyon-juniper woodlands and shrublands (New Mexico) | Abundance was lower on sites with compressors compared to quiet well pads. The number of nests was lower on sites with compressors compared to quiet well pads. | Effects are assumed to be long-term because the study occurred at sites subject to chronic noise. | Francis and others (2011b) |
Table 2.6.
Brief findings from studies about the effects of noise from oil and gas development on Zenaida macroura (mourning dove; family Columbidae).[Findings from each study that are not specific to the effects of noise are not included in the table. dBA, A-weighted decibel]
Noise type and description | Study system and geographic location | Relevant study findings | Intensity of effect | Reference |
---|---|---|---|---|
Researchers observed noise near well pads with and without compressors in an active oil and gas field. Sound levels were not reported. Noise was analyzed as a categorical and continuous variable. | Pinus spp. (pinyon)-Juniperus spp. (juniper) woodlands and shrublands (New Mexico) | Individuals strongly preferred quiet well pads more than study sites with compressors for nest site selection. | Effects are assumed to be long-term because the study occurred at sites subject to chronic noise. Twenty-two of 23 observed nests were at quiet well pad study sites. | Francis and others (2009) |
Researchers observed noise near well pads with (range of 46–68 dBA) and without (range of 32–46 dBA) compressors in an active oil and gas field. Noise was analyzed as a categorical variable. | Pinyon-juniper woodlands and shrublands (New Mexico) | Abundance was lower on sites with compressors compared to quiet well pads. | Effects are assumed to be long-term because the study occurred at sites subject to chronic noise. | Francis and others (2011b) |
Table 2.7.
Brief findings from studies about the effects of noise from oil and gas development on species in the family Corvidae (jays).[Findings from each study that are not specific to the effects of noise are not included in the table. %, percent; dBC, C-weighted decibel; m, meter; dBA, A-weighted decibel; NA, not applicable]
Noise type and description | Study system and geographic location | Relevant study findings | Intensity of effect | Reference |
---|---|---|---|---|
Researchers observed noise near well pads with and without compressors in an active oil and gas field. Sound levels were not reported. Noise was analyzed as a categorical and continuous variable. | Pinus spp. (pinyon)-Juniperus spp. (juniper) woodlands and shrublands (New Mexico) | Noise was negatively associated with occupancy. | Effects are assumed to be long-term because the study occurred at sites subject to chronic noise. Occupancy was predicted to be 32% higher at quiet well pad sites than at sites with compressors. | Francis and others (2009) |
Researchers modeled noise for four development scenarios in an existing oil and gas field: (1) the existing development pattern, which included well sites with and without active compressors and no noise barriers; (2) the existing development pattern and noise barriers around wells with compressors, which would reduce noise levels from an average of 82 dBC to an average of 71 dBC 30 m from source; (3) a central compression station (meaning all compressors would be concentrated in a single point source) without barrier walls; and (4) a central compression station surrounded by a noise barrier. | Pinyon-juniper woodlands and shrublands (New Mexico) | Modeled occupancy was highest in scenario 2 with existing dispersed compressors and noise barriers. Modeled occupancy was second highest in scenario 4 with a central compression station surrounded by a noise barrier. | Effects are assumed to be long-term because the modeled noise is chronic compressor noise. Modeled occupancy was approximately 5% lower in scenarios without sound barriers. | Francis and others (2011d) |
Researchers observed noise near well pads with and without compressors in an active oil and gas field. Sound levels at compressor sites were approximately 14 dBA greater than quiet well pads. Average sound levels were not reported for either treatment or control sites. Noise was analyzed as a categorical variable. | Pinyon-juniper woodlands and shrublands (New Mexico) | Individuals were observed removing pinyon seeds only at quiet well pads and never at sites with compressor stations. | Effects are assumed to be long-term because the study occurred at sites subject to chronic noise. | Francis and others (2012b) |
Researchers observed noise in an active oil and gas field. The study included four noise categories: sound levels near to (100–300 m) and far from (400–700 m) compressor stations and quiet well pads. The average noise level near compressor stations was 48 dBA. Sound levels were not reported for quiet well pads or point count locations far from compressors. | Upland boreal forest (Alberta, Canada) | Site did not affect occupancy. Density effects were not measured for this species. | NA | Bayne and others (2008) |
Table 2.8.
Brief findings from studies about the effects of noise from oil and gas development on Haemorhous mexicanus (house finch; family Fringillidae).[Findings from each study that are not specific to the effects of noise are not included in the table. dBA, A-weighted decibel]
Noise type and description | Study system and geographic location | Relevant study findings | Intensity of effect | Reference |
---|---|---|---|---|
Researchers observed noise near well pads with and without compressors in an active oil and gas field. Sound levels were not reported. Noise was analyzed as a categorical and continuous variable. | Pinus spp. (pinyon)-Juniperus spp. (juniper) woodlands and shrublands (New Mexico) | Individuals strongly preferred study sites with compressors more than quiet well pads for nest site selection. | Effects are assumed to be long-term because the study occurred at sites subject to chronic noise. Twenty-nine of 31 observed nests were at study sites with compressors. | Francis and others (2009) |
Researchers observed noise near well pads with (range of 46–68 dBA) and without (range of 32–46 dBA) compressors in an active oil and gas field. Noise was analyzed as a categorical variable. | Pinyon-juniper woodlands and shrublands (New Mexico) | Abundance was higher on sites with compressors compared to quiet well pads. The number of nests was higher on sites with compressors compared to quiet well pads. | Effects are assumed to be long-term because the study occurred at sites subject to chronic noise. | Francis and others (2011b) |
Table 2.9.
Brief findings from studies about the effects of noise from oil and gas development on Tachycineta bicolor (tree swallow; family Hirundinidae).[Findings from each study that are not specific to the effects of noise are not included in the table. ~, about; dBC, C-weighted decibel; %, percent]
Noise type and description | Study system and geographic location | Relevant study findings | Intensity of effect | Reference |
---|---|---|---|---|
Researchers simulated natural gas compressor noise at treatment nest boxes and compared effects to control nest boxes. Sound levels were ~84 dBC at treatment nest boxes and ~55 dBC at control nest boxes. | Agricultural fields and woodlands (Pennsylvania) | Noisy sites had lower incubation rates, lower hatching success, and lower probability of eggs surviving to fledglings. Noise did not affect nest site selection, clutch size, food delivered per nestling, probability of hatched young fledging, or nest success. | Effects are assumed to be long-term because the simulated noise was continuous during the nesting period. The percentage of time spent incubating was 77% at control nest boxes and 72% at noisy nest boxes. The percentage of eggs that hatched was 97% at control nest boxes and 88% at noisy nest boxes. The percentage of eggs that survived to fledglings was 92% at control nest boxes and 83% at noisy nest boxes. | Williams and others (2021) |
Table 2.10.
Brief findings from studies about the effects of noise from oil and gas development on species in the family Icteridae (blackbirds).[Findings from each study that are not specific to the effects of noise are not included in the table. m, meter; km2, square kilometer; dBA, A-weighted decibel; NA, not applicable; dBC, C-weighted decibel]
Noise type and description | Study system and geographic location | Relevant study findings | Intensity of effect | Reference |
---|---|---|---|---|
Researchers observed noise near well pads with and without compressors in an active oil and gas field. Sound levels were not reported. Noise was analyzed as a categorical and continuous variable. | Pinus spp. (pinyon)-Juniperus spp. (juniper) woodlands and shrublands (New Mexico) | Parasitized nests were farther from the well pad at study sites with compressors than at quiet well pads. | Effects are assumed to be long-term because the study occurred at sites subject to chronic noise. Parasitized nest sites were an average of 298 m from the well pad at study sites with compressors and an average of 172 m from the well pad at quiet well pads. | Francis and others (2009) |
Researchers simulated natural gas compressor noise at 6–8 treatment sites and compared effects to 6–7 control sites across a 100 km2 study area. Noise treatments were broadband 50 m from the sound source (average 56 dBA), broadband 250 m from the sound source (average 46 dBA), narrowband 50 m from the sound source (average 58 dBA), and narrowband 250 m from the sound source (average 40 dBA). Average sound levels at control sites ranged from 31 to 37 dBA. Noise was analyzed as a categorical and continuous variable. | Artemisia spp. (sagebrush) steppe (Idaho) | Noise did not affect abundance. | NA | Cinto Mejia and others (2019) |
Researchers observed noise at active well sites and control sites. Noise was estimated to range from 62 to 73 dBC 10 m from wells. Control sites were quiet, inactive well pads. Sound levels were not reported for control sites. | Mixed-grass prairie (Alberta, Canada) | Noise treatment (active wells) did not affect abundance. | NA | Nenninger and Koper (2018) |
Table 2.11.
Brief findings from studies about the effects of noise from oil and gas development on Oreoscoptes montanus (sage thrasher; family Mimidae).[Findings from each study that are not specific to the effects of noise are not included in the table. km2, square kilometer; dBA, A-weighted decibel; m, meter; NA, not applicable]
Noise type and description | Study system and geographic location | Relevant study findings | Intensity of effect | Reference |
---|---|---|---|---|
Researchers simulated natural gas compressor noise at 6–8 treatment sites and compared effects to 6–7 control sites across a 100 km2 study area. Noise treatments were broadband 50 m from the sound source (average 56 dBA), broadband 250 m from the sound source (average 46 dBA), narrowband 50 m from the sound source (average 58 dBA), and narrowband 250 m from the sound source (average 40 dBA). Average sound levels at control sites ranged from 31 to 37 dBA. Noise was analyzed as a categorical and continuous variable. | Artemisia spp. (sagebrush) steppe (Idaho) | Noise did not affect abundance. | NA | Cinto Mejia and others (2019) |
Table 2.12.
Brief findings from studies about the effects of noise from oil and gas development on Anthus spragueii (Sprague’s pipit; family Motacillidae).[Findings from each study that are not specific to the effects of noise are not included in the table. dBC, C-weighted decibel; NA, not applicable; m, meter; h/day, hour per day; %, percent]
Noise type and description | Study system and geographic location | Relevant study findings | Intensity of effect | Reference |
---|---|---|---|---|
Researchers observed noise at active well and gas compressor sites and control sites. Noise was estimated to range from 68 to 78 dBC at sites with generator-powered pumpjacks, 59 to 65 dBC at sites with grid-powered pumpjacks, 75.5 to 82.5 dBC at sites with generator-powered screwpumps, 57 to 61 dBC at sites with grid-powered screwpumps, and 79 to 85 dBC at sites with compressor stations. Control sites were quiet, inactive well pads that had sound levels estimated to range from 47 to 57 dBC. | Mixed-grass prairie (Alberta, Canada) | Noise treatment (active sites) did not affect nesting success. | NA | Bernath-Plaisted and Koper (2016) |
Researchers observed noise at active well sites and control sites. Noise was estimated to range from 62 to 73 dBC 10 m from wells. Control sites were quiet, inactive well pads. Sound levels were not reported for control sites. | Mixed-grass prairie (Alberta, Canada) | Noise treatment (active wells) did not affect abundance. | NA | Nenninger and Koper (2018) |
Researchers simulated three types of well pad noise at treatment sites and compared the effects to two types of control sites. Treatments included simulated drilling noise (84–92 dBC 10 m from speaker) broadcasted 24 h/day for two 10-day periods, simulated generator-powered pumpjack noise (68–78 dBC 10 m from speaker) broadcasted for 24 h/day for approximately 95 days, and simulated grid-powered pumpjacks (59–65 dBC 10 m from speaker) broadcasted 24 h/day for approximately 87 days. Control sites for noise treatments were quiet, infrastructure-only sites or sites without infrastructure (48–54 dBC). | Mixed-grass prairie (Alberta, Canada) | Noise simulations did not affect abundance relative to quiet, infrastructure-only sites. Nest success decreased across sites with simulated drilling noise. Nestling body condition and clutch size were not measured for this species. | Effects on nest success occurred during the 10-day noise simulation. Nest success was approximately 85% lower at sites with simulated drilling noise relative to all other sites. | Rosa and Koper (2022) |
Table 2.13.
Brief findings from studies about the effects of noise from oil and gas development on species in the family Paridae (chickadees and titmice).[Findings from each study that are not specific to the effects of noise are not included in the table. dBF, flat frequency response; NA, not applicable; m, meter; dBA, A-weighted decibel]
Noise type and description | Study system and geographic location | Relevant study findings | Intensity of effect | Reference |
---|---|---|---|---|
Researchers observed noise near well pads with (average 71 dBF) and without (average 60 dBF) compressors in an active oil and gas field. Noise was analyzed as a continuous variable. | Pinus spp. (pinyon)-Juniperus spp. (juniper) woodlands and shrublands (New Mexico) | Noise did not affect nest site selection. | NA | Kleist and others (2017) |
Researchers observed noise in an active oil and gas field. The study included four noise categories: sound levels near to (100–300 m) and far from (400–700 m) compressor stations and quiet well pads. The average noise level near compressor stations was 48 dBA. Sound levels were not reported for quiet well pads or point count locations far from compressors. | Upland boreal forest (Alberta, Canada) | Site did not affect occupancy. Density effects were not measured for this species. | NA | Bayne and others (2008) |
Table 2.14.
Brief findings from studies about the effects of noise from oil and gas development on species in the family Parulidae (New World warblers).[Findings from each study that are not specific to the effects of noise are not included in the table. m, meter; dBA, A-weighted decibel; NA, not applicable; %, percent]
Noise type and description | Study system and geographic location | Relevant study findings | Intensity of effect | Reference |
---|---|---|---|---|
Researchers observed noise in an active oil and gas field. The study included four noise categories: sound levels near to (100–300 m) and far from (400–700 m) compressor stations and quiet well pads. The average noise level near compressor stations was 48 dBA. Sound levels were not reported for quiet well pads or point count locations far from compressors. | Upland boreal forest (Alberta, Canada) | Site did not affect occupancy. Density effects were not measured for this species. | NA | Bayne and others (2008) |
Researchers observed noise in an active oil and gas field. The study included four noise categories: sound levels near to (100–300 m) and far from (400–700 m) compressor stations and quiet well pads. The average noise level near compressor stations was 48 dBA. Sound levels were not reported for quiet well pads or point count locations far from compressors. | Upland boreal forest (Alberta, Canada) | Site did not affect density or occupancy. | NA | Bayne and others (2008) |
Researchers observed noise in an active oil and gas field. The study included four noise categories: sound levels near to (100–300 m) and far from (400–700 m) compressor stations and quiet well pads. The average noise level near compressor stations was 48 dBA. Sound levels were not reported for quiet well pads or point count locations far from compressors. | Upland boreal forest (Alberta, Canada) | Site did not affect occupancy. Density effects were not measured for this species. | NA | Bayne and others (2008) |
Researchers observed noise in an active oil and gas field. The study included four noise categories: sound levels near to (100–300 m) and far from (400–700 m) compressor stations and quiet well pads. The average noise level near compressor stations was 48 dBA. Sound levels were not reported for quiet well pads or point count locations far from compressors. | Upland boreal forest (Alberta, Canada) | Site did not affect occupancy. Density effects were not measured for this species. | NA | Bayne and others (2008) |
Researchers observed noise in an active oil and gas field. The study included four noise categories: sound levels near to (100–300 m) and far from (400–700 m) compressor stations and quiet well pads. The average noise level near compressor stations was 48 dBA. Sound levels were not reported for quiet well pads or point count locations far from compressors. | Upland boreal forest (Alberta, Canada) | Site did not affect density or occupancy. | NA | Bayne and others (2008) |
Researchers observed noise in an active oil and gas field. The study included treatment sites around active compressor stations and control sites around quiet well pads. Sound levels were not reported. | Upland boreal forest (Alberta, Canada) | Noisy sites were associated with lower pairing success of male individuals compared to control sites. Young males were a greater proportion of the total males occupying noisy sites compared to control sites. | Effects are assumed to be long-term because the study occurred at sites subject to chronic noise. Pairing success was 92% at control sites and 77% at noisy sites. The proportion of young males was 30% at control sites and 48% at noisy sites. | Habib and others (2007) |
Researchers observed noise in an active oil and gas field. The study included four noise categories: sound levels near to (100–300 m) and far from (400–700 m) compressor stations and quiet well pads. The average noise level near compressor stations was 48 dBA. Sound levels were not reported for quiet well pads or point count locations far from compressors. | Upland boreal forest (Alberta, Canada) | Density was lower at compressor sites compared to quiet well pad sites. Individuals were less likely to occur at compressor sites compared to well pad sites. | Effects are assumed to be long-term because the study occurred at sites subject to chronic noise. | Bayne and others (2008) |
Researchers observed noise in an active oil and gas field. The study included four noise categories: sound levels near to (100–300 m) and far from (400–700 m) compressor stations and quiet well pads. The average noise level near compressor stations was 48 dBA. Sound levels were not reported for quiet well pads or point count locations far from compressors. | Upland boreal forest (Alberta, Canada) | Site did not affect occupancy. Density effects were not measured for this species. | NA | Bayne and others (2008) |
Researchers observed noise near well pads with and without compressors in an active oil and gas field. Sound levels were not reported. Noise was analyzed as a categorical and continuous variable. | Pinus spp. (pinyon)-Juniperus spp. (juniper) woodlands and shrublands (New Mexico) | Individuals nested farther from the well pad at study sites with compressors than at quiet well pads. | Effects are assumed to be long-term because the study occurred at sites subject to chronic noise. Nest sites were an average of 286 m from the well pad at study sites with compressors and an average of 189 m from the well pad at quiet well pad study sites. | Francis and others (2009) |
Researchers observed noise in an active oil and gas field. The study included four noise categories: sound levels near to (100–300 m) and far from (400–700 m) compressor stations and quiet well pads. The average noise level near compressor stations was 48 dBA. Sound levels were not reported for quiet well pads or point count locations far from compressors. | Upland boreal forest (Alberta, Canada) | Site did not affect occupancy. Density effects were not measured for this species. | NA | Bayne and others (2008) |
Researchers observed noise in an active oil and gas field. The study included four noise categories: sound levels near to (100–300 m) and far from (400–700 m) compressor stations and quiet well pads. The average noise level near compressor stations was 48 dBA. Sound levels were not reported for quiet well pads or point count locations far from compressors. | Upland boreal forest (Alberta, Canada) | Individuals were less likely to occur at compressor sites compared to well pad sites. Density effects were not measured for this species. | Effects are assumed to be long-term because the study occurred at sites subject to chronic noise. | Bayne and others (2008) |
Table 2.15.
Brief findings from studies about the effects of noise from oil and gas development on species in the family Passerellidae (New World sparrows and towhees).[Findings from each study that are not specific to the effects of noise are not included in the table. km2, square kilometer; dBA, A-weighted decibel; m, meter; NA, not applicable; dBC, C-weighted decibel; ha, hectare; kHz, kilohertz; h/day, hour per day; %, percent; Hz, hertz; dBZ, Z-weighted decibel; ~, about; ≤, less than or equal to]
Noise type and description | Study system and geographic location | Relevant study findings | Intensity of effect | Reference |
---|---|---|---|---|
Researchers simulated natural gas compressor noise at 6–8 treatment sites and compared the effects to 6–7 control sites across a 100 km2 study area. Noise treatments were broadband 50 m from the sound source (average 56 dBA), broadband 250 m from the sound source (average 46 dBA), narrowband 50 m from the sound source (average 58 dBA), and narrowband 250 m from the sound source (average 40 dBA). Average sound levels at control sites ranged from 31 to 37 dBA. Noise was analyzed as a categorical and continuous variable. | Artemisia spp. (sagebrush) steppe (Idaho) | Noise did not affect abundance. | NA | Cinto Mejia and others (2019) |
Researchers simulated drilling noise for 10 days at three sites without noise-producing infrastructure and compared the effects to pretreatment and post-treatment periods. Noise levels at treatment sites averaged 88 dBC 10 m from the speaker. | Mixed-grass prairie (Alberta, Canada) | Individuals adjusted songs during the noise treatment, with shifts to increase song sound level when singing at lower frequencies. | Effects occurred during the 10-day duration of the noise simulation. Five of eight song syllables and song type changed in the presence of noise. | Curry and others (2018a) |
Researchers observed noise at active well sites and control sites. Noise was estimated to range from 62 to 73 dBC 10 m from wells. Control sites were quiet, inactive well pads. Sound levels were not reported for control sites. | Mixed-grass prairie (Alberta, Canada) | Noise treatment (active wells) did not affect abundance. | NA | Nenninger and Koper (2018) |
Researchers deployed sound recording units arranged in a 3,600-ha grid at three sites across a gradient of oil and gas development. Absolute sound levels were not reported. | Upland boreal forest (Alberta, Canada) | Occupancy was high in areas with greater vegetation disturbance and earlier age-class forest but decreased with increased sound level of high frequency sound bands (2–8 kHz). Sound level of low frequency sound bands (0.5–1 kHz) did not affect occupancy. | Effects are assumed to be long-term because the study occurred at sites subject to chronic noise. High frequency noise had a slight negative effect on occupancy. Occupancy estimates at higher sound levels had high variability. | Sánchez and others (2022) |
Researchers observed noise at active well and gas compressor sites and control sites. Noise levels measured at grid-powered screwpumps were approximately 55 dBA (59 dBC) 10 m from the source, at generator-powered screwpumps were approximately 68 dBA (79 dBC) 10 m from the source, and at compressor stations were approximately 69 dBA (82 dBC) 10 m from the source. Sound levels at control sites were approximately 33 dBA (52 dBC). Sound level was also analyzed as a continuous variable regardless of site and compared across categories of frequencies, including broadband (0–24,000 Hz), low (0–3,000 Hz), and high (3,000–12,000 Hz). | Mixed-grass prairie (Alberta, Canada) | In the presence of compressor stations, individuals reduced delay in returning to nests to feed in response to conspecific alarm calls and control (Sturnella neglecta [western meadowlark]) calls compared to control sites. Baseline feeding latency (without alarm or predator calls) decreased at compressor stations. Feeding latency increased in response to control calls with increased noise levels. | Effects are assumed to be long-term because the study occurred at sites subject to chronic noise. | Antze and Koper (2018) |
Researchers observed noise at active well and gas compressor sites and control sites. Noise was estimated to range from 68 to 78 dBC at sites with generator-powered pumpjacks, 59 to 65 dBC at sites with grid-powered pumpjacks, 75.5 to 82.5 dBC at sites with generator-powered screwpumps, 57 to 61 dBC at sites with grid-powered screwpumps, and 79 to 85 dBC at sites with compressor stations. Control sites were quiet, inactive well pads that had sound levels estimated to range from 47 to 57 dBC. | Mixed-grass prairie (Alberta, Canada) | Noise treatment (active sites) did not affect nesting success. | NA | Bernath-Plaisted and Koper (2016) |
Researchers simulated drilling noise for 10 days at three sites without noise-producing infrastructure and compared effects to pre-treatment and post-treatment periods. Noise levels at treatment sites averaged 88 dBC 10 m from the speaker. | Mixed-grass prairie (Alberta, Canada) | Individuals adjusted songs by increasing song frequency during the noise simulation. | Effects occurred during the 10-day duration of the noise simulation. | Curry and others (2018a) |
Researchers recorded variation in individuals’ responses to song simulations based on the presence of noise in an active oil and gas field at sites within 400 m of pumpjacks, sites within 400 m of screw pumps, and control sites farther than 800 m from wells. Song simulations were recorded at sites with simulated drilling noise (average of 88 dBC 10 m from speaker) and control sites with no noise-producing infrastructure. | Mixed-grass prairie (Alberta, Canada) | Simulated noise-adjusted songs produced more calls and fewer attacks and wing flicks at control sites than unadjusted songs at control sites and similar responses at noisy sites compared to simulated unadjusted songs at control sites. Simulated unadjusted songs produced different responses at noisy sites compared to control sites. Stress response was higher near pumpjacks compared to screwpumps or control sites. Individuals with a greater stress response benefited more from song adjustment at pumpjacks but not screwpumps. | Effects are assumed to be long-term because the study occurred at sites subject to chronic noise. Variable intensity of effect for behavioral and stress changes by treatment combination. | Curry and others (2018b) |
Researchers observed noise at active well sites and control sites. Noise was estimated to range from 62 to 73 dBC 10 m from wells. Control sites were quiet, inactive well pads. Sound levels were not reported for control sites. | Mixed-grass prairie (Alberta, Canada) | Noise treatment (active wells) did not affect abundance. | NA | Nenninger and Koper (2018) |
Researchers simulated three types of well pad noise at treatment sites and compared the effects to two types of control sites. Treatments included simulated drilling noise (84–92 dBC 10 m from speaker) broadcasted 24 h/day for two 10-day periods, simulated generator-powered pumpjack noise (68–78 dBC 10 m from speaker) broadcasted for 24 h/day for approximately 95 days, and simulated grid-powered pumpjacks (59–65 dBC 10 m from speaker) broadcasted 24 h/day for approximately 87 days. Control sites for noise treatments were quiet, infrastructure-only sites or sites without infrastructure (48–54 dBC). | Mixed-grass prairie (Alberta, Canada) | Abundance decreased with simulated drilling noise. Nest success decreased across sites with simulated drilling noise. Nestling body condition decreased with increased proximity to simulated drilling noise. Noise simulations did not affect clutch size. | Effects on nest success occurred during the 10-day noise simulation. Abundance was approximately 30% lower with simulated drilling noise relative to quiet infrastructure sites and control sites. Abundance effects occurred at all spatial scales (local and site). Nest success was approximately 35% lower at sites with simulated drilling noise relative to all other sites. Nestling body condition was lower near simulated drilling noise relative to all other locations near all other noise treatments and quiet sites. | Rosa and Koper (2022) |
Researchers observed noise at active well and gas compressor sites and control sites. Noise levels measured at generator-powered screw pumps were 80–90 dBZ less than 10 m from the source, at compressor stations were 75–80 dBZ less than 10 m from the source, and at grid-powered screw pumps and generator-powered pumpjacks were 55–80 dBZ less than 10 m from the source. Control sites were located at least 800 m away from infrastructure. Sound levels were not reported for control sites. The effects model included sound level as a continuous variable and infrastructure type as a categorical variable. | Mixed-grass prairie (Alberta, Canada) | Increases in the minimum frequency of songs were associated with increasing ambient sound levels. Noise did not affect song maximum frequency. Song syllables shifted in the presence of noise. Song features changed at all noise-producing infrastructure types and had the highest degree of change at generator-powered screw pumps. | Effects are assumed to be long-term because the study occurred at sites subject to chronic noise. Song minimum frequency increased approximately 13 Hz per 1 dBZ increase in ambient sound level. Three song syllables shifted substantially in the presence of noise. High frequency syllables increased in bandwidth approximately 17–26 Hz per 1 dBZ increase in ambient sound level. The lowest frequency syllable increased in bandwidth approximately 11–15 Hz per 1 dBZ increase in ambient sound level. One higher frequency syllable became quieter by approximately 0.3 dBZ with increasing ambient sound level. | Warrington and others (2018) |
Researchers observed noise near well pads with and without compressors in an active oil and gas field. Sound levels were not reported. Noise was analyzed as a categorical and continuous variable. | Pinus spp. (pinyon)-Juniperus spp. (juniper) woodlands and shrublands (New Mexico) | Individuals nested farther from the well pad at study sites with compressors than at quiet well pads. Increases in noise level were associated with lower daily nest predation. | Effects are assumed to be long-term because the study occurred at sites subject to chronic noise. Nest sites were an average of 268 m from the well pad at study sites with compressors and an average of 157 m from the well pad at quiet well pad sites. | Francis and others (2009) |
Researchers observed noise near well pads with (46–68 dBA) and without (32–46 dBA) compressors in an active oil and gas field. Noise was analyzed as a continuous variable. | Pinyon-juniper woodlands and shrublands (New Mexico) | Noise did not affect occupancy. Noise was associated with an increased peak frequency of songs but did not affect peak frequency of the lowest note of songs. | Effects are assumed to be long-term because the study occurred at sites subject to chronic noise. Peak frequency of songs increased by 314 Hz per 10 dBA. | Francis and others (2012a) |
Researchers observed noise near well pads with and without compressors in an active oil and gas field. Sound levels ranged from ~35 to 52 dBA across all study sites. Noise was analyzed as a continuous variable. | Pinyon-juniper woodlands and shrublands (New Mexico) | Increased noise was correlated with delayed and reduced territory defense behavior (slower vocal response, fewer vocalizations, and slower movement toward an intruder) in males in the presence of a simulated intraspecific competitor’s song. | Effects are assumed to be long-term because the study occurred at sites subject to chronic noise. Apparent masking effects occurred at 17 m from study individuals when surrounding noise levels were ≤52 dBA. | Kleist and others (2016) |
Researchers observed noise at active well and gas compressor sites and control sites. Noise was estimated to range from 68 to 78 dBC at sites with generator-powered pumpjacks, 59 to 65 dBC at sites with grid-powered pumpjacks, 75.5 to 82.5 dBC at sites with generator-powered screwpumps, 57 to 61 dBC at sites with grid-powered screwpumps, and 79 to 85 dBC at sites with compressor stations. Control sites were quiet, inactive well pads that had sound levels estimated to range from 47 to 57 dBC. | Mixed-grass prairie (Alberta, Canada) | Noise treatment (active sites) did not affect nesting success. | NA | Bernath-Plaisted and Koper (2016) |
Researchers simulated three types of well pad noise at treatment sites and compared the effects to two types of control sites. Treatments included simulated drilling noise (84–92 dBC 10 m from speaker) broadcasted 24 h/day for two 10-day periods, simulated generator-powered pumpjack noise (68–78 dBC 10 m from speaker) broadcasted for 24 h/day for approximately 95 days, and simulated grid-powered pumpjacks (59–65 dBC 10 m from speaker) broadcasted 24 h/day for approximately 87 days. Control sites for noise treatments were quiet, infrastructure-only sites or sites without infrastructure (48–54 dBC). | Mixed-grass prairie (Alberta, Canada) | Noise simulations did not affect abundance relative to quiet infrastructure sites. Noise simulations did not affect nest success relative to control sites. Nestling body condition and clutch size were not measured for this species. | NA | Rosa and Koper (2022) |
Researchers simulated natural gas compressor noise at 6–8 treatment sites and compared effects to 6–7 control sites across a 100 km2 study area. Noise treatments were broadband 50 m from the sound source (average 56 dBA), broadband 250 m from the sound source (average 46 dBA), narrowband 50 m from the sound source (average 58 dBA), and narrowband 250 m from the sound source (average 40 dBA). Average sound levels at control sites ranged from 31 to 37 dBA. Noise was analyzed as a categorical and continuous variable. | Sagebrush steppe (Idaho) | Abundance was lower at sites subject to broadband and narrowband treatments than at control sites. Abundance decreased with increased noise levels at broadband and narrowband sites. | The effect was measured for the duration of the experiment, which occurred during the breeding season in 2 consecutive years. Abundance was 30% lower at narrowband sites at 50 m, 52% lower at broadband sites at 50 m, and 13% lower at broadband sites at 250 m compared to control sites. Noise was associated with a 15% reduction in abundance per 9 dBA at narrowband sites and a 17% reduction per 9 dBA at broadband sites. | Cinto Mejia and others (2019) |
Researchers observed noise in an active oil and gas field. The study included four noise categories: sound levels near to (100–300 m) and far from (400–700 m) compressor stations and quiet well pads. The average noise level near compressor stations was 48 dBA. Sound levels were not reported for quiet well pads or point count locations far from compressors. | Upland boreal forest (Alberta, Canada) | Site did not affect occupancy. Density effects were not measured for this species. | NA | Bayne and others (2008) |
Researchers observed noise near well pads with and without compressors in an active oil and gas field. Noise levels were not reported. Noise was analyzed as a categorical and continuous variable. | Pinyon-juniper woodlands and shrublands (New Mexico) | Increases in noise level were associated with lower daily nest predation. | Effects are assumed to be long-term because the study occurred at sites subject to chronic noise. | Francis and others (2009) |
Researchers observed noise near well pads with (range of 46–68 dBA) and without (range of 32–46 dBA) compressors in an active oil and gas field. Noise was analyzed as a categorical variable. | Pinyon-juniper woodlands and shrublands (New Mexico) | Noise did not affect abundance or the number of nests. | NA | Francis and others (2011b) |
Researchers observed noise near well pads with and without compressors in an active oil and gas field. Sound levels ranged from ~35 to 52 dBA across all study sites. Noise was analyzed as a continuous variable. | Pinyon-juniper woodlands and shrublands (New Mexico) | Noise was associated with delayed and reduced territory defense behavior (slower vocal response, fewer vocalizations, and slower movement toward an intruder) in males in the presence of a simulated intraspecific competitor’s song. | Effects are assumed to be long-term because the study occurred at sites subject to chronic noise. Apparent masking effects occurred at 17 m from study individuals when surrounding noise levels were ≤52 dBA. | Kleist and others (2016) |
Researchers observed noise in an active oil and gas field. Treatments included four noise categories: sound levels near to (100–300 m) and far from (400–700 m) compressor stations and quiet well pads. The average noise level near compressor stations was 48 dBA. Sound levels were not reported for quiet well pads or point count locations far from compressors. | Upland boreal forest (Alberta, Canada) | Density was lower at compressor sites compared to quiet well pad sites. Site did not affect occupancy. | Effects are assumed to be long-term because the study occurred at sites subject to chronic noise. Density was 0.5–0.7 birds per 10 ha at compressor sites and 1.5–1.8 birds per 10 ha at quiet well pad sites. | Bayne and others (2008) |
Table 2.16.
Brief findings from studies about the effects of noise from oil and gas development on Polioptila caerulea (blue-gray gnatcatcher; family Polioptilidae).[Findings from each study that are not specific to the effects of noise are not included in the table. dBA, A-weighted decibel; NA, not applicable]
Noise type and description | Study system and geographic location | Relevant study findings | Intensity of effect | Reference |
---|---|---|---|---|
Researchers observed noise near well pads with (46–68 dBA) and without (32–46 dBA) compressors in an active oil and gas field. Noise was analyzed as a continuous variable. | Pinus spp. (pinyon)-Juniperus spp. (juniper) woodlands and shrublands (New Mexico) | Noise did not affect occupancy or song frequency. | NA | Francis and others (2012a) |
Table 2.17.
Brief findings from studies about the effects of noise from oil and gas development on Corthylio calendula (ruby-crowned kinglet; family Regulidae).[Findings from each study that are not specific to the effects of noise are not included in the table. m, meter; dBA, A-weighted decibel; NA, not applicable]
Noise type and description | Study system and geographic location | Relevant study findings | Intensity of effect | Reference |
---|---|---|---|---|
Researchers observed noise in an active oil and gas field. The study included four noise categories: sound levels near to (100–300 m) and far from (400–700 m) compressor stations and quiet well pads. The average noise level near compressor stations was 48 dBA. Sound levels were not reported for quiet well pads or point count locations far from compressors. | Upland boreal forest (Alberta, Canada) | Site did not affect occupancy. Density effects were not measured for this species. | NA | Bayne and others (2008) |
Table 2.18.
Brief findings from studies about the effects of noise from oil and gas development on Sitta canadensis (red-breasted nuthatch; family Sittidae).[Findings from each study that are not specific to the effects of noise are not included in the table. m, meter; dBA, A-weighted decibel]
Noise type and description | Study system and geographic location | Relevant study findings | Intensity of effect | Reference |
---|---|---|---|---|
Researchers observed noise in an active oil and gas field. The study included four noise categories: sound levels near to (100–300 m) and far from (400–700 m) compressor stations and quiet well pads. The average noise level near compressor stations was 48 dBA. Sound levels were not reported for quiet well pads or point count locations far from compressors. | Upland boreal forest (Alberta, Canada) | Individuals were less likely to occur at compressor sites compared to well pad sites. Density effects were not measured for this species. | Effects are assumed to be long-term because the study occurred at sites subject to chronic noise. | Bayne and others (2008) |
Table 2.19.
Brief findings from studies about the effects of noise from oil and gas development on Archilochus alexandri (black-chinned hummingbird; family Trochilidae).[Findings from each study that are not specific to the effects of noise are not included in the table. dBA, A-weighted decibel]
Noise type and description | Study system and geographic location | Relevant study findings | Intensity of effect | Reference |
---|---|---|---|---|
Researchers observed noise near well pads with and without compressors in an active oil and gas field. Sound levels were not reported. Noise was analyzed as a categorical and continuous variable. | Pinus spp. (pinyon)-Juniperus spp. (juniper) woodlands and shrublands (New Mexico) | Individuals strongly preferred study sites with compressors more than quiet well pads for nest site selection. | Effects are assumed to be long-term because the study occurred at sites subject to chronic noise. Thirty-six of 39 observed nests were at study sites with compressors. | Francis and others (2009) |
Researchers observed noise near well pads with (range of 46–68 dBA) and without (range of 32–46 dBA) compressors in an active oil and gas field. Noise was analyzed as a categorical variable. | Pinyon-juniper woodlands and shrublands (New Mexico) | Abundance and the number of nests were higher on sites with compressors compared to quiet well pads. | Effects are assumed to be long-term because the study occurred at sites subject to chronic noise. | Francis and others (2011b) |
Researchers observed noise near well pads with and without compressors in an active oil and gas field. Sound levels at compressor sites were approximately 12 dBA greater than quiet well pads. Average sound levels were not reported for either treatment or control sites. Noise was analyzed as a categorical variable. | Pinyon-juniper woodlands and shrublands (New Mexico) | Individuals were more commonly observed pollinating flowers in study sites with compressors than at quiet well pads. | Effects are assumed to be long-term because the study occurred at sites subject to chronic noise. Pollination observations were five times more frequent at study sites with compressors. | Francis and others (2012b) |
Table 2.20.
Brief findings from studies about the effects of noise from oil and gas development on Troglodytes hiemalis (winter wren; family Troglodytidae).[Findings from each study that are not specific to the effects of noise are not included in the table. m, meter; dBA, A-weighted decibel; NA, not applicable]
Noise type and description | Study system and geographic location | Relevant study findings | Intensity of effect | Reference |
---|---|---|---|---|
Researchers observed noise in an active oil and gas field. The study included four noise categories: sound levels near to (100–300 m) and far from (400–700 m) compressor stations and quiet well pads. The average noise level near compressor stations was 48 dBA. Sound levels were not reported for quiet well pads or point count locations far from compressors. | Upland boreal forest (Alberta, Canada) | Site did not affect occupancy. Density effects were not measured for this species. | NA | Bayne and others (2008) |
Table 2.21.
Brief findings from studies about the effects of noise from oil and gas development on species in the family Turdidae (thrushes).[Findings from each study that are not specific to the effects of noise are not included in the table. m, meter; dBA, A-weighted decibel; NA, not applicable; dBF, flat frequency response; ~, about; dBC, C-weighted decibel; %, percent]
Noise type and description | Study system and geographic location | Relevant study findings | Intensity of effect | Reference |
---|---|---|---|---|
Researchers observed noise in an active oil and gas field. The study included four noise categories: sound levels near to (100–300 m) and far from (400–700 m) compressor stations and quiet well pads. The average noise level near compressor stations was 48 dBA. Sound levels were not reported for quiet well pads or point count locations far from compressors. | Upland boreal forest (Alberta, Canada) | Site did not affect occupancy. Density effects were not measured for this species. | NA | Bayne and others (2008) |
Researchers observed noise in an active oil and gas field. The study included four noise categories: sound levels near to (100–300 m) and far from (400–700 m) compressor stations and quiet well pads. The average noise level near compressor stations was 48 dBA. Sound levels were not reported for quiet well pads or point count locations far from compressors. | Upland boreal forest (Alberta, Canada) | Site did not affect occupancy. Density effects were not measured for this species. | NA | Bayne and others (2008) |
Researchers observed noise near well pads with (average 71 dBF) and without (average 60 dBF) compressors in an active oil and gas field. Noise was analyzed as a continuous variable. | Pinus spp. (pinyon)-Juniperus spp. (juniper) woodlands and shrublands (New Mexico) | Noise was negatively associated with nest site selection. | Effects are assumed to be long-term because the study occurred at sites subject to chronic noise. Noise and vegetation cover were associated with reduced nest box occupancy at the scale of the well pad site (250 m radius). Noise was not associated with reduced nest box occupancy at the scale of the nest box territory (45 m radius). | Kleist and others (2017) |
Researchers observed noise near well pads with (average 71 dBF) and without (average 60 dBF) compressors in an active oil and gas field. Noise was analyzed as a continuous variable. | Pinyon-juniper woodlands and shrublands (New Mexico) | Noise was negatively associated with baseline stress hormone levels in adult nesting females and nestlings. Noise was positively associated with acute stress hormone levels in nestlings. Moderate noise levels were positively associated with nestling feather growth and body size. Noise did not affect hatching success. | Effects are assumed to be long-term because the study occurred at sites subject to chronic noise. Noise was positively associated with nestling feather growth and body size up to ~70 dBF and negatively associated with feather growth and body size at sound levels greater than ~70 dBF. | Kleist and others (2018) |
Researchers observed noise near well pads with (average 71 dBF) and without (average 60 dBF) compressors in an active oil and gas field. Noise was analyzed as a continuous variable. | Pinyon-juniper woodlands and shrublands (New Mexico) | Noise did not affect nest site selection. | NA | Kleist and others (2017) |
Researchers observed noise near well pads with (average 71 dBF) and without (average 60 dBF) compressors in an active oil and gas field. Noise was analyzed as a continuous variable. | Pinyon-juniper woodlands and shrublands (New Mexico) | Noise was negatively associated with baseline stress hormone levels in adult nesting females and nestlings. Noise was positively associated with acute stress hormone levels in nestlings. Moderate noise levels were positively associated with nestling feather growth and body size. Noise was negatively associated with hatching success. | Effects are assumed to be long-term because the study occurred at sites subject to chronic noise. Noise was positively associated with nestling feather growth and body size up to ~70 dBF and negatively associated with feather growth and body size at sound levels greater than ~70 dBF. | Kleist and others (2018) |
Researchers simulated natural gas compressor noise at treatment nest boxes and compared effects to control nest boxes. Sound levels were ~84 dBC at treatment nest boxes and ~55 dBC at control nest boxes. | Agricultural fields and woodlands (Pennsylvania) | Noisy sites had lower incubation rates, lower hatching success, and lower probability of eggs surviving to fledglings. Noise did not affect nest site selection, clutch size, food delivered per nestling, probability of hatched young fledging, or nest success. | Effects are assumed to be long-term because the simulated noise was continuous during the nesting period. The percentage of time spent incubating was 70% at control nest boxes and 64% at noisy nest boxes. The percentage of eggs that hatched was 95% at control nest boxes and 80% at noisy nest boxes. The percentage of eggs that survived to fledglings was 85% at control nest boxes and 71% at noisy nest boxes. | Williams and others (2021) |
Researchers observed noise in an active oil and gas field. The study included four noise categories: sound levels near to (100–300 m) and far from (400–700 m) compressor stations and quiet well pads. The average noise level near compressor stations was 48 dBA. Sound levels were not reported for quiet well pads or point count locations far from compressors. | Upland boreal forest (Alberta, Canada) | Site did not affect occupancy. Density effects were not measured for this species. | NA | Bayne and others (2008) |
Table 2.22.
Brief findings from studies about the effects of noise from oil and gas development on species in the family Tyrannidae (tyrant flycatchers).[Findings from each study that are not specific to the effects of noise are not included in the table. m, meter; dBA, A-weighted decibel; NA, not applicable; %, percent; dBC, C-weighted decibel; Hz, hertz; ~, about; dBF, flat frequency response]
Noise type and description | Study system and geographic location | Relevant study findings | Intensity of effect | Reference |
---|---|---|---|---|
Researchers observed noise in an active oil and gas field. The study included four noise categories: sound levels near to (100–300 m) and far from (400–700 m) compressor stations and quiet well pads. The average noise level near compressor stations was 48 dBA. Sound levels were not reported for quiet well pads or point count locations far from compressors. | Upland boreal forest (Alberta, Canada) | Site did not affect occupancy. Density effects were not measured for this species. | NA | Bayne and others (2008) |
Researchers observed noise in an active oil and gas field. The study included four noise categories: sound levels near to (100–300 m) and far from (400–700 m) compressor stations and quiet well pads. The average noise level near compressor stations was 48 dBA. Sound levels were not reported for quiet well pads or point count locations far from compressors. | Upland boreal forest (Alberta, Canada) | Individuals were less likely to occur at compressor sites compared to well pad sites. Density effects were not measured for this species. | Effects are assumed to be long-term because the study occurred at sites subject to chronic noise. | Bayne and others (2008) |
Researchers observed noise near well pads with and without compressors in an active oil and gas field. Sound levels were not reported. Noise was analyzed as a categorical and continuous variable. | Pinus spp. (pinyon)-Juniperus spp. (juniper) woodlands and shrublands (New Mexico) | Individuals nested farther from the well pad at study sites with compressors than at quiet well pads. Increases in noise level were associated with lower daily nest predation. | Effects are assumed to be long-term because the study occurred at sites subject to chronic noise. Nest sites were an average of 262 m from the well pad at study sites with compressors and an average of 206 m from the well pad at quiet well pad sites. | Francis and others (2009) |
Researchers observed noise near well pads with (46–68 dBA) and without (32–46 dBA) compressors in an active oil and gas field. Noise was analyzed as a continuous variable. | Pinyon-juniper woodlands and shrublands (New Mexico) | Noise was negatively associated with occupancy. Noise did not affect song or call frequency. | Effects are assumed to be long-term because the study occurred at sites subject to chronic noise. Individuals were detected at 68% of point count locations at quiet well pads and 52% of point count locations at well pads with compressors. | Francis and others (2011c) |
Researchers modeled noise for four development scenarios in an existing oil and gas field: (1) the existing development pattern, which included well sites with and without active compressors and no noise barriers; (2) the existing development pattern and noise barriers around wells with compressors, which would reduce noise levels from an average of 82 dBC to and average of 71 dBC 30 m from source; (3) a central compression station (meaning all compressors would be concentrated in a single point source) without barrier walls; and (4) a central compression station surrounded by a noise barrier. | Pinyon-juniper woodlands and shrublands (New Mexico) | Modeled occupancy was highest in scenario 2 with existing dispersed compressors and noise barriers. Modeled occupancy was second highest in scenario 4 with a central compression station surrounded by a noise barrier. Modeled nest success was highest in scenario 3 with central compression and no noise barrier. Modeled nest success was second highest in scenario 1 with the existing oil and gas field conditions. Modeled nest success in scenarios 2 and 4 with noise barriers was similar to a control scenario with background sound levels. | Effects are assumed to be long-term because the modeled noise is chronic compressor noise. Modeled occupancy was approximately 5% lower in scenarios without sound barriers. Modeled nest success was approximately 8% higher in scenarios without sound barriers. | Francis and others (2011d) |
Researchers observed noise near well pads with (46–68 dBA) and without (32–46 dBA) compressors in an active oil and gas field. Noise was analyzed as a continuous variable. | Pinyon-juniper woodlands and shrublands (New Mexico) | Noise did not affect occupancy. Peak frequency of the lowest note of songs and calls and minimum frequency of calls increased with noise level. Song bandwidth decreased with increased noise level. | Effects are assumed to be long-term because the study occurred at sites subject to chronic noise. Song and call frequencies increased ~200 Hz across the range of ambient noise levels in which songs were recorded (37–64 dBA). | Francis and others (2011c) |
Researchers observed noise near well pads with (average 71 dBF) and without (average 60 dBF) compressors in an active oil and gas field. Noise was analyzed as a continuous variable. | Pinyon-juniper woodlands and shrublands (New Mexico) | Noise was negatively associated with nest site selection. | Effects are assumed to be long-term because the study occurred at sites subject to chronic noise. Noise had greater predictive power for nest box occupancy than vegetation cover at the scale of the well pad site (250 m radius) and lower predictive power than vegetation cover at the scale of the nest box territory (45 m radius). | Kleist and others (2017) |
Researchers observed noise near well pads with (average 71 dBF) and without (average 60 dBF) compressors in an active oil and gas field. Noise was analyzed as a continuous variable. | Pinyon-juniper woodlands and shrublands (New Mexico) | Noise was negatively associated with baseline stress hormone levels in adult nesting females and nestlings. Noise was positively associated with acute stress hormone levels in nestlings. Moderate noise levels were positively associated with nestling feather growth and body size. Noise did not affect hatching success. | Effects are assumed to be long-term because the study occurred at sites subject to chronic noise. Noise was positively associated with nestling feather growth and body size at sound levels less than ~70 dBF and negatively associated with feather growth and body size at sound levels greater than ~70 dBF. | Kleist and others (2018) |
Table 2.23.
Brief findings from studies about the effects of noise from oil and gas development on species in the family Vireonidae (vireos).[Findings from each study that are not specific to the effects of noise are not included in the table. m, meter; dBA, A-weighted decibel; ha, hectare; Hz, hertz]
Noise type and description | Study system and geographic location | Relevant study findings | Intensity of effect | Reference |
---|---|---|---|---|
Researchers observed noise in an active oil and gas field. The study included four noise categories: sound levels near to (100–300 m) and far from (400–700 m) compressor stations and quiet well pads. The average noise level near compressor stations was 48 dBA. Sound levels were not reported for quiet well pads or point count locations far from compressors. | Upland boreal forest (Alberta, Canada) | Density was lower near compressor stations than near quiet well pads. Individuals were less likely to occur at compressor sites compared to well pad sites. | Effects are assumed to be long-term because the study occurred at sites subject to chronic noise. Density was 3 birds per 10 ha near compressors and 5.4 birds per 10 ha near quiet well pads. | Bayne and others (2008) |
Researchers observed noise near well pads with and without compressors in an active oil and gas field. Sound levels were not reported. Noise was analyzed as a continuous variable. | Pinus spp. (pinyon)-Juniperus spp. (juniper) woodlands and shrublands (New Mexico) | Noise did not affect occupancy, singing rate, or peak or maximum song frequency. Noise was associated with increased minimum frequency, decreased bandwidth, and decreased duration of songs. | Effects are assumed to be long-term because the study occurred at sites subject to chronic noise. Minimum frequency increased 700 Hz across the range of ambient noise levels during which songs were recorded. | Francis and others (2011a) |
Researchers observed noise near well pads with and without compressors in an active oil and gas field. Sound levels were not reported. Noise was analyzed as a categorical and continuous variable. | Pinyon-juniper woodlands and shrublands (New Mexico) | Individuals nested farther from the well pad as study sites with compressors than at quiet well pads. | Effects are assumed to be long-term because the study occurred at sites subject to chronic noise. Nest sites were an average of 275 m from the well pad at study sites with compressors and an average of 187 m from the well pad at quiet well pad sites. | Francis and others (2009) |
Researchers observed noise near well pads with and without compressors in an active oil and gas field. Sound levels were not reported. Noise was analyzed as a continuous variable. | Pinyon-juniper woodlands and shrublands (New Mexico) | Noise did not affect occupancy, singing rate, or peak or minimum song frequency. Noise was associated with increased maximum frequency, bandwidth, and duration of songs. | Effects are assumed to be long-term because the study occurred at sites subject to chronic noise. Maximum frequency increased 900 Hz across the range of ambient noise levels during which songs were recorded. | Francis and others (2011a) |
Conversion Factors
International System of Units to U.S. customary units
Temperature in degrees Celsius (°C) may be converted to degrees Fahrenheit (°F) as follows: °F = (1.8 × °C) + 32
Abbreviations
BLM
Bureau of Land Management
CFR
Code of Federal Regulations
CO
carbon monoxide
dB
decibel
dBA
A-weighted decibel
dBC
C-weighted decibel
dBF
flat frequency response
dBZ
Z-weighted decibel
GIS
geographic information system
H2
hydrogen
Hz
hertz
km
kilometer
L
sound pressure level
L50
sound pressure level exceeded 50 percent of the timeframe of interest
L90
sound pressure level exceeded 90 percent of the timeframe of interest
Leq
equivalent sound level
Lmax
maximum sound pressure level during the timeframe of interest
Lmin
minimum sound pressure level during the timeframe of interest
Lx
exceedance percentile
m
meter
NEPA
National Environmental Policy Act
NMSim
Noise Model Simulation
NPS
National Park Service
SEL
sound exposure level
spp.
several species of
U.S.C.
United States Code
USGS
U.S. Geological Survey
VOC
volatile organic compound
Species Names
Common Name
Scientific Name
alder flycatcher
Empidonax alnorum
American redstart
Setophaga ruticilla
American robin
Turdus migratorius
ash-throated flycatcher
Myiarchus cinerascens
Baird’s sparrow
Centronyx bairdii
barn owl
Tyto alba
barred owl
Strix varia
black-and-white warbler
Mniotilta varia
black-capped chickadee
Poecile atricapillus
black-chinned hummingbird
Archilochus alexandri
black-headed grosbeak
Pheucticus melanocephalus
black-throated gray warbler
Setophaga nigrescens
blue-gray gnatcatcher
Polioptila caerulea
boreal owl
Aegolius funereus
Brewer’s sparrow
Spizella breweri
brown-headed cowbird
Molothrus ater
burrowing owl
Athene cunicularia
bushtit
Psaltriparus minimus
California scrub-jay
Aphelocoma californica
Canada jay
Perisoreus canadensis
Cassin’s finch
Haemorhous cassinii
cedar waxwing
Bombycilla cedrorum
chestnut-collared longspur
Calcarius ornatus
chipping sparrow
Spizella passerina
Connecticut warbler
Oporornis agilis
eastern bluebird
Sialia sialis
eastern screech owl
Megascops asio
gray flycatcher
Empidonax wrightii
gray vireo
Vireo vicinior
great horned owl
Bubo virginianus
greater sage-grouse
Centrocercus urophasianus
hermit thrush
Catharus guttatus
horned lark
Eremophila alpestris
house finch
Haemorhous mexicanus
juniper titmouse
Baeolophus ridgwayi
least flycatcher
Empidonax minimus
lesser prairie-chicken
Tympanuchus pallidicinctus
Lincoln’s sparrow
Melospiza lincolnii
long-eared owl
Asio otus
magnolia warbler
Setophaga magnolia
Mexican spotted owl
Strix occidentalis lucida
mottled owl
Strix virgata
mountain bluebird
Sialia currucoides
mourning dove
Zenaida macroura
mourning warbler
Geothlypis philadelphia
northern goshawk
Accipiter gentilis
northern harrier
Circus hudsonius
northern saw-whet owl
Aegolius acadicus
northern spotted owl
Strix occidentalis caurina
ovenbird
Seiurus aurocapilla
peregrine falcon
Falco peregrinus
plumbeous vireo
Vireo plumbeus
polar bear
Ursus maritimus
red-breasted nuthatch
Sitta canadensis
red-cockaded woodpecker
Leuconotopicus borealis
red-eyed vireo
Vireo olivaceus
rose-breasted grosbeak
Pheucticus ludovicianus
ruby-crowned kinglet
Corthylio calendula
sage thrasher
Oreoscoptes montanus
sagebrush sparrow
Artemisiospiza nevadensis
savannah sparrow
Passerculus sandwichensis
short-eared owl
Asio flammeus
spotted towhee
Pipilo maculatus
Sprague’s pipit
Anthus spragueii
Swainson’s thrush
Catharus ustulatus
Tennessee warbler
Leiothlypis peregrina
tree swallow
Tachycineta bicolor
vesper sparrow
Pooecetes gramineus
western bluebird
Sialia mexicana
western meadowlark
Sturnella neglecta
western tanager
Piranga ludoviciana
white-throated sparrow
Zonotrichia albicollis
winter wren
Troglodytes hiemalis
yellow warbler
Setophaga petechia
yellow-rumped warbler
Setophaga coronata
Publishing support provided by the Science Publishing Network,
Denver Publishing Service Center
For more information concerning the research in this report, contact the
Center Director, USGS Fort Collins Science Center
2150 Centre Ave., Bldg. C
Fort Collins, CO 80526-8118
(970) 226-9100
Or visit the Fort Collins Science Center website at:
Disclaimers
Any use of trade, firm, or product names is for descriptive purposes only and does not imply endorsement by the U.S. Government.
Although this information product, for the most part, is in the public domain, it also may contain copyrighted materials as noted in the text. Permission to reproduce copyrighted items must be secured from the copyright owner.
Suggested Citation
Maxwell, L.M., Rutherford, T.K., Kleist, N.J., Teige, E.C., Lehrter, R.J., Gilbert, M.A., Wood, D.J.A., Johnston, A.N., Tull, J.C., Haby, T.S., and Carter, S.K., 2024, Effects of noise from oil and gas development on raptors and songbirds—A science synthesis to inform National Environmental Policy Act analyses: U.S. Geological Survey Scientific Investigations Report 2024–5087, 66 p., https://doi.org/10.3133/sir20245087.
ISSN: 2328-0328 (online)
Publication type | Report |
---|---|
Publication Subtype | USGS Numbered Series |
Title | Effects of noise from oil and gas development on raptors and songbirds—A science synthesis to inform National Environmental Policy Act analyses |
Series title | Scientific Investigations Report |
Series number | 2024-5087 |
DOI | 10.3133/sir20245087 |
Year Published | 2024 |
Language | English |
Publisher | U.S. Geological Survey |
Publisher location | Reston, VA |
Contributing office(s) | Fort Collins Science Center |
Description | xi, 99 p. |
Online Only (Y/N) | Y |
Google Analytic Metrics | Metrics page |