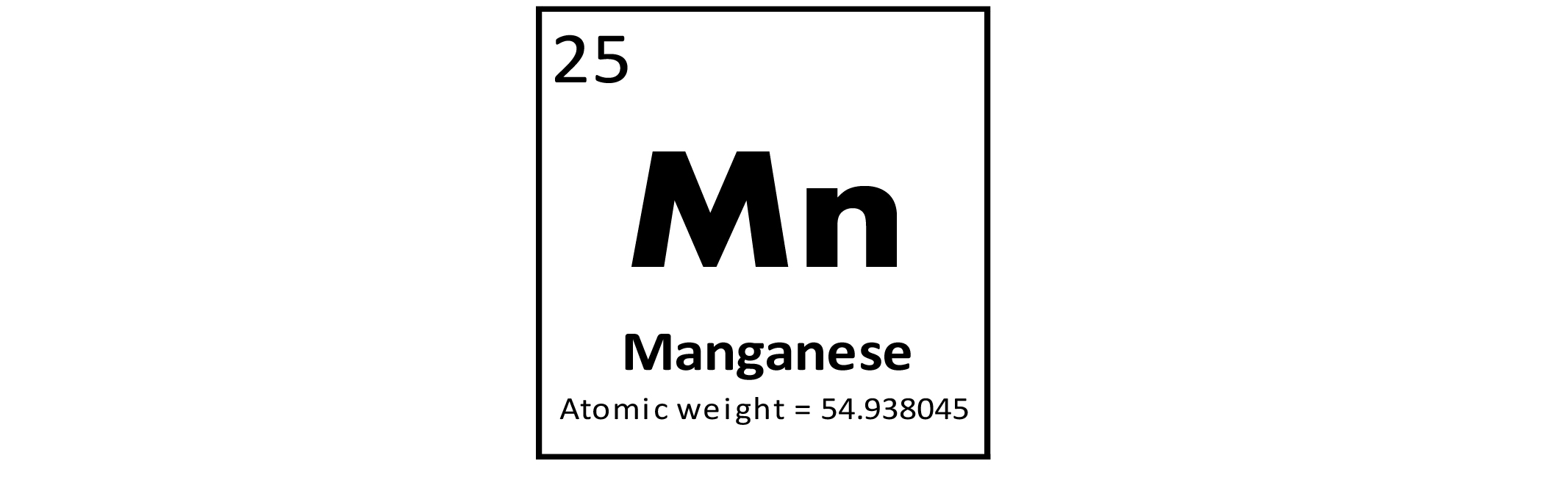
The purpose of these interpretive discussions is to provide a perspective on regional- and national-scale variations in element and mineral distributions in soils and their likely causes. The significant spatial variations shown by most elements and minerals can commonly be attributed to geologic sources in underlying parent materials, but other spatial variations seem clearly related to additional factors such as climate, the age of soils, transported source material, and anthropogenic influences. We attempt to distinguish the influence of these various factors on a regional and national scale. Numerous more local features might similarly be related to these same factors, but these features also have some probability of being an artifact of a random sampling of variable compositions, so that there is some probability of samples with similar compositions occurring in clusters of two or more adjacent sites by chance. Distinguishing such random occurrences from true variability is beyond the scope of the data from which these maps are constructed. Some caution, therefore, is advisable in interpreting the significance of these more local features unless some unique sources or processes can clearly be related to them.
Manganese (Mn) is a metallic element used primarily in alloys, such as steel. Manganese steel contains about 13 percent Mn and is extremely strong. It is used for railway tracks, safes, rifle barrels, and prison bars. Drink cans are made of an alloy of aluminum (Al) with 1.5 percent Mn to improve resistance to corrosion. Manganese is essential for all organisms and many enzymes contain Mn. Without it, bones grow spongier and break more easily. Manganese is often given as a food supplement to grazing animals. Both toxicity and deficiency of Mn are observed in plants. Toxic levels of Mn for humans are generally encountered only in industrial settings. More information about the toxicity of Mn (or other elements and substances) and its potential negative human health impacts can be found at the Agency for Toxic Substances and Disease Registry (ATSDR) website, or click to download a fact sheet about Mn.
Manganese can combine with oxygen, carbon, and silicon to form many different minerals. Pyrolusite (MnO2) is the most common Mn ore. An additional source of Mn is in iron (Fe)–Mn nodules that form on the seafloor in many parts of the world. Black "desert varnish" is a thin coating of Mn oxides present on rock exposures in arid environments. Native Americans produced artistic symbols known as petroglyphs by scraping through the desert varnish to expose the lighter colored rock on a black background. Manganese occurs in several common rock–forming minerals such as amphibole, pyroxene, olivine, garnet, mica, calcite, and dolomite.
The abundance of Mn in the Earth's upper continental crust is estimated to be approximately 774 milligrams per kilogram (mg/kg) (Rudnick and Gao, 2003). Manganese in trace quantities is present in a number of ferromagnesian silicate minerals common in ultramafic and mafic rocks, as well as more intermediate igneous rocks. Ultramafic rocks have an average Mn concentration of about 1,200 mg/kg and mafic rocks have an average Mn concentration of about 1,500 mg/kg. Manganese can form carbonate minerals and substitute for calcium (Ca) or magnesium (Mg) in calcite or dolomite. Limestone contains, on average, about 700 mg/kg Mn. Sandstone and shale have Mn average concentrations of 100 mg/kg and 850 mg/kg, respectively. Unconsolidated alluvial, eolian, and glacial deposits generally carry the Mn signature of the original source material.
Secondary Mn–bearing minerals (both carbonates and oxides) can form by hydrothermal or metamorphic processes; low–grade Mn deposits are present throughout the West and parts of the central and eastern United States. Manganese is an essential commodity. The distribution of mineral resource deposits with Mn as a commodity (major or minor) in the United States, extracted from the U.S. Geological Survey (USGS) Mineral Resource Data System (MRDS) website, can be seen by hovering the mouse here. Statistics and information on the worldwide supply of, demand for, and flow of Mn–bearing materials are available through the USGS National Minerals Information Center (NMIC) website.
Manganese, along with Fe, released by weathering of silicate or carbonate minerals during soil formation can form a variety of oxide and hydroxide minerals (referred to here as Mn oxides) that vary with soil environmental conditions, such as pH and redox state. These generally insoluble secondary Mn oxides can complex a number of different elements, including cadmium (Cd), cobalt (Co), copper (Cu), lead (Pb), nickel (Ni), phosphorus (P), and zinc (Zn) (Dixon and Weed, 1989). Thus, changing soil conditions that impact the solubility of Mn will also impact the behavior of other elements. Manganese also is an essential soil micronutrient. In its soluble form, Mn is easily taken up by plants and may be transferred to surface soils from deeper soils through plant uptake and plant residue cycling. Manganese fertilizer may be applied if agricultural soils are determined to be Mn deficient.
In our data, the median Mn concentration is 392 mg/kg in the soil C horizon, 498 mg/kg in the soil A horizon, and 492 mg/kg in the top 0- to 5-cm layer (see the summary statistics [open in new window]). Less than 0.5 percent of all samples have Mn concentrations less than the 5-mg/kg lower limit of determination (LLD). The broad spatial map patterns for Mn among the three sample types are generally similar to one another, with minor, somewhat local differences between topsoils (soil A horizon and top 0- to 5-cm layer) and the soil C horizon. Across the conterminous United States, there often is good correlation between Fe and Mn concentrations in soils, and some sites with elevated Mn concentrations may also contain detectable hematite (an Fe oxide mineral) and goethite (an Fe hydroxide mineral), suggesting that Mn concentration in those soils can be related to the presence of Fe–Mn oxides.
Examples of geographic areas that include ultramafic, mafic, or intermediate igneous rocks that potentially contribute Mn to soils include:
- Parts of the Southern Rocky Mountains (USDA, 2006), Colorado (a variety of rock types, including basalt and andesite);
- Mogollon Transition (USDA, 2006), Arizona and New Mexico (basalt and andesite, and related alluvium);
- Southern part of the Wasatch and Uinta Mountains (USDA, 2006), Utah (basalt and other volcanic rocks); and
- Trans–Pecos volcanics (Schruben and others, 1997), Texas (rhyolite, alkalic volcanic rocks, and related alluvium).
Soils with elevated Mn in the Northern Rocky Mountains (USDA, 2006) developed on varied sedimentary and igneous bedrock and related glacial deposits. In eastern Texas, soils developed on Fe–Mn–rich sandstone, siltstone, and claystone (bedrock unit called the Claiborne Group). In eastern Kansas, soils with high Mn developed on shale. In the Kentucky Bluegrass area and Nashville Basin (USDA, 2006), soils developed on weathered phosphatic limestone with reported Fe–Mn nodules and secondary Mn phosphates. Additional scattered occurrences of enriched Mn concentrations in soils are throughout the Central Allegheny Plateau (USDA, 2006) (West Virginia and western Pennsylvania) and the Glaciated Allegheny Plateau and Catskill Mountains (USDA, 2006) (northern Pennsylvania and southern New York), where soils developed on cyclic layers of sandstone, siltstone, and shale bedrock, and related unconsolidated material.
The distribution of Mn in soils of the Upper Midwest is controlled by glacial provenance. In the Upper Midwest, melting of glacial ice following late Wisconsinan period advances (16,000 to 12,000 years ago) left the region north of the southern glacial limit (Soller and others, 2012) mantled with a blanket of mixed, immature sediments, from which present–day soils developed. Individual ice lobes (Grimley, 2000) created distinct patterns in soil mineralogy and geochemistry because of varying provenance and ice transport paths. Elevated Mn concentrations are associated with 'gray' (carbonate– and shale–rich) till in the James and Des Moines lobes (Grimley, 2000) that was derived from Cretaceous sedimentary rocks (dolostone, limestone, and shale). In contrast, Mn concentrations are low in 'red' quartz– and feldspar–rich till deposited in central Minnesota, northern Wisconsin, and northwestern Michigan that has a Precambrian crystalline rock/red sandstone provenance with no material derived from carbonate bedrock. Till in northeastern Minnesota has a local Precambrian basalt and gabbro provenance (Duluth Complex), and Mn in soils is derived from ferromagnesian minerals rather than sedimentary rocks. Soils developed on calcareous till of the James lobe (Grimley, 2000) in central and eastern South Dakota have higher Mn (and strontium (Sr)) concentrations than soils in other areas of 'gray' till in western Minnesota, although the two areas have similar glacial provenance.
Examples of areas with relatively low concentrations of Mn in soil, likely because of a dearth of Mn in soil parent materials are:
- Colorado Plateau (USDA, 2006) (quartz–rich sandstone and eolian sands);
- Nebraska Sand Hills (USDA, 2006) (soils dominated by quartz and plagioclase feldspar in an area of unconsolidated sand dunes and sand sheets);
- Parts of the Southern High Plains (USDA, 2006) of eastern New Mexico and northwestern Texas (quartz–rich eolian sands and alluvial sediments);
- Parts of the Piedmont (Fenneman and Johnson, 1946) (intensely weathered Precambrian and younger metamorphic and igneous rocks); and
- Gulf and Atlantic Coastal Plain (Fenneman and Johnson, 1946) (quartz–rich sedimentary rocks and unconsolidated sediments).
The Gulf and Atlantic Coastal Plain is bisected by the Southern Mississippi River Alluvium and the Southern Mississippi Valley Loess (USDA, 2006). Alluvial sediments have deposited in the Mississippi River valley as the river flooded in recent geologic time. When these sediments dried, winds picked up the fine material and deposited it in thick loess sheets, mainly along the east side of the river valley. The youngest loess sheets are about 10,000 years old. A pattern of higher Mn in soils developed on these young sediments reflects long–range transport of Mn–bearing materials (likely oxide coatings on particles) from the upper part of the Mississippi River drainage basin.
When comparing the map patterns for the three sample types, the authors noted some differences in Mn concentrations where topsoils may be enriched in Mn compared to deeper soils, and vice versa. The many processes affecting Mn in a soil profile, including release of soluble anions by weathering and potential Mn fixing as insoluble oxide minerals, plant uptake and cycling transport to surface soils and possible application of Mn fertilizers make it difficult to generalize about Mn differences among the three sample types.
Statistics - 0 TO 5 CM
Number of samples | 4,841 |
LLD | 5 mg/kg |
Number below LLD | 16 |
Minimum | <5 mg/kg |
5 percentile | 79 mg/kg |
25 percentile | 290 mg/kg |
50 percentile | 492 mg/kg |
75 percentile | 791 mg/kg |
95 percentile | 1,520 mg/kg |
Maximum | 7,780 mg/kg |
MAD | 353 mg/kg |
Robust CV | 71.7% |
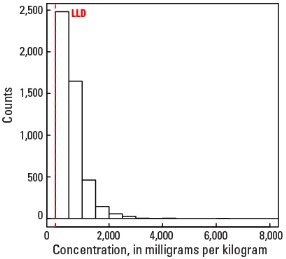
Histogram
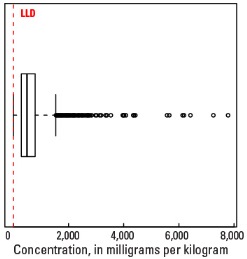
Boxplot
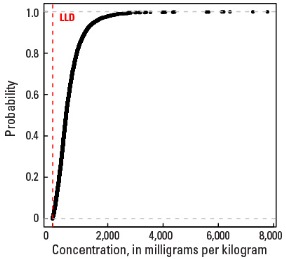
Empirical cumulative distribution function
Statistics - A Horizon
Number of samples | 4,813 |
LLD | 5 mg/kg |
Number below LLD | 14 |
Minimum | <5 mg/kg |
5 percentile | 73 mg/kg |
25 percentile | 289 mg/kg |
50 percentile | 498 mg/kg |
75 percentile | 796 mg/kg |
95 percentile | 1,580 mg/kg |
Maximum | 6,850 mg/kg |
MAD | 365 mg/kg |
Robust CV | 73.2 % |
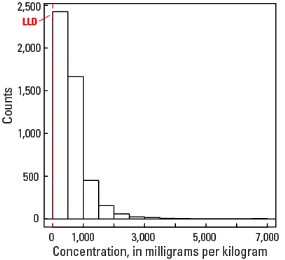
Histogram
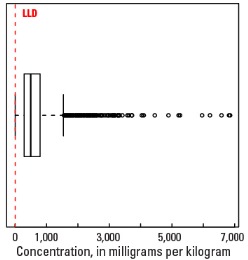
Boxplot
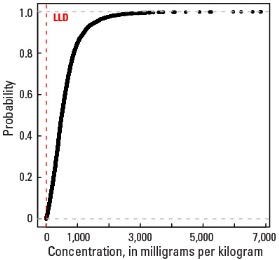
Empirical cumulative distribution function
Statistics - C Horizon
Number of samples | 4,780 |
LLD | 5 mg/kg |
Number below LLD | 23 |
Minimum | <5 mg/kg |
5 percentile | 45 mg/kg |
25 percentile | 202 mg/kg |
50 percentile | 392 mg/kg |
75 percentile | 646 mg/kg |
95 percentile | 1,240 mg/kg |
Maximum | 12,000 mg/kg |
MAD | 322 mg/kg |
Robust CV | 82.2 % |
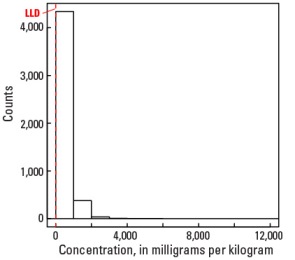
Histogram
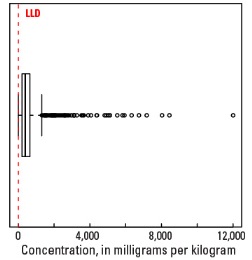
Boxplot
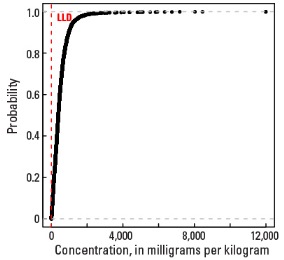